Sulfuric acid-modified PEG-6000 (PEG-OSO3H): an efficient Brönsted acid–surfactant combined catalyst for the one-pot three component synthesis of α-aminonitriles in water
Received
30th November 2011
, Accepted 1st February 2012
First published on 6th February 2012
Introduction
One of the fundamental aspects of Green Chemistry is linked to the number of steps in organic synthesis as well as atom economy. Multi-component reactions (MCRs) are thus becoming a more and more important class of reactions since they allow combining several starting materials in a single compound and in one-flask operation and have become a significant part of today's arsenal of methods in combinatorial chemistry due to their valued features such as atom economy, straightforward reaction design, and the opportunity to construct target compounds by the introduction of several diversity elements in a single chemical event.1 Moreover, MCRs offer the advantage of simplicity and synthetic efficiency over conventional chemical reactions.1 One of the most important multi-component reactions is the one-pot three component synthesis of α-aminonitriles.
The addition of cyanide anions to imines (the Strecker reaction)2 provides one of the most important and straightforward methods for the synthesis of α-aminonitriles, which are useful intermediates for the synthesis of amino acids3,4 and nitrogen containing heterocycles such as thiadiazoles and imidazoles5,6 and other biologically useful molecules such as saframycin A, a natural product with anti-tumour activity, or phthalascidi, a synthetic analogue, which exhibits even greater potency.7 Several modifications of Strecker reaction have been reported using a variety of cyanide reagents, such as alkaline cyanides2,8,9 diethylphosphoro cyanidate10 Bu3SnCN,11 and Et2AlCN,12 as well as catalysts such as InCl3,13 BiCl3,14 montmorillonite KSF clay,15 silica-based scandium(III),16 SO42−/ZrO2,17 ferric perchlorate,18 Fe(Cp)2PF6,19 InI3,20 I2,21 K5CoW12O40·3H2O,22 vanadyl triflate,23 Fe3O4,24 guanidine hydrochloride,25 xanthan sulfuric acid,26 [bmim]BF4,27 silica sulfuric acid,28 hydrophobic sulfonic acid based nanoreactors29 and silica-bonded S-sulfonic acid30 under various reaction conditions. However, many of these methods involve the use of stoichiometric reagents, low yields of the products, the use of hazardous and often expensive catalysts, harsh reaction conditions, extended reaction times, tedious procedure for the preparation of catalysts, the use of volatile and hazardous organic solvents, no compliance with the green chemistry protocols and also require tedious workup leading to the generation of a large amount of toxic waste. Furthermore many of these catalysts are deactivated or sometimes decomposed by amines and water that exist during imine formation. Also it has been shown that trimethylsilyl cyanide (TMSCN) is a very effective, relatively safe and easy-to-handle cyanide source for this purpose.31–38 Consequently, development of general, efficient, inexpensive and environmentally benign methods for the synthesis of α-aminonitriles is in demand.
The development of simple and efficient chemical processes or methodologies for the synthesis of biologically active compounds in water is one of the major challenges for chemists, although water is a safe, very cheap, readily available, and environmentally benign solvent.39 Although today's environmental consciousness imposes the use of water as a solvent on both industrial and academic chemists, organic solvents are still used instead of water for mainly two reasons. First, most organic substances are insoluble in water, and as a result, water does not function as a reaction medium. Second, many reactive substrates, reagents, and catalysts are decomposed or deactivated by water. Some of these problems were solved with the discovery of surfactant combined catalysts by Kobayashi et al.40
Besides this, the utility of polymer-supported catalysts is now well recognized because of their ease of workup and separation of products and catalysts, from the economical point of view, and in application to industrial processes.41 Most polymers of this type have high molecular weights and unique solubility profile. Among them, PEGs with Mw > 2000 Da are mostly soluble in many polar solvents and insoluble in a few nonpolar solvents. Because of this solubility profile, PEG-based supports combine the advantageous features of homogeneous catalysis such as high reactivity, lack of diffusion phenomena and analytical simplicity, as well as solid phase methods.42 Sulfuric acid-modified PEG-6000 (PEG-OSO3H) is an example of polyethylene glycol supported catalyst that is functionalized by acidic groups and is a mild, non-volatile and non-corrosive organic acid. Moreover, because of surfactant behaviour of the ethylene glycol skeleton, PEG-OSO3H can be used as a Brönsted acid–surfactant combined catalyst for the synthesis of organic compounds in water.43,48 This catalyst has been used for the synthesis of 3,4-dihydropyrimidones via the Biginelli reaction under microwave and solvent-free conditions,44 regioselective ring opening of epoxides with thiocyanate anion in water,43 Beckmann rearrangement and dehydration of oximes,45 solvent-free synthesis of triazolo[1,2-a]indazole-triones and novel spiro triazolo[1,2-a]indazole-tetraones,46 synthesis of poly-substituted quinolines under microwave irradiation47 and synthesis of bis(indolyl)methanes and 4,4′-(arylmethylene)-bis(3-methyl-1-phenyl-1H-pyrazol-5-ol) derivatives in water.48
Considering the above subjects and along with our previous studies on application of PEG-OSO3H in organic synthesis,46–48 we wish to report a convenient and rapid method for the synthesis of α-aminonitriles via a one-pot three component condensation reaction between aldehydes or ketones (1), amines (2) and trimethylsilyl cyanide (TMSCN) (3) in the presence of PEG-OSO3H as an efficient, green, inexpensive, reusable and biodegradable polymeric catalyst in water at room temperature (Scheme 1).
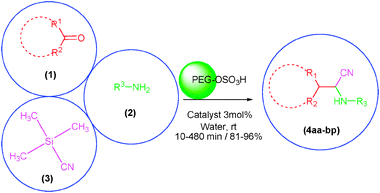 |
| Scheme 1 The one-pot three component synthesis of α-aminonitriles in the presence of PEG-OSO3H in water at room temperature. | |
Results and discussions
In the first step to find the best reaction conditions for the synthesis of α-aminonitrile derivatives, the one-pot three component condensation of benzaldehyde (1a) (1 mmol), aniline (2a) (1.2 mmol) and TMSCN (3) (1.2 mmol) was examined in the presence of different amounts of PEG-OSO3H under various reaction conditions and the obtained results are summarized in Table 1 (Scheme 2).
Table 1 The one-pot three component condensation reaction of benzaldehyde (1a) (1 mmol), aniline (2a) (1.2 mmol) and TMSCN (3) (1.2 mmol) in the presence of PEG-OSO3H under various reaction conditions
Entry |
Solvent (mL) |
Cat. (mol%) |
Temp./°C |
Time/min |
Yielda (%) |
Yields refer to isolated pure products.
|
1 |
CH2Cl2 (3) |
3 |
rt |
60 |
35 |
2 |
DMF (3) |
3 |
rt |
60 |
62 |
3 |
CH3CN (3) |
3 |
rt |
60 |
55 |
4 |
THF (3) |
3 |
rt |
60 |
32 |
5 |
EtOH (3) |
3 |
rt |
45 |
89 |
6 |
H2O (3) |
3 |
rt |
10 |
91 |
7 |
H2O (3) |
3 |
50 |
10 |
91 |
8 |
H2O (3) |
3 |
70 |
8 |
93 |
9 |
H2O (3) |
1 |
rt |
60 |
88 |
10 |
H2O (3) |
5 |
rt |
10 |
91 |
11 |
H2O (1) |
3 |
rt |
35 |
86 |
12 |
H2O (5) |
3 |
rt |
10 |
91 |
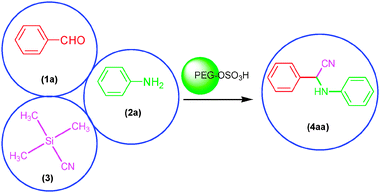 |
| Scheme 2 The one-pot three component condensation reaction of benzaldehyde (1a) (1 mmol), aniline (2a) (1.2 mmol) and TMSCN (3) (1.2 mmol) in the presence of PEG-OSO3H under various reaction conditions. | |
As shown in Table 1, the best results were obtained in the presence of 3 mol% of catalyst in water (3 mL) as a solvent. However increasing the amount of water has no effect on reaction yield and time, but decreasing the amount of solvent redounds to the increase in the reaction time due to the formation of insoluble products that make the stirring of the reaction mixture difficult. Although the reaction rate increases along with increasing temperature, we found that the reaction rate is also acceptable and in a good range at room temperature. As a major adverse effect on the environment for many chemical processes is the consumption of energy for heating and cooling, room temperature synthesis of chemical compounds is a useful strategy to overcome this problem. So the room temperature was selected as the best. The reaction was also checked without the catalyst and it was found that the reaction did not proceed even after 12 h. These observations established the crucial role of PEG-OSO3H in the expedition of the reaction time and the product yield. In another study, the model reaction was examined in the presence of H2SO4 (3 mol%) in water, in which a highly sticky reaction mixture was obtained and the reaction time was increased (12 h) and the yield was decreased extremely (31%). The reaction yield was improved (88%) and the reaction time was decreased (10 min) when H2SO4 (3 mol%) was applied in combination with PEG-6000 (3 mol%). These observations illustrate the surfactant role of the PEG skeleton of PEG-OSO3H.
The scope and efficiency of the process was investigated under optimized conditions. For this purpose, a broad range of structurally diverse aldehydes (aliphatic and aromatic) as well as ketones and amines (aliphatic or aromatic) were condensed with TMSCN. The results are displayed in Table 2.
Table 2 The one-pot three component synthesis of α-aminonitriles in the presence of PEG-OSO3H in water at room temperature
Entry |
Aldehyde or ketone |
Amine |
Product |
Time/min |
Yielda (%) |
Yields refer to isolated pure products.
|
4aa
|
|
|
|
10 |
91 |
4ab
|
|
|
|
15 |
93 |
4ac
|
|
|
|
10 |
94 |
4ad
|
|
|
|
10 |
93 |
4ae
|
|
|
|
60 |
91 |
4af
|
|
|
|
40 |
92 |
4ag
|
|
|
|
60 |
92 |
4ah
|
|
|
|
50 |
85 |
4ai
|
|
|
|
75 |
89 |
4aj
|
|
|
|
15 |
93 |
4ak
|
|
|
|
60 |
92 |
4al
|
|
|
|
10 |
92 |
4am
|
|
|
|
10 |
92 |
4an
|
|
|
|
10 |
93 |
4ao
|
|
|
|
10 |
93 |
4ap
|
|
|
|
50 |
92 |
4aq
|
|
|
|
75 |
91 |
4ar
|
|
|
|
90 |
87 |
4as
|
|
|
|
50 |
92 |
4at
|
|
|
|
5 |
96 |
4au
|
|
|
|
70 |
93 |
4av
|
|
|
|
70 |
91 |
4aw
|
|
|
|
90 |
83 |
4ax
|
|
|
|
25 |
90 |
4ay
|
|
|
|
90 |
89 |
4az
|
|
|
|
60 |
83 |
4ba
|
|
|
|
140 |
84 |
4bb
|
|
|
|
180 |
83 |
4bc
|
|
|
|
240 |
85 |
4bd
|
|
|
|
360 |
89 |
4be
|
|
|
|
240 |
86 |
4bf
|
|
|
|
240 |
83 |
4bg
|
|
|
|
380 |
81 |
4bh
|
|
|
|
380 |
85 |
4bi
|
|
|
|
300 |
89 |
4bj
|
|
|
|
360 |
90 |
4bk
|
|
|
|
240 |
91 |
4bl
|
|
|
|
450 |
79 |
4bm
|
|
|
|
450 |
82 |
4bn
|
|
|
|
15 |
91 |
4bo
|
|
|
|
10 |
93 |
4bp
|
|
|
|
480 |
87 |
As can be seen from Table 2, all reactions proceeded efficiently and the desired products were produced in good to excellent yields in relatively short reaction times without formation of any by-products. Aromatic aldehydes having electron withdrawing groups (Table 2, entries 4an, 4ao and 4at) reacted at a faster rate compared with those substituted with electron releasing groups (Table 2, entries 4aq, 4ar and 4as). Besides, our methodology has been used successfully for heteroaromatic aldehydes, and corresponding α-aminonitriles were obtained in excellent yields and without any byproduct (Table 2, entries 4au, 4av and 4aw). As is clear from the obtained results, the present methodology can be used in the order of oxygen, sulfur and nitrogen containing heteroaromatic aldehydes. Interestingly, cyclic ketones such as cyclopentanone, cyclohexanone and cycloheptanone, as well as aryl–alkyl ketones, diaryl ketones and tetralones were successfully reacted with amines and TMSCN to afford the respective α-aminonitriles in reasonable yields and in short reaction times (Table 2, entries 4bc–4bm and 4bp). In the case of aliphatic amines, relatively slow reaction rate occurred due to the unstable nature of the formed aliphatic imines in the presence of water (Table 2, entries 4ae, 4af, 4aq, 4ah, 4ai, 4ar, 4au, 4av and 4aw).
Indole and indoline derivatives are important classes of nitrogen containing heterocycles and have attracted attention because of their applications in dyes and pharmaceuticals and have been used as building blocks for the synthesis of organic semiconductors.49 Considering these facts new α-aminonitriles bearing an indoline moiety were synthesized via a one-pot three component condensation reaction between isatin derivatives (5), amines and TMSCN in water in the presence of PEG-OSO4H at room temperature. Besides, acenaphthenequinone (6) was applied successfully for the first time and desired α-aminonitriles were obtained in excellent yields and relatively short reaction times and obtained results are summarized in Table 3 (Scheme 3).
Entry |
Carbonyl compound |
Amine |
Product |
Time/min |
Yielda (%) |
Yields refer to isolated pure products.
|
7aa
|
|
|
|
8 |
89 |
7ab
|
|
|
|
6 |
92 |
7ac
|
|
|
|
8 |
90 |
7ad
|
|
|
|
8 |
94 |
7ae
|
|
|
|
10 |
94 |
7af
|
|
|
|
8 |
91 |
7ag
|
|
|
|
10 |
93 |
To assess the capability and efficiency of our catalyst with respect to the reported catalysts for the synthesis of α-aminonitrile derivatives, the results of the application of these catalysts are tabulated in Table 4. As is clear from Table 4, PEG-OSO3H was more efficient.
Table 4 Comparison of the condensation of benzaldehyde (1a) and aniline (2a) with TMSCN (3) using the reported methods versus the present method
Entry |
Conditions [ref.] |
Time/min |
Yield (%) |
1 |
InCl3 (20 mol%), dry THF, rt37 |
360 |
75 |
2 |
BiCl3 (10 mol%), CH3CN, rt38 |
600 |
84 |
3 |
Montmorillonite KSF clay, 1.0 g, CH2Cl2, rt39 |
210 |
90 |
4 |
Silica-based scandium(III) (3 mol%), CH2Cl2, rt40 |
840 |
94 |
5 |
SO42−/ZrO2 (10 mol%), dry THF, N2 atmosphere, rt41 |
90 |
93 |
6 |
Guanidine hydrochloride (3 mol%), EtOH, 40 °C49 |
60 |
94 |
7 |
Xanthane sulfuric acid (6 mol%), dry CH3CN, rt50 |
65 |
97 |
8 |
Silica sulfuric acid (25 mol%), CH2Cl2, rt52 |
360 |
88 |
9 |
Silica bonded S-sulfonic acid (6.6 mol%), EtOH, rt30 |
30 |
94 |
10 |
PEG-OSO3H (3 mol%), water, rt [this work] |
10 |
91 |
The possibility of recycling the catalyst was examined using the reaction of benzaldehyde (1a) and aniline (2a) with TMSCN (3) under optimized conditions. Upon completion, ethyl acetate (5 mL) was added to the reaction mixture, shaked thoroughly, organic layer was washed with brine (5 mL), dried (MgSO4), and concentrated. In order to recover the catalyst, H2O was evaporated under reduced pressure, and the resulting solid was washed with t-butylmethyl ether, and dried. The recovered catalyst was reused fifteen times in the condensation of benzaldehyde (1a) and aniline (2a) with TMSCN (3) and smooth loss of catalytic activity was observed from the 12th time of reuse (Fig. 1).
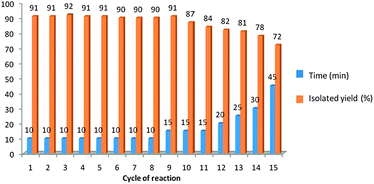 |
| Fig. 1 The catalytic activity of PEG-OSO3H in fifteen cycles for the reaction of benzaldehyde (1a) and aniline (2a) with TMSCN (3). | |
In another study, to recognize the applicability of our method at large scales, we examined some reactions in scales of 25 and 50 mmol. The results are summarized in Table 5. As shown in Table 5, the reactions were successfully performed at large scales without significant loss of yields.
Table 5 The large scale one-pot three component synthesis of α-aminonitriles using PEG-OSO3H in water at room temperature
Amounts of carbonyl compound/mmol |
Product |
Time/min |
Yielda (%) |
Yields refer to isolated pure products.
|
1 |
4aa
|
10 |
91 |
25 |
4aa
|
50 |
80 |
50 |
4aa
|
170 |
78 |
1 |
4ag
|
60 |
92 |
25 |
4ag
|
110 |
85 |
50 |
4ag
|
290 |
77 |
1 |
4bc
|
240 |
85 |
25 |
4bc
|
380 |
80 |
50 |
4bc
|
510 |
73 |
1 |
7aa
|
20 |
89 |
25 |
7aa
|
70 |
81 |
50 |
7aa
|
180 |
79 |
We observed considerable effects on the rate of reaction, obtained yield, and simplicity of the procedure using water compared to other solvents such as CH2Cl2, CH3CN, DMF, and THF in the Strecker reaction. This may be attributed mainly to the insolubility of the products in water. Furthermore, TMSCN itself can be activated by water as a Lewis base (Scheme 4). The latter property may be anticipated for solvents such as DMF and THF in lower intensities. Indeed, products precipitated out of the reaction mixture and therefore afforded higher yields. To investigate the reaction mechanism, progress of the reaction was monitored by TLC using authentic samples of corresponding cyanohydrin trimethylsilyl ethers (8)50 and imines (9)51 in the reaction of benzaldehyde (1a) with aniline (2a). It was found that cyanohydrin trimethylsilyl ether products (8) were not observed and the reaction proceeds through the formation of the imine intermediate. However, some (E)- and (Z)-isomers of the corresponding imine were observed, which were completely converted to the desired products (4aa) after the times indicated in Table 2. In an additional experiment, the corresponding imine of the model reaction was treated with TMSCN (3) under the optimal reaction conditions. It was found that the desired product (4aa) was obtained quantitatively (91%) after 5 min. These findings along with substituent effects all demonstrate that formation of the imine intermediate is the key step in the one-pot three-component Strecker reaction catalyzed by PEG-SO3H (Scheme 4).
Conclusions
In summary, we have reported a highly efficient and green method for the one-pot three component synthesis of α-aminonitriles using PEG-OSO3H as an inexpensive, biodegradable and reusable Brönsted acid–surfactant combined catalyst. This method not only offers substantial improvements in the reaction rates and yields, but also avoids the use of hazardous catalysts or solvents. The promising points for the presented methodology are its efficiency, generality, high yield, short reaction time, ease of handling of the catalyst, cleaner reaction profile, ease of product isolation, simplicity, potential for recycling of the catalyst, and finally agreement with the green chemistry protocols, which all make it a useful and attractive process for the synthesis of α-aminonitrile derivatives.
Experimental
All chemicals were purchased from Merck or Fluka Chemical Companies. All known compounds were identified by comparison of their melting points and 1H NMR data with those in the authentic samples. The 1H NMR (500 MHz) and 13C NMR (125 MHz) were run on a Bruker Avance DPX-250, FT-NMR spectrometer. Microanalysis was performed on a Perkin-Elmer 240-B microanalyzer. Melting points were recorded on a Stuart Scientific Apparatus SMP3 (UK) in open capillary tubes.
Preparation of PEG-OSO3H
At 0 °C, chlorosulfonic acid (1.16 g, 10 mmol) was added to a solution of PEG-6000 (6.0 g, 1 mmol) in dried CH2Cl2 (10 mL). Then the resulting solution was stirred at room temperature overnight, and the solution was concentrated under vacuum. Appropriate t-butylmethyl ether (50 mL) was added, and the precipitate was filtered and washed with t-butylmethyl ether (30 mL) three times to afford the PEG-OSO3H as a white solid (98%, 6.03 g).441H NMR (CDCl3, 500 MHz): d (ppm) 3.40–3.54 (m, PEG), 4.18 (s, 2H, CH2OSO3H), 12.69 (s, 1H, SO3H).
General procedure for the synthesis of α-aminonitriles
Caution: TMSCN is very toxic and could produce hydrogen cyanide when in contact with water. Though there are various reports of safe application of TMSCN in protic solvents (water and ethanol)20,52 all operations should be performed with special care under a well-ventilated fume hood.
A well-ground mixture of aldehyde or ketone (1 mmol) and amine (1.2 mmol) was added to a solution of PEG-OSO3H (0.18 g, 0.03 mmol, 3 mol%) in water (3 mL), and the resulting mixture was stirred vigorously at room temperature for 2 minutes. TMSCN (1.2 mmol) was added and the completion of reaction was followed by thin layer chromatography. The insoluble crude products were filtered and recrystallized from EtOH/H2O 4
:
1. In the case of oil products, appropriate amounts of t-butylmethyl ether (5 mL, two times) were added; the organic layer was washed with brine (5 mL), dried (MgSO4), and concentrated. The residue was chromatographed over silica gel (15% ethyl acetate in hexane) to give a pure product. In order to recover the catalyst, H2O was evaporated under reduced pressure; the resulting solid was washed with t-butylmethyl ether (2 mL) and dried. The recovered PEG-OSO3H was reused for another time without loss of its activity.
General procedure for the synthesis of α-aminonitriles in large scale
Caution: TMSCN is very toxic and could produce hydrogen cyanide when in contact with water. Though there are various reports of safe application of TMSCN in protic solvents (water and ethanol)20,52 all operations should be performed with special care under a well-ventilated fume hood.
A well-ground mixture of aldehyde or ketone (50 mmol) and amine (60 mmol) was added to a solution of PEG-OSO3H (9.24 g, 1.5 mmol, 3 mol%) in water (150 mL), and the resulting mixture was stirred vigorously at room temperature for 5 minutes. TMSCN (60 mmol) was added and the completion of reaction was followed by thin layer chromatography. The insoluble crude products were filtered and recrystallized from EtOH/H2O 4
:
1 (60 mL). In the case of oil products, appropriate amounts of t-butylmethyl ether (300 mL, two times) were added; the organic layer was washed with brine (300 mL), dried (MgSO4), and concentrated. The residue was chromatographed over silica gel (15% ethyl acetate in hexane) to give a pure product. In order to recover the catalyst, H2O was evaporated under reduced pressure; the resulting solid was washed with t-butylmethyl ether (110 mL) and dried. The recovered PEG-OSO3H was reused for another time without loss of its activity.
Selected spectral data of the products
2-Phenyl-2-(phenylamino)acetonitrile (compound 4aa).
White crystalline solid, mp: 71–72 °C (73–74 °C),15νmax (KBr): 3367, 3029, 2954, 2230 cm−1. 1H NMR (500 MHz, CDCl3): δ (ppm) 4.08 (br s, 1H), 5.47 (s, 1H), 6.67 (d, J = 8.1 Hz, 2H), 6.90 (t, J = 7.9 Hz, 1H), 7.21 (t, J = 7.9 Hz, 2H), 7.41–7.53 (m, 3H), 7.59–7.67 (m, 2H). 13C NMR (125 MHz, CDCl3): δ (ppm) 50.3, 114.1, 118.7, 120.5, 127.8, 129.9, 130.1, 130.3, 134.3, 145.4. Anal. calcd for C14H12N2: C, 80.74; H, 5.81; N, 13.45%. Found: C, 80.70; H, 5.62; N, 13.61%.
2-(3,4-Dimethylphenylamino)-2-phenylacetonitrile (compound 4ab).
White crystalline solid, mp: 93–95 °C (92–94 °C),30νmax (KBr): 3332, 3025, 2925, 2229 cm−1. 1H NMR (500 MHz, CDCl3): δ (ppm) 2.09 (s, 3H), 2.17 (s, 3H), 3.92 (br s, 1H), 5.37 (s, 1H), 6.44 (dd, J = 8.0, 2.5 Hz, 1H), 6.56 (s, 1H), 6.85 (d, J = 8.0 Hz, 1H), 7.33–7.39 (m, 3H), 7.50–7.54 (m, 2H). 13C NMR (125 MHz, CDCl3): δ (ppm) 18.8, 21.1, 50.8, 111.6, 116.0, 118.1, 126.9, 128.8, 129.2, 129.4, 130.5, 134.0, 137.8, 142.5. Anal. calcd for C16H16N2: C, 81.32; H, 6.82; N, 11.85%. Found: C, 81.30; H, 6.85; N, 11.84%.
2-(4-Bromophenylamino)-2-phenylacetonitrile (compound 4ac).
White crystalline solid, mp: 90–92 °C (91–93 °C),30νmax (KBr): 3337, 3021, 2928, 2235 cm−1. 1H NMR (500 MHz, CDCl3): δ (ppm) 3.99 (s, 1H), 5.39 (s, 1H), 6.55 (d, J = 8.7 Hz, 2H), 7.29 (d, J = 8.7 Hz, 2H), 7.34–7.39 (m, 3H), 7.50–7.54 (m, 2H). 13C NMR (125 MHz, CDCl3): δ (ppm) 50.0, 112.3, 115.5, 117.6, 127.1, 129.0, 129.5, 132.4, 133.3, 143.9. Anal. calcd for C14H11BrN2: C, 58.56; H, 3.86; N, 9.76%. Found: C, 58.49; H, 3.79; N, 9.70%.
2-(p-Tolylamino)-2-phenylacetonitrile (compound 4ad).
White crystalline solid, mp: 103–104 °C (102–104 °C),30νmax (KBr): 3330, 3020, 2910, 2234 cm−1. 1H NMR (500 MHz, CDCl3): δ (ppm) 2.35 (s, 3H), 3.91 (br s, 1H), 5.44 (s, 1H), 6.71 (d, J = 8.0 Hz, 2H), 7.11 (d, J = 8.0 Hz, 2H), 7.45–7.51 (m, 3H), 7.64–7.68 (m, 2H). 13C NMR (125 MHz, CDCl3): δ (ppm) 20.4, 50.6, 114.4, 127.2, 127.9, 129.2, 129.8, 129.9, 130.0, 134.6, 142.1. Anal. calcd for C15H14N2: C, 81.05; H, 6.35; N, 12.60%. Found: C, 81. 33; H, 6.64; N, 12.98%.
2-Morpholino-2-phenylacetonitrile (compound 4ae).
White crystalline solid, mp: 70–72 °C (69–71 °C),30νmax (KBr): 3019, 2921, 2234 cm−1. 1H NMR (500 MHz, CDCl3): δ (ppm) 2.61 (t, J = 4.5 Hz, 4H), 3.70–3.82 (m, 4H), 4.72 (s, 1H), 7.41–7.53 (m, 3H), 7.56–7.59 (m, 2H). 13C NMR (125 MHz, CDCl3): δ (ppm) 50.0, 62.3, 67.0, 115.1, 128.0, 128.5, 129.0, 132.3. Anal. calcd for C12H14N2O: C, 71.26; H, 6.98; N, 13.85%. Found: C, 71.50; H, 7.27; N, 14.04%.
2-(Benzylamino)-2-phenylacetonitrile (compound 4af).
Colorless oil (colorless oil),15νmax (neat): 3410, 3019, 2985, 2229 cm−1. 1H NMR (500 MHz, CDCl3): δ (ppm) 1.99 (br s, 1H), 3.85 (AB System, J = 13.7 Hz, 1H), 3.91 (AB System, J = 13.7 Hz, 1H), 4.78 (s, 1H), 6.71 (d, J = 8.0 Hz, 1H), 7.10 (t, J = 7.9 Hz, 1H), 7.28–7.43 (m, 6H), 7.48–7.50 (m, 2H). 13C NMR (125 MHz, CDCl3): δ (ppm) 51.2, 53.9, 119.0, 128.0, 128.9, 129.3, 129.7, 130.1, 135.9, 138.2, 143.9. Anal. calcd for C15H14N2: C, 81.05; H, 6.35; N, 12.60%. Found: C, 81.09; H, 6.34; N, 12.55%.
2-(Butylamino)-2-phenylacetonitrile (compound 4ag).
Colorless oil (colorless oil),13νmax (neat): 3375, 3012, 2950, 2215 cm−1. 1H NMR (500 MHz, CDCl3): δ (ppm) 0.89 (t, J = 7.0 Hz, 3H), 1.25–1.50 (m, 5H), 2.85 (m, 2H), 4.81 (s, 1H), 7.36 (m, 3H), 7.66–7.79 (m, 2H). 13C NMR (125 MHz, CDCl3): δ (ppm) 13.9, 19.2, 31.8, 45.0, 52.1, 119.3, 127.6, 129.2, 129.8, 134.6. Anal. calcd for C12H16N2: C, 76.55; H, 8.57; N, 14.88%. Found: C, 76.38; H, 8.42; N, 14.76%.
2-(Isopropylamino)-2-phenylacetonitrile (compound 4ah).
Colorless oil (colorless oil),13νmax (neat): 3325, 3018, 2979, 2211 cm−1. 1H NMR (500 MHz, CDCl3): δ (ppm) 1.09 (d, J = 6.9 Hz, 6H), 2.91 (m, 1H), 3.18 (s, 1H), 4.86 (s, 1H), 7.31–7.34 (m, 3H), 7.44–7.50 (m, 2H). 13C NMR (125 MHz, CDCl3): δ (ppm) 20.1, 20.9, 46.9, 52.1, 116.3, 126.9, 129.0, 129.4, 135.5. Anal. calcd for C11H14N2: C, 75.82; H, 8.10; N, 16.08%. Found: C, 75.54; H, 8.04; N, 15.88%.
2-Phenyl-2-(pyrrolidin-1-yl)acetonitrile (compound 4ai).
Colorless oil (colorless oil),13νmax (neat): 3018, 2985, 2224 cm−1. 1H NMR (500 MHz, CDCl3): δ (ppm) 1.63–1.76 (m, 4H), 2.48–2.79 (m, 4H), 4.98 (s, 1H), 7.37–7.48 (m, 3H), 7.51–7.54 (m, 2H), 7. 13C NMR (125 MHz, CDCl3): δ (ppm) 24.1, 50.2, 58.6, 116.1, 128.0, 129.2, 129.3, 135.1. Anal. calcd for C12H14N2: C, 77.38; H, 7.58; N, 15.04%. Found: C, 77.19; H, 7.45; N, 15.10%.
2-(p-Tolylamino)-2-(4-chlorophenyl)acetonitrile (compound 4al).
White crystalline solid, mp: 83–85 °C (85–87 °C),30νmax (KBr): 3339, 3019, 2920, 2229 cm−1. 1H NMR (500 MHz, CDCl3): δ (ppm) 2.33 (s, 3H), 3.95 (s, 1H), 5.39 (s, 1H), 6.78 (d, J = 8.5 Hz, 2H), 7.10 (d, J = 8.0 Hz, 2H), 7.45 (d, J = 8.5 Hz, 2H), 7.56 (d, J = 8.5 Hz, 2H). 13C NMR (125 MHz, CDCl3): δ (ppm) 21.0, 50.2, 114.6, 118.0, 128.3, 130.0, 130.1, 132.5, 135.9, 142.1. Anal. calcd for C15H13ClN2: C, 70.18; H, 5.10; N, 10.91%. Found: C, 70.09; H, 5.05; N, 10.88%.
2-(4-Fluorophenyl)-2-(phenylamino)acetonitrile (compound 4an).
White crystalline solid, mp: 100–102 °C (99–101 °C),30νmax (KBr): 3300, 3018, 2959, 2231 cm−1. 1H NMR (500 MHz, CDCl3): δ (ppm) 3.92 (br s, 1H), 5.41 (s, 1H), 6.70 (dd, J = 8.6, 1.0 Hz, 2H), 6.80 (t, J = 7.3 Hz, 1H), 7.11 (t, J = 8.5 Hz, 2H), 7.20–7.25 (m, 2H), 7.50–7.55 (m, 2H). 13C NMR (125 MHz, CDCl3): δ (ppm) 50.4, 114.1, 116.5, 116.6, 120.5, 129.2, 129.4, 129.6, 144.6, 162.0 (d, 1JC–F = 270 Hz). Anal. calcd for C14H11FN2: C, 74.32; H, 4.90; N, 12.38%. Found: C, 74.30; H, 4.81; N, 12.41%.
2-(p-Tolylamino)-2-(4-fluorophenyl)acetonitrile (compound 4ao).
White crystalline solid, mp: 103–105 °C (101–103 °C),30νmax (KBr): 3345, 3021, 2923, 2230 cm−1. 1H NMR (500 MHz, CDCl3): δ (ppm) 2.15 (s, 3H), 3.97 (br s, 1H), 5.42 (s, 1H), 6.66 (d, J = 8.5 Hz, 2H), 7.05 (d, J = 8.0 Hz, 2H), 7.12 (t, J = 8.5 Hz, 2H), 7.5–7.55 (m, 2H). 13C NMR (125 MHz, CDCl3): δ (ppm) 20.6, 50.0, 114.7, 114.9, 116.4, 116.6, 118.1, 129.1, 130.2, 142.1, 161.4 (d, 1JC–F = 275 Hz). Anal. calcd for C15H13FN2: C, 74.98; H, 5.45; N, 11.66%. Found: C, 74.81; H, 5.31; N, 11.69%.
2-(p-Tolylamino)-2-(2,4-dichlorophenyl)acetonitrile (compound ap).
White crystalline solid, mp: 116–118 °C (115–117 °C),30νmax (KBr): 3330, 3025, 2921, 2230 cm−1. 1H NMR (500 MHz, CDCl3): δ (ppm) 2.18 (s, 3H), 4.01 (br s, 1H), 5.66 (s, 1H), 6.77 (dd, J = 8.5, 0.7 Hz, 2H), 6.99 (t, J = 7.2 Hz, 1H), 7.25–7.31 (m, 2H), 7.41 (dd, J = 8.5, 2.0 Hz, 1H), 7.61 (d, J = 2.0 Hz 1H), 7.72 (d, J = 8.5 Hz, 1H). 13C NMR (125 MHz, CDCl3): δ (ppm) 21.9, 51.5, 114.3, 117.2, 120.5, 122.4, 128.0, 129.6, 129.8, 130.3, 134.3, 136.6, 144.2. Anal. calcd for C14H10Cl2N2: C, 60.67; H, 3.64; N, 10.11%. Found: C, 60.50; H, 3.60; N, 1.48%.
2-(4-Methoxyphenyl)-2-(phenylamino)acetonitrile (compound 4aq).
White crystalline solid, mp: 96–98 °C (95–97 °C),30νmax (KBr): 3340, 3025, 2918, 2229 cm−1. 1H NMR (500 MHz, CDCl3): δ (ppm) 3.88 (s, 3H), 4.06 (br s, 1H), 5.38 (s, 1H), 6.82 (d, J = 7.5 Hz, 2H), 6.93 (t, J = 7.5 Hz, 1H), 7.01 (d, J = 8.6 Hz, 2H), 7.29 (dd, J = 7.0, 1.0 Hz, 2H), 7.55 (d, J = 8.5 Hz, 2H). 13C NMR (125 MHz, CDCl3): δ (ppm) 50.9, 55.6, 114.1, 114.5, 118.3, 120.3, 126.0, 128.7, 129.3, 144.9, 160.4. Anal. calcd for C15H14N2O: C, 75.61; H, 5.92; N, 11.76%. Found: C, 75.55; H, 5.83; N, 11.77%.
2-(3-Nitrophenyl)-2-(phenylamino)acetonitrile (compound 4at).
Pale yellow crystalline solid, mp: 90–91 °C (89–92 °C),30νmax (KBr): 3350, 3015, 2870, 2239 cm−1. 1H NMR (500 MHz, CDCl3): δ (ppm) 4.12 (br s, 1H), 5.52 (s, 1H), 6.77 (d, J = 8.0 Hz, 2H), 6.97 (t, J = 7.8 Hz, 1H), 7.33 (m, 2H), 7.72 (t, J = 7.8 Hz, 1H), 8.01 (d, J = 8.0 Hz, 1H), 8.33 (d, J = 8.5 Hz 1H), 8.50 (s, 1H). 13C NMR (125 MHz, CDCl3): δ (ppm) 50.0, 114.5, 121.0, 122.3, 124.6, 129.33, 129.39, 130.8, 131.4, 133.0, 133.9, 143.9. Anal. calcd for C14H13N3O2: C, 66.40; H, 4.38; N, 16.59%. Found: C, 66.31; H, 4.31; N, 16.40%.
2-(Butylamino)-2-(pyridin-3-yl)acetonitrile (compound 4aw).
Colorless oil, νmax (KBr): 3340, 3025, 2960, 2220 cm−1. 1H NMR (500 MHz, CDCl3): δ (ppm) 0.93 (t, J = 7.1 Hz, 3H), 1.23–1.38 (m, 2H), 1.44–1.59 (m, 2H), 3.04 (q, J = 6.5 Hz, 2H), 3.91–4.15 (m, 2H), 7.44 (d, J = 8.1 Hz, 1H), 7.83 (dd, J = 2.0, 8.0 Hz, 1H), 8.51 (d, J = 8.5 Hz, 1H), 8.82 (s, 1H). 13C NMR (125 MHz, CDCl3): δ (ppm) 13.8, 20.2, 32.1, 45.5, 49.1, 116.8, 123.0, 132.4, 134.1, 147.2, 150.8. Anal. calcd for C11H15N3: C, 69.81; H, 7.99; N, 22.20%. Found: C, 69.88; H, 7.81; N, 22.12%.
2-(Phenylamino)hexanenitrile (compound 4az).
White crystalline solid, mp: 57–60 °C (55–57 °C),30νmax (KBr): 3350, 3015, 2945, 2229 cm−1. 1H NMR (500 MHz, CDCl3): δ (ppm) 0.91 (t, J = 6.5 Hz, 3H), 1.21–1.45 (m, 2H), 1.54–1.63 (m, 2H), 3.10 (q, J = 6.5 Hz, 2H), 3.95–4.20 (m, 2H), 6.92–7.29 (m, 5H). 13C NMR (125 MHz, CDCl3): δ (ppm) 14.3, 22.2, 25.4, 31.5, 48.6, 113.1, 116.2, 117.8, 129.3, 147.3. Anal. calcd for C12H16N2: C, 76.55; H, 8.57; N, 14.88%. Found: C, 76.54; H, 8.50; N, 14.83%.
2-(Benzylamino)-3-methylbutanenitrile (compound 4ba).
Colorless oil (colorless oil),30νmax (KBr): 3350, 3017, 2950, 2221 cm−1. 1H NMR (500 MHz, CDCl3): δ (ppm) 1.05 (d, J = 6.5 Hz, 3H), 1.07 (d, J = 6.5 Hz, 3H), 1.56 (br s, 1H), 1.97 (m, 1H), 2.04–2.15 (distorted AB System, 2H), 3.25 (d, J = 6.0 Hz, 1H), 3.82 (d, J = 12.5 Hz, 1H), 4.11 (d, J = 12.5 Hz, 1H), 7.25–7.41 (m, 5H). 13C NMR (125 MHz, CDCl3): δ (ppm) 18.3, 19.4, 31.4, 51.9, 56.7, 119.0, 127.3, 128.9, 128.9, 138.4. Anal. calcd for C12H16N2: C, 76.55; H, 8.57; N, 14.88%. Found: C, 76.45; H, 8.51; N, 14.72%.
1-(Phenylamino)cyclopentanecarbonitrile (compound 4be).
White crystalline solid, mp: 55–56 °C, νmax (KBr): 3345, 3021, 2950, 2236 cm−1. 1H NMR (500 MHz, CDCl3): δ (ppm) 1.43 (m, 2H), 1.54 (m, 2H), 1.87 (m, 2H), 2.09 (m, 2H), 3.95 (br s, 1H), 6.67 (d, J = 7.5 Hz, 2H), 6.93 (t, J = 7.5 Hz, 1H), 7.13 (t, J = 7.5 Hz, 2H). 13C NMR (125 MHz, CDCl3): δ (ppm) 22.6, 33.8, 53.2, 113.3, 117.0, 119.8, 131.2, 143.1. Anal. calcd for C12H14N2: C, 77.38; H, 7.58; N, 15.04%. Found: C, 77.25; H, 7.55; N, 14.99%.
1-(p-Tolylamino)cyclopentanecarbonitrile (compound 4bf).
White crystalline solid, mp: 57–59 °C, νmax (KBr): 3350, 3018, 2930, 2233 cm−1. 1H NMR (500 MHz, CDCl3): δ (ppm) 1.44 (m, 2H), 1.56 (m, 2H), 1.85 (m, 2H), 2.12 (m, 2H), 2.29 (s, 3H), 3.96 (br s, 1H), 6.81 (d, J = 7.6 Hz, 2H), 6.93 (d, J = 7.6 Hz, 2H). 13C NMR (125 MHz, CDCl3): δ (ppm) 22.7, 24.1, 32.5, 51.6, 113.2, 119.3, 126.6, 128.9, 143.8. Anal. calcd for C13H16N2: C, 77.96; H, 8.05; N, 13.99%. Found: C, 77.90; H, 8.06; N, 13.92%.
1-(Phenylamino)cycloheptanecarbonitrile (compound 4bg).
White crystalline solid, mp: 56–57 °C, νmax (KBr): 3345, 3035, 2920, 2225 cm−1. 1H NMR (500 MHz, CDCl3): δ (ppm) 1.28 (br s, 4H), 1.35 (br s, 4H), 1.74 (br s, 4H), 4.01 (s, 1H), 6.66 (d, J = 8.0 Hz, 2H), 6.83 (t, J = 8.0 Hz, 1H), 7.09 (t, J = 8.0 Hz, 2H). 13C NMR (125 MHz, CDCl3): δ (ppm) 21.2, 27.7, 33.6, 52.1, 113.1, 117.3, 119.5, 127.1, 143.9. Anal. calcd for C14H18N2: C, 78.46; H, 8.47; N, 13.07%. Found: C, 78.33; H, 8.41; N, 13.12%.
1-(4-Bromophenylamino)cycloheptanecarbonitrile (compound 4bh).
White crystalline solid, mp: 62–64 °C, νmax (KBr): 3405, 3028, 2935, 2233 cm−1. 1H NMR (500 MHz, CDCl3): δ (ppm) 1.27 (br s, 4H), 1.36 (br s, 4H), 1.72 (br s, 4H), 4.02 (s, 1H), 6.84 (d, J = 8.1 Hz, 2H), 6.83 (d, J = 8.1 Hz, 2H). 13C NMR (125 MHz, CDCl3): δ (ppm) 21.3, 27.4, 33.5, 51.9, 113.0, 115.5, 120.4, 133.7, 143.9. Anal. calcd for C14H17BrN2: C, 57.35; H, 5.84; N, 9.55%. Found: C, 57.32; H, 5.72; N, 9.62%.
2-Phenyl-2-(phenylamino)propanenitrile (compound 4bi).
White crystalline solid, mp: 143–145 °C, νmax (KBr): 3320, 3046, 2225 cm−1. 1H NMR (500 MHz, CDCl3): δ (ppm) 1.92 (s, 3H), 4.56 (br s, 1H), 6.71–6.83 (m, 3H), 7.20–7.31 (m, 5H), 7.40 (d, J = 8.5 Hz, 2H). 13C NMR (125 MHz, CDCl3): δ (ppm) 22.5, 66.3, 113.8, 120.0, 120.8, 127.6, 128.1, 128.8, 129.3, 130.4, 147.7. Anal. calcd for C15H14N2: C, 81.05; H, 6.35; N, 12.60%. Found: C, 80.91; H, 6.33; N, 12.72%.
2-(4-Bromophenylamino)-2-p-tolylpropanenitrile (compound 4bj).
White crystalline solid, mp: 179–181 °C, νmax (KBr): 3330, 3070, 2955, 2310 cm−1. 1H NMR (500 MHz, CDCl3): δ (ppm) 1.94 (s, 3H), 2.38 (s, 3H), 4.29 (br s, 1H), 6.68 (d, J = 8.5 Hz, 2H), 7.10 (d, J = 8.5 Hz, 2H), 7.18–7.29 (m, 4H). 13C NMR (125 MHz, CDCl3): δ (ppm) 21.3, 23.8, 68.5, 114.3, 115.0, 120.1, 127.0, 128.1, 128.7, 132.4, 137.7, 146.6. Anal. calcd for C16H15BrN2: C, 60.97; H, 4.80; N, 8.89%. Found: C, 61.02; H, 4.88; N, 8.95%.
2-(Isopropylamino)-2-(3-nitrophenyl)propanenitrile (compound 4bk).
Yellow crystalline solid, mp: 115–117 °C, νmax (KBr): 3364, 3052, 2948, 2220 cm−1. 1H NMR (500 MHz, CDCl3): δ (ppm) 1.07 (d, J = 6.9 Hz, 6H), 1.72 (br s, 1H), 1.83 (s, 3H), 2.83 (m, 1H), 7.64–7.68 (m, 2H), 8.03 (d, J = 8.5 Hz, 1H), 8.19 (d, J = 2.0 Hz, 1H). 13C NMR (125 MHz, CDCl3): δ (ppm) 23.5, 24.5, 46.6, 63.2, 119.8, 123.7, 125.5, 127.9, 131.0, 134.8, 147.2. Anal. calcd for C12H15N3O2: C, 61.79; H, 6.48; N, 18.01%. Found: C, 61.88; H, 6.45; N, 17.89%.
2-(Benzylamino)-2,2-diphenylacetonitrile (compound 4bl).
White crystalline solid, mp: 155–157 °C, νmax (KBr): 3350, 3061, 2235 cm−1. 1H NMR (500 MHz, CDCl3): δ (ppm) 2.01–2.11 (distorted AB System, 2H), 1.92 (s, 1H), 7.23–7.36 (m, 15H). 13C NMR (125 MHz, CDCl3): δ (ppm) 46.5, 68.7, 1143, 127.1, 126.5, 127.9, 128.3, 128.5, 129.2, 140.6, 143.7. Anal. calcd for C21H18N2: C, 84.53; H, 6.08; N, 9.39%. Found: C, 84.56; H, 6.17; N, 9.32%.
2-(4-Bromophenylamino)-2,2-diphenylacetonitrile (compound 4bm).
White crystalline solid, mp: 171–173 °C, νmax (KBr): 3363, 3042, 2230 cm−1. 1H NMR (500 MHz, CDCl3): δ (ppm) 4.63 (br s, 1H), 6.59 (d, J = 8.3 Hz, 2H), 7.16 (d, J = 8.3 Hz, 2H), 7.21–7.35 (m, 10H). 13C NMR (125 MHz, CDCl3): δ (ppm) 68.6, 114.3, 114.6, 115.3, 126.5, 128.6, 129.0, 132.3, 143.0, 146.9. Anal. calcd for C20H15BrN2: C, 66.13; H, 4.16; N, 7.71%. Found: C, 66.19; H, 4.23; N, 7.83%.
3-Oxo-3-phenyl-2-(phenylamino)propanenitrile (compound 4bn).
White crystalline solid, mp: 59–61 °C, νmax (KBr): 3475, 3015, 2920, 2235, 1690 cm−1. 1H NMR (500 MHz, CDCl3): δ (ppm) 4.25 (br s, 1H), 5.09 (s, 1), 6.48 (d, J = 8.0 Hz, 2H), 6.64 (t, J = 8.0 Hz, 1H), 7.03 (t, J = 8.0 Hz, 2H), 7.45 (t, J = 7.5 Hz, 2H), 7.56 (t, J = 7.7 Hz, 1H), 7.81 (d, J = 7.5 Hz, 2H). 13C NMR (125 MHz, CDCl3): δ (ppm) 63.1, 113.1, 116.9, 126.5, 126.9, 128.1, 129.6, 133.0, 135.1, 143.7, 197.2. Anal. calcd for C15H12N2O: C, 76.25; H, 5.12; N, 11.86%. Found: C, 76.20; H, 5.02; N, 11.94%.
2-(p-Tolylamino)-3-oxo-3-phenylpropanenitrile (compound 4bo).
White crystalline solid, mp: 63–65 °C, νmax (KBr): 3395, 3025, 2930, 2233, 1695 cm−1. 1H NMR (500 MHz, CDCl3): δ (ppm) 1.96 (s, 3H), 4.01 (br s, 1H), 5.12 (s, 1), 6.51 (d, J = 8.5 Hz, 2H), 7.08 (d, J = 8.5 Hz, 2H), 7.48 (t, J = 7.6 Hz, 2H), 7.59 (t, J = 7.5 Hz, 2H), 7.85 (d, J = 7.6 Hz, 2H). 13C NMR (125 MHz, CDCl3): δ (ppm) 24.6, 62.8, 113.4, 116.1, 126.7, 126.9, 128.0, 129.4, 133.2, 135.5, 143.1, 197.0. Anal. calcd for C16H14N2O: C, 76.78; H, 5.64; N, 11.19%. Found: C, 76.62; H, 5.71; N, 11.01%.
1,2,3,4-Tetrahydro-1-(phenylamino)naphthalene-1-carbonitrile (compound 4bp).
White crystalline solid, mp: 123–125 °C, νmax (KBr): 3360, 3042, 2945, 2225 cm−1. 1H NMR (500 MHz, CDCl3): δ (ppm) 1.59–1.76 (m, 2H), 2.01–2.18 (m, 2H), 2.84–2.89 (m, 2H), 4.62 (br s, 1H), 6.83–6.92 (m, 7H), 7.25 (t, J = 8.1 Hz, 2H). 13C NMR (125 MHz, CDCl3): δ (ppm) 14.5, 29.9, 38.6, 63.4, 113.2, 120.0, 120.8, 125.1, 127.3, 129.8, 134.1, 135.6, 147.2. Anal. calcd for C17H16N2: C, 82.22; H, 6.49; N, 11.28%. Found: C, 82.12; H, 6.40; N, 11.33%.
2-Oxo-3-(phenylamino)indoline-3-carbonitrile (compound 7a).
White crystalline solid, mp: 91–92 °C, νmax (KBr): 3400, 3310, 3050, 2950, 2235, 1670 cm−1. 1H NMR (500 MHz, CDCl3): δ (ppm) 4.95 (br s, 1H), 6.92 (d, J = 7.0 Hz, 1H), 7.25–7.50 (m, 8H), 8.38 (s, 1H). 13C NMR (125 MHz, CDCl3): δ (ppm) 71.2, 111.1, 119.3, 121.5, 125.1, 126.3, 129.4, 131.1, 132.5, 140.4, 148.2, 152.7, 171.1. Anal. calcd for C15H11N3O: C, 72.28; H, 4.45; N, 16.86%. Found: C, 72.10; H, 4.52; N, 16.95%.
3-(p-Tolylamino)-2-oxoindoline-3-carbonitrile (compound 7b).
White crystalline solid, mp: 99–101 °C, νmax (KBr): 3410, 3312, 3045, 2930, 2233, 1670 cm−1. 1H NMR (500 MHz, CDCl3): δ (ppm) 2.18 (s, 3H), 4.98 (br s, 1H), 6.97 (d, J = 7.2 Hz, 1H), 7.20–7.46 (m, 7H), 8.66 (br s, 1H). 13C NMR (125 MHz, CDCl3): δ (ppm) 24.3, 71.5, 111.8, 119.0, 122.3, 125.1, 126.0, 129.2, 131.5, 132.9, 140.4, 148.2, 152.8, 171.1. Anal. calcd for C16H13N3O: C, 72.99; H, 4.98; N, 15.96%. Found: C, 72.86; H, 4.86; N, 15.89%.
5-Methyl-2-oxo-3-(phenylamino)indoline-3-carbonitrile (compound 7c).
White crystalline solid, mp: 110–112 °C, νmax (KBr): 3410, 3300, 3045, 2950, 2233, 1676 cm−1. 1H NMR (500 MHz, CDCl3): δ (ppm) 2.25 (s, 3H), 5.01 (br s, 1H), 6.75 (s, 1H), 6.99–7.02 (br s, 2H), 7.94 (m, 5H), 9.91 (br s, 1H). 13C NMR (125 MHz, CDCl3): δ (ppm) 21.4, 71.3, 111.4, 119.1, 121.6, 125.0, 125.8, 126.2, 129.4, 131.0, 131.6, 132.9, 140.3, 171.4. Anal. calcd for C16H13N3O: C, 72.99; H, 4.98; N, 15.96%. Found: C, 72.91; H, 4.95; N, 16.01%.
3-(4-Bromophenylamino)-5-methyl-2-oxoindoline-3-carbonitrile (compound 7d).
White crystalline solid, mp: 119–121 °C, νmax (KBr): 3410, 3315, 3050, 2940, 2220, 1670 cm−1. 1H NMR (500 MHz, CDCl3): δ (ppm) 2.22 (s, 3H), 5.05 (br s, 1H), 6.71 (s, 1H), 6.99–7.01 (br s, 2H), 7.89–8.15 (m, 4H), 9.82 (br s, 1H). 13C NMR (125 MHz, CDCl3): δ (ppm) 22.6, 71.3, 111.4, 119.6, 122.1, 125.6, 125.7, 126.6, 129.4, 131.0, 131.6, 132.8, 140.7, 171.3. Anal. calcd for C16H12BrN3O: C, 56.16; H, 3.53; N, 12.28%. Found: C, 56.02; H, 3.59; N, 12.39%.
1,2-Dihydro-2-oxo-1-(phenylamino)acenaphthylene-1-carbonitrile (compound 7e).
Pale yellow crystalline solid, mp: 135–137 °C, νmax (KBr): 3460, 3045, 2950, 2233, 1710 cm−1. 1H NMR (500 MHz, CDCl3): δ (ppm) 5.12 (br s, 1H), 7.39 (t, J = 6.7 Hz, 1H), 7.45–7.52 (m, 5H), 7.61 (d, J = 7.0 Hz, 1H), 7.76 (t, J = 7.5 Hz, 1H), 7.83 (d, J = 7.5 Hz, 1H), 7.97 (d, J = 8.0 Hz, 1H), 8.21 (d, J = 8.0 Hz, 1H). 13C NMR (125 MHz, CDCl3): δ (ppm) 73.2, 120.4, 121.1, 123.6, 126.1, 127.0, 128.9, 129.1, 129.4, 130.9, 131.1, 131.3, 132.8, 133.2, 134.8, 142.5, 195.3. Anal. calcd for C19H12N2O: C, 80.27; H, 4.25; N, 9.85%. Found: C, 80.19; H, 4.20; N, 9.76%.
1-(p-Tolylamino)-1,2-dihydro-2-oxoacenaphthylene-1-carbonitrile (compound 7f).
Pale yellow crystalline, mp: 138–140 °C, νmax (KBr): 3440, 3050, 2935, 2220, 1705 cm−1. 1H NMR (500 MHz, CDCl3): δ (ppm) 2.21 (s, 3H), 5.08 (br s, 1H), 7.39 (t, J = 7.0 Hz, 1H), 7.45–7.52 (m, 4H), 7.68 (d, J = 7.0 Hz, 1H), 7.76 (t, J = 7.6 Hz, 1H), 7.89 (d, J = 7.6 Hz, 1H), 7.97 (d, J = 8.1 Hz, 1H), 8.19 (d, J = 8.1 Hz, 1H). 13C NMR (125 MHz, CDCl3): δ (ppm) 22.8, 72.1, 120.3, 121.8, 123.4, 126.3, 127.5, 128.9, 129.1, 129.4, 130.3, 131.6, 131.9, 132.6, 133.0, 134.5, 142.6, 196.1. Anal. calcd for C20H14N2O: C, 80.52; H, 4.73; N, 9.39%. Found: C, 80.48; H, 4.62; N, 9.47%.
1-(4-Bromophenylamino)-1,2-dihydro-2-oxoacenaphthylene-1-carbonitrile (compound 7g).
Pale yellow crystalline, mp: 168–170 °C, νmax (KBr): 3410, 3035, 2950, 2230, 1715 cm−1. 1H NMR (500 MHz, CDCl3): δ (ppm) 5.10 (s, 1H), 7.48–7.53 (m, 2H), 7.55–7.59 (m, 2H), 7.64 (d, J = 7.5 Hz, 1H), 7.76 (d, J = 8.5 Hz, 1H), 7.83 (t, J = 7.8 Hz, 1H), 8.05 (d, J = 8.5 Hz, 1H), 8.14 (d, J = 7.5 Hz, 1H), 8.26 (d, J = 8.3 Hz, 1H). 13C NMR (125 MHz, CDCl3): δ (ppm) 71.0, 120.5, 121.3, 123.4, 127.3, 128.7, 129.2, 129.9, 130.1, 130.8, 131.4, 133.3, 134.7, 135.1, 142.8, 148.1, 196.1. Anal. calcd for C19H11BrN2O: C, 62.83; H, 3.05; N, 7.71%. Found: C, 62.71; H, 3.01; N, 7.89%.
Acknowledgements
The authors thank the Research Committee of Islamic Azad University (Shiraz Branch) for financial support of this work.
Notes and references
- H. Bienayme, C. Hulme, G. Oddon and P. Schmitt, Chem.–Eur. J., 2000, 6, 3321–3329 CrossRef CAS.
- A. Strecker, Ann. Chem. Pharm., 1850, 75, 27–45 CrossRef.
- Y. M. Shafran, V. S. Bakulev and V. S. Mokrushin, Russ. Chem. Rev., 1989, 58, 148–162 CrossRef.
-
J. March, Advanced Organic Chemistry, Wiley, New York, 1995, 4th edn, p. 965 Search PubMed.
- L. M. Weinstock, P. Davis, B. Handelsman and R. Tull, J. Org. Chem., 1967, 32, 2823–2829 CrossRef CAS.
- W. L. Matier, D. A. Owens, W. T. Comer, D. Dietchman, H. C. Ferguson, R. J. Seidehamel and J. R. Young, J. Med. Chem., 1973, 16, 901–908 CrossRef CAS.
- R. O. Duthaler, Tetrahedron, 1994, 50, 1539–1650 CrossRef CAS.
- H. Groger, Chem. Rev., 2003, 103, 2795–2827 CrossRef.
- S. Kobayashi and H. Ishitani, Chem. Rev., 1999, 99, 1069–1094 CrossRef CAS.
- S. Harusawa, Y. Hamada and T. Shioiri, Tetrahedron Lett., 1979, 20, 4663–4666 CrossRef.
- P. Vachal and E. N. Jacobsen, J. Am. Chem. Soc., 2002, 124, 10012–10014 CrossRef CAS.
- S. Nakamura, N. Sato, M. Sugimoto and T. Toru, Tetrahedron: Asymmetry, 2004, 15, 1513–1516 CrossRef CAS.
- B. C. Ranu, S. S. Dey and S. Hajra, Tetrahedron, 2002, 58, 2529–2532 CrossRef CAS.
- S. K. De and R. A. Gibbs, Tetrahedron Lett., 2004, 45, 7407–7408 CrossRef CAS.
- J. S. Yadav, B. V. S. Reddy, B. Eswaraiah and M. Srinivas, Tetrahedron, 2004, 60, 1767–1771 CrossRef CAS.
- B. Karimi and A. A. Safari, J. Organomet. Chem., 2008, 693, 2967–2970 CrossRef CAS.
- B. M. Reddy, B. Thirupathi and M. K. Patil, J. Mol. Catal. A: Chem., 2009, 307, 154–159 CrossRef CAS.
- H. A. Oskooie, M. M. Heravi, A. Sadnia, F. Jannati and F. K. Behbahani, Synth. Commun., 2007, 37, 2543–2548 CrossRef CAS.
- N. H. Khan, S. Agrawal, R. I. Kureshy, S. H. R. Abdi, S. Singh, E. Suresh and R. V. Jasra, Tetrahedron Lett., 2008, 49, 640–644 CrossRef CAS.
- Z. L. Shen, S. J. Ji and T. P. Loh, Tetrahedron, 2008, 64, 8159–8163 CrossRef CAS.
- S. H. Wang, L. F. Zhao and Z. M. Du, Chin. J. Chem., 2006, 24, 135–137 CrossRef.
- E. Rafiee and A. Azad, Synth. Commun., 2007, 37, 1127–1132 CrossRef CAS.
- S. K. De, Synth. Commun., 2005, 35, 1577–1582 CrossRef.
- M. M. Mojtahedi, S. Abaee and T. Alishiri, Tetrahedron Lett., 2009, 50, 2322–2325 CrossRef CAS.
- A. Heydari, A. Arefi, S. Khaksar and R. K. Shiroodi, J. Mol. Catal. A: Chem., 2007, 271, 142–144 CrossRef CAS.
- A. Shaabani, A. Maleki, M. R. Soudi and H. Mofakham, Catal. Commun., 2009, 10, 945–949 CrossRef CAS.
- J. S. Yadav, B. V. S. Reddy, B. Eshwaraiah, M. Srinivas and P. Vishnumurthy, New J. Chem., 2003, 27, 462–465 RSC.
- W. Y. Chen and J. Lu, Silica sulfuric acid catalyzed one-pot synthesis of α-aminonitriles, Synlett, 2005, 2293–2296 CrossRef CAS.
- B. Karimi and D. Zareyee, J. Mater. Chem., 2009, 19, 8665–8670 RSC.
- K. Niknam, D. Saberi and M. Nouri Sefat, Tetrahedron Lett., 2010, 51, 2959–2962 CrossRef CAS.
- B. A. Bhanu, A. Bisai and V. K. Singh, Tetrahedron Lett., 2004, 45, 9565–9567 CrossRef.
- J. S. Yadav, B. V. S. Reddy, B. Eeshwaraiah and M. Srinivas, Tetrahedron, 2004, 60, 1767–1771 CrossRef CAS.
- A. Heydari, P. Fatemi and A. A. Alizadeh, Tetrahedron Lett., 1998, 39, 3049–3050 CrossRef CAS.
- J. Lablanc and H. W. Gibson, Tetrahedron Lett., 1992, 33, 6295–6298 CrossRef.
- T. K. Chakraborty, G. V. Reddy and K. A. Hussain, Tetrahedron Lett., 1991, 32, 7597 CrossRef CAS.
- H. A. Oskooie, M. M. Heravi, K. Bakhtiari, V. Zadsirjan and F. F. Bamoharram, Synlett, 2006, 1768–1770 CAS.
- A. Baeza, C. Nájera and J. M. Sansano, Synthesis, 2007, 1230–1234 CAS.
- I. V. P. Raj, G. Suryavanshi and A. Sudalai, Tetrahedron Lett., 2007, 48, 7211–7214 CrossRef CAS.
-
(a)
P. A. Grieco, Organic Synthesis in Water, Blackie Academic and Professional, London, 1998 Search PubMed;
(b)
Green Chemistry, ed. P. T. Anastas and T. C. Williamson, ACS Symposium Series 626, American Chemical Society, Washington, DC, 1996 Search PubMed;
(c)
V. J. Li and T. H. Chan, Organic Reactions in Aqueous Media, Wiley, New York, 1997 Search PubMed;
(d)
P. Anastas and J. C. Warner, Green Chemistry: Theory and Practice, Oxford University Press, Oxford, 1998 Search PubMed;
(e) C. J. Li, Chem. Rev., 2005, 105, 3095–3165 CrossRef CAS.
-
(a)
S. Kobayashi, K. Manabe and S. Nagayama, in Modern Carbonyl Chemistry, ed. J. Otera, Wiley-VCH, Weinheim, 2000 Search PubMed;
(b) K. Manabe, Y. Mori, T. Wakabayashi, S. Nagayama and S. Kobayashi, J. Am. Chem. Soc., 2000, 122, 7202–7207 CrossRef CAS;
(c) S. Kobayashi, S. Nagayama and T. Busujima, J. Am. Chem. Soc., 1998, 120, 8287–8288 CrossRef CAS.
- D. C. Bailey and S. H. Langer, Chem. Rev., 1981, 81, 109–148 CrossRef CAS.
- L. A. Thompson and J. A. Ellman, Chem. Rev., 1996, 96, 555–600 CrossRef CAS.
- A. Kiasat and M. F. Mehrjardi, Catal. Commun., 2008, 9, 1497–1500 CrossRef CAS.
- X. Wang, Z. Quan, F. Wang, M. Wang, Z. Zhang and Z. Li, Synth. Commun., 2006, 36, 451–456 CrossRef CAS.
- X. C. Wang, L. Li, Z. J. Quan, H. P. Gong, H. L. Ye and X. F. Cao, Chin. Chem. Lett., 2009, 20, 651–655 CrossRef CAS.
- A. Hasaninejad, A. Zare and M. Shekouhy, Tetrahedron, 2011, 67, 390–400 CrossRef CAS.
- A. Hasaninejad, A. Zare, M. Shekouhy and J. Ameri-Rad, Green Chem., 2011, 13, 958–964 RSC.
- A. Hasaninejad, M. Shekouhy, A. Zare, S. M. S. Hoseini Ghattali and N. Golzar, J. Iran. Chem. Soc., 2011, 8, 411–423 CAS.
-
(a) K. C. Joshi and P. Chand, Pharmazie, 1982, 37, 864–865 Search PubMed;
(b) A. H. Abdel-Rahman, E. M. Keshk, M. A. Hanna and S. M. El-Bady, Bioorg. Med. Chem., 2006, 12, 2483–2488 CrossRef;
(c) T. H. Kang, K. Matsumoto, Y. Murakami, H. Takayama, M. Kitajima, N. Aimi and H. Watanabe, Eur. J. Pharmacol., 2002, 444, 39–45 CrossRef CAS;
(d) J. Ma and S. M. Hecht, Chem. Commun., 2004, 1190–1191 RSC;
(e) M. M. Khafagy, A. H. F. A. El-Wahas, F. A. Eid and A. M. El-Agrody, Farmaco, 2002, 57, 715–722 CrossRef CAS.
-
(a) M. G. Dekamin, S. Javanshir, M. R. Naimi-Jamal, R. Hekmatshoar and J. Mokhtari, J. Mol. Catal. A: Chem., 2008, 283, 29–32 CrossRef CAS;
(b) M. G. Dekamin, J. Mokhtari and M. R. Naimi-Jamal, Catal. Commun., 2009, 10, 582–585 CrossRef CAS;
(c) M. G. Dekamin, S. Sagheb Asl and M. R. Naimi-Jamal, Tetrahedron Lett., 2009, 50, 4063–4066 CrossRef CAS;
(d) M. G. Dekamin, R. Alizadeh and M. R. Naimi-Jamal, Appl. Organomet. Chem., 2010, 24, 229–235 CrossRef CAS;
(e) M. G. Dekamin, M. Farahmand, M. R. Naimi-Jamal and S. Javanshir, Catal. Commun., 2008, 9, 1352–1355 CrossRef CAS;
(f) M. G. Dekamin and Z. Karimi, J. Organomet. Chem., 2009, 694, 1789–1794 CrossRef CAS.
-
(a) D. Enders and J. P. Shilvock, Chem. Soc. Rev., 2000, 29, 359–373 RSC;
(b) P. Vongvilai and O. Ramstrom, J. Am. Chem. Soc., 2009, 131, 14419–14425 CrossRef CAS;
(c) V. Banphavichit, W. Mansawat, W. Bhanthumnavin and T. Vilaivan, Tetrahedron, 2004, 60, 10559–10568 CrossRef CAS;
(d) B. A. Bhanu Prasad, A. Bisai and V. K. Singh, Tetrahedron Lett., 2004, 45, 95–98 CrossRef.
- M. G. Dekamin and Z. Mokhtari, Tetrahedron, 2012, 68, 922–930 CrossRef CAS.
|
This journal is © The Royal Society of Chemistry 2012 |