DOI:
10.1039/B9AY00057G
(Paper)
Anal. Methods, 2009,
1, 220-224
Preparation of a highly specific polyclonal antibody against fluvastatin and its use in development of ELISA for determination of fluvastatin in plasma
Received
2nd June 2009
, Accepted 15th October 2009
First published on 28th October 2009
Abstract
For therapeutic monitoring and pharmacokinetic studies of the potent hypocholesterolaemic agent fluvastatin (FLV), a specific antibody was required for the development of a sensitive enzyme-linked immunosorbent assay (ELISA) for the accurate determination of FLV in plasma. In this study, a highly specific polyclonal antibody against FLV has been prepared. FLV was coupled to keyhole limpet hemocyanin (KLH) and bovine serum albumin (BSA) using carbodiimide reagent. FLV-KLH conjugate was used as an immunogen. Female 8-weeks old New Zealand white rabbits were immunized with an emulsion of FLV-KLH with Freund's adjuvant. The immune response of the rabbits was monitored by direct ELISA using FLV-BSA immobilized onto microwell plates as a solid phase. The rabbit that showed the highest antibody titer and affinity to FLV was scarified and its sera were collected. The IgG fraction was isolated and purified by affinity chromatography on a protein A column. The specificity of the purified antibody for FLV was evaluated by indirect competitive ELISA using various competitors from the FLV-structural analogues and therapeutic agents used with FLV in a combination therapy. The high affinity of the antibody (IC50 = 150 pg ml−1) enabled the determination of FLV in plasma at concentrations as low as 20 pg ml−1.
Introduction
Fluvastatin (FLV) [R*,S*-(E)]-(±)-7-[3-(4-fluorophenyl)-1-(1-methylethyl)-1H-indol-2-yl]-3,5-dihydroxy-6-heptenoic acid, is a totally synthetic highly potent hypocholesterolemic drug.1 It exerts its effects by inhibition of the 3-hydroxy-3-methylglutaryl-coenzyme A (HMG-CoA) reductase, the enzyme that catalyzes the conversion of HMG-CoA to mevalonate, which is the early rate-limiting step in the biosynthesis of cholesterol in the body.2 As well, FLV reduces the level of low density lipoprotein (LDL) in plasma, and it is well tolerated by the patients.3FLV has a short half-life, and its bioavailability is not affected by food. Because of the hydrophilicity of FLV, it does not cross the blood–brain barrier, therefore it has no central nervous system side effects (e.g. insomnia).4 These qualities made FLV one of the most prescribed drugs for the treatment of hypercholesterolemia.
Because of the therapeutic importance and clinical success of FLV, several methods have been developed for its quantitative determination in plasma samples. These methods include HPLC,5–10gas chromatography,11 and capillary electrophoresis.12 These methods involved tedious steps for the pre-treatment of samples, pre-derivatization with critical derivatizing reagents, and use of expensive tandem mass spectrometric detectors that are not available in most clinical laboratories. For these reasons, the development of new alternative analytical technology for determination of FLV in plasma with adequate sensitivity, improved simplicity, and lower cost was seriously needed.
Immunoassays are preferable in the field of clinical analysis because of their applicability for a wide range of analytes, high-throughput, low cost, convenience for screening of a large number samples, and their specificity for the analyte of interest even in a multi-component complex sample matrix such as plasma.13 The specificity of the antibody to the analyte of interest is the limiting factor in the validity of any immunoassay system. In order to establish a specific and sensitive immunoassay for FLV, a specific antibody with high affinity for FLV was required. The present study describes, for the first time, the preparation of a polyclonal antibody that can specifically recognize FLV with high affinity. The immunoassay method developed using this antibody is able to determine FLV in plasma samples at concentrations as low as 20 pg ml−1.
Experimental
Instruments
ELX808 microplate reader (Bio-Tek Instruments Inc., USA). ELX50 microplate washer (Bio-Tek Instruments Inc., USA). EM-36N microtube shaker (Taitec, Japan). Biofuge Pico centrifuge (Heraeus Instruments, Germany). Mini/18 incubator (Genlab Ltd., UK). Water purification system (Milli-Q Labo, Millipore Ltd., Bedford, USA).
Materials
Fluvastatin (FLV) was obtained from Biocon India Ltd., India. Horseradish peroxidase labeled goat anti-rabbit IgG (HRP-IgG), bovine serum albumin (BSA), 2,4,6-trinitrobenzene sulfonic acid, 1-ethyl-3-(3-dimethylaminopropyl) carbodiimide hydrochloride (EDC), and Tween-20 were purchased from Sigma Chemical Co. (St. Louis, MO, USA). Keyhole limpet hemocyanin (KLH) was purchased from Novabiochem Co. (La Jolla, CA, USA). 3,3′,5,5′-Tetramethylbenzidine (TMB) peroxidase substrate was obtained from Kirkegaard-Perry Laboratories (Gaithersburg, MD, USA). ELISA high-binding microwell plates were a product of Corning/Costar, Inc. (Cambridge, MA, USA). Centricon-30 filter (Amicon, Inc., Beverly, MA, USA). BCA reagent for protein assay and protein A column were obtained from Pierce Biotechnology Inc. (Rockford, IL, USA).
Procedures
All experimental procedures were approved by the institutional committee and performed in compliance with the relevant laws and institutional guidelines.
Preparation of FLV-protein conjugates.
FLV was conjugated with keyhole limpt hemocyanin (KLH) and bovine serum albumin (BSA) according to the method described by Darwish et al.14 Briefly, EDC (150 mg) was added to FLV solution (10-ml, 5 mg ml−1) in 12.5 mM phosphate buffer (PB) of pH 5, and the pH of the reaction mixture was maintained at pH 5–5.5 using 0.01 M HCl for 5 min. Five ml of protein solution (5 mg ml−1, in 50 mM PB of pH 7.2) was added, and the pH was rapidly adjusted to pH 6.4 and maintained constant for 90 min. The reaction was left to proceed overnight in the dark at 4 °C. The unconjugated FLV was removed from the FLV-protein conjugates by buffer exchange using a Centricon-30 filter. Protein content of each conjugate was determined by BCA reagent kit and the extent of substitution of free amino groups on the protein was determined by estimation of free amino groups on equal amounts of the corresponding protein which underwent the same experimental treatment and on protein subjected to the conjugation by the procedure described by Habeeb.15 The extent of conjugation = (AFLV-Protein/AProtein) ×100; where AFLV-Protein and AProtein were the absorbances obtained from the reaction of 2,4,6-trinitrobenzene sulfonic acid with FLV-protein and protein, respectively. The extent of conjugation was found to be 16.5 and 24.8% of the total amino group residues on KLH and BSA, respectively.
Immunization of animals and purification of antibody.
The immunogen used was FLV-KLH protein conjugate. Four female 8-weeks old New Zealand white rabbits were injected subcutaneously with 1 mg of FLV-KLH emulsified in Freund's complete adjuvant, divided in different sites for each rabbit. The same immunization procedure was repeated 6 times with 2-weeks interval, however incomplete adjuvant was used instead. After 4–7 days from each immunization, blood samples (∼500 μl) were collected and diluted tenfold with PBS. The diluted blood samples were centrifuged at 10,000 g at 4 °C for 10 min, and the sera (supernatants) were collected. The antibody response in each rabbit was determined by analysis of the collected antisera by direct enzyme immunoassay.16 The rabbit whose diluted serum gave the highest affinity (lower IC50) to FLV was selected as the most appropriate rabbit for collecting its total serum as crude anti-FLV polyclonal antibody sample.
The serum (∼20 ml) was kept overnight at 4 °C and then centrifuged at 4 °C for 10 min. To 5 ml of the supernatant, an equal volume of a saturated ammonium sulfate solution was gradually added and gently mixed. For complete precipitation of the IgG, the solution was kept over ice for 3 h. The precipitate was collected by centrifugation at 10,000 g at 4 °C for 30 min. The precipitate was resuspended in 10 ml phosphate buffered saline (PBS; 137 mM NaCl, 3 mM KCl, and 10 mM sodium phosphate, pH 7.4) followed by reprecipitation with ammonium sulfate. After repeating this step three times, the precipitate was dissolved in 10 ml of PBS. The produced antibody solution was purified by protein A column chromatography. One milliliter aliquot of the solution was mixed with an equal volume of the binding buffer (1.5 M glycine-NaOH containing 3 M NaCl, pH 8.7) and the mixture was applied to the protein A column and the eluent was monitored for protein by measuring the absorbance of the eluted fractions at 280 nm. The column was washed with 50–60 ml of binding buffer, and the bound immunoglobulin was eluted with 0.1 M sodium citrate buffer (pH 3.0). The eluate was collected in 1.5 ml fractions into tubes containing 100 μl of 1 M Tris-HCl buffer (pH 9.0), and mixed. The pooled fractions were dialyzed overnight against five changes of PBS (∼4 h intervals). The protein content of the dialyzate was determined by BCA reagent kit, and used as the pure anti-FLV antibody sample.
ELISA procedures and data analysis.
Aliquot (50 μl) of either FLV-BSA conjugate or BSA protein solution (5 μg ml−1 in PBS) was dispensed in each well of the microwell plate. The plates were incubated for 2 h at 37 °C. The wells were washed with PBS containing 0.05% Tween-20 (PBS-T) and blocked with 200 μl of 3% BSA by incubation at 37 °C for 1 h. A 50 μl of anti-FLV antibody sample (rabbit serum or purified IgG) was dispensed in each well and the plate was incubated for 1.5 h at 37 °C, then the plates were washed with PBS-T, and 50 μl of HRP-IgG (1/5,000 in PBS) was added to each well. After 1.5 h incubation, the plates were washed with PBS-T and the amount of the bound HRP-IgG was quantified using TMB microwell substrate.
In competitive ELISA, 50 μl of FLV sample (standard FLV solution or plasma that have been 5-fold diluted with PBS) was mixed with antibody solution and 50 μl of the mixture was dispensed into microplate wells that have been previously coated and blocked. After, the competition reaction, the signal was generated as above.
The data were acquisitioned by KC Junior software (Bio-Tek Instruments Inc., USA), and transformed to a four-parameter curve fitting using Slide Write software, version 5.011 (Advanced Graphics Software, Inc., USA). Values for IC50 were those that gave the best fit to the following equation:
A = A0 − {(A0 − A1)[FLV]/(IC50 + [FLV])} |
Where
A is the signal at a definite known concentration of FLV,
A0 is the signal in the absence of FLV,
A1 is the signal at the saturating concentration of FLV, and IC
50 is the FLV concentration that produces a 50% inhibition of the signal. The concentrations of FLV in the samples were then obtained by interpolation on the standard curve.
Results and discussion
Preparation and characterization of FLV-protein conjugates
Since FLV is a small molecule, it is not naturally immunogenic. In order to produce antibody specific to FLV, immunogenic conjugate (immunogen) must be first prepared by its covalently linking to a carrier protein. FLV contains reactive carboxylic group through which conjugation with protein could proceed directly. Although the introducing of a “spacer group” between the hapten molecule and the carrier protein usually increases the specificity of the antibody aimed to be produced,17 however the reactive COOH group of FLV is adequately spaced (6 carbon atoms) from its aromatic strong epitopic moieties18 that is characteristic for the FLV molecule. Therefore, FLV was directly linked to the carrier proteins (BSA and KLH) by carbodiimide reagent (Fig. 1).
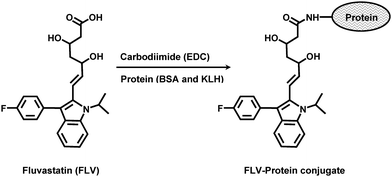 |
| Fig. 1 Preparation of FLV-protein (BSA and KLH) conjugates. | |
In order to ascertain the extent to which FLV was conjugated to the proteins, spectral analysis of the proteins and FLV-protein conjugates were conducted under the same pH conditions. The apparent molar absorptivity of FLV-protein conjugates was higher than that of the unconjugated protein. This hyperchromic effect was evident for the successful conjugation of the chromophoric FLV molecule with both BSA and KLH. The extent of conjugation was determined employing BCA reagent kit for protein assay, and the spectrophotometric procedures described by Habeeb.15 The percentages of FLV residues in FLV-BSA and FLV-KLH conjugates were found to be 24.8 and 16.5%, respectively.
Preparation and characterization of anti-FLV antibody
Because of the high immunogenicity of KLH,18 FLV-KLH was selected as immunogen for immunization of animals and FLV-BSA was selected for immobilization onto the microwell plates in the ELISA. To monitor the progress of the immune response of the rabbits and confirm that they were sufficiently immunized, serum samples were collected from the rabbits on the fourth to seventh days after each immunization, and were analyzed by direct ELISA (Fig. 2). As shown in Fig. 3A, the titers of the antisera (indicated by the absorbance values) increased with the repetitive immunizations. As well, it was observed that the reactivity of the produced antibodies to the immobilized protein (BSA) was comparable to that before immunization (Fig. 3B). These data indicated the specificity of the raised antibodies to the FLV residues in the immobilized FLV-BSA, but not BSA molecules. The measured small absorbance values were attributed to the nonspecific binding. Although, all rabbits responded well to the repetitive immunizations, however in order to select the most appropriate animal for scarifying and collecting the total sera, the affinity of these antisera from all animals were checked by competitive ELISA as described above. As shown in Fig. 4, the serum from rabbit No. 1 has the highest affinity (lowest IC50) for FLV. Therefore this rabbit was scarified and its total sera were collected as crude anti-FLV antibody, and it was purified by affinity chromatography on a protein A column.
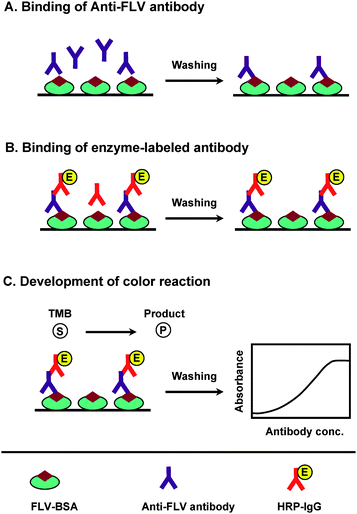 |
| Fig. 2 Schematic diagram of direct ELISA for FLV. (A) Anti-FLV antibody is dispensed into microwell plates, allowed to bind to immobilized FLV–BSA conjugate, and the excess unbound antibody is washed. (B) An enzyme-labeled secondary antibody (HRP–IgG) is added, and allowed to bind to the anti-FLV antibody. A second wash step removes unbound secondary antibody. (C) The signal is generated by the addition of TMB as a colorimetric substrate, and the measured color intensities are used for correlation with the anti-FLV antibody bound to the immobilized FLV-BSA conjugate. | |
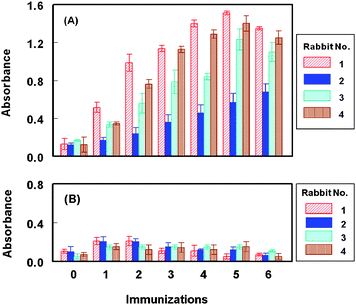 |
| Fig. 3 Monitoring the immune response of rabbits immunized with FLV-KLH. Microwell plates were coated with FLV-BSA (panel A) and BSA (panel B). Antiserum samples after different consecutive immunizations were allowed to bind to the immobilized antigen (BSA and FLV-BSA). Signals were generated as described in the Experimental section. | |
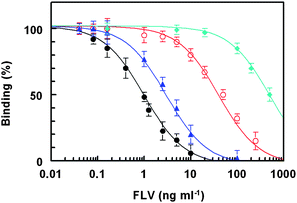 |
| Fig. 4 Affinity of antisera from rabbits No. 1 (●), No. 2 (▲), No. 3 (○), and No. 4 (♦) for FLV. FLV-BSA was coated onto the microwell plates. Antiserum samples were transferred into the microwells. Binding reaction was allowed to proceed and the signals were generated as described in the Experimental Section. | |
Specificity of anti-FLV antibody
In order to determine the optimum limiting concentration of the purified anti-FLV antibody for effective competition between free FLV (as competitor in the test sample) and immobilized FLV (FLV-BSA coated onto the plate wells), titration of anti-FLV versus the solid phase FLV-BSA was carried. The limited (not saturating) antibody concentration that gave 1–1.5 absorbance unit (in the direct ELISA) was 1 μg ml−1, respectively (Fig. 5). This concentration was used in assessment of the specificity of the anti-FLV antibody by the competitive ELISA.
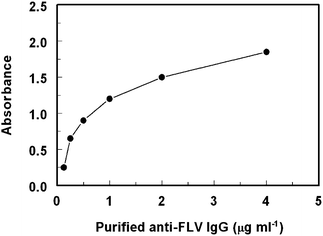 |
| Fig. 5
Titration of the purified anti-FLV IgG antibodyversus immobilized FLV-BSA conjugate. | |
The specificity of the antibody was determined by carrying out the competitive assay using various HMG-Co A reductase inhibitors (atorvastatin, pravastatin, lovastatin, and simvastatin), and various drugs that are administered in combined therapy with FLV (ezetimibe,19gemfibrozil,20aspirin,21 gemcitabine,22cholestyramine,23 and estradiol24) as competitors (Fig. 6, Table 1). The cross reactivity was calculated using the formula: IC50 (FLV)/IC50 (competitor) × 100, defining IC50 as the concentration of the competitor that causes 50% reduction of the maximum binding of anti-FLV antibody to the coating conjugates (i.e. 50% of the maximum color signal). The values of IC50 that have been obtained for all the competitors and their corresponding cross reactivities are given in Table 1. From these data, it was revealed that insignificant slight cross reactivity was exhibited by the HMG-Co A reductase inhibitors, however none of the other competitors showed any cross reactivity with FLV in the competitive assay proving the high specificity of the antibody for FLV, and its proposed ELISA for FLV determination.
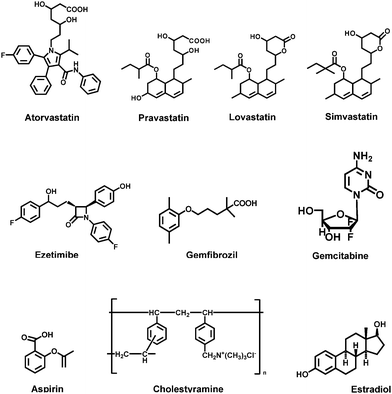 |
| Fig. 6 Competitors that have been used in the evaluation of the specificity of anti-FLV antibody. | |
Table 1 Specificity of anti-FLV antibody
Competitive ELISA for quantitation of FLV
The calibration curve of FLV using the proposed ELISA is shown in Fig. 7. This curve was generated using FLV at concentrations from 10 to 1000 pg ml−1, prepared in PBS. The data showed a good correlation coefficient (r = 0.998) on the four-parameter curve fit. The limit of detection of the proposed ELISA, defined as the lowest FLV concentration significantly different from zero concentration at 95% confidence limit (mean of zero ±4.65 SD) was determined.25 Based on the basis of 8 replicate measurements, the limit of detection in 5-fold diluted plasma samples was found to be 20 pg ml−1 and the working range was 30–500 pg ml−1. This high sensitivity enables the determination of low therapeutic concentrations of FLV in plasma.
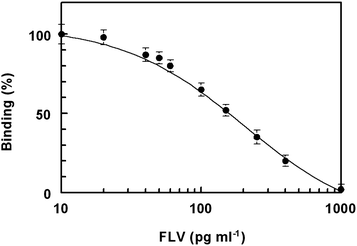 |
| Fig. 7 Calibration curve for determination of FLV in plasma by ELISA. | |
The intra-assay precision was assessed by analyzing 8 replicates of each sample in a single run and the inter-assay precision was assessed by analyzing the same sample, as duplicates, in 4 separate runs. According to the recommendation of immunoassay validation,26 the assay gave satisfactory results as the values of relative standard deviations did not exceed 5%.
Since the proposed assay was designed for quantitation of FLV in plasma, matrix effect was studied. FLV-free plasma sample was serially diluted into PBS and each dilution was spiked with 100 pg ml−1 of FLV standard. The spiked samples were then analyzed by the proposed assay to investigate the feasibility of the assay. The measured concentrations increased with the increase in the plasma dilution, and then leveled off when the plasma dilution was 5-fold. Therefore, plasma samples should be 5-fold diluted with PBS in order to avoid the possible false-positive analytical results. It is worth mentioning that the high sensitivity of the assay (limit of detection was 20 pg ml−1) allowed the high dilution of a clinical specimen to attain the FLV concentrations in the working range of the assay.
The accuracy of the method was checked by recovery studies. FLV-free plasma samples (50 μl) were spiked with 50 μl of varying concentrations of FLV, and the spiked samples were mixed with 400 μl of PBS to give final concentrations of 40–400 pg ml−1. The samples were subjected to the analysis by the competitive ELISA, and the recovery values were calculated. The overall mean analytical recovery was 97.8 ± 3.6%. This indicated the accuracy of the proposed method for determination of FLV in plasma samples, and absence of endogenous interfering substances in the plasma samples.
Conclusions
The present study described the preparation of a highly specific polyclonal antibody against FLV. The antibody recognizes FLV with high affinity. The high specificity and affinity of the produced antibody enabled the development of highly specific and sensitive ELISA for the accurate determination of FLV in plasma without pretreatment. The method is expected to contribute to the therapeutic monitoring and pharmacokinetic studies of FLV.
Acknowledgements
The authors thank King Abdulaziz City for Science and Technology for funding the work (KACS–16-98AT).
References
- D. W. Kaufman, J. P. Kelly, L. Rosenberg, T. E. Anderson and A. A. Mitchell, J. Am. Med. Assoc., 2002, 287, 337 CrossRef.
-
J. H. Block, J. M. Beale, Organic Medicinal and Pharmaceutical Chemistry, 11th edition, Lippincott Williams & Wilkins, New York, 2004, pp. 662, 663 Search PubMed.
- A. Yamamoto, K. Hoshi and K. Ichihara, Eur. J. Pharmacol., 1998, 361, 143 CrossRef CAS.
- W. R. Garnett, Am. J. Cardiol., 1996, 78, 20 CrossRef CAS.
- S. Ertürk, A. Onal and C. S. Müge, J. Chromatogr., B: Anal. Technol. Biomed. Life Sci., 2003, 793, 193 CrossRef CAS.
- S. Al-Rawithi, R. F. Hussein and A. Alzahranif, Ther. Drug Monit., 2003, 25, 88 CrossRef CAS.
- R. Nirogi, K. Mudigonda and V. Kandikere, J. Pharm. Biomed. Anal., 2007, 44, 379 CrossRef CAS.
- S. Y. Um, S. H. Jung, S. J. Jung, J. I. Kim, S. Y. Chung, H. J. Lee, S. B. Han and S. O. Choi, J. Pharm. Biomed. Anal., 2006, 41, 1458 CrossRef.
- D. P. Giuliano, C. E. Barbosa, G. T. Magalhaes, M. M. Paula and L. V. Lucia, J. Chromatogr., B: Anal. Technol. Biomed. Life Sci., 2006, 832, 256 CrossRef CAS.
- R. V. S. Nirogi, V. N. Kandikere, W. Shrivastava and K. Mudigonda, Rapid Commun. Mass Spectrom., 2006, 20, 1225 CrossRef CAS.
- H. J. Leis and W. Windischhofer, Rapid Commun. Mass Spectrom., 2005, 19, 128 CrossRef CAS.
- T. Q. Trung, P. T. Dung, N. N. Hoan, D. J. Kim, J. H. Lee and K. H. Kim, Arch. Pharm. Res., 2008, 31, 1066 Search PubMed.
- I. A. Darwish, Int. J. Biomed. Sci., 2006, 2, 217 Search PubMed.
- I. Darwish, T. Akizawa, K. Hirose, K. Omura, N. EL-Rabbata and M. Yoshioka, Anal. Chim. Acta, 1998, 365, 121 CrossRef CAS.
- A. F. Habeeb, Anal. Biochem., 1966, 14, 328.
- I. Darwish, S. Emara, H. Askal, N. N. EL-Rabbata, K. Omura, K. Hirose, T. Akizawa and M. Yoshioka, Anal. Chim. Acta, 2000, 413, 79 CrossRef CAS.
-
I. Weeks, in: G. Sevehla (Ed.), Chemiluminescence Immunoassay, Vol. XXIX, Elsevier, Amsterdam, 1992 Search PubMed.
- S. Mastronicolis, V. Kapoulas and H. Kröger, Z. Naturforsch., 1981, 36c, 459 Search PubMed.
- E. A. Stein, C. M. Ballantyne, E. Windler, P. A. Sirnes, A. Sussekov, Z. Yigit, C. Seper and C. R. Gimpelewicz, Am. J. Cardiol., 2008, 101, 490 CrossRef CAS.
- J. D. Spence, C. E. Munoz, L. Hendricks, L. Latchinian and H. E. Khouri, Am. J. Cardiol., 1995, 76, 80A CrossRef CAS.
- V. Zhdanova, S. V. Tsvirenko, S. S. Barats, O. G. Smolenskaya, R. F. Volkova and T. O. Serezhenko, Terapevtičeskij arhiv, 2002, 74, 9 Search PubMed.
- D. R. Budman and A. Calabro, Oncology, 2006, 70, 147 CrossRef CAS.
- E. Hagen, H. Istad, L. Ose, E. Bodd, H. M. Eriksen, V. Selvig, J. M. Bard, J. C. Fruchart, M. Borge and M. C Wolf, Eur. J. Clin. Pharmacol., 1994, 46, 445 CAS.
- A. O. Mueck, H. Seeger, F. U. Deuringer and D. Wallwiener, Menopause, 2001, 8, 216 CrossRef CAS.
- D. J. Anderson, Clin. Chem., 1989, 35, 2152 CAS.
- J. W. A. Findlay, W. C. Smith, J. W. Lee, G. D. Nordblom, I. Das, B. S. DeSilva, M. N. Khan and R. R. Bowsher, J. Pharm. Biomed. Anal., 2000, 21, 1249 CrossRef CAS.
|
This journal is © The Royal Society of Chemistry 2009 |