DOI:
10.1039/D2QO00128D
(Research Article)
Org. Chem. Front., 2022,
9, 2534-2540
Visible-light-driven photoredox-catalyzed C(sp3)–C(sp3) cross-coupling of N-arylamines with cycloketone oxime esters†
Received
24th January 2022
, Accepted 22nd March 2022
First published on 24th March 2022
Abstract
A novel photoredox-catalyzed C(sp3)–C(sp3) cross-coupling between N-arylamines and cycloketone oxime esters under mild conditions has been accomplished. The redox-neutral reaction proceeds with good functional group tolerance and excellent regioselectivity without any extra base or ligand control. In addition, this approach was successfully applied for the late-stage modification of short peptides and analogues.
Introduction
Functional amines are regarded as an important class of structural motifs that exist in a large number of pharmaceutically important molecules and natural products. Due to their rich chemical and biological activities, the discovery of a general methodology for the preparation or modification of these compounds has attracted more and more attention.1,2 Among them, the alkylation of N-arylamines is one of the most efficient pathways to produce N-containing organic compounds, which introduce a C(sp3) moiety to change the spatial conformation, and increase both the lipophilicity and hydrophilicity as drug candidates. A typical method for the alkylation of N-arylamines mainly relies on CDC (cross-dehydrogenative coupling) reactions;3 although significant advances have been made along this line, there are still some considerable limitations: (1) most approaches rely heavily on activated alkyl substrates, whose C(sp3)–H bonds are adjacent to the heteroatom (such as O, S and N) or the electron-withdrawing group (such as a nitrile, an ester, a ketone and an amide) and (2) super-stoichiometric oxidants and high temperatures are usually required, leading to poor chemoselectivity and atom economy. Therefore, the development of mild and sustainable synthetic strategies to accomplish challenging C(sp3)–C(sp3) cross-coupling through α-amino C–H functionalization is still highly desirable.
Visible light photocatalysis has been developed as a powerful tool for organic transformation due to its environmental friendliness and sustainability,4,5 and recently applied in alkylation of N-arylamines.6 In 2017, Yu and co-workers reported a novel visible-light-driven palladium-catalyzed radical alkylation of N-arylamines with alkyl bromides; various tertiary, secondary, and primary unactivated alkyl bromides undergo this simple reaction for the construction of quaternary centers (Scheme 1a).6a Soon after, Cong and co-workers developed the visible light photoredox decarboxylative alkylation of N-arylamines with N-(acyloxy)phthalimides, using erythrosine B sensitized TiO2 as the photocatalyst.6b Zhang and co-workers described a similar decarboxylative alkylation through the photoactivation of an electron donor–acceptor (EDA) complex (Scheme 1b).6c In addition to alkyl bromides and N-(acyloxy)phthalimides, Katritzky salts also proved to be efficient, with regard to alkyl radical precursors in the visible-light-mediated deaminative alkylation of N-arylamines (Scheme 1c).6d,e Although these methods provide an efficient way to access alkylation of N-arylamines, they mainly focus on such three active alkyl radical sources, the distal unactive alkyl radical for the alkylation of N-arylamines was still rare.
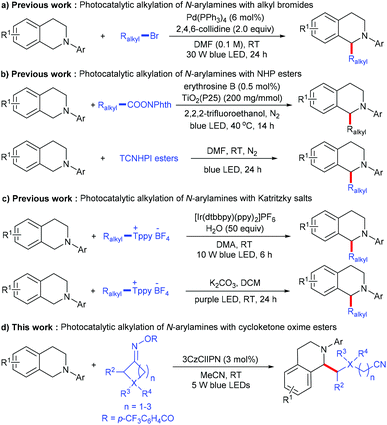 |
| Scheme 1 Photocatalytic radical alkylation of N-arylamines. | |
Recently, redox-active cycloketone oxime esters have been intensively explored as radical precursors under transition-metal or photoredox catalysis via C–C bond activation.7,8 In 2021, Gong, Lu and co-workers developed a direct C–H cyanoalkylation of glycine derivatives with cyclobutanone oxime esters; the key of this transformation is the combination of Fe(NTf2)2 and pyridine-oxazoline ligands as an efficient catalytic system. Moreover, the 2-amino carbonyl moiety was essential to achieve chelation between the catalyst system and glycine derivatives.9 Inspired by the above work, we wondered whether it would be possible to exploit the radical coupling of N-arylamines and cycloketone oxime esters under photoredox conditions.
Herein, we report a novel metal-free C(sp3)–C(sp3) cross-coupling between N-arylamines and cycloketone oxime esters involving direct C(sp3)–H functionalization; various N-arylamines and cycloketone oxime esters can be transformed into the corresponding products in good to excellent yields under mild conditions (Scheme 1d). This reaction proceeds via a sequence of the iminyl radical generation, C–C bond cleavage to produce a cyanoalkyl radical, single electron transfer to form a nitrogen radical cation, deprotonation and radical–radical coupling.
Results and discussion
We started our investigations using 2-phenyl-1,2,3,4-tetrahydroisoquinoline 1a and cyclobutylidenemethyl 4-(trifluoromethyl)benzoate 2a as the model reaction partners to clarify the standard reaction conditions (Table 1). We found that N-arylamine 1a underwent successfully the α-amino C–H functionalization with cycloketone oxime ester 2a, with 3CzClIPN as a photocatalyst and acetonitrile as a solvent at room temperature under irradiation with blue LEDs for 12 h, affording the desired 4-(2-phenyl-1,2,3,4-tetrahydroisoquinolin-1-yl)butanenitrile 3aa in 86% isolated yield (entry 1). The reaction did not occur in the absence of irradiation or a photocatalyst (entries 2 and 3). The photocatalyst as a crucial factor was investigated; metal photocatalysts such as fac-Ir(ppy)3 and Ru(bpy)3Cl2 resulted in good catalytic activity but were less efficient than 3CzClIPN (entries 4 and 5). Other organophotocatalysts including Eosin Y, [Acr-Mes]+(ClO4)−, rhodamine B and methylene blue all turned out to be inferior to 3CzClIPN (entries 6–9). Screening of photocatalyst loading revealed that a lower catalytic amount of 3CzClIPN (1 mol%) reduced the yield sharply and a higher catalytic amount (5 mol%) affected the yield slightly (entries 10 and 11). When other solvents such as dichloroethane, methanol, toluene and DMSO were employed to replace acetonitrile, a notable decrease of the yield was observed (entries 12–15). To our delight, the reaction scaled up to 1g of 2-phenyl-1,2,3,4-tetrahydroisoquinoline 1a also smoothly delivered 3aa in 80% yield (entry 16).
Table 1 Screening of the optimal reaction conditionsa
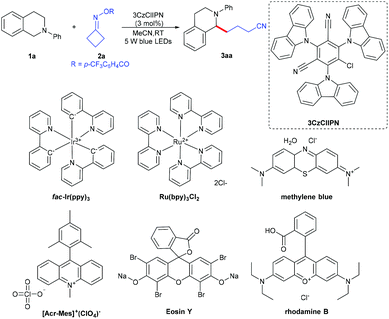
|
Entry |
Variation from the standard conditions |
Yield (%) |
Reaction conditions: 1a (0.2 mmol), 2a (0.24 mmol), 3CzClIPN (3 mol%), MeCN (2 mL), RT, 5 W blue LEDs, argon, and 12 h.
1a (1 g) for 36 h.
|
1 |
None |
86 |
2 |
Without blue LEDs |
0 |
3 |
Without 3CzClIPN |
0 |
4 |
fac-Ir(ppy)3 instead of 3CzClIPN |
41 |
5 |
Ru(bpy)3Cl2 instead of 3CzClIPN |
58 |
6 |
Eosin Y instead of 3CzClIPN |
38 |
7 |
[Acr-Mes]+(ClO4)− instead of 3CzClIPN |
40 |
8 |
Rhodamine B instead of 3CzClIPN |
19 |
9 |
Methylene blue instead of 3CzClIPN |
58 |
10 |
3CzClIPN (1 mol%) |
48 |
11 |
3CzClIPN (5 mol%) |
87 |
12 |
DCE instead of MeCN |
61 |
13 |
MeOH instead of MeCN |
65 |
14 |
Toluene instead of MeCN |
13 |
15 |
DMSO instead of MeCN |
43 |
16b |
None |
80 |
With the optimized reaction conditions in hand, we evaluated the generality of this reaction with N-aryl tetrahydroisoquinolines 1 and cycloketone oxime esters 2. As shown in Table 2, the substrates bearing different kinds of groups including methyl 1b, methoxy 1c, fluoro 1d, bromo 1e and trifluoromethyl 1f groups at the para position of the N-phenyl ring were well tolerated, affording the C(sp3)–C(sp3) coupling products 3ba–3fa in 48%–84% yields; the ortho and meta position substituents 1g and 1h did not affect the reaction and produced the corresponding products in good yields. Furthermore, substrate 1i with the naphthalene group at the nitrogen atom was also highly active. 2-Methyl-1,2,3,4-tetrahydroisoquinoline 1j was not suitable under the standard conditions; the N-aryl group might be essential for the electron transfer. Several kinds of electron-donating and electron-withdrawing groups 1k–1o at different positions of the benzene ring smoothly transformed into selective alkylation products in 72%–93% yields. A series of cycloketone oxime esters 2b–2i could serve as suitable substrates to afford the desired products 3ab–3ai under mild conditions. Symmetric monosubstituted cyclobutanone oximes bearing various functional groups such as alkyl 2b, ester 2c, and phenyl 2d substituents at the 3-position all underwent ring-opening processes to give the corresponding products 3ab–3ad in 68%–81% yields. The disubstituted cycloketone oxime ester 2e was compatible with the reaction. The heterocyclobutanone oxime esters oxetan-3-one 2f and 1-Boc-3-azetidinone 2g also showed comparable reactivity, delivering the corresponding products 3af and 3ag in 82% and 70% yields. To our surprise, this protocol was not only suitable for four-membered ring oxime esters, but other less-strained substrates such as cyclopentanone 2h and cyclohexanone oxime ester 2i were also converted to the desired products in 65% and 53% yields.
Table 2 Variation of the N-aryl tetrahydroisoquinolines and cycloketone oxime esters (2)a
Reaction conditions: 1 (0.2 mmol), 2 (0.24 mmol), 3CzClIPN (3 mol%), MeCN (2 mL), RT, 5 W blue LEDs, argon, and 12 h.
|
|
We further explored the scope of α-amino carbonyl compounds 4 with cycloketone oxime ester 2a (Table 3). The ethyl 4a, tertiary butyl 4b and benzyl 4c esters of N-aryl glycine were amenable for this catalytic system, giving the corresponding products in 61%–91% yields. Various functional groups 4d–4j on the benzene ring of glycine derivatives were examined; the results showed that electron-donating groups performed better than electron-withdrawing groups, and the position nature had no detrimental effect on the reaction. The dimethyl-substituted and naphthalenyl groups of glycine esters 4k–4m were also tolerated to give the corresponding products 5ka–5ma in 74%–90% yields. Not only glycine esters, other α-amino carbonyl compounds such as amide 4n and ketone 4o could also smoothly transform into the desired products in excellent yields, which indicated that this protocol could be used to modify peptides. Even N-benzylaniline 4p could successfully give the alkylation product 5pa in 41% yield. Unfortunately, N-phenylglycinonitrile 4q was not suitable for this transformation.
Table 3 Variation of the α-amino carbonyl compounds (4)a
Reaction conditions: 4 (0.2 mmol), 2a (0.24 mmol), 3CzClIPN (3 mol%), MeCN (2 mL), RT, 5 W blue LEDs, argon, and 12 h.
|
|
Next, the practicability of these mild conditions for the site-selective modification of peptides was tested (Table 4). N-Phenyl protected dipeptide 4r was successfully coupled with cycloketone oxime ester 2a and heterocyclobutanone oxime ester 2f in good yields. Other dipeptides 4s–4u bearing different units also selectively underwent alkylation at the α-position of glycine carbonyl in 71%–75% yields. Even substrate 4v containing the sensitive thiomethyl group could smoothly transform into the corresponding product 5va in a moderate yield.
Table 4 Site-selective modification of short peptidesa
Reaction conditions: 4 (0.2 mmol), 2 (0.24 mmol), 3CzClIPN (3 mol%), MeCN (2 mL), RT, 5 W blue LEDs, argon, and 12 h.
|
|
To gain insight into this photoredox-catalyzed cross coupling reaction, mechanistic studies were conducted with radical trapping experiments. When TEMPO, hydroquinone and BHT were added into the standard conditions, the reaction was completely suppressed (Scheme 2a), and the radical generated from cycloketone oxime ester 2a was trapped by TEMPO to give product 6 in 19% yield; the radicals from substrates 1a and 2a could be identified through HRMS (see the ESI†). In addition, Stern–Volmer quenching studies indicated that N-aryl tetrahydroisoquinoline 1a quenched the excited state of 3CzClIPN (Scheme 2b). These results implied that the reaction involves a radical process.
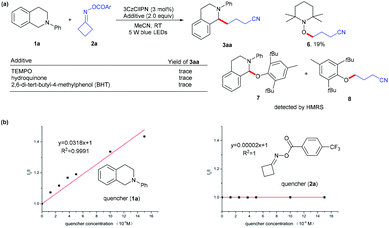 |
| Scheme 2 Control experiments. | |
Based on the obtained results and previous reports,6 a plausible mechanism for this visible-light-driven photoredox-catalyzed C(sp3)–C(sp3) cross-coupling reaction was proposed, as shown in Scheme 3. Firstly, the photocatalyst 3CzClIPN is excited under visible light irradiation to produce its excited state species 3CzClIPN* (E1/2 [PC*/PC˙−] = +1.56 V vs. SCE in MeCN),4j which oxidizes N-arylamine 1a (Ep0/+1(1a) = +1.04 V versus SCE in MeCN)6p to give radical cation A and 3CzClIPN˙−. 3CzClIPN˙− (E1/2 [PC/PC˙−] = −1.16 V vs. SCE in MeCN) undergoes another SET process with cycloketone oxime ester 2a (Ep0/–1(2a) = −0.99 V versus SCE in MeCN)8j to form iminyl radical B and the 4-(trifluoromethyl)benzoate anion, along with the regeneration of 3CzClIPN. Subsequently, iminyl radical B undergoes a ring-opening to give the highly reactive cyanoalkyl radical C; radical cation A undergoes deprotonation with the 4-(trifluoromethyl)benzoate anion to produce the benzylic radical D. Finally, radical–radical coupling between intermediate C and intermediate D provides the desired product 3aa.
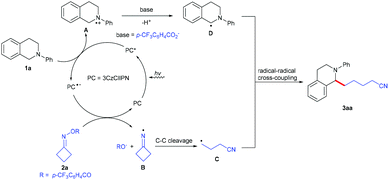 |
| Scheme 3 Possible mechanism. | |
Conclusions
In summary, we have developed a new site selective photoredox-catalyzed C(sp3)–C(sp3) cross-coupling between N-arylamines and cycloketone oxime esters without employing any extra base or ligand control. A variety of N-aryl tetrahydroisoquinolines, glycine derivatives and cycloketone oxime esters can be transformed into the corresponding products in good to excellent yields. In addition, this protocol is also successfully applied in the alkylated modification of short peptides, which is highly attractive for medicinal chemistry and organic synthesis.
Conflicts of interest
There are no conflicts to declare.
Acknowledgements
We thank the National Natural Science Foundation of China (No. 22001108) and Jiangxi Province Science and Technology Project (No. 20212BAB203025) for the financial support.
Notes and references
- For selected examples, see:
(a) A. Cabré, X. Verdaguer and A. Riera, Recent Advances in the Enantioselective Synthesis of Chiral Amines via Transition Metal-Catalyzed Asymmetric Hydrogenation, Chem. Rev., 2022, 122, 269–339 CrossRef;
(b) S. France, D. J. Guerin, S. J. Miller and T. Lectka, Nucleophilic Chiral Amines as Catalysts in Asymmetric Synthesis, Chem. Rev., 2003, 103, 2985–3012 CrossRef CAS PubMed;
(c) G. Guillena, D. J. Ramón and M. Yus, Hydrogen Autotransfer in the N-Alkylation of Amines and Related Compounds using Alcohols and Amines as Electrophiles, Chem. Rev., 2010, 110, 1611–1641 CrossRef CAS PubMed;
(d) P. Kalck and M. Urrutigoïty, Tandem Hydroaminomethylation Reaction to Synthesize Amines from Alkenes, Chem. Rev., 2018, 118, 3833–3861 CrossRef CAS PubMed;
(e) T. E. Müller, K. C. Hultzsch, M. Yus, F. Foubelo and M. Tada, Hydroamination: Direct Addition of Amines to Alkenes and Alkynes, Chem. Rev., 2008, 108, 3795–3892 CrossRef PubMed;
(f) A. Trowbridge, S. M. Walton and M. J. Gaunt, New Strategies for the Transition-Metal Catalyzed Synthesis of Aliphatic Amines, Chem. Rev., 2020, 120, 2613–2692 CrossRef CAS.
- For selected reviews and examples, see:
(a) W. Ding, L.-Q. Lu, J. Liu, D. Liu, H.-T. Song and W.-J. Xiao, Visible Light Photocatalytic Radical–Radical Cross-Coupling Reactions of Amines and Carbonyls: A Route to 1,2-Amino Alcohols, J. Org. Chem., 2016, 81, 7237–7243 CrossRef CAS PubMed;
(b) X. Li, M. Chen, X. Xie, N. Sun, S. Li and Y. Liu, Synthesis of Multiple-Substituted Pyrroles via Gold(I)-Catalyzed Hydroamination/Cyclization Cascade, Org. Lett., 2015, 17, 2984–2987 CrossRef CAS;
(c) J. Liu, Y. Song, X. Wu and L. Ma,
N-Dimethylation and N-Functionalization of Amines Using Ru Nanoparticle Catalysts and Formaldehyde or Functional Aldehydes as the Carbon Source, ACS Omega, 2021, 6, 22504–22513 CrossRef CAS PubMed;
(d) H. T. Ten Brink, J. T. Meijer, R. V. Geel, M. Damen, D. W. P. M. Löwik and J. C. M. van Hest, Solid-Phase Synthesis of C-Terminally Modified Peptides, J. Pept. Sci., 2006, 12, 686–692 CrossRef CAS PubMed;
(e) W.-T. Wei, F. Teng, Y. Li, R.-J. Song and J.-H. Li, Oxidative [4+2] Cycloaddition of α-(N-Arylamino) Carbonyls with Aryl Alkenes by Multiple C-H Functionalizations and [1,2]-Aryl Shifts, Org. Lett., 2019, 21, 6285–6288 CrossRef CAS PubMed;
(f) H. Deng, H. Li, W. Zhang and L. Wang, RhIII-Catalyzed Site-Selective Amidation with Nitrone as a Traceless Directing Group: An Approach to Functionalized Arylaldehydes, Chem. Commun., 2017, 53, 10322–10325 RSC;
(g) Z.-W. Hou, L. Li and L. Wang, Organocatalytic Electrochemical Amination of Benzylic C-H Bonds, Org. Chem. Front., 2021, 8, 4700–4705 RSC.
- For selected reviews, see:
(a) J. Chen, Y.-J. Liang, P.-Z. Wang, G.-Q. Li, B. Zhang, H. Qian, X.-D. Huan, W. Guan, W.-J. Xiao and J.-R. Chen, Photoinduced Copper-Catalyzed Asymmetric C-O Cross-Coupling, J. Am. Chem. Soc., 2021, 143, 13382–13392 CrossRef CAS PubMed;
(b) X. Liu, J. Pu, X. Luo, X. Cui, Z. Wu and G. Huang, CBr4-Mediated Cross-Coupling Reactions of α-Amino Carbonyl Compounds with Alcohols and Thiols to Build C-O and C-S Bonds, Respectively, Org. Chem. Front., 2018, 5, 361–365 RSC;
(c) X.-X. Liu, Z.-Y. Wu, Y.-Q. He, X.-Q. Zhou, T. Hu, C.-W. Ma and G.-S. Huang, Copper-Catalyzed C-N Bond Formation via Oxidative Cross-Coupling of Amines with α-Aminocarbonyl Compounds, Adv. Synth. Catal., 2016, 358, 2385–2391 CrossRef CAS;
(d) M. R. Mutra, G. K. Dhandabani and J.-J. Wang, Mild Access to N-Formylation of Primary Amines using Ethers as C1 Synthons under Metal-Free Conditions, Adv. Synth. Catal., 2018, 360, 3960–3968 CrossRef CAS;
(e) H. Peng, J.-T. Yu, Y. Jiang, H. Yang and J. Cheng, Di-tert-butyl Peroxide-Promoted α-Alkylation of α-Amino Carbonyl Compounds by Simple Alkanes, J. Org. Chem., 2014, 79, 9847–9853 CrossRef CAS PubMed;
(f) M. San Segundo and A. Correa, Site-Selective Cu-Catalyzed Alkylation of α-Amino Acids and Peptides toward the Assembly of Quaternary Centers, ChemSusChem, 2018, 11, 3893–3898 CrossRef CAS PubMed;
(g) D. Tang, X. Guo, Y. Wang, J. Wang, J. Li, Q. Huang and B. Chen, Metal Free, I2-Catalyzed [3+1+1] Cycloaddition Reactions to Synthesize 1,2,4-Trisubstituted Imidazoles, Tetrahedron Lett., 2015, 56, 5982–5985 CrossRef CAS;
(h) H. Tian, W. Xu, Y. Liu and Q. Wang, Unnatural α-Amino Acid Synthesized through α-Alkylation of Glycine Derivatives by Diacyl Peroxides, Org. Lett., 2020, 22, 5005–5008 CrossRef CAS;
(i) G.-Z. Wang, M.-C. Fu, B. Zhao and R. Shang, Photocatalytic Decarboxylative Alkylations of C(sp3)-H and C(sp2)-H Bonds Enabled by Ammonium Iodide in Amide Solvent, Sci. China: Chem., 2021, 64, 439–444 CrossRef CAS;
(j) Z.-H. Wang, P.-S. Gao, X. Wang, J.-Q. Gao, X.-T. Xu, Z. He, C. Ma and T.-S. Mei, TEMPO-Enabled Electrochemical Enantioselective Oxidative Coupling of Secondary Acyclic Amines with Ketones, J. Am. Chem. Soc., 2021, 143, 15599–15605 CrossRef CAS PubMed;
(k) W.-T. Wei, H.-B. Li, R.-J. Song and J.-H. Li, Copper-Catalyzed Oxidative Oxyalkylation of Enol ethers with α-Amino Carbonyl Compounds and Hydroperoxides, Chem. Commun., 2015, 51, 11325–11328 RSC;
(l) W.-T. Wei, R.-J. Song and J.-H. Li, Copper-Catalyzed Oxidative α-Alkylation of α-Amino Carbonyl Compounds with Ethers via Dual C(sp3)-H Oxidative Cross-Coupling, Adv. Synth. Catal., 2014, 356, 1703–1707 CrossRef CAS;
(m) X.-H. Wei, G.-W. Wang and S.-D. Yang, Enantioselective Synthesis of Arylglycine Derivatives by Direct C-H Oxidative Cross-Coupling, Chem. Commun., 2015, 51, 832–835 RSC;
(n) J.-C. Wu, R.-J. Song, Z.-Q. Wang, X.-C. Huang, Y.-X. Xie and J.-H. Li, Copper-Catalyzed C-H Oxidation/Cross-Coupling of α-Amino Carbonyl Compounds, Angew. Chem., Int. Ed., 2012, 51, 3453–3457 CrossRef CAS PubMed;
(o) L. Yang, Z. Qiu, J. Wu, J. Zhao, T. Shen, X. Huang and Z.-Q. Liu, Molecular Oxygen-Mediated Radical Alkylation of C(sp3)-H Bonds with Boronic Acids, Org. Lett., 2021, 23, 3207–3210 CrossRef CAS PubMed;
(p) M. Zhu, D. Chen, S. Zeng, C. Xing, W. Deng, J. Xiang and R.-J. Wang, Synthesis of (Z)-Nitroalkene Derivatives through Oxidative Dehydrogenation coupling of α-Aminocarbonyl Compounds with Nitromethane by Copper Catalysis, Tetrahedron Lett., 2018, 59, 3214–3219 CrossRef CAS;
(q) Y. Li, B. Liu, H.-B. Li, Q. Wang and J.-H. Li, Oxidative Radical 1,2-Alkylarylation of Alkenes with α-C(sp3)-H Bonds of Acetonitriles Involving 1,2-Aryl Migration, Chem. Commun., 2015, 51, 1024–1026 RSC;
(r) B. Wei, J.-H. Qin, Y.-Z. Yang, Y.-X. Xie, X.-H. Ouyang and R.-J. Song, Electrochemical Radical C(sp3)-H Arylation of Xanthenes with Electron-Rich Arenes, Org. Chem. Front., 2022, 9, 816–821 RSC.
- For selected examples, see:
(a) R. Asahi, T. Morikawa, H. Irie and T. Ohwaki, Nitrogen-Doped Titanium Dioxide as Visible-Light-Sensitive Photocatalyst: Designs, Developments, and Prospects, Chem. Rev., 2014, 114, 9824–9852 CrossRef CAS;
(b) F. Juliá, T. Constantin and D. Leonori, Applications of Halogen-Atom Transfer (XAT) for the Generation of Carbon Radicals in Synthetic Photochemistry and Photocatalysis, Chem. Rev., 2022, 122, 2292–2352 CrossRef PubMed;
(c) K. Kwon, R. T. Simons, M. Nandakumar and J. L. Roizen, Strategies to Generate Nitrogen-Centered Radicals That May Rely on Photoredox Catalysis: Development in Reaction Methodology and Applications in Organic Synthesis, Chem. Rev., 2022, 122, 2353–2428 CrossRef CAS;
(d) S. P. Pitre and L. E. Overman, Strategic Use of Visible-Light Photoredox Catalysis in Natural Product Synthesis, Chem. Rev., 2022, 122, 1717–1751 CrossRef CAS PubMed;
(e) C. K. Prier, D. A. Rankic and D. W. C. MacMillan, Visible Light Photoredox Catalysis with Transition Metal Complexes: Applications in Organic Synthesis, Chem. Rev., 2013, 113, 5322–5363 CrossRef CAS PubMed;
(f) K. P. S. Cheung, S. Sarkar and V. Gevorgyan, Visible Light-Induced Transition Metal Catalysis, Chem. Rev., 2022, 122, 1543–1625 CrossRef PubMed;
(g) X.-Y. Yu, J.-R. Chen and W.-J. Xiao, Visible Light-Driven Radical-Mediated C-C Bond Cleavage/Functionalization in Organic Synthesis, Chem. Rev., 2021, 121, 506–561 CrossRef CAS PubMed;
(h) J.-Y. Chen, Y.-H. Li, L. Mei and H.-Y. Wu, Application of Photosensitizer 2,4,5,6-Tetrakis(carbazol-9-yl)-1,3-dicyanobenzene in Photo-Induced Transition-Metal-Free Organic Synthesis, Chin. J. Org. Chem., 2019, 39, 3040–3050 CrossRef CAS;
(i) Y. Chen, L.-Q. Lu, D.-G. Yu, C.-J. Zhu and W.-J. Xiao, Visible Light-Driven Organic Photochemical Synthesis in China, Sci. China: Chem., 2019, 62, 24–57 CrossRef CAS;
(j) E. Speckmeier, T. G. Fischer and K. Zeitler, A Toolbox Approach to Construct Broadly Applicable Metal-Free Catalysts for Photoredox Chemistry: Deliberate Tuning of Redox Potentials and Importance of Halogens in Donor-Acceptor Cyanoarenes, J. Am. Chem. Soc., 2018, 140, 15353–15365 CrossRef CAS PubMed.
- For selected examples, see:
(a) T. Chatterjee, J. Y. Cho and E. J. Cho, Synthesis of Substituted Oxazoles by Visible-Light Photocatalysis, J. Org. Chem., 2016, 81, 6995–7000 CrossRef CAS PubMed;
(b) Y. Pan, S. Wang, C. W. Kee, E. Dubuisson, Y. Yang, K. P. Loh and C.-H. Tan, Graphene Oxide and Rose Bengal: Oxidative C-H Functionalisation of Tertiary Amines Using Visible Light, Green Chem., 2011, 13, 3341–3344 RSC;
(c) B. Sun, J. Deng, D. Li, C. Jin and W. Su, Photocatalytic Aerobic Cross-Coupling Reaction of N-Substituted Anilines with N-Aryl Glycine Esters: Synthesis of α-Aryl α-Amino Esters, Tetrahedron Lett., 2018, 59, 4364–4369 CrossRef CAS;
(d) C. Wang, R. Qi, H. Xue, Y. Shen, M. Chang, Y. Chen, R. Wang and Z. Xu, Visible-Light-Promoted C(sp3)-H Alkylation by Intermolecular Charge Transfer: Preparation of Unnatural α-Amino Acids and Late-Stage Modification of Peptides, Angew. Chem., Int. Ed., 2020, 59, 7461–7466 CrossRef CAS PubMed;
(e) H. Xue, M. Guo, C. Wang, Y. Shen, R. Qi, Y. Wu, Z. Xu and M. Chang, Photo-Induced Preparation of Unnatural α-Amino Acids: Synthesis and Characterization of Novel Leu5-Enkephalin Analogues, Org. Chem. Front., 2020, 7, 2426–2431 RSC;
(f) J. Wang, Y. Su, Z. Quan, J. Li, J. Yang, Y. Yuan and C. Huo, Visible-Light Promoted α-Alkylation of Glycine Derivatives with Alkyl Boronic Acids, Chem. Commun., 2021, 57, 1959–1962 RSC;
(g) Y.-X. Chen, Z.-J. Wang, J.-A. Xiao, K. Chen, H.-Y. Xiang and H. Yang, Visible-Light-Driven Sulfonation of α-Trifluoromethylstyrenes: Access to Densely Functionalized CF3-Substituted Tertiary Alcohol, Org. Lett., 2021, 23, 6558–6562 CrossRef CAS;
(h) X.-J. Liu, S.-Y. Zhou, Y. Xiao, Q. Sun, X. Lu, Y. Li and J.-H. Li, Photocatalytic Decarboxylative [3+2] and [4+2] Annulation of Enynals and γ,σ-Unsaturated N-(Acyloxy)phthalimides by NaI/PPh3 Catalysis, Org. Lett., 2021, 23, 7839–7844 CrossRef CAS PubMed;
(i) P.-Z. Wang, Y. Gao, J. Chen, X.-D. Huan, W.-J. Xiao and J.-R. Chen, Asymmetric Three-Component Olefin Dicarbofunctionalization Enabled by Photoredox and Copper Dual Catalysis, Nat. Commun., 2021, 12, 1815 CrossRef CAS;
(j) C. Zhu, S. Zhumagazy, H. Yue and M. Rueping, Metal-Free C–Se Cross-Coupling Enabled by Photoinduced Inter-Molecular Charge Transfer, Chem. Commun., 2022, 58, 96–99 RSC.
-
(a) W.-J. Zhou, G.-M. Cao, G. Shen, X.-Y. Zhu, Y.-Y. Gui, J.-H. Ye, L. Sun, L.-L. Liao, J. Li and D.-G. Yu, Visible-Light-Driven Palladium-Catalyzed Radical Alkylation of C-H Bonds with Unactivated Alkyl Bromides, Angew. Chem., Int. Ed., 2017, 56, 15683–15687 CrossRef CAS PubMed;
(b) L. Ren and H. Cong, Visible-Light-Driven Decarboxylative Alkylation of C–H Bond Catalyzed by Dye-Sensitized Semiconductor, Org. Lett., 2018, 20, 3225–3228 CrossRef CAS PubMed;
(c) P. Ma, Y. Liu, L. Chen, X. Zhao, B. Yang and J. Zhang, Photocatalyst- and Additive-Free Decarboxylative Alkylation of N-Aryl Tetrahydroisoquinolines Induced by Visible Light, Org. Chem. Front., 2021, 8, 2473–2479 RSC;
(d) Y. Xu, Z.-J. Xu, Z.-P. Liu and H. Lou, Visible-Light-Mediated De-Aminative Alkylation of N-Arylamines with Alkyl Katritzky Salts, Org. Chem. Front., 2019, 6, 3902–3905 RSC;
(e) Q. Xia, Y. Li, X. Wang, P. Dai, H. Deng and W.-H. Zhang, Visible Light-Driven α-Alkylation of N-Aryl Tetrahydroisoquinolines Initiated by Electron Donor-Acceptor Complexes, Org. Lett., 2020, 22, 7290–7294 CrossRef CAS PubMed;
(f) J. Dhineshkumar, M. Lamani, K. Alagiri and K. R. Prabhu, A Versatile C-H Functionalization of Tetrahydroisoquinolines Catalyzed by Iodine at Aerobic Conditions, Org. Lett., 2013, 15, 1092–1095 CrossRef CAS PubMed;
(g) Z. Džambaski and B. P. Bondžić, Dehydrogenative C(sp3)-H Bond Functionalization of Tetrahydroisoquinolines Mediated by Organic Oxidants under Mild Conditions, Org. Biomol. Chem., 2019, 17, 6420–6425 RSC;
(h) H. E. Ho, Y. Ishikawa, N. Asao, Y. Yamamoto and T. Jin, Highly Efficient Heterogeneous Aerobic Cross-Dehydrogenative Coupling via C-H Functionalization of Tertiary Amines Using a Nanoporous Gold Skeleton Catalyst, Chem. Commun., 2015, 51, 12764–12767 RSC;
(i) Z. Li and C.-J. Li, CuBr-Catalyzed Direct Indolation of Tetrahydroisoquinolines via Cross-Dehydrogenative Coupling between sp3 C-H and sp2 C-H Bonds, J. Am. Chem. Soc., 2005, 127, 6968–6969 CrossRef CAS PubMed;
(j) W. Liang, T. Zhang, Y. Liu, Y. Huang, Z. Liu, Y. Liu, B. Yang, X. Zhou and J. Zhang, Polydimethylsiloxane Sponge-Supported Nanometer Gold: Highly Efficient Recyclable Catalyst for Cross-Dehydrogenative Coupling in Water, ChemSusChem, 2018, 11, 3586–3590 CrossRef CAS PubMed;
(k) H. Tian, W. Xu, Y. Liu and Q. Wang, Radical Alkylation of C(sp3)-H Bonds with Diacyl Peroxides under Catalyst-Free Conditions, Chem. Commun., 2019, 55, 14813–14816 RSC;
(l) C. Wang, M. Guo, R. Qi, Q. Shang, Q. Liu, S. Wang, L. Zhao, R. Wang and Z. Xu, Visible-Light-Driven, Copper-Catalyzed Decarboxylative C(sp3)-H Alkylation of Glycine and Peptides, Angew. Chem., Int. Ed., 2018, 57, 15841–15846 CrossRef CAS PubMed;
(m) J. Xuan, T.-T. Zeng, Z.-J. Feng, Q.-H. Deng, J.-R. Chen, L.-Q. Lu, W.-J. Xiao and H. Alper, Redox-Neutral α-Allylation of Amines by Combining Palladium Catalysis and Visible-Light Photoredox Catalysis, Angew. Chem., Int. Ed., 2015, 54, 1625–1628 CrossRef CAS PubMed;
(n) W. Zhang, S. Yang and Z. Shen, Copper-Catalyzed Cyanomethylation of Substituted Tetrahydroisoquinolines with Acetonitrile, Adv. Synth. Catal., 2016, 358, 2392–2397 CrossRef CAS;
(o) S.-S. Zhu, Y. Liu, X.-L. Chen, L.-B. Qu and B. Yu, Polymerization-Enhanced Photocatalysis for the Functionalization of C(sp3)-H Bonds, ACS Catal., 2022, 12, 126–134 CrossRef CAS;
(p) S.-S. Zhu, Y. Liu, X.-L. Chen, L.-B. Qu and B. Yu, Polymerization-Enhanced Photocatalysis for the Functionalization of C(sp3)-H Bonds, ACS Catal., 2022, 12, 126–134 CrossRef CAS.
- W. Xiao and J. Wu, Recent Advances for the Photoinduced C-C Bond Cleavage of Cycloketone Oximes, Chin. Chem. Lett., 2020, 31, 3083–3094 CrossRef CAS.
-
(a) J. Chen, Y.-J. Liang, P.-Z. Wang, G.-Q. Li, B. Zhang, H. Qian, X.-D. Huan, W. Guan, W.-J. Xiao and J.-R. Chen, Photoinduced Copper-Catalyzed Asymmetric C-O Cross-Coupling, J. Am. Chem. Soc., 2021, 143, 13382–13392 CrossRef CAS PubMed;
(b) H. Qian, J. Chen, B. Zhang, Y. Cheng, W.-J. Xiao and J.-R. Chen, Visible-Light-Driven Photoredox-Catalyzed Three-Component Radical Cyanoalkylfluorination of Alkenes with Oxime Esters and a Fluoride Ion, Org. Lett., 2021, 23, 6987–6992 CrossRef CAS PubMed;
(c) B. Shuai, P. Fang and T.-S. Mei, Nickel-Catalyzed Favorskii-Type Rearrangement of Cyclobutanone Oxime Esters to Cyclopropanecarbonitriles, Synlett, 2021, 32, 1637–1641 CrossRef CAS;
(d) P.-Z. Wang, X. Wu, Y. Cheng, M. Jiang, W.-J. Xiao and J.-R. Chen, Photoinduced Copper-Catalyzed Asymmetric Three-Component Coupling of 1,3-Dienes: An Alternative to Kharasch-Sosnovsky Reaction, Angew. Chem., Int. Ed., 2021, 60, 22956–22962 CrossRef CAS PubMed;
(e) X.-Y. Yu, J.-R. Chen, P.-Z. Wang, M.-N. Yang, D. Liang and W.-J. Xiao, A Visible-Light-Driven Iminyl Radical-Mediated C-C Single Bond Cleavage/Radical Addition Cascade of Oxime Esters, Angew. Chem., Int. Ed., 2018, 57, 738–743 CrossRef CAS PubMed;
(f) X.-Y. Yu, Q.-Q. Zhao, J. Chen, J.-R. Chen and W.-J. Xiao, Copper-Catalyzed Radical Cross-Coupling of Redox-Active Oxime Esters, Styrenes, and Boronic Acids, Angew. Chem., Int. Ed., 2018, 57, 15505–15509 CrossRef CAS PubMed;
(g) B.-Q. He, X.-Y. Yu, P.-Z. Wang, J.-R. Chen and W.-J. Xiao, A Photoredox Catalyzed Iminyl Radical-Rriggered C-C Bond Cleavage/Addition/Kornblum Oxidation Cascade of Oxime Esters and Styrenes: Synthesis of Ketonitriles, Chem. Commun., 2018, 54, 12262–12265 RSC;
(h) Y.-X. Jiang, L. Chen, C.-K. Ran, L. Song, W. Zhang, L.-L. Liao and D.-G. Yu, Visible-Light Photoredox-Catalyzed Ring-Opening Carboxylation of Cyclic Oxime Esters with CO2, ChemSusChem, 2020, 13, 6312–6317 CAS;
(i) C.-K. Ran, H. Huang, X.-H. Li, W. Wang, J.-H. Ye, S.-S. Yan, B.-Q. Wang, C. Feng and D.-G. Yu, Cu-Catalyzed Selective Oxy-Cyanoalkylation of Allylamines with Cycloketone Oxime Esters and CO2, Chin. J. Chem., 2020, 38, 69–76 CrossRef CAS;
(j) L. Li, H. Chen, M. Mei and L. Zhou, Visible-Light Promoted γ-Cyanoalkyl Radical Generation: Three-Component Cyanopropylation/Etherification of Unactivated Alkenes, Chem. Commun., 2017, 53, 11544–11547 RSC.
- D. Lu, J. Cui, S. Yang and Y. Gong, Iron-Catalyzed Cyanoalkylation of Glycine Derivatives Promoted by Pyridine-Oxazoline Ligands, ACS Catal., 2021, 11, 4288–4293 CrossRef CAS.
|
This journal is © the Partner Organisations 2022 |