DOI:
10.1039/D2QO00102K
(Research Article)
Org. Chem. Front., 2022,
9, 2343-2350
Metal ion determined self-assembly using terpyridine building blocks†
Received
29th January 2022
, Accepted 7th March 2022
First published on 8th March 2022
Abstract
Due to the dynamic reversibility of the coordination-driven force, the structures of metallocages are sensitive to many stimuli, including ligand geometry, temperature, concentration, anions, pH, light, and so on. Among these, the strength of the metal–ligand dative bonds is distinctly important; however, it has largely been ignored. In this contribution, a novel metal–organic ligand L was synthesized. On coordinating with transition metals possessing distinct binding abilities like Zn(II), Co(II), and Cd(II), diverse three-dimensional metal–organic supramolecular structures could be generated. To sum up, using metal ions with a strong ligand binding ability (Co, Zn) favors the development of larger structures [M8L4], whereas metal ions with a weak ligand binding ability (Cd) lead to smaller structures [M6L3].
Introduction
Fabricating sophisticated discrete supramolecular structures, particularly three-dimensional supramolecular cages, from simple molecular building blocks has attracted extensive attention.1,2 It is not only because of their intriguing structures, but also owing to their diverse applications in molecular recognition,3 sensing,4,5 and supramolecular catalysis.6 Along with the interest in self-assembly processes, the transition of two structures has received a significant attention.7,8 Owing to the dynamic and reversible nature of the coordination-driven force, the structure of metal supramolecular self-assembly is mostly determined by two major elements: (i) the nature of the building blocks,9 such as metal ions10 and ligands,11 and (ii) extrinsic factors such as concentration,12,13 solvents,14 anions,15,16 and light,17 among others.18 Ligands and metal ions are undoubtedly the most important building blocks in terms of design. Metals usually serve as the polyhedron's vertex, with ligands constituting the polyhedral cage's edge.19,20 Nitschke reported metal ion and anion determined supramolecular transformations in 2014.21 However, in such systems, multiple factors were affected and only a limited number of metal ions were used. Either due to the shortage of the metals accessible for ligand coordination or due to the characterization difficulties, the effect of varied metal–ligand binding abilities on the self-assembly of metallostructures has rarely been described. A comprehensive understanding of the influencing factors is vital to attain precise control over the self-assembly process and achieve specific practical applications.
2,2′:6′,2′′-Terpyridines are widely used as tridentate ligands due to their ability to coordinate with various transition metals,22,23 and the binding ability with different metal ions increases in the order of Mn < Cu < Cd < Zn < Co.24 This unique characteristic supports the primacy of the terpyridyl ligand in enhancing the complexity of complexes. Inspired by this fact, it was aimed to investigate the influence of using metals with different binding capacities on the structure of metal–organic supramolecular cages. In-depth research on this aspect can help us to effectively understand the self-assembly process, as well as provide a new technique for enhancing the supramolecular diversity, thus inspiring the construction of various adaptive or stimuli-responsive materials.
We have previously described the assembly of a series of two-dimensional clover leaf-shaped supramolecules using a tetrakis-terpyridine ligand possessing the same angle as various transition metal ligands.25 Based on this study, a novel tetratopic metal–organic ligand L was synthesized using dibromobenzofuran to link with an “X” shaped tetrakis-terpyridine ligand via 〈tpy-Ru2+-tpy〉 connectivity (Scheme S1†). Subsequently, as ligand L was self-assembled with metals possessing a strong binding ability (Co, Zn), it afforded the tetrameric cages [Co8L4] and [Zn8L4], whereas a trimeric cage [Cd6L3] was obtained in the case of Cd2+ (weak binding ability with tpy) (Scheme 1). In terms of more labile Mn and Cu, simple oligomers could be derived. As a result, the generation of metallocages can be controlled by the binding ability of the metal–organic dative bonds. Furthermore, the introduction of different metal ions is expected to facilitate a study on metal-dependent redox reactions such as the oxygen evolution reaction (OER).
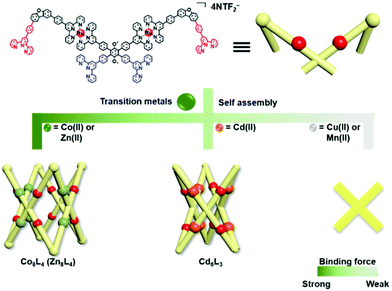 |
| Scheme 1 Self-assembly of Zn8L4, Co8L4, and Cd6L3 obtained through the coordination of L with Zn2+, Co2+, and Cd2+. | |
Results and discussion
Synthesis and characterization of Zn8L4 and Co8L4
The key ligand L was obtained by a 4-fold Suzuki coupling reaction of precursor 7 with 4-(2,2′:6′,2′′-terpyridyl)-phenylboronic acid (Fig. 1a). Subsequently, the ligand L was assembled with Zn(NTf2)2 in an exact stoichiometric ratio of 1
:
2. After heating the mixture at 60 °C for 8 h, a saturated solution of LiNTf2 in methanol was added, and the resulting red precipitate was washed multiple times with methanol and water. The final solid Zn8L4 was obtained after drying in vacuo with a yield of 98%. Similarly, self-assembly with other metals was performed using CoCl2·6H2O.
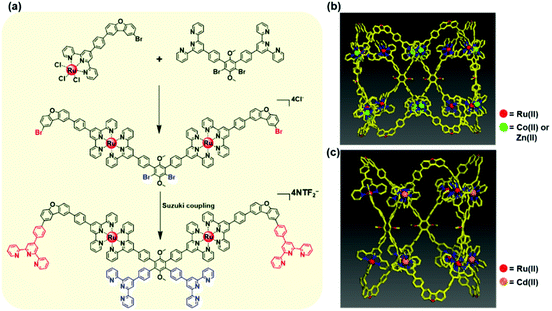 |
| Fig. 1 (a) Synthesis of ligand L, (b) representative energy-minimized structure of Co8L4 (Zn8L4), and (c) representative energy-minimized structure of Cd6L3. | |
Firstly, as observed in Fig. 2a, four sets of single peaks appear at 9.11, 9.02, 8.87, and 8.80 ppm, attributed to the four different sets of tpy-H3′5′ protons for ligand L, A–D, respectively. The peaks of 6,6′′ protons in the uncoordinated tpy units in L (tpy-C, D) exhibit a dramatic upfield shift from 8.78 and 8.76 ppm to 7.48 ppm (Fig. 2a, Δδ = 1.33 ppm), owing to the electron shielding effect. Meanwhile, as compared with the signals of the free tpy group of L, those of the protons attributed to C-tpyH3′,5′ and D-tpyH3′,5′ shifted downfield, from 8.87 and 8.80 ppm to 9.12 ppm. The whole proton assignments of ligands and Zn8L4 were confirmed by 2D COSY and NEOSY NMR (Fig. S22–S26†). Moreover, the narrow bands at log
D = −9.85 in the DOSY spectrum unambiguously reveal the presence of a single discrete species in CD3CN (Fig. 2b). The diffusion coefficient D has been calculated to be 1.41 × 10−10. Based on the Stokes–Einstein equation, the diameter of Zn8L4 is 4.22 nm, which is consistent with the model data (4.18 nm). As is well known, Co(II) exhibits stronger paramagnetic properties, which make it difficult to characterize the Co(II) complexes by 1H NMR; thus, a satisfactory result has not been obtained.26,27
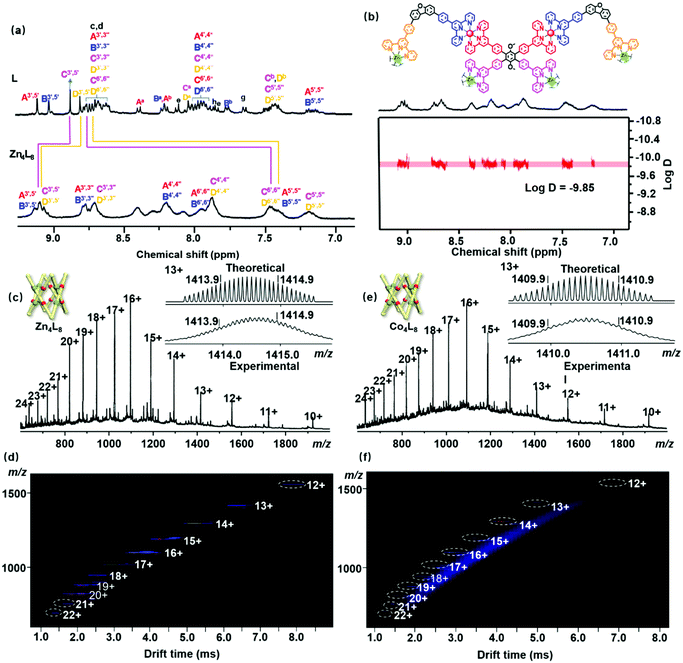 |
| Fig. 2 (a) 1H NMR of ligand L and Zn8L4 (500 MHz, CD3CN, 300 K), (b) 2D DOSY of Zn8L4 (500 MHz, CD3CN, 300 K), (c) and (d) ESI-MS of and TWIM-MS plots of Zn8L4, and (e) and (f) ESI-MS of and TWIM-MS plots of Co8L4. | |
Furthermore, the ESI-MS experiments supported the formation of supramolecular structures. Fig. 2c depicts a series of continuous charge peaks from 10+ to 24+, which is attributed to the continuous loss of the anion. It is observed to be in perfect agreement with the values obtained from the simulations, and the calculated molecular weight of 22
029 Da corresponds to the molecular mass of the tetramer [(C200H130N24O4)4Ru8Zn8(NTf2−)32]. The experimentally determined isotope patterns for each charge state match well with the corresponding theoretical distribution (Fig. S7†). Subsequently, traveling wave ion mobility mass (TWIM)28 spectrometry experiments were employed to distinguish the presence of isomers and provide structural information. A narrow series of drift time spectra from 12+ to 22+ can be observed in Fig. 2d, and the ESI-MS data indicated no other isomers and conformers. Moreover, the molecular weights of Co8L4 are confirmed to correspond to their proposed molecular compositions (Fig. 2e), and the complexes with Co(II) have comparable drift times in the same charge states (Fig. 2f), thus indicating similar shapes of the complexes.
Synthesis and characterization of Cd6L3
The previous investigations have shown that the different transition metals exhibit varying binding abilities to terpyridine. Hence, it is assumed that a distinct structure will be generated on carrying out the assembly with Cd. As illustrated in Fig. 3a, the NMR spectrum of Cd6L3 is entirely different from the former; however, the spectrum of the obtained supramolecular structure exhibits a broad peak in the aromatic region compared to that of the ligand L. It may be caused by the slow motion of the large structure on the NMR timescale. The signals assigned to the 6,6′′-tpy protons of the free tpy units are noted to exhibit a distinct upfield shift from 8.78 and 8.80 ppm to 7.50 ppm (Δδ = 1.28 ppm) after coordination with Cd(II). Similar to the DOSY spectrum of Zn8L4, the formation of a single component in CD3CN can be confirmed from the diffusion coefficient log
D = −9.70 m2 s−1 for all relevant peaks (Fig. 3b). In addition, the ESI-MS analysis reveals a new set of dominant peaks ranging from 8+ to 18+ ions, thus confirming the composition of the generated structure consisting of six Cd2+ ions and three ligands L (Fig. 3c). The isotopic pattern of each charge state is consistent with the corresponding simulated values (Fig. S8†). In addition, in the 2D TWIM-MS plot, all charge states are found in a narrow band, indicating a single component with high conformational rigidity (Fig. 3d). Furthermore, as the assembly is performed using Cu and Mn, only simple oligomeric structures are obtained, most likely due to the weak binding ability to the tpy units.29
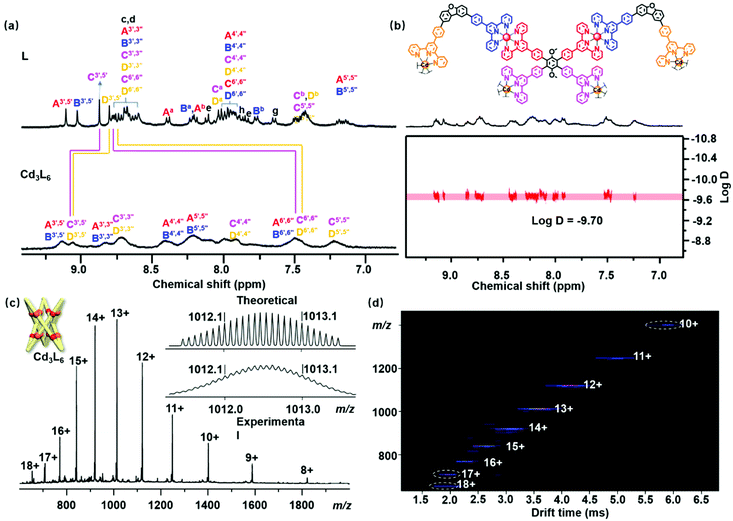 |
| Fig. 3 (a) 1H NMR of ligand L and Cd6L3 (500 MHz, CD3CN, 300 K), (b) 2D DOSY of Zn8L4 (500 MHz, CD3CN, 300 K), and (c) and (d) ESI-MS of and TWIM-MS plots of Cd6L3. | |
To further investigate the strength of the binding ability of the three metals, the stability of the three supramolecular structures was examined by gradually enhancing the collision energy using gradient tandem mass spectrometry.30 No obvious fragments of 13+ ion for Co8L4 can be detected below 20 V. As the voltage is increased to 28 V, the fragmentation peak starts to appear, and the ion peak is completely dissociated by 36 V. Zn8L4 and Cd6L3 have also been measured under the same conditions, with the former completely splitting at 30 V, while the latter becoming fragmented at 23 V (Fig. S9†). These results further demonstrate that the binding capability of Cd ions is substantially lower than that of Zn and Co ions, implying that the stability of the supramolecular structures formed will be inferior.31 It is concluded that entropy plays a critical role during the process of molecular change. For metal ions with a weak binding ability, it is difficult to support the larger structures when coordinated with ligands, which tends to form small structures with more molecules in the system for achieving an entropy increase. In contrast, for metal ions with a strong binding ability, there exist sufficient binding forces to support the formation of larger structures.13,32
Size characterization by transmission electron microscopy (TEM) and atomic force microscopy (AFM)
To provide additional structural evidence, TEM and AFM analyses were performed on ultrathin carbon film-coated Cu grids or newly cleaved mica flakes by placing drops of dilute solutions of the three supramolecular structures dissolved in CH3CN at a concentration of 10−6 M. As shown in Fig. 4e–j, the TEM images reveal several homogeneous dots with a diameter of 6.5 nm in the case of Zn8L4 and Co8L4 and 4.1 nm for Cd6L3 (Fig. S32†), which are substantially similar to the sizes simulated using the molecular model (Fig. 4a–d). Similarly, the AFM images demonstrated a series of dots with an average height of 4.2 and 4.4 nm (Fig. 4o–q), according to the height statistical histogram of AFM for 100 particles (Fig. 4l–n), which is consistent with the calculated heights of 4.0 and 4.1 nm for the model structures of Zn8L4 (Co8L4) and Cd6L3 structures. Due to the unavoidable tip broadening effect, the measured width of the dots in the AFM image displays large values in the 2D AFM images (Fig. S33†).33
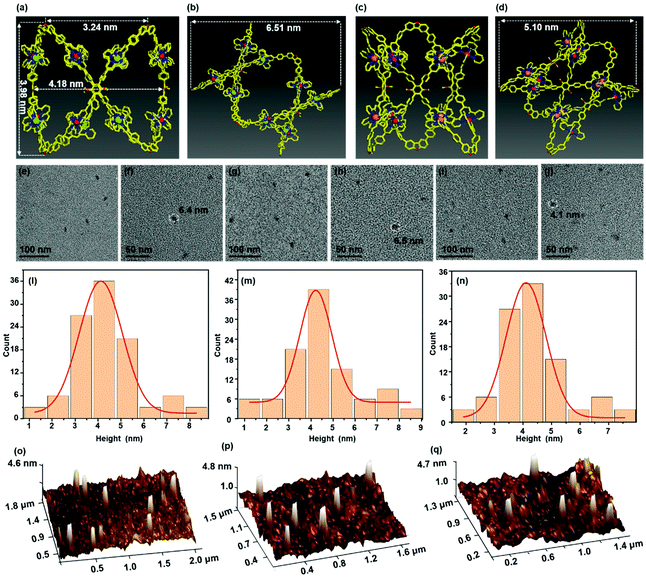 |
| Fig. 4 (a) and (b) Representative energy-minimized structure of Co8L4 (Zn8L4), (c) and (d) representative energy-minimized structure of Cd6L3, (e) and (f) TEM images of Co8L4, (g) and (h) TEM images of Zn8L4, (i) and (j) TEM images of Cd6L3, (l)–(n) AFM height statistical histogram for 100 particles of Co8L4, Zn8L4, and Cd6L3, and (o)–(q) AFM images of Co8L4, Zn8L4 and Cd6L3. | |
CV and photophysical properties of supramolecular structures
Finally, the electrochemical properties of these fractals were investigated in a three-electrode electrochemical cell, which is composed of a 3 mm glassy carbon working electrode (WE), a platinum wire auxiliary electrode (CE), and an Ag/AgCl reference electrode (RE) with Bu4NPF6 (0.1 M) as the electrolyte. As can be observed from Fig. 5c, due to the reversible oxidation of Ru(III)/Ru(II) and Ru(IV)/Ru(III), the ligand L exhibits two oxidation peaks near 0.58 and 1.31 V.34 As compared to ligand L, the oxidation peaks of the supramolecular structure demonstrate a slight positive shift. For [Zn8L4] and [Cd6L3], the oxidation peaks of Ru can be observed at 0.64 and 1.33 V, exhibiting a slight increment in the positive oxidation potential. Zn(II) and Cd(II) are difficult to oxidize under these conditions; thus, only Ru oxidation peaks can be observed for [Zn8L4] and [Cd6L3] (Fig. 5c and e).35 For [Co8L4], the oxidation peaks of Ru exhibit a similar slight positive shift (0.66 V, 1.32 V). Interestingly, the oxidation potential of Co(III)/Co(II) appears at 0.66 V in [Co8L4], while the oxidation peak of the common Co-terpyridine complex is observed at 0.45 V (Fig. 5d).24,36 The obvious positive shift indicates an effective improvement of oxidation ability which is anticipated to facilitate the oxygen evolution reactions.37 The photophysical properties of these complexes were also investigated using UV-vis and low-temperature fluorescence spectroscopy. The absorption spectra of the ligands and all the complexes demonstrate a characteristic absorption peak near 485 nm (Fig. 5a), which can be attributed to the metal–ligand charge transfer transition of the tpy-Ru-tpy unit. The emission of L and supramolecules was examined in CH3CN below 73 K (Fig. 5b). Compared with the ligand L, all supramolecules exhibit a slight decrease in the emission spectra and a minor shift, from 651 nm to 648 nm.38
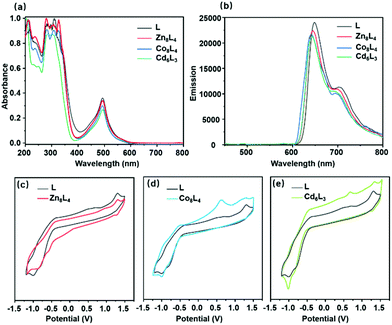 |
| Fig. 5 UV–vis (a) (10−6 M in CH3CN, room temperature) and emission (b) (10−6 M in CH3CN, 73 K) of Zn8L4, Co8L4, and Cd6L3, CV of L with (c) Zn8L4, (d) Co8L4, and (e) Cd6L3 (in a 0.1 M solution of Bu4NPF6 in CH3CN). | |
Conclusion
Briefly, the tetra-terpyridine metal–organic ligand L with multi-axial coordination was designed and synthesized using a self-assembly-directed binding strategy. Subsequently, different three-dimensional metal–organic supramolecular structures were assembled based on the difference in the transition metal-binding ability. When L was combined with strongly binding metals (Zn and Co), tetrameric structures were formed. However, on combining with the weaker binding metal Cd, a trimeric structure was obtained. 1D and 2D NMR, ESI-MS, TWIM-MS, gMS2, TEM, and AFM analyses were performed to characterize the molecular structure, size, and symmetry of the target assemblies. In addition, the photovoltaic properties of the supramolecular structures were also characterized by UV-vis, FL, and CV. The findings reported in this study are conducive to the comprehensive understanding of the effect of metal–ligand binding abilities on the supramolecular structures. As a result, these findings inspire us to obtain adaptive or stimuli-responsive materials, with potential applications in electrocatalytic oxygen precipitation, etc.
Author contributions
All authors have approved the final version of the manuscript. P. W. and Z. Z. designed the experiments; Q. B. completed the synthesis; T. W. and Y. L. carried out the NMR analysis; G. C. and Y. G. did the TEM tests; Q. B. and Z. Z. did the ESI-MS test and data curation; Y. L. and Q. B. analyzed the experiment data. M. W. and H. S. did the AFM tests; Q. B. wrote the manuscript. Z. Z., T. X., and P. W. edited the manuscript. All the authors discussed the results, and commented on and proofread the manuscript.
Conflicts of interest
There are no conflicts to declare.
Acknowledgements
This research was supported by the National Natural Science Foundation of China (21971257 to P. W., 22101061 to Z. Z., 21971048 for T.-Z. X.), the Guangdong Natural Science Foundation (2019A1515011358 to Z. Z.), the Science and Technology Research Project of Guangzhou (202002030257 to Z. Z.) and the Open Fund of Guangdong Provincial Key Laboratory of Functional Supramolecular Coordination Materials and Applications (2020A07 to Z. Z.). The authors thank for the TEM test and the assistance during data collection from the Modern Analysis and Testing Center of Guangzhou University. The authors would like to express their gratitude to EditSprings (https://www.editsprings.cn) for the expert linguistic services provided.
Notes and references
-
(a) G. R. Newkome and C. N. Moorefield, From 1 → 3 dendritic designs to fractal supramacromolecular constructs: understanding the pathway to the Sierpiński gasket, Chem. Soc. Rev., 2015, 44, 3954–3967 RSC;
(b) Y. Sun, C. Chen, J. Liu and P. J. Stang, Recent developments in the construction and applications of platinum-based metallacycles and metallacages via coordination, Chem. Soc. Rev., 2020, 49, 3889–3919 RSC.
-
(a) Y. Jin, Y. Hu and W. Zhang, Tessellated multiporous two-dimensional covalent organic frameworks, Nat. Rev. Chem., 2017, 1, 0056–0066 CrossRef;
(b) G.-Y. Wu, X. Shi, H. Phan, H. Qu, Y.-X. Hu, G.-Q. Yin, X.-L. Zhao, X. Li, L. Xu, Q. Yu and H.-B. Yang, Efficient self-assembly of heterometallic triangular necklace with strong antibacterial activity, Nat. Commun., 2020, 11, 3178–3188 CrossRef CAS PubMed.
- K. Ariga, H. Ito, J. P. Hill and H. Tsukube, Molecular recognition: from solution science to nano/materials technology, Chem. Soc. Rev., 2012, 41, 5800–5835 RSC.
- L.-J. Chen, Y.-Y. Ren, N.-W. Wu, B. Sun, J.-Q. Ma, L. Zhang, H. Tan, M. Liu, X. Li and H.-B. Yang, Hierarchical self-assembly of discrete organoplatinum(II) metallacycles with polysaccharide via electrostatic interactions and their application for heparin detection, J. Am. Chem. Soc., 2015, 137, 11725–11735 CrossRef CAS PubMed.
- M. H. Lee, J. S. Kim and J. L. Sessler, Small molecule-based ratiometric fluorescence probes for cations, anions, and biomolecules, Chem. Soc. Rev., 2015, 44, 4185–4191 RSC.
-
(a) J. S. Mugridge, A. Zahl, R. van Eldik, R. G. Bergman and K. N. Raymond, Solvent and pressure effects on the motions of encapsulated guests: tuning the flexibility of a supramolecular host, J. Am. Chem. Soc., 2013, 135, 4299–4306 CrossRef CAS PubMed;
(b) M. Schulze, V. Kunz, P. D. Frischmann and F. Würthner, A supramolecular ruthenium macrocycle with high catalytic activity for water oxidation that mechanistically mimics photosystem II, Nat. Chem., 2016, 8, 576–583 CrossRef CAS PubMed.
- W. Wang, Y.-X. Wang and H.-B. Yang, Supramolecular transformations within discrete coordination-driven supramolecular architectures, Chem. Soc. Rev., 2016, 45, 2656–2693 RSC.
- A. J. McConnell, C. S. Wood, P. P. Neelakandan and J. R. Nitschke, Stimuli-responsive metal-ligand assemblies, Chem. Rev., 2015, 115, 7729–7793 CrossRef CAS PubMed.
- A. M. Lifschitz, M. S. Rosen, C. M. McGuirk and C. A. Mirkin, Allosteric supramolecular coordination constructs, J. Am. Chem. Soc., 2015, 137, 7252–7261 CrossRef CAS PubMed.
- I. A. Riddell, Y. R. Hristova, J. K. Clegg, C. S. Wood, B. Breiner and J. R. Nitschke, Five discrete multinuclear metal-organic assemblies from one ligand: deciphering the effects of different templates, J. Am. Chem. Soc., 2013, 135, 2723–2733 CrossRef CAS PubMed.
- S. M. Jansze, G. Cecot, M. D. Wise, K. O. Zhurov, T. K. Ronson, A. M. Castilla, A. Finelli, P. Pattison, E. Solari, R. Scopelliti, G. E. Zelinskii, A. V. Vologzhanina, Y. Z. Voloshin, J. R. Nitschke and K. Severin, Ligand aspect ratio as a decisive factor for the self-assembly of coordination cages, J. Am. Chem. Soc., 2016, 138, 2046–2054 CrossRef CAS PubMed.
-
(a) X. Lu, X. Li, K. Guo, T.-Z. Xie, C. N. Moorefield, C. Wesdemiotis and G. R. Newkome, Probing a hidden world of molecular self-assembly: concentration-dependent, three-dimensional supramolecular interconversions, J. Am. Chem. Soc., 2014, 136, 18149–18155 CrossRef CAS PubMed;
(b) T. Wu, Z. Jiang, X. Xue, S.-C. Wang, M. Chen, J. Wang, H. Liu, J. Yan, Y.-T. Chan and P. Wang, Molecular hexagram and octagram: Position determined 3D metallo-supermolecules and concentration-induced transformation, Chin. Chem. Lett., 2021, 32, 1911–1914 CrossRef CAS.
- T.-Z. Xie, K. Guo, Z. Guo, W.-Y. Gao, L. Wojtas, G.-H. Ning, M. Huang, X. Lu, J.-Y. Li, S.-Y. Liao, Y.-S. Chen, C. N. Moorefield, M. J. Saunders, S. Z. D. Cheng, C. Wesdemiotis and G. R. Newkome, Precise molecular fission and fusion: quantitative self-Assembly and chemistry of a metallo-cuboctahedron, Angew. Chem., Int. Ed., 2015, 54, 9224–9229 CrossRef CAS PubMed.
-
(a) O. Gidron, M. Jirásek, N. Trapp, M.-O. Ebert, X. Zhang and F. Diederich, Homochiral [2]catenane and bis[2]catenane from alleno-acetylenic helicates - a highly selective narcissistic self-sorting process, J. Am. Chem. Soc., 2015, 137, 12502–12505 CrossRef CAS PubMed;
(b) B. Kilbas, S. Mirtschin, R. Scopelliti and K. Severin, A solvent-responsive coordination cage, Chem. Sci., 2012, 3, 701–704 RSC.
- B. Li, W. Zhang, S. Lu, B. Zheng, D. Zhang, A. Li, X. Li, X.-J. Yang and B. Wu, Multiple transformations among anion-based A2nL3n assemblies: bicapped trigonal antiprism A8L12, tetrahedron A4L6, and triple helicate A2L3 (A = Anion), J. Am. Chem. Soc., 2020, 142, 21160–21168 CrossRef CAS PubMed.
- K. Oji, A. Igashira-Kamiyama, N. Yoshinari and T. Konno, Formation, expansion, and interconversion of metallarings in a sulfur-bridged auICoIII coordination system, Angew. Chem., Int. Ed., 2014, 53, 1992–1996 CrossRef CAS PubMed.
- C. S. Pecinovsky, E. S. Hatakeyama and D. L. Gin, Polymerizable photochromic macrocyclic metallomesogens: design of supramolecular polymers with responsive nanopores, Adv. Mater., 2008, 20, 174–178 CrossRef CAS.
-
(a) N. Kishi, M. Akita and M. Yoshizawa, Selective host–guest interactions of a transformable coordination capsule/tube with fullerenes, Angew. Chem., Int. Ed., 2014, 53, 3604–3607 CrossRef CAS PubMed;
(b) M. D. Ward, C. A. Hunter and N. H. Williams, Coordination cages based on bis(pyrazolylpyridine) ligands: structures, dynamic behavior, guest binding, and catalysis, Acc. Chem. Res., 2018, 51, 2073–2082 CrossRef CAS PubMed.
- S. J. Lee and W. Lin, Chiral metallocycles: rational synthesis and novel applications, Acc. Chem. Res., 2008, 41, 521–537 CrossRef CAS PubMed.
- F. Würthner, C.-C. You and C. R. Saha-Möller, Metallosupramolecular squares: from structure to function, Chem. Soc. Rev., 2004, 33, 133–146 RSC.
- I. A. Riddell, T. K. Ronson, J. K. Clegg, C. S. Wood, R. A. Bilbeisi and J. R. Nitschke, Cation- and anion-exchanges induce multiple distinct rearrangements within metallosupramolecular architectures, J. Am. Chem. Soc., 2014, 136, 9491–9498 CrossRef CAS PubMed.
-
(a) J. Wang, H. Zhao, M. Chen, Z. Jiang, F. Wang, G. Wang, K. Li, Z. Zhang, D. Liu, Z. Jiang and P. Wang, Construction of macromolecular pinwheels using predesigned metalloligands, J. Am. Chem. Soc., 2020, 142, 21691–21701 CrossRef CAS PubMed;
(b) T. Wu, Z. Jiang, Q. Bai, Y. Li, S. Mao, H. Yu, L. Wojtas, Z. Tang, M. Chen, Z. Zhang, T.-Z. Xie, M. Wang, X. Li and P. Wang, Supramolecular triangular orthobicupola: Self-assembly of a giant Johnson solid J27, Chem, 2021, 7, 2429–2441 CrossRef CAS.
- Z. Zhang, Y. Li, B. Song, Y. Zhang, X. Jiang, M. Wang, R. Tumbleson, C. Liu, P. Wang, X.-Q. Hao, T. Rojas, A. T. Ngo, J. L. Sessler, G. R. Newkome, S. W. Hla and X. Li, Intra- and intermolecular self-assembly of a 20 nm-wide supramolecular hexagonal grid, Nat. Chem., 2020, 12, 468–474 CrossRef CAS PubMed.
- L. Wang, B. Song, S. Khalife, Y. Li, L.-J. Ming, S. Bai, Y. Xu, H. Yu, M. Wang, H. Wang and X. Li, Introducing seven transition metal ions into terpyridine-based supramolecules: self-assembly and dynamic ligand exchange study, J. Am. Chem. Soc., 2020, 142, 1811–1821 CrossRef CAS PubMed.
- Q. Bai, T. Wu, Z. Zhang, L. Xu, Z. Tang, Y. Guan, T.-Z. Xie, M. Chen, P. Su, H. Wang, P. Wang and X. Li, Clover leaf-shaped supramolecules assembled using a predesigned metallo-organic ligand, Org. Chem. Front., 2021, 8, 3244–3249 RSC.
- E. C. Constable, K. Harris, C. E. Housecroft, M. Neuburger and J. A. Zampese, Turning {M(tpy)2}n+ embraces and CH⋯π interactions on and off in homoleptic cobalt(ii) and cobalt(iii) bis(2,2′:6′,2′′-terpyridine) complexes, CrystEngComm, 2010, 12, 2949–2961 RSC.
- H. S. Chow, E. C. Constable, C. E. Housecroft, K. J. Kulicke and Y. Tao, When electron exchange is chemical exchange-assignment of 1H NMR spectra of paramagnetic cobalt(ii)-2,2′:6′,2′′-terpyridine complexes, Dalton Trans., 2005, 2, 236–237 RSC.
- S. Perera, X. Li, M. Soler, A. Schultz, C. Wesdemiotis, C. N. Moorefield and G. R. Newkome, Hexameric palladium(II) terpyridyl metallomacrocycles: assembly with 4,4′-Bipyridine and characterization by TWIM mass spectrometry, Angew. Chem., Int. Ed., 2010, 49, 6539–6544 CrossRef CAS PubMed.
- M. A. R. Meier, B. G. G. Lohmeijer and U. S. Schubert, Relative binding strength of terpyridine model complexes under matrix-assisted laser desorption/ionization mass spectrometry conditions, J. Mass Spectrom., 2003, 38, 510–516 CrossRef CAS PubMed.
- X. Li, Y.-T. Chan, G. R. Newkome and C. Wesdemiotis, Gradient tandem mass spectrometry interfaced with ion mobility separation for the characterization of supramolecular architectures, Anal. Chem., 2011, 83, 1284–1290 CrossRef CAS PubMed.
- M. Satterfield and J. S. Brodbelt, Relative binding energies of gas-phase pyridyl ligand/metal complexes by energy-variable collisionally activated dissociation in a quadrupole ion trap, Inorg. Chem., 2001, 40, 5393–5400 CrossRef CAS PubMed.
- T. Kraus, M. Buděšínský, J. Cvačka and J.-P. Sauvage, Copper(I)-directed formation of a cyclic pseudorotaxane tetramer and its trimeric homologue, Angew. Chem., Int. Ed., 2006, 45, 258–261 CrossRef CAS PubMed.
-
(a) T. Ichijo, S. Sato and M. Fujita, Size-, mass-, and density-controlled preparation of TiO2 banoparticles in a spherical cccoordination template, J. Am. Chem. Soc., 2013, 135, 6786–6789 CrossRef CAS PubMed;
(b) C. Wang, X.-Q. Hao, M. Wang, C. Guo, B. Xu, E. N. Tan, Y.-Y. Zhang, Y. Yu, Z.-Y. Li, H.-B. Yang, M.-P. Song and X. Li, Self-assembly of giant supramolecular cubes with terpyridine ligands as vertices and metals on edges, Chem. Sci., 2014, 5, 1221–1226 RSC.
-
(a) L. Duan, F. Bozoglian, S. Mandal, B. Stewart, T. Privalov, A. Llobet and L. Sun, A molecular ruthenium catalyst with water-oxidation activity comparable to that of photosystem II, Nat. Chem., 2012, 4, 418–423 CrossRef CAS PubMed;
(b) M. Schulze, V. Kunz, P. D. Frischmann and F. Würthner, A supramolecular ruthenium macrocycle with high catalytic activity for water oxidation that mechanistically mimics photosystem II, Nat. Chem., 2016, 8, 576–583 CrossRef CAS PubMed.
- Z. Zhang, H. Wang, X. Wang, Y. Li, B. Song, O. Bolarinwa, R. A. Reese, T. Zhang, X.-Q. Wang, J. Cai, B. Xu, M. Wang, C. Liu, H.-B. Yang and X. Li, Supersnowflakes: stepwise self-assembly and dynamic exchange of rhombus starshaped supramolecules, J. Am. Chem. Soc., 2017, 139, 8174–8185 CrossRef CAS PubMed.
- I. F. Mansoor, D. I. Wozniak, Y. Wu and M. C. Lipke, A delocalized cobaltoviologen with seven reversibly accessible redox states and highly tunable electrochromic behaviour, Chem. Commun., 2020, 56, 13864–13867 RSC.
- Q. Liu, Q. Wang, J. Wang, Z. Li, J. Liu, X. Sun, J. Li, Y. Lei, L. Dai and P. Wang, TpyCo2+-based coordination polymers by water-induced gelling trigged efficient oxygen evolution reaction, Adv. Funct. Mater., 2020, 30, 2000593–2000599 CrossRef CAS.
- M. Galletta, S. Campagna, M. Quesada, G. Ulrich and R. Ziessel, The elusive phosphorescence of pyrromethene–BF2 dyes revealed in new multicomponent species containing Ru(ii)–terpyridine subunits, Chem. Commun., 2005, 33, 4222–4224 RSC.
Footnotes |
† Electronic supplementary information (ESI) available. See DOI: 10.1039/d2qo00102k |
‡ These co-first authors contributed equally. |
|
This journal is © the Partner Organisations 2022 |
Click here to see how this site uses Cookies. View our privacy policy here.