DOI:
10.1039/C9AY00722A
(Paper)
Anal. Methods, 2019,
11, 3101-3107
Analysis of synthetic cannabinoid agonists and their degradation products after combustion in a smoking simulator†
Received
5th April 2019
, Accepted 7th May 2019
First published on 7th May 2019
Abstract
Synthetic cannabinoids are a major class of NPS with a high impact on analytical, forensic and toxicological fields. The main route of administration of synthetic cannabinoids is through inhalation, delivered by smoking a conventional or electronic cigarette, pyrolysis possibly altering the nature of these compounds and resulting in unknown combustion products with unknown biological activity/toxicology. In this study, a twin-trap smoking inhalation model that simulates human inhalation has been developed. The smoking simulator allows the efficient trapping of the combustion products. Analysis using UHPLC-TOF-ESI-MS and GC-MS allowed the detection of all six synthetic cannabinoids tested in the smoking simulator. A novel combustion product of MDMB-CHMICA was identified, a dihydro-1H-pyridoindole-dione with its structure and mechanism of formation proposed. This study of SC combustion products provides markers which may be useful for drug analysts and toxicologists in examining samples obtained from suspected SC users/abusers.
1. Introduction
Synthetic cannabinoids (SCs) act as agonists at the same receptors as the natural product cannabinoids, but they do so with much higher potency for both CB1 and CB2 receptor subtypes. The original SC drugs, e.g. the Hebrew University compound HU-210, Pfizer compounds CP 47,497 and CP 59,540, have been further developed into second and now third generation analogues.1 In the early 1990s, John W. Huffman from Clemson University synthesized a series of SCs with a core structure of naphthoylindole, most notably JWH-018 (Fig. 1) which, along with CP 47,497, constituted the first wave of illicitly synthesized SCs that started emerging on the streets in 2008, with the help of internet vendors that promoted these compounds as apparent research chemicals, herbal incense and so called legal highs, at least before the 2016 Psychoactive Substance Act.2–4 Later SCs, illicitly manufactured, retained the indole core, but with different functional group modifications to the head, tail and linker moieties (Fig. 1), resulting in hundreds of compounds in this second generation. Some of these were also based on published medicinal chemistry compounds, including the AM series developed by Prof. A. Makriyannis at the University of Connecticut, CT, USA.5 Continuous banning of SCs by Governments worldwide resulted in further developments, including compounds that possess the indazole core structure instead of the indole, and amino acids such as alanine and phenylalanine in the head components, replacing the naphthalene and substituted aromatic moiety. Most of these newer generation SCs are novel compounds.6
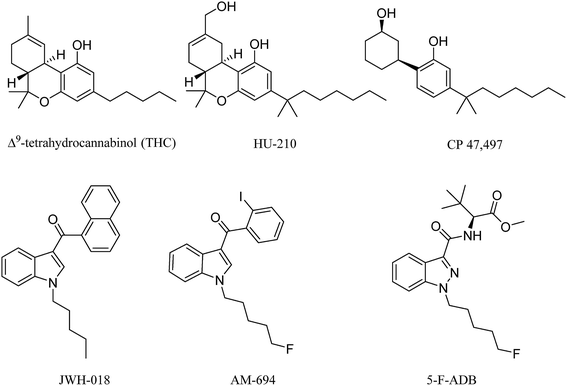 |
| Fig. 1 A natural phytocannabinoid (THC) and five SCs. | |
These SCs are still known by the street name “spice” due to the first brands promoted over the internet and sold on the street, e.g. Spice and K2. They are still sold in the form of herbal blends of potpourri, Damiana (Turnera diffusa), Marshmallow plant (Althaea officinalis) and other herbal blends soaked or sprayed with the SC. SC analysis involves the characterization and quantification, using GC-and LC-hyphenated techniques, of seized powders or often herbal blends containing SCs and also toxicological samples, the latter relying on MS techniques for analysis. Analytical methods that can be applied to crude samples are of great value to drug analysts, but due to the fact that SCs in herbal blends are mostly smoked, analytical studies of combustion products are required.7 Simulation of smoking is challenging for a number of reasons, mainly relating to the temperature and the flow rate. Temperature in cigarette smoking can routinely reach 700 °C and even goes up 900 °C at some hot spots.8 Potentially toxic pyrolysis compounds are released from herbal materials when temperatures exceed 200 °C, which is the case in cigarette smoking, e.g. toxic compounds include toluene, benzene, and naphthalene.9 The presence of several nitrogen atoms in the majority of SCs could potentially result in a number of chemically variable combustion products due to the thermal lability of C–N bonds. For an accurate simulation of the smoking process, a flow rate of 30 L min−1 needs to be maintained.10
The first reported SC pyrolysis analysis was performed on UR-144, an indole tetramethyl-cyclopropyl closely related to JWH-018 using LC-MS/MS.11 Raso and Bell12 performed GC-MS analysis, revealing pyrolysis products of first generation (JWH-018, JWH-073) and second generation (AM-694) SC using an in-house built pyrolysis device comprised of a quartz tube inserted into a round-bottom flask, where the sample is combusted using a blow torch. Common and distinctive fragments were revealed for each type of SC, e.g. naphthalene for the JWH family and cinnolineamine for the indazole family. The authors also acknowledged the problem of maintaining a stable temperature.12 Kevin et al.13 recently by-passed the issue of fluctuating temperature by conducting a temperature controlled study on six carboxamide type SC at 200, 400, 600, and 800 °C using a pyro-probe coupled to a GC-MS, thus creating temperature-controlled pyrolysis conditions where the degradants were analysed at each temperature.13
A focus on the simulation of smoking is the aim of this paper rather than pyrolysis at high temperatures coupled to anaerobic conditions. Work on the combustion and degradation products is an area that requires further investigation due to the generation of potentially harmful products with possible alteration in the target receptor or the affinity for that receptor of any new compounds. In particular, it is essential that we determine the nature of the volatile products resulting from combustion so that there is a better understanding of what ab/users of SCs are actually inhaling.14 In this study, a SC smoking model was designed and constructed. It was then employed for the analysis of different SCs and the detection of their combustion products. The resulting combustion products may help drug analysts to uncover markers for SC smoking that will facilitate toxicological drug detection and inform research into the biological effects of SC use.
2. Experimental section
Chemicals and sample preparation
All extraction solvents 99.9% anhydrous (chloroform, methanol, and acetonitrile), were purchased from Fisher Scientific (UK) and ACROS Organics (UK). The samples were in the form of herbal blends (1.0–3.0 g) as commercially packaged brands (Exodus, Loco Elite). Turnera diffusa (Damiana) dried herb (illicit-drug free) was purchased from Spiceworks (Hereford, UK).
UHPLC-TOF-ESI-MS and GC-MS
Analysis of the combustion products used UHPLC-TOF-ESI-MS and GC-MS. The UHPLC-QTOF-ESI-MS analysis was conducted using a MaXis HD Quadrupole electrospray ionization Time-of-Flight (ESI-QTOF) mass spectrometer (MS) (Bruker Daltonik GmbH, Bremen, Germany), operated in ESI positive mode. The QTOF was coupled to an Ultimate 3000 UHPLC (Thermo Fisher Scientific, CA, USA). The capillary voltage was set to 4500 V, nebulizing gas at 4 bar, drying gas at 12 L min−1 at 220 °C. The TOF scan range was from 75–1000 mass-to-charge ratio (m/z). For LC-MS/MS capabilities, the in-source CID was set to 0.0 eV, with the collision energy for TOF MS acquisition at 3.0 eV. The collision energy was set to a sliding scale from 100 m/z at 14.0 eV, 500 m/z at 20.0 eV and 1000 m/z at 30.0 eV. For the analytes, the actual collision energy was between 15.0–18.0 eV. Liquid chromatography separation was performed using an Acquity UPLC BEH C18, 1.7 μM, 2.1 × 50 mm RP-column (Waters, Milford, MA, USA) with a flow rate of 0.4 mL min−1, and an injection volume of 10 μL at 40 °C column temperature. Mobile phase A consisted of LC-MS grade water 0.1% formic acid v/v, mobile phase B consisted of acetonitrile 0.1% formic acid v/v. For Gradient 1 starting with 1% B for 2 min followed by a linear increase from 2.0 min to 100% B at 5.0 min, held for 3 min, followed by return to 1% B at 8.1 min, where it was held for equilibration for 3.9 min, total run time of 12.0 min. For gradient 2, starting with 1% B for 2 min followed by a linear increase from 2.0 min to 100% B at 10.0 min, held for 3 min followed by return to 1% B at 13.1 min, where it was held for equilibration for 2.9 min, total run time of 16.0 min. Data analysis used Bruker data analysis 4.3. Gradient 3 and mobile phases were: mobile phase A consisted of LC-MS grade water 0.1% FA v/v; mobile phase B consisted of acetonitrile 0.1% FA v/v. Solvent gradient started from 1% B for 2 min followed by a linear increase from 2.1 min to 100% B at 9.0 min, held for 3 min, followed by a return to 1% B at 12.1 min, where it was held for 2.9 min for equilibration, giving a total run time of 15.0 min.
GC-MS analysis of SC combustion was achieved on a Thermofisher ISQ series GC-MS. Full scan mode 50–650 m/z, injection volume splitless 1 μL, with He as the carrier gas, flow rate 12.0 mL min−1. HP-1 column was used (50 m × 0.32 mm, 0.17 μm thickness) Temperature gradient started at 80 °C, it was increased to 320 °C at 20°C min−1, then maintained for 3.0 min with a total run time of 15.0 min. Transfer line temperature 310 °C, ion source temperature 310 °C, scan rate of 0.2 s per scan. Data analysis used Xcalibur software.
SC smoking simulation apparatus
The smoking model is a two-stage trap rig (Fig. 2), constructed in-house using two 250 mL gas washing bottles Lenz® (VWR, UK), filled to the midpoint with 8 mm glass beads (VWR, UK) to increase the surface area. Volatile products from the combustion were collected in two dry ice/acetone traps. The gas washing bottle heads were without filters, with traps placed in a dry ice/acetone slush-bath allowing the efficient trapping of the volatiles. A quartz tube 8 mm OD × ID 6 mm was purchased from Almath crucibles (Suffolk, UK) where the SC cigarettes were inserted. The traps were connected to a GAST G626X pump (General Electric, USA), the pump dial was used to control the flow rate which was measured using a Copley® DFM 2000 flow regulator (Nottingham, UK). The flow rate was set between 28–30 L min−1 and the temperature was monitored using a TENMA thermocouple. SC-doped herb (200 mg with SC concentration between 1–6% by weight)12 was rolled into a cigarette and inserted into the quartz tube followed by lighting it with a cigarette lighter.
 |
| Fig. 2 In-house constructed SC smoking simulation model: (A) graphic, (B) cigarette inserted into the quartz tube, (C) Copley flow rate meter in position. | |
Sample collection
The condensed volatiles were extracted by washing the glass beads in each trap with methanol (3 × 25.0 mL), followed by concentration by solvent evaporation under a stream of nitrogen gas and finally the samples were reconstituted in methanol (1.0 mL) for UHPLC-TOF-ESI-MS and GC-MS analysis.
3. Results and discussion
Analysis of combustion showed the recovery of all six SCs tested by UHPLC-TOF-ESI-MS (AB-CHMINACA, AM-694, 5F-ADB, MDMB-CHMICA, MMB-2201, 5F-PB-22) (Fig. 3). Three areas of the apparatus were tested for the presence of the SC, trap 1, trap 2 and the combustion zone (CZ) in the quartz tube where the 200–300 mg cigarettes were fixed. Most of the SCs were detected in the first trap (80–90%) and interestingly no to very little SCs were detected in the CZ, proving that the tested SCs have very good volatility. This is supporting evidence for reports of prisoners and prison guards being overcome by smoke inhalation in UK prisons.15 There was neither visible evidence of the presence of American (Virginian) tobacco mixed with any of the SCs on herbal material tested, T. diffusa (Damiana), nor by HR-MS scanning the samples' MS data for any nicotine molecular ions e.g. C10H15N2 requires 163.1229 [M + H]+ and C20H29N4Cl dimer-HCl salt requires 360.2069 (Fig. S1†).
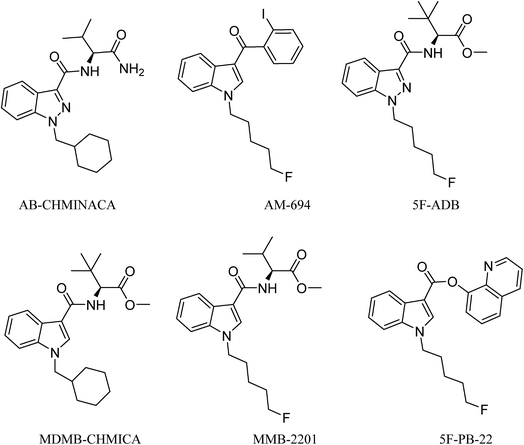 |
| Fig. 3 SCs tested by combustion analysis. | |
UHPLC-TOF-ESI-MS/MS analysis resulted in the detection of the combustion samples of AB-CHMINACA, AM-694, 5F-ADB/MMB-2201 mixture, and 5F-PB-22 (Fig. 4), with their mass ions and fragmentation pattern assignments confirmed by comparison with our in-house built database.16 The total ion current (TIC) chromatograms are shown. Each TIC chromatogram represents the summed intensity across the entire range of masses being detected at every point in the analysis. Then the HR-MS data are shown followed by the extracted-ion chromatograms (EIC) of each analyte m/z value that is being sought. That the chromatographic peaks are homogenous, nothing is co-eluting, is seen from the clean HR-MS data. GC-MS analysis resulted in the detection of the parent SC in trap 1 as well as some combustion products from some of the SCs tested. 5F-PB-22 GC-MS combustion analysis resulted in the detection of 5F-PB-22 at RT = 8.20 min as well as the combustion products 1-(5-fluoropentyl)-indole (205 m/z) and 1-pentyl-indole (185 m/z) at RT = 5.71 min and RT = 6.15 min respectively (Fig. S2†).
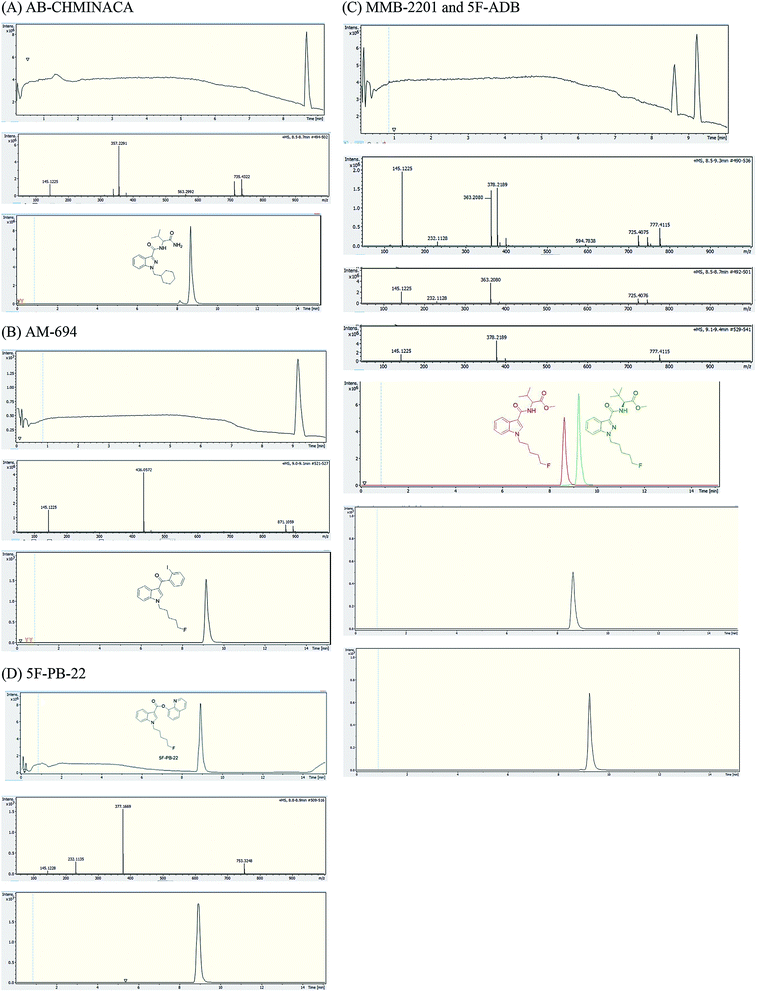 |
| Fig. 4 Each TIC chromatogram is shown above the respective HR-MS data followed by the EIC of each specific analyte m/z value. Typical UHPLC-TOF-ESI-MS/MS analysis of trap 1 for: (A) AB-CHMINACA RT = 8.7 min, (B) AM-694 RT = 9.1 min, (C) MMB-2201 RT = 8.6 min, 5F-ADB RT = 9.2 min, (D) 5F-PB-22 RT = 8.9 min. | |
MDMB-CHMICA EI MS afforded a more extensive and slightly different fragmentation than the ESI-MS/MS. The first fragment resulted from the cleavage of the tert-butyl side chain (328) rather than the initial loss of methoxy observed in the ESI-MS/MS. In addition to the 240 base peak, also seen in the MS/MS analysis, 245 is concluded to be the result of the loss of the tert-butyl side-chain along with the cleavage of the cyclohexyl moiety with the formation of 1-methylidene-indolinium ion (Fig. S3†). These results are in agreement with those of Langer et al.17
Analysis of MDMB-CHMICA combustion showed that there had been the formation of a not previously reported combustion product. Two peaks appear in the combustion sample TIC chromatogram (Fig. 5), MDMB-CHMICA RT = 11.74 min, and the novel combustion product, a keto-δ-lactam (a dihydro-1H-pyridoindole-dione) RT = 10.80 min, which was absent in the crude sample of MDMB-CHMICA that had not been subjected to combustion. The proposed mechanism for the formation of the keto-δ-lactam is rearrangement involving intramolecular electrophilic substitution of indole by the terminal methyl ester of MDMB-CHMICA during the combustion process (Fig. S4†). Unlike in the MS fragmentation pattern of MDMB-CHMICA, the 296 m/z peak is more intense and is the result of the formation of an indole-1-cyclohexylmethyl-N-propylidene ion, while the rest of the fragments are similar to MDMB-CHMICA with a base-peak fragment of 240 m/z. UHPLC separation of the two products and their HR-MS molecular ion identification using UHPLC-TOF-ESI-MS showed a low intensity peak and [M + H]+ for the keto-δ-lactam at RT = 10.7 min, 353.2229 found for C22H29N2O2 requires 353.2223, and MDMB-CHMICA RT = 9.7 min, [M + H]+ 385.2493 found for C23H33N2O3 requires 385.2485 (Fig. S5†).
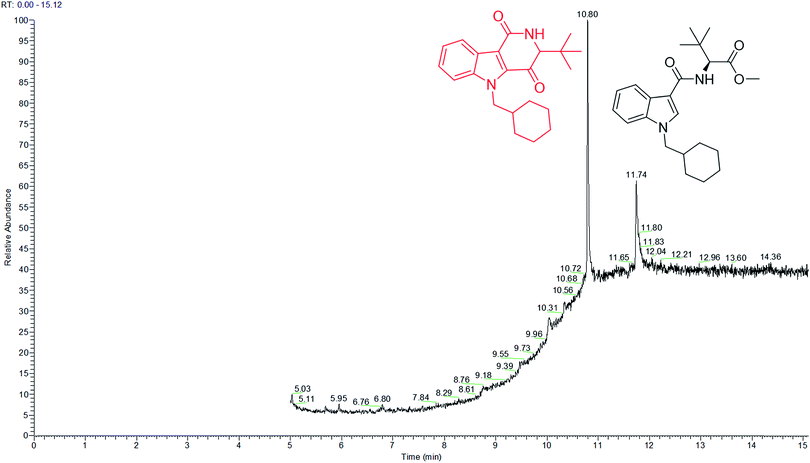 |
| Fig. 5 TIC chromatogram of a combustion sample of MDMB-CHMICA showing the novel combustion product at RT = 10.80 min and MDMB-CHMICA at RT = 11.74 min. | |
4. Conclusions
The in-house constructed SC smoking simulator provided a good method for the recovery of combusted SCs. SCs showed good volatility with parent SCs being captured in the traps with little or no SC left in the combustion zone (CZ) as tested by UHPLC-MS and GC-MS. GC-MS analysis revealed combustion products for 5F-PB-22 that were not detected using the UHPLC method. Combustion of MDMB-CHMICA resulted in the formation of a novel keto-δ-lactam that could be detected by both GC-MS and UHPLC-ESI-MS analysis.
This study of combustion products of SCs provides markers which may be useful for drug analysts and toxicologists in examining samples obtained from suspected SC users. Thus, taken together, making reliable methods for the identification and quantification of substituted 3rd generation SCs.
Conflicts of interest
There are no conflicts of interest to declare.
Acknowledgements
We acknowledge the DEAT, Avon and Somerset Constabulary, for the provision of seized samples, and the Government of Kuwait (a fully funded studentship to HAN).
References
- I. Vardakou, C. Pistos and C. Spiliopoulou, Spice drugs as a new trend: Mode of action, identification and legislation, Toxicol. Lett., 2010, 197, 157–162 CrossRef CAS PubMed.
- J. W. Huffman, D. Dai, B. R. Martin and D. R. Compton, Design, synthesis, and pharmacology of cannabimimetic indoles, Bioorg. Med. Chem. Lett., 1994, 4, 563–566 CrossRef.
- V. Auwarter, S. Dresen, W. Weinmann, M. Muller, M. Putz and N. Ferreiros, ‘Spice’ and other herbal blends: Harmless incense or cannabinoid designer drugs?, J. Mass Spectrom., 2009, 44, 832–837 CrossRef PubMed.
- EMCDDA–Europol Annual Report on the implementation of Council Decision 2005/387/JHA, 2008, http://www.emcdda.europa.eu/attachements.cfm/att_132902_EN_2008_Implementation.report.pdf, accessed 05/04/19.
-
A. Makriyannis and H. Deng, Cannabimimetic indole derivatives, USP US 6900236 B1, University of Connecticut, 2005, https://patentimages.storage.googleapis.com/9d/ec/b4/ea8af239e287da/US6900236.pdf, accessed 05/04/19.
- S. D. Banister, M. Moir, J. Stuart, R. C. Kevin, K. E. Wood, M. Longworth, S. M. Wilkinson, C. Beinat, A. S. Buchanan, M. Glass, M. Connor, I. S. McGregor and M. Kassiou, Pharmacology of indole and indazole synthetic cannabinoid designer drugs AB-FUBINACA, ADB-FUBINACA, AB-PINACA, ADBPINACA, 5F-AB-PINACA, 5F-ADB-PINACA, ADBICA, and 5F-ADBICA, ACS Chem. Neurosci., 2015, 6, 1546–1559 CrossRef CAS PubMed.
- E. W. Gunderson, H. M. Haughey, N. Ait-Daoud, A. S. Joshi and C. L. Hart, A survey of synthetic cannabinoid consumption by current cannabis users, Subst. Abuse: Res. Treat., 2014, 35, 184–189 CrossRef PubMed.
- R. R. Baker, Temperature distribution inside a burning cigarette, Nature, 1974, 247, 405–406 CrossRef.
- R. R. Baker, Product formation mechanisms inside a burning cigarette, Prog. Energy Combust. Sci., 1981, 7, 135–153 CrossRef.
- C. Darquenne, Aerosol deposition in health and disease, J. Aerosol Med. Pulm. Drug Delivery, 2012, 25, 140–147 CrossRef CAS PubMed.
- P. Kavanagh, A. Grigoryev, S. Savchuk, I. Mikhura and A. Formanovsky, UR-144 in products sold via the Internet: Identification of related compounds and characterization of pyrolysis products, Drug Test. Anal., 2013, 5, 683–692 CrossRef CAS PubMed.
- S. Raso and S. Bell, Qualitative analysis and detection of the pyrolytic products of JWH-018 and 11 additional synthetic cannabinoids in the presence of common herbal smoking substrates, J. Anal. Toxicol., 2017, 41, 551–558 CrossRef CAS PubMed.
- R. C. Kevin, A. L. Kovach, T. W. Lefever, T. F. Gamage, J. L. Wiley, I. S. McGregor and B. F. Thomas, Toxic by design? Formation of thermal degradants and cyanide from carboxamide-type synthetic cannabinoids CUMYL-PICA, 5F-CUMYL-PICA, AMB-FUBINACA, MDMB-FUBINACA, NNEI, and MN-18 during exposure to high temperatures, Forensic Toxicol., 2019, 37, 17–26 CrossRef CAS PubMed.
- A. Kaizaki-Mitsumoto, K. Hataoka, M. Funada, Y. Odanaka, H. Kumamoto and S. Numazawa, Pyrolysis of UR-144, a synthetic cannabinoid, augments an affinity to human CB1 receptor and cannabimimetic effects in mice, J. Toxicol. Sci., 2017, 42, 335–341, DOI:10.2131/jts.42.335.
- C. Howgego, The Guardian, Prisoners say ‘spice’ use has tripled fuelling violence, illness and debt, 2016, https://www.theguardian.com/society/2016/jun/01/prisoners-reveal-regular-spice-use-tripled-legal-high-violence-illness-debt, accessed 05/04/19.
-
H. A. Naqi, T. J. Woodman, S. M. Husbands and I. S. Blagbrough, 19F and 1H quantitative-NMR spectroscopic analysis of fluorinated third-generation synthetic cannabinoids, Anal. Methods, 2019, 10.1039/c9ay00814d, accompanying paper in this issue.
- N. Langer, R. Lindigkeit, H.-M. Schiebel, U. Papke, L. Ernst and T. Beuerle, Identification and quantification of synthetic cannabinoids in “spice-like” herbal mixtures: update of the German situation for the spring of 2015, Forensic Toxicol., 2016, 34, 94–107 CrossRef CAS.
Footnote |
† Electronic supplementary information (ESI) available. See DOI: 10.1039/c9ay00722a |
|
This journal is © The Royal Society of Chemistry 2019 |
Click here to see how this site uses Cookies. View our privacy policy here.