DOI:
10.1039/C7CE00654C
(Highlight)
CrystEngComm, 2017,
19, 5157-5172
cis-Protected palladium(II) based binuclear complexes as tectons in crystal engineering and the imperative role of the cis-protecting agent†
Received
5th April 2017
, Accepted 14th June 2017
First published on 14th June 2017
Abstract
A survey of the Cambridge Structural Database (CSD) for crystal structures of binuclear coordination complexes, formed by the combination of suitable ligand(s) and Pd(II) centres which are protected in a cis fashion by 2,2′-bipyridine (bpy), 1,10-phenanthroline (phen), tetramethylethylenediamine (tmeda) or ethylenediamine (en), was carried out. The structures of these binuclear complexes could be classified into five different categories – “opened jaws”, “helicate”, “plateau”, “step” and “bow-shaped” based on the relative spatial orientation of the Pd(II) square planes of the cations and the conformation of the ligand(s). Investigation of the molecular packing in these complexes revealed involvement of the cis-protected Pd(II) units in the self-assembly of the cations. Cations in the complexes that contained bpy and phen moieties often assembled via π⋯π stacking and C–H⋯π interactions between the aromatic rings of the cis-protecting Pd(II) units. In contrast, in the en and tmeda complexes, molecular self-organisation in most cases occurred through noncovalent interactions between the cis-protected Pd(II) units and the ligand, counteranion or included solvent molecules. Hence, the cations can be defined as ‘tectons’, each of which contains two cis-protecting units, which play the role of ‘supramolecular synthons’ in the self-assembly of these binuclear complexes. The study of these crystal structures provides information about preferred patterns of molecular association in these complexes, which is invaluable for the crystal engineering of pre-designed self-assembled coordination complexes for desired functions or properties.
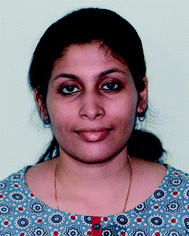 Shobhana Krishnaswamy | Shobhana Krishnaswamy received her MSc in Organic Chemistry (2005) and PhD (2011) from the University of Pune, India. Her dissertation work at the National Chemical Laboratory in Pune, under the supervision of Dr. Mohan M. Bhadbhade and Dr. Mysore S. Shashidhar, involved the synthesis, characterization and solid-state transesterification studies of crystalline ester derivatives of myo-inositol 1,3,5-orthoformate. She is currently an Institute Postdoctoral Fellow at the Indian Institute of Technology Madras. Her research interests include supramolecular chemistry, crystal engineering of functional assemblies and physical and chemical transformations in crystalline solids. |
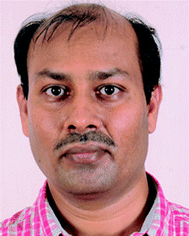 Dillip Kumar Chand | Dillip Kumar Chand received his MSc from Sambalpur University (1992) and PhD from IIT Kanpur (1998) under the supervision of Prof. P. K. Bharadwaj. Subsequently, he acquired postdoctoral experience as an Alexander von Humboldt Fellow at the Universität des Saarlandes, Germany (1999–2000, Host: Prof. H.-J. Schneider) and as a JSPS Research Fellow at the University of Tokyo, Japan (2000–2002, Host: Prof. M. Fujita). He joined the Indian Institute of Technology Madras as an Assistant Professor in 2003 and was promoted to the position of Professor in 2014. His research interests include supramolecular coordination chemistry, crystal engineering and catalysis. |
1. Introduction
Solid-state chemistry deals with the synthesis, characterisation and applications of solid materials. It includes areas of study as diverse as materials science, organic chemistry, solid-state physics, inorganic chemistry, physical chemistry, metallurgy and the chemistry of minerals.1 The solid-state structures and properties of chemical compounds are often different from those observed in the solution state, arising from the packing of the constituent molecules, atoms or ions. Advances in the field of X-ray crystallography have led to the increased availability of modern instruments and software for determining single crystal structures rapidly and accurately, resulting in an upsurge of interest in structural chemistry. Organic chemists have recognised the potential of the crystalline state in recent decades, with numerous literature accounts devoted to understanding phenomena such as polymorphism, solvatomorphism, phase transitions and solid-state reactions. These studies led to the development of the field of crystal engineering which deals with the understanding of intermolecular interactions and their subsequent use in the design and synthesis of molecular solids with desired structures and properties.2 Molecular subunits termed ‘supramolecular synthons’ can be reliably utilised to build a structure with the desired molecular organisation, an approach that parallels retrosynthesis in synthetic organic chemistry. In contrast, tectons are molecules that contain information regarding intermolecular recognition, which results in their self-assembly into crystalline networks upon combination with suitable atoms or molecules.3 For instance, the carboxylic acid dimer and amide synthons are ubiquitous in organic crystal engineering, while 4,4′-bipyridine and related linear linkers are commonly used as tectons in the construction of coordination networks and metallosupramolecular structures.4
Crystal engineering in organic compounds has been the focus of many literature reports, reviews,5 books2a,6 and even a textbook.7 However, the crystal structures of metal–organic compounds have not received as much attention, apart from coordination polymers and metal organic frameworks.8 Robson and Hoskins described the metal atom as a node and the coordinated ligand as a connector or linker in their report of the crystal structure of zinc cyanide, setting the stage for crystal engineering in coordination polymers.9 Since then, the field has grown rapidly, with the synthesised networks finding applications as NLO materials,10 materials for gas adsorption and storage11 and catalysis,12 as chemical sensors13 and as substrates for solid-state reactions.14
Transition metal ions provide a wide range of coordination geometries upon complexation, which can be exploited for preparing a variety of discrete complexes or polymeric networks. There are several literature reports describing the formation of pre-designed coordination complexes obtained by judiciously choosing ligands and metal ions.15 However, studies of noncovalent interactions involving specific molecular sub-units in the complexes that contribute to different modes of molecular organisation in the solid-state, are less common. This information can provide insight into the role of intermolecular interactions in the structures of functional coordination complexes, aiding in the design of crystalline metal–organic solids with desired molecular packing and in the modification of their solid-state properties.16 In general, it is observed that the hydrogen bonding patterns associated with common functional groups in organic crystals are also seen in the crystal structures of metal–organic compounds,17 leading to their use as supramolecular synthons in the crystal engineering of coordination complexes. Despite the availability of this information at the disposal of the solid-state chemist, crystalline forms different from those desired or envisaged are often obtained during crystallisation. They appear concomitantly with the required crystals in the form of polymorphs or solvates (formed by the mediation of solvents during crystallisation). In coordination complexes, counteranions or solvents in the crystal lattice often influence hydrogen bonding outcomes and the molecular packing, in addition to the occurrence of phenomena such as supramolecular isomerism.18
2. Background
The Cambridge Structural Database19 (CSD, version 5.36, with updates to May 2015) contains 758
844 structures out of which 323
149 are organic structures (42%) while more than 375
561 (49%) contain at least one transition metal. Of these, a total of 37
731 structures (10%) contain either Pd (19
632 structures) or Pt (18
099 structures) atoms. The Pd(II) metal centre has been exploited for the design and preparation of various self-assembled coordination compounds, particularly because of its ability to form discrete and stable square planar complexes. The added advantage of using Pd(II) is the favourable dynamic nature of the metal–ligand interaction, which is indispensable for the self-healing of wrongly formed structures, and leads to the formation of a thermodynamically stable equilibrium.15b,20
In our laboratory, we synthesised a series of pre-designed Pd(II) based self-assembled coordination compounds by combining selected nonchelating ligands with cis-protected metal centres. We determined the crystal structures of cis-protected Pd(II) based binuclear complexes containing pyridyl N-donor ligands with urea,21 piperazine22 and imidazole cores.23 The effect of changing the cis-protected Pd(II) unit on the structure and molecular packing of these self-assembled complexes prompted a survey of the CSD for the structures of similar complexes. We present some of our results here along with comparisons of the structures available in the CSD.
The complexation of cis-protected Pd(II) units (Pd(L′)) with chosen ligand(s) (L) results in discrete PdxL′xLy type coordination complexes, where the ratio and values of ‘x’ and ‘y’ depend upon the denticity of the ligand(s) and the direction of coordination vectors, respectively. The general molecular formula of the complex is represented as [Pdx(L′)x(L)y](monoanion)2x. In this formula, L′ denotes a chelating bidentate ligand, i.e., ethylenediamine (en), tetramethylethylenediamine (tmeda), 2,2′-bipyridine (bpy) or 1,10-phenanthroline (phen). The nonchelating ligand(s), L, often contains N-donor atoms (belonging to pyridine rings) and may also be a metalloligand with the metal centre in most cases being Pt(II). A discrete structure is obtained in most of the designs, whereas, a dynamic equilibrium containing two or more species is observed in some cases. For instance, mixtures of mononuclear and binuclear,24 binuclear and trinuclear,25 or trinuclear and tetranuclear complexes26 have been reported. A few examples of ligands that form coordination polymers when combined with cis-protected Pd(II) units are also reported.27 In this review, we present the analysis and comparison of related structures of complexes containing two cis-protected Pd(II) units, designated as binuclear complexes. Other metal atoms may be present in the structure (ligand moiety) if the coordinating ligand is a metalloligand.
3. Methodology
A survey of the CSD19 was undertaken for examining the crystal structures of cis-protected Pd(II) based binuclear complexes. We searched for structures containing [Pd(en)]2+, [Pd(tmeda)]2+, [Pd(bpy)]2+ and [Pd(phen)]2+ moieties, in turn, with no restrictions on the ligand(s) coordinated to the Pd(II) metal centre or counteranions. Structures which showed disorder in the main cationic unit were not considered further for analysis. The structures were sorted manually into categories based on the cis-protected Pd(II) unit and the relative orientation of the Pd–(N)2(X)2 planes, where N and X (usually N/S/O/C) represent the donor atoms of the cis-protecting unit and the chosen ligand, respectively. The different modes of packing of the cationic units in the crystal structures of these self-assembled complexes were analysed in the context of the role played by the cis-protecting agent in the molecular self-assembly. The imperative role of the cis-protecting units containing π-surfaces in steering the packing of the cations was observed and is discussed in the following sections.
4. Classification of binuclear complexes
Binuclear complexes may be formed by the combination of two units of selected cis-protected Pd(II) centres Pd(L′) with: (a) two units of a bidentate ligand LB, i.e. [Pd2(L′)2(LB)2], (b) one unit each of two different bidentate ligands LB1 and LB2, i.e. [Pd2(L′)2(LB1)(LB2)], (c) one unit of a bidentate ligand LB and two units of a monodentate ligand LM, i.e. [Pd2(L′)2(LB)(LM)2], (d) one unit of a bis-chelating tetradentate ligand LQ, i.e. [Pd2(L′)2(LQ)] or (e) one unit each of a tridentate ligand LT and a monodentate ligand LM, i.e. [Pd2(L′)2(LT)(LM)], the first category accounting for the largest number of examples. As shown in Fig. 1, the approximate shapes of various cis-[Pd2(L′)2(LB)2] type molecules, derived by displacement of one of the Pd–(N)2(X)2 planes, while keeping the other plane at a fixed position are: (a) “plateau” (no displacement); (b) “step” (axially displaced from plateau by an acute angle θ1); (c) “bow-shaped” (radially displaced from plateau by an acute angle θ2); (d) “opened jaws” (radially displaced from plateau by an obtuse angle θ2); (e) “zigzag plateau” (displacement from “plateau” in the xy-plane by an acute angle θ3) and (f), (g) left-handed and right-handed “helicate” (circularly displaced from “plateau” in either direction by θ4). A variant of the “helicate” is the “twisted” architecture, which comprises a single tetradentate ligand coordinated to two cis-protected Pd(II) centres with the two Pd–(N)2(X)2 planes oriented nearly perpendicular to each other. The “opened jaws”, “step” and “plateau” architectures are common, whereas the “bow” and “zigzag plateau” shapes are seldom observed (Fig. 1). A plot of the number of structures vs. the type of assembly shows the distribution of the various types across the four cis-protected Pd(II) units (Fig. 2). For clarity and simplicity, the formula [Pd2(L′)2(LB)2] and its corresponding schematic diagram (Fig. 1) are used here to represent the binuclear complex. The relative positions of the two Pd–(N)2(X)2 square planes in a [Pd2(L′)2(LB)2] structure depend upon the spatial positions of the coordination vectors of the nonchelating bidentate ligand component.
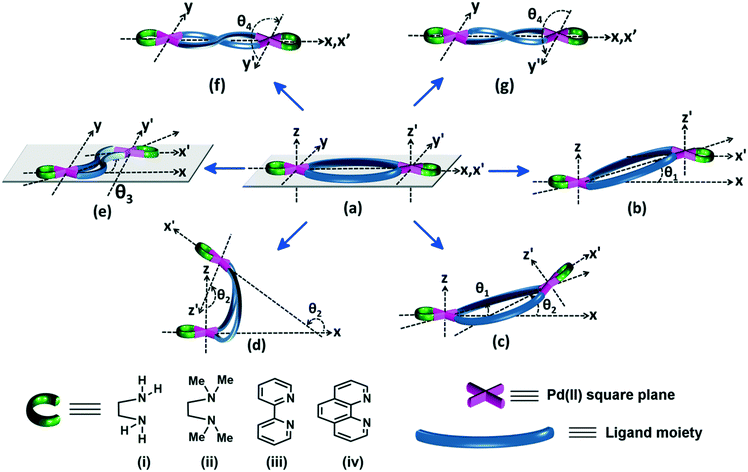 |
| Fig. 1 Schematic depiction of coordination complexes of Pd2(L′)2(LB)2 formulation showing the spatial orientation of ligands and relative positions of the two Pd–(N)2(X)2 square planes in a variety of architectures: (a) “plateau”, (b) “step”, (c) “bow-shaped”, (d) “opened jaws”, (e) “zigzag plateau”, and (f) and (g) left-handed (M, or minus) and right-handed (P, or plus) “helicates”, respectively, derived from “plateau” (adapted from ref. 23 reprinted with permission from M. C. Naranthatta, S. Bandi, R. Jagan, and D. K. Chand, Cryst. Growth Des., 2016, 16, 6722. © 2016 American Chemical Society). The “twisted” Pd2(L′)2(LQ) type complexes are depicted in Fig. 7. | |
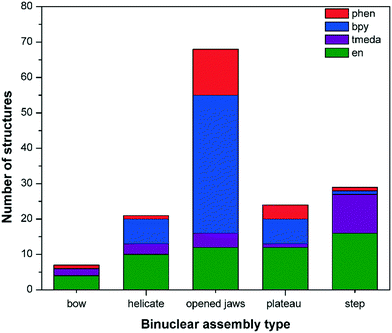 |
| Fig. 2 Plot of the number of structures (irrespective of the number and denticity of the ligand(s) coordinated to Pd(II)) vs. the binuclear assembly type shows distribution of the various types of complexes across the four cis-protected Pd(II) units. “Zigzag plateau” and “twisted” types are placed in the “plateau” and “helicate” categories, respectively. | |
The largest number of structures belong to the “opened jaws” category (Table S1†), followed by “step”, “plateau”, “helicate” and “bow” (Tables S2–S5†). Structures containing en and tmeda are distributed across all types, but many belong to the “opened jaws”, “plateau” or “step” category. The θ1 values (Fig. 1b) for a few structures of the “step” type lie in the range of 0–1°, particularly when the cis-protecting unit is en, blurring the line between the “plateau” and “step” types of assemblies (Tables S3 and S4†). These examples have been placed in the “plateau” category. Complexes of the “zigzag plateau” and “twisted” types are placed in the “plateau” and “helicate” categories, respectively. In a few examples of the “opened jaws” and “twisted” categories, the second Pd–(N)2(X)2 plane lies slightly displaced from the ideal positions described in Fig. 1. Incidentally, the helicate type complexes are the rarest when the “twisted” type is not counted.
Each structure has been assigned a number (see Table 1, Tables S1–S5†) and is also identified by its CSD refcode. Representative examples for each type of packing are discussed in the sections below and molecular packing diagrams for all structures are available in the ESI.†
Table 1 Summary of structure numbers for different architectures
Architecture of the binuclear complexes |
Structure number corresponding to the cis-protecting units: |
bpy |
phen |
en |
tmeda |
“opened jaws” |
1–38 |
39–51 |
52–62 |
63–66 |
“helicate”/“twisted” |
67–73 |
74 |
75–84 |
85–87 |
“plateau”/“zigzag plateau” |
88–94 |
95–98 |
99–110 |
111–112 |
“step” |
113 |
114 |
115–130 |
131–141 |
“bow” |
— |
142 |
143–145, 108 |
146–147 |
4.1. “Opened jaws”
The solid-state conformation of the molecule in many examples in the “opened jaws” category is driven by intra/inter molecular π⋯π stacking interactions and/or intramolecular metal–metal interactions.28 The intramolecular Pd⋯Pd distances29 in most of the “opened jaws” structures containing bpy and phen lie in the range of 2.7–3.7 Å (and exhibit intramolecular metal⋯metal interactions), whereas, those which contain tmeda and en often exhibit larger Pd⋯Pd distances in their structures (Table S1†). This structural variation can be attributed to the fact that structures containing bpy or phen units are also likely to exhibit intramolecular π⋯π stacking interactions30 between the aromatic rings of the bipyridine or phenanthroline units. Accordingly, the intramolecular Pd⋯Pd distances are defined as ‘short’ (2.7–3.7 Å) or ‘long’ (>4.0 Å) in these complexes (Table S1†) with there being no examples with the Pd⋯Pd distance in the range of 3.7–4.0 Å. The types of molecular packing adopted by the cations in these complexes may be broadly divided into four categories. In the case of complexes with ‘short’ intramolecular Pd⋯Pd distances, cations may associate directly via Pd⋯Pd interactions and/or π⋯π stacking interactions (bpy/phen units) and form infinitely long columns (Fig. 3b) or dimers (Fig. 4b), which may be further linked by anions or solvent molecules. With increasing Pd⋯Pd distance, a cavity is created between the “opened jaws”, which may be occupied by anions or solvent molecules (Fig. 5b). However, one or more cis-protected Pd(II) units may also be partially accommodated in the cavity, resulting in a closed packed structure (Fig. 6b). Illustrative examples of each type of packing are discussed below, along with related structures.
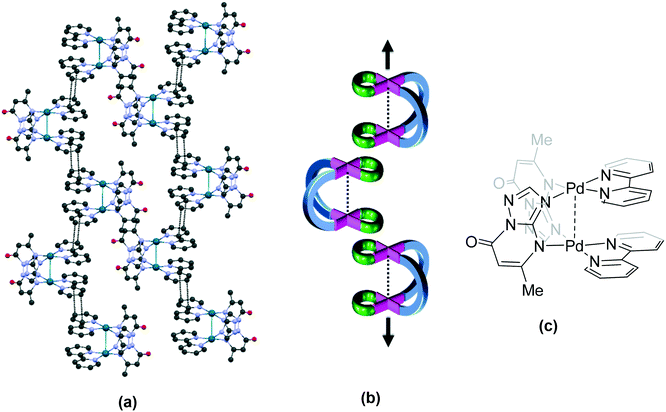 |
| Fig. 3 Association of cationic units in (a) 18 (RIZKUG)31 through π⋯π stacking interactions between bpy units forming a column-like assembly; (b) its schematic representation and (c) the chemical structure of the cationic unit in 18. Some anions, solvent and hydrogen atoms and interactions are omitted for clarity. Dotted lines between Pd atoms indicate metal⋯metal interaction. | |
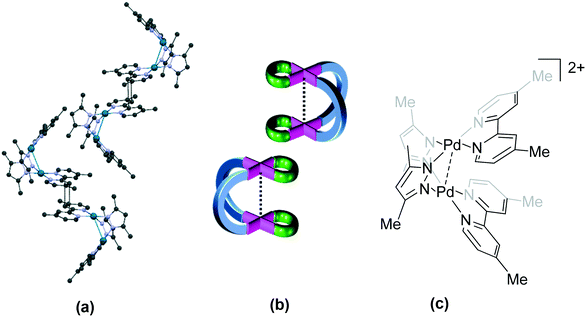 |
| Fig. 4 Dimeric association of cationic units in (a) 29 (VERRUG)51via π stacking interactions between bpy units; (b) its schematic representation and (c) the chemical structure of the cationic unit in 29. Some anions, solvent and hydrogen atoms and interactions are omitted for clarity. Dotted lines between Pd atoms indicate metal⋯metal interaction. | |
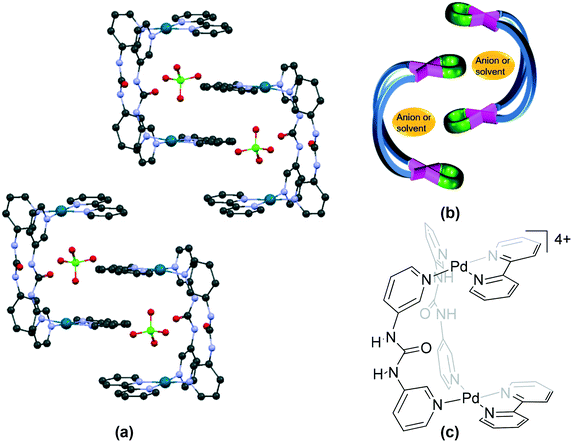 |
| Fig. 5 Self-assembly of molecules in (a) 7 (GEQSEC)21; (b) its schematic representation and (c) the chemical structure of the cationic unit in 7. Some anions, solvent and hydrogen atoms and interactions are omitted for clarity. | |
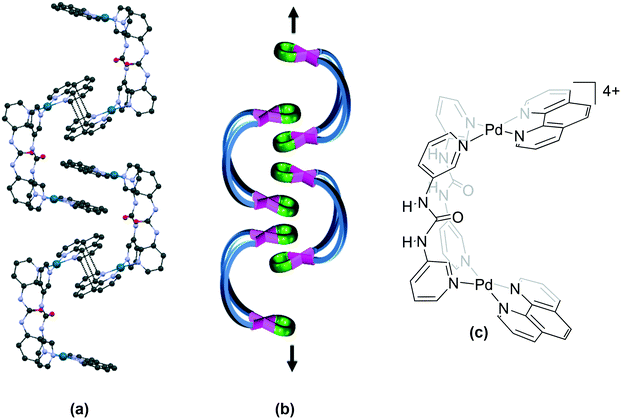 |
| Fig. 6 Association of cations in (a) 40 (GEQSUS)21; (b) its schematic representation and (c) the chemical structure of the cationic unit in 40. Some anions, solvent and hydrogen atoms and interactions are omitted for clarity. | |
4.1.1. Complexes with short intramolecular Pd⋯Pd distances (2.7–3.7 Å).
Adjacent cationic units in the crystals of 18 (RIZKUG),31 which contains a substituted pyrimidine ligand, associate via π⋯π stacking interactions between the aromatic rings of the bpy units, forming infinitely long molecular columns (Fig. 3a). This pattern of molecular packing is observed in the bpy complexes 1 (AGIGAZ),32 formed with a 2,2′-azanediyldibenzoic dianion as the ligand; 2 (BATMOY),333 (BATPIV),335 (FASQUL)34 and 6 (FASRAS),34 formed using the related ligands 1,8-naphthyridin-2,7-dione, 7-amino-1,8-naphthyridin-2-one, 1,8-naphthyridin-2-one and 7-methyl-1,8-naphthyridin-2-one, respectively; 14 (NAGSIZ)35 and 15 (NERHIB),36 containing chelating bidentate nitrate ligands; 19 (RONQUI),3720 (RONRAP)37 and 21 (RONRET)37 formed using bis-chelating tartrate as the ligand; 27 (SELSUX),38 formed using the ligand 1-methylthymine; structurally related 31 (WUHCEI)39 and 32 (WUHCOS)39 prepared with the ligands 1-methylcytosine and 2-aminopyridine, respectively; 34 (XIMZEZ)40 formed using acetamide as the ligand and the tris-osmium complex 35 (YUMWEI).41 The diacetate complex formed by Pd(OAc)2(OTf)2 with neocuproine, 44 (JIWVUH),42 and the phen complex 42 (JAMLOZ)43 also exhibit packing of the cationic units in the form of infinitely long molecular columns through π⋯π stacking interactions between the aromatic rings of the phenanthroline moieties.
The bpy complexes 22 (RONRIX)37 and 33 (XIMZAV)40 and the bis-acetato and bis-acetamido phen complexes 41 (IVUCIM),4448 (RASZIV)45 and 49 (RASZOB),45 respectively, exhibit a slightly different mode of packing, wherein the cations are alternately linked through Pd⋯Pd interactions and π stacking interactions, forming columns. In 9 (LABVAN)46 and 13 (MUBNOM)47 the cations are packed in infinitely long columns through hydrogen bonding interactions involving the atoms of the cis-protecting unit and the ligand. The bpy complexes 8 (LABTUF),4612 (MUBNIG)47 and 23 (RONROD)37 and the phen complexes 43 (JEGNEP)48 and 46 (MUBNUS)47 (with a slightly longer intramolecular Pd⋯Pd distance) represent structures where the second Pd–(N)2(X)2 is displaced from the ideal position (Fig. 1). The patterns of molecular self-assembly in 8 (LABTUF),4612 (MUBNIG)47 and 46 (MUBNUS)47 are like that observed in 18 (RIZKUG)31 (Fig. 3), i.e. formation of infinitely long molecular columns through π stacking interactions. However, in 23 (RONROD)37 and 43 (JEGNEP),48 the cations assemble in the form of parallel catemeric chains via π stacking interactions. The cations in 62 (XIMZOJ),40 the en analogue of 33 (XIMZAV),40 assemble in infinitely long columns through N–H⋯O interactions between the en unit and ligand, analogous to the molecular assembly through π⋯π stacking observed in bpy/phen complexes. The corresponding packing diagrams for these structures are available in the ESI† (Fig. S1–S30).
The tetranuclear [Pd2(L′)2(ML)2] (where ML is a metalloligand, M is Pd in this case) “opened jaws” complexes, formed by 2-aminoethanethiolate upon complexation with cis-protected Pd(II) units containing bpy (38),49 phen (50),50 dimethyl bpy (37),50 and dimethyl phen (51)50 units, also exhibit short Pd⋯Pd distances and adopt different modes of molecular packing in the solid state. In 38 (DEBKEA),49 pairs of molecules associated through π⋯π stacking interactions between the bpy units. Anion⋯π interactions in 38 (DEBKEA)49 prevent the extension of these dimers into infinitely long molecular columns, unlike the structure of its phen counterpart 50 (XIDSOS).50 The introduction of a pair of methyl substituents in the aromatic rings results in molecular assembly in the form of columns through π⋯π and C–H⋯π interactions in 37 (XIDSIM)50 and π⋯π stacking in 51 (XIDSUY).50 These modes of packing can be correlated with those observed in the case of simple binuclear complexes. The corresponding packing diagrams for these structures are available in the ESI† (Fig. S31–S34).
In some of the structures with short Pd⋯Pd distances, cationic units associate via π⋯π stacking interactions (in the case of bpy and phen) or Pd⋯Pd interactions (in the case of en and tmeda) and form dimers. These dimers may be linked by anions or solvent molecules which interrupt further propagation of the cationic assembly. For instance, in 29 (VERRUG),51 where L is dimethylpyrazole, the cations associate through π⋯π stacking interactions between the bpy units, forming dimers (Fig. 4a) which are linked by PF6 anions (not shown). The cations in the bpy complex 25 (SAZMEN),52 formed using a pyridinyl dimethylpyrazole ligand, and the phen complex 47 (MULKAF)53 assemble in this fashion, as well as those in the bpy complexes of the [Pd2(L′)2(LB)(LM)2] variety, 24 (RORFUZ)54 and 36 (YUPMAY).55
The cations in the α-pyridone complexes 52 (ACIMIJ)56 and 61 (VENHEB10)57 containing the counteranions perchlorate and nitrate, respectively, and en as the cis-protecting unit, assemble as dimers through intermolecular Pd⋯Pd interactions and supporting N–H⋯O and C–H⋯π interactions between the ligand and en units. Interestingly, all the tmeda complexes exhibit Pd⋯Pd distances greater than 4.0 Å (Table S1†). This is perhaps due to the steric aspects associated with the proximity of the methyl groups in an “opened jaws” conformation. The corresponding packing diagrams for these structures are available in the ESI† (Fig. S35–S40).
4.1.2. Complexes with long intramolecular Pd⋯Pd distances (>4.0 Å).
Complex 26 (SEHKUN),58 which contains two tert-butyl substituted Pd(bpy)2+ units coordinated to four pyridine 4-thiol ligands separated by ZnCl2 spacers and a ‘long’ Pd⋯Pd distance, reveals packing of the cationic units in the form of infinitely long helical chains through π⋯π stacking interactions between the bpy units. The phen complex 39 (EMEHIO)59 also contains Pd atoms separated by greater than 4.0 Å, wherein the cations assemble to form infinitely long chains via π⋯π stacking interactions.
This category also contains a family of complexes (bpy – 7 (GEQSEC), phen – 40 (GEQSUS), en – 53 (GEQSIG) and tmeda – 63 (GEQSOM)) formed from the combination of a urea based ligand and the four cis-protected Pd(II) units,21 reported recently from our laboratory.21 The bpy complex 7 (GEQSEC) shows a completely different type of packing due to the presence of the perchlorate anion in the cavity of the cationic unit (Fig. 5a). The perchlorate forms hydrogen bonding interactions with the urea protons and prevents the close approach of the second cationic unit. The aromatic rings of complexed ligands in adjacent cationic units interact through π stacking interactions. A similar kind of molecular packing is observed in its en and tmeda analogues, 53 (GEQSIG)21 and 63 (GEQSOM),21 though the nitrate counteranions in the former structure are not present in the cavity of the “opened jaws”. Further, the introduction of a methyl group in the aromatic ring of the urea based ligand followed by complexation with [Pd(en)(NO3)2] results in the complex 54 (KAXJOK60) with molecular packing like that observed in 63.
The diphenylpyrazolato bridged bpy and phen complexes 11 (MAQFIU)61 and 45 (MAQFEQ),61 respectively, exhibit modes of self-assembly like 7, even though the intramolecular Pd⋯Pd distances in the structures are ∼3.0 Å. Tetrafluoroborate and nitrate anions, respectively, occupy the cavity created by the “opened jaws”. These complexes also exhibit intramolecular Pd⋯Pd interactions. The en complex 56 (NEPCER),25b containing a bis-pyridyl benzene ligand, can also be classified in this category, with nitrates occupying the cavity of the cationic unit. In the [Pd2(L′)2(LQ)] tmeda complex 66 (SUHFUX),62 the anions do not occupy the cavity of the displaced “opened jaws”. However, the packing of the cations is like that observed in 7.21 The corresponding packing diagrams for these structures are available in the ESI† (Fig. S41–S49).
A fourth type of molecular packing in “opened jaws” complexes is seen in the crystal structure of 40 (GEQSUS).21 The cationic units in the phen complex associate through π stacking interactions between the aromatic rings of the cis-protected Pd(II) unit, resulting in two cis-protected metal centres, one each from two different molecules accommodated in the cavity of a third molecule (Fig. 6a).
Apart from the four types of molecular packing discussed here, some “opened jaws” complexes exhibit other modes of organization in the solid state. These structures cannot be readily classified into different categories and hence, their packing diagrams are placed in the ESI† (Fig. S50–S62). They include the bpy complexes 4 (EDUSIG),6310 (LAJVOH),6416 (PEYBOL),6517 (POZGEQ),6628 (SOSQUO)67 and 30 (VIDJAU);68 the en complexes 55 (LABDAU),6957 (RIZKOA),3158 (UFAWEE),7059 (UFAWII)70 and 60 (VAGRUQ)71 and the tmeda complexes 64 (IWOVAS)72 and 65 (NABQEO).73
4.2. “Helicate/twisted”
The category of “helicate/twisted” structures comprises binuclear complexes where the second Pd–(N)2(X)2 plane is twisted in a circular fashion about the Pd⋯Pd axis through angle θ4 (Fig. 1f and g and Table S2†). In this category, some complexes are formed from two units of a bidentate ligand (“helicate”, Fig. 7a) while others contain a single unit of a tetradentate ligand (“twisted” complexes, Fig. 7b).
 |
| Fig. 7 Schematic representation of (a) a “helicate” complex formed from two bidentate ligands and (b) a “twisted” complex formed from one unit of a tetradentate ligand. | |
4.2.1. “Helicate”.
The first examples of binuclear cis-protected Pd(II) helicates were recently reported from our laboratory,23 namely the tmeda complexes 85 (IZOJUE) and 86 (IZOKAL). The cationic units of the (P) and (M) configurations in 85 are arranged in enantiomeric stacks (Fig. 8a) via π⋯π interactions.
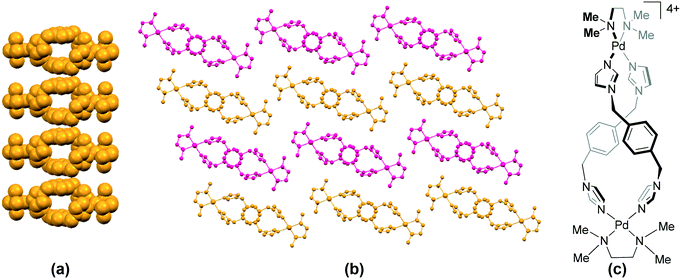 |
| Fig. 8 Association of cations in the helicate 85 (IZOJUE)23 where: (a) cations of (M) and (P) (not shown) configuration are linked by π⋯π stacking interactions between ligand moieties; (b) top view of the molecules shows alternating rows of (P) (pink) and (M) (yellow) isomers and (c) the chemical structure of the cationic unit in 85. Some anions, solvent and hydrogen atoms and interactions are omitted for clarity. | |
However, in the structurally related complex 86 (IZOKAL), which contains a biphenyl spacer in the ligand backbone, the cationic units of the (P) and (M) configurations are stacked alternately through C–H⋯π interactions between ligand units of adjacent cations. Further, 111 (IZOKEP) containing a terphenyl spacer in the ligand exhibits a “zigzag step” structure. The cis-protecting agent in these structures is tmeda, which does not contain a π-surface. In the bimetallic helicates 67 (ETASEY),7468 (ETASIC)74 and 69 (ETASOI)74 which contain metalloligands (ML, where M is Pt), the cations assemble as chains through π⋯π stacking interactions between the bpy units (Fig. 9).
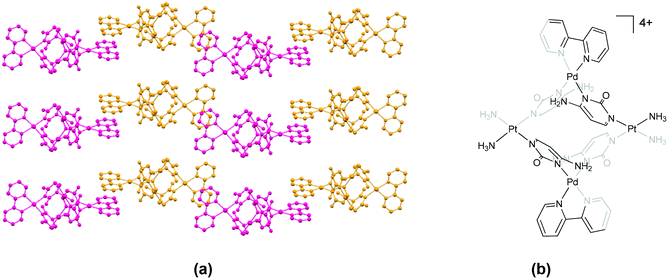 |
| Fig. 9 Association of cations in (a) 67 (ETASEY)74 through π⋯π stacking interactions between (P) (pink) and (M) (yellow) isomers and (b) the chemical structure of the cationic unit in 67. Some anions, solvent and hydrogen atoms and interactions are omitted for clarity. | |
The corresponding packing diagrams for these structures are available in the ESI† (Fig. S63–S65).
4.2.2. “Twisted” complexes.
In the case of “twisted” complexes a uniform mode of self-assembly was not observed, but rather the molecular organisation in the solid state depends upon the ligand, cis-protecting units and anions. Complexes 72 (IBUHAQ)75 and 73 (UJAGIV)76 represent “twisted” molecules of the [Pd2(L′)2(LB)(LM)2] variety, containing two pyridyl monodentate ligands and a single bidentate ligand. The cations in 7275 self-assemble through C–H⋯π interactions between the tert-butyl substituted bpy groups present in the cis-protecting units, whereas those in 7376 associate via π⋯π interactions between the aromatic rings of the dimethyl bpy units. The cations in the tmeda complex, 87 (XUPKEZ),77 (also of the [Pd2(L′)2(LB)(LM)2] variety) assemble through C–H⋯Br interactions between the protons of en and the Br atoms of the ligand in addition to Br⋯Br interactions between ligand molecules. All other examples belonging to the “twisted” complex category consist of a tetradentate ligand coordinated to cis-protected Pd(II) centres. Complex 70 (OLIXOW)78 formed from a metalloligand (ML, M = Li) and dimethyl bpy as the cis-protected Pd(II) unit, contains BPh4− as the anion. The cations assemble through a combination of weak π stacking between the aromatic rings of the dimethyl bpy units and the aromatic rings of the anions. The cations in the bisacetylacetonato complexes 71 (VENCEX)79 (Fig. 10) and 74 (KERBUF)80 assemble through π⋯π stacking interactions between the aromatic rings of their bpy and phen units, respectively.
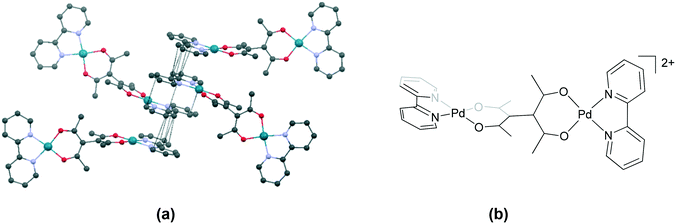 |
| Fig. 10 Association of cations as tetrads in (a) 71 (VENCEX)79 through π⋯π interactions between the aromatic rings of bpy and (b) the chemical structure of the cationic unit in 71. Some anions, solvent and hydrogen atoms and interactions are omitted for clarity. | |
The en complexes formed with the reducing sugars β-D-arabinopyranose, β-D-galactofuranose, β-rac-mannopyranose and β-D-ribopyranose as the ligands, 75 (EHUDOA),8176 (EHUDUG),8177 (EHUFAO)81 and 78 (EHUFES),81 respectively, and the complexes 79 (IFOTAY),8280 (KAKDAD),8381 (KAKDIL)83 and 82 (PIGMUO)84 featuring lyxose, glucose and xylitol based ligands are also members of the “twisted” complexes category. The cations in these complexes (except for 77) self-assemble through N–H⋯O interactions between the amine protons of en and ligand oxygen atoms to form dimers or catemers that are further linked by water molecules. In the case of 77 (EHUFAO), the molecular self-assembly is brought about by O–H⋯O hydrogen bonding interactions between the coordinated ligand molecules. In the en complexes 83 (WIJRUD)85 and 84 (WIJSAK),85 the cations self-assemble via C–H⋯π interactions between the protons of en and the aromatic rings in the ligand, in addition to weak C–H⋯π and π⋯π interactions between the ligands of adjacent cations. The corresponding packing diagrams for these structures are available in the ESI† (Fig. S66–S81).
4.3. “Plateau” and “Step”
We reported a series of binuclear complexes formed from bis(4-pyridylmethyl) piperazine and cis-protected Pd(II) units,86 of which the bpy complex (92, HITHID, Fig. 11a) is of the “plateau” variety (Fig. 1a), whereas the phen (114, HITHOJ, Fig. 12a) and tmeda (133, HITHUP) complexes can be classified in the “step” category (Fig. 1b). Similarities in the molecular packing of complexes belonging to the “plateau” and “step” varieties (Tables S3 and S4†) arise due to the flattened structure of the cationic units. The “plateau” category also includes examples of the “zigzag plateau” variety (Fig. 1e).
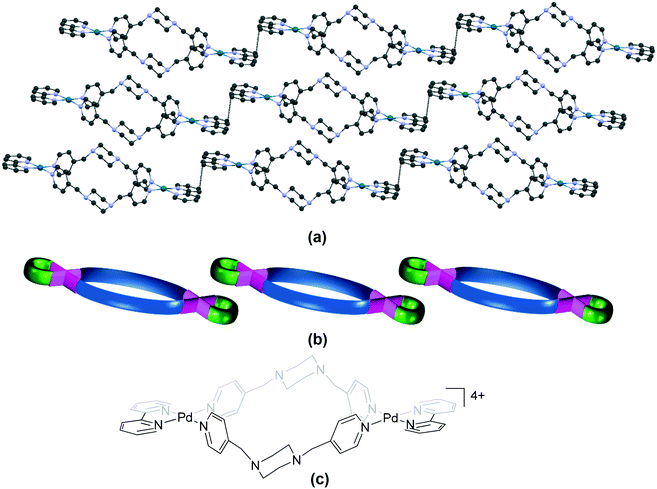 |
| Fig. 11 Association of cations in (a) 92 (HITHID)86 (“plateau”); (b) its schematic representation and (c) the chemical structure of the cationic unit in 92. Some anions, solvent and hydrogen atoms and interactions are omitted for clarity. | |
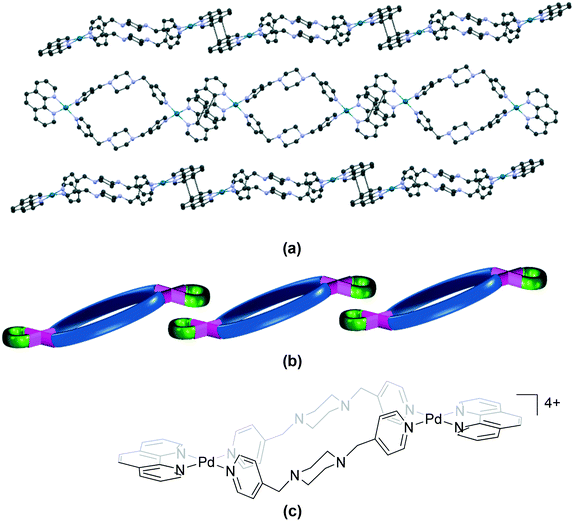 |
| Fig. 12 Association of cations in (a) 114 (HITHOJ)86 (“step”); (b) its schematic representation and (c) the chemical structure of the cationic unit in 114. Some anions, solvent and hydrogen atoms and interactions are omitted for clarity. | |
The bpy complex 113 (HUXXAA)87 also belongs to the “step” category. The cations in 92,8611486 and 11387 assemble primarily through π⋯π stacking interactions between the aromatic rings of the bpy/phen units of adjacent molecules, forming a one-dimensional π-polymer. This mode of molecular arrangement also resembles toppled dominoes.
Some bpy and phen complexes exhibit short intramolecular Pd⋯Pd distances by virtue of the ligand's structure. The cations in these complexes self-assemble through intermolecular π⋯π stacking interactions to form dimers which form the toppled dominoes through aromatic stacking interactions with adjacent dimers. The bpy complexes 90 (DEGKUW)88 and 91 (GEJJAI),89 and the phen complexes 95 (JEGMUE),9096 (WEYJUH),9197 (WEYJUH01)92 and 98 (WIVNIZ)93 exhibit this type of packing in their structures. In the bpy complex 88 (AQIXON),94 the cations associate in a dimeric fashion via aromatic stacking interactions but these dimers do not assemble as toppled dominoes due to the presence of the counteranions. There are many parallels between the modes of packing observed in the “opened jaws” complexes (section 4.1) and “plateau/step” complexes containing bpy and phen as the Pd(II) cis-protecting units.
The bimetallic bpy complex 89 (DAQSAQ),95 and structurally related 93 (KIYKIN)96 and 94 (KIYKOT)96 all contain tert-butyl substituted bpy units. The cations assemble in a toppled domino fashion in these complexes, though no aromatic stacking interactions are observed between the bpy units. In 94, C–H⋯π interactions are observed between the protons of the tert-butyl unit and aromatic rings of the cis-protecting units. The bulky tert-butyl units prevent the overlap of the aromatic rings of adjacent bpy units.
The unusual bimetallic en complex 99 (DAHBOF)97 of the “zigzag plateau” variety shows arrangement of the cations in the form of toppled dominoes through C–H⋯π interactions. Similarly, the en complexes of the [Pd2L′2(LQ)] variety, 103 (PIGMIC),98104 (PIGNOJ)98 and 105 (PIGMOI)98 all contain sugar alcohols as tetraanionic polyol ligands and exhibit assembly of the cations in the form of molecular dominoes through N–H⋯O interactions between the en units and the oxygen atoms of the ligands. The related complex 106 (PIGNAV)98 shows catemeric association of the cations through similar N–H⋯O interactions. The structures of the en complexes 125 (WAFTEC)99 and 130 (YIQFOT)100 revealed association of the cations through C–H⋯π and N–H⋯O interactions, respectively, giving rise to a layered arrangement. The tmeda complexes 131 (GAVDUE)101 and 137 (IWOVEW)72 contain aromatic rings in the ligand moiety which engage in π⋯π stacking interactions and contribute towards the layer-like assembly of the cations.
Many of the other en (100 (DOMBIR),102102 (HOVWIZ),103107 (RAKCOX),104127 (XIVGEP),105108 (XUQZUF),106109 (YIMDEE)107 and 110 (YIMDII)107) and tmeda (112, (QEGXIJ)108) complexes belonging to the “plateau” category exhibit layer-like assemblies. There are no interactions between the cationic units in these structures and hence anions, solvents and guest molecules often link the cations through hydrogen bonding contacts. Notably, the en complex 108 (XUQZUF)106 contains cations of two different conformations, namely, the “plateau” and “bow-shaped” units (discussed in the next section) in the same crystal. Unlike the related complexes 85 (IZOJUE)23 and 86 (IZOKAL),23 which belong to the “helicate” category, the tmeda complex 111 (IZOKEP)23 is of the “zigzag step” variety. Cations assemble via π⋯π stacking interactions between the phenyl rings of the ligand to form columns which are further connected by molecules of the solvent and anions.
The en and tmeda complexes of the “step” category routinely show molecular packing in the form of layers due to the flat shape of the molecule. These include the en complexes 116 (DOMBEN),102118 (ENBAPD),109119 (MAXHOI),110120 (NIHVOP),111123 (VITVEA),112126 (XAGWOT),113129 (XURBAO)106 and the tmeda complexes 132 (GAWHUJ),114134 (IXUQAU),115135 (IXUQEY),115136 (IXUQIC),115138 (IWOVIA),72139 (SUWVEM)116 and 140 (SUWVOW).116 Some of the structures exhibit zigzag or antiparallel arrangements of the layers in different directions. These include the en complex of the “plateau” variety 101 (HETQAY)117 and the en complexes of the “step” variety, 115 (COCBED),118117 (DUDPAU),119121 (RAKCUD),104122 (SUWVIQ),116124 (VITVIE)112 and 128 (XIVGIT).105 The tmeda complexes of the “step” variety, 133 (HITHUP),86137 (IWOVEW)72 and 141 (XAYGOU),120 also show zigzag arrangement of the cationic layers in their structures. These layers are interlinked with the help of anions, solvents or included guest molecules. The corresponding packing diagrams for structures belonging to the “plateau” and “step” categories are available in the ESI† (Fig. S82–S133).
4.4. “Bow-shaped”
The last category of binuclear Pd(II) complexes consists of the “bow-shaped” variety (Fig. 1c and Table S5†). A representative example is the tmeda complex 147 (WINQIV),121 wherein the bow is formed by the coordination of the ligand 1,3-bis(4-pyridylethynyl)benzene to cis-protected Pd(II) units. The aromatic rings of the ligands of adjacent molecules are involved in π⋯π stacking interactions leading to a molecular arrangement that appears like waves (Fig. 13). The μ-hydroxo Pd(neocuproine) complex 142 (JIWWAO)42 of the [Pd2(L′)2(LB)2] variety also belongs to this category. The cations assemble through π⋯π stacking interactions between the aromatic rings of the phen units, forming wavy layers which are interconnected by the anions. The en complex 143 (DAHBUL)97 contains a metalloligand wherein a Pt atom is present at the centre of the bow. Though the cations do not directly interact with each other, the pattern of assembly resembles that observed in 142.42 The cations in the en complex 145 (ODALIO)122 (which contains a glucopyranose tetrol ligand) self-assemble in a catemeric fashion through N–H⋯O interactions between the –NH protons of en and the oxygen atoms of the ligand. The cis-protected Pd(II) units in the en complex 144 (XIMZID)40 are held together by a bridging amidate ligand and a hydroxo bridge, giving rise to the “bow” shape. The cations of each wavy layer are linked by C–H⋯O and N–H⋯O interactions between the en unit and hydroxo oxygen atoms and the adjacent layers are interlinked by N–H⋯N interactions between the en units and amidate nitrogen atoms.
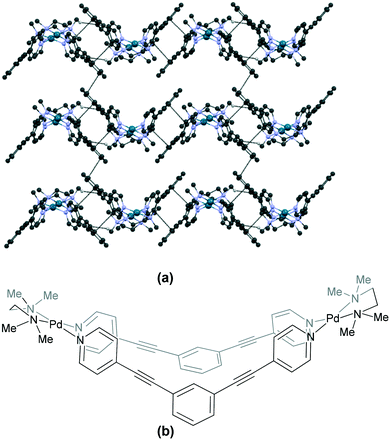 |
| Fig. 13 Association of cations in (a) 147 (WINQIV)121 through π⋯π stacking interactions between the aromatic rings of the ligands of adjacent molecules, an arrangement that appears like waves and (b) the chemical structure of the cationic unit in 147. Some hydrogen atoms and interactions are omitted for clarity. | |
The phenyl rings of the ligand moiety play a greater role than the cis-protecting unit in the molecular packing of the tmeda complexes 147 (WINQIV)121 and 146 (GAVDOY).123 Cationic units in 146 are linked through weak C–H⋯π interactions between the aromatic protons and phenyl rings of ligands of neighbouring molecules. The corresponding packing diagrams for these structures are available in the ESI† (Fig. S134–S138).
Finally, complex 108 (XUQZUF)106 has a unique structure that contains two types of cationic units, namely, “plateau” and “bow-shaped” (Fig. 14). This phenomenon is similar to the ‘conformational isomerism’ observed in organic compounds.
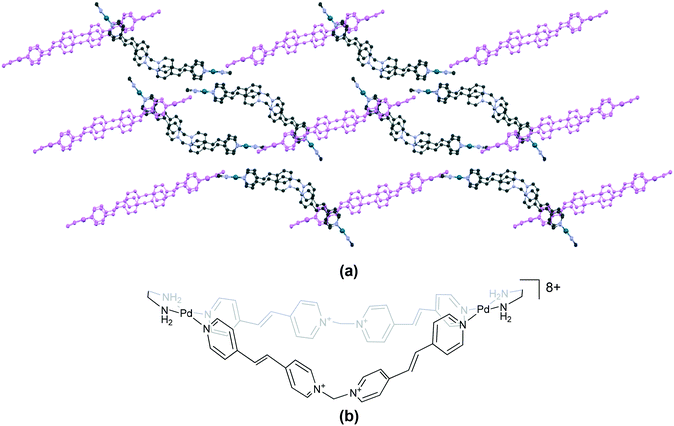 |
| Fig. 14 Assembly of two types of cations (“plateau” (violet) and “bow-shaped”) in (a) 108 (XUQZUF)106 and (b) the chemical structure of the cationic unit in 108. Some anions, solvent and hydrogen atoms and interactions are omitted for clarity. | |
5. Summary
In summary, five main categories of binuclear complexes could be identified based on the relative spatial orientation of the Pd–(N)2(X)2 planes. The “opened jaws” structure is the most commonly observed orientation followed by the closely related “step” and “plateau” structures. The combination of the same ligand with different cis-protected Pd(II) units can result in structures which belong to diverse categories. Complexes containing bpy and phen moieties often tend to self-assemble through π⋯π stacking interactions between the aromatic rings, irrespective of the type of structure. In a few cases, anions or included solvent molecules can interrupt this self-assembly by their interactions with the cis-protected Pd(II) units. The presence of methyl or tert-butyl groups on the bpy and phen aromatic rings can prevent the close approach of cationic units and hence, assembly through π stacking interactions. The “step” and “plateau” complexes often self-assemble as layers which are interconnected by interactions with anions and solvent molecules which occupy the gaps between adjacent layers. Some modes of self-assembly of the cationic units in the “opened jaws” and “plateau/step” architectures are comparable, particularly in the case of the cis-protecting units, bpy and phen. Hence, the cations can be termed ‘tectons’ which tend to self-assemble in a predictable manner through π stacking interactions between the aromatic rings of the cis-protected Pd(II) units, bpy or phen, which function as the ‘supramolecular synthons’ in these crystals. The relatively small number of crystal structures and wide variety of ligands and anions available for analysis preclude any discussion of the direct impact of the ligands' structure or specific anions on the orientation of the Pd–(N)2(X)2 planes. Many of the examples studied are complexes obtained by the combination of the ligand with a single kind of cis-protected Pd(II) unit; the structures of its complexes containing the other cis-protecting units are unknown and hence unavailable for analysis.
6. Outlook
The variation in the crystal structure and packing of organic molecules upon perturbation of the functional groups in the chemical structure has been well explored. Such analyses in the case of coordination complexes or metal–organic compounds have not been attempted, with the exception of coordination polymers or MOFs. The difficulties associated with conducting such a study are obtaining X-ray quality, stable crystals of large complexes, greater probability of disorder in the cationic and anionic units, inclusion of solvent or free ligand molecules in the crystal and structure solution of compounds containing many non-hydrogen atoms. The present study of structures of binuclear Pd(II) complexes reported in the CSD attempts to analyse the distinct modes of molecular packing observed upon varying the cis-protecting unit (bpy, phen, en, tmeda). This information is vital for the crystal engineering of pre-designed supramolecular architectures for specific functions such as anion encapsulation, guest recognition, photoluminescence and solid-state reactivity.
Acknowledgements
S. K. thanks IIT Madras for an Institute Postdoctoral Fellowship. DKC thanks the Science and Engineering Research Board (SERB), Department of Science and Technology, Government of India (Project No. SB/S1/IC-05/2014) for financial support.
References
-
A. R. West, Solid State Chemistry and its Applications, John Wiley & Sons, Chichester, 1992 Search PubMed.
-
(a)
G. R. Desiraju, Crystal Engineering: The Design of Organic Solids, Elsevier, Amsterdam, 1989 Search PubMed;
(b) G. R. Desiraju, Angew. Chem., Int. Ed., 2007, 46, 8342 CrossRef CAS PubMed;
(c) G. R. Desiraju, J. Am. Chem. Soc., 2013, 135, 9952 CrossRef CAS PubMed.
-
P. Metrangolo and G. Resnati, in Encyclopedia of Supramolecular Chemistry, ed. J. L. Atwood and J. W. Steed, Marcel Dekker Inc., 2004, p. 1484 Search PubMed.
-
(a) H. W. Roesky and M. Andruh, Coord. Chem. Rev., 2003, 236, 91 CrossRef CAS;
(b) A. Nedelcu, Z. Zak, A. M. Madalan, J. Pinkas and M. Andruh, Polyhedron, 2003, 22, 789 CrossRef CAS;
(c) K. Biradha, M. Sarkar and L. Rajput, Chem. Commun., 2006, 4169 RSC;
(d)
J. H. Jia, P. Hubberstey, N. R. Champness and M. Schroder, in Molecular Networks, ed. M. W. Hosseini, 2009, vol. 132, p. 135 Search PubMed.
-
(a) D. Braga, L. Brammer and N. R. Champness, CrystEngComm, 2005, 7, 1 RSC;
(b) A. Nangia, Cryst. Growth Des., 2008, 8, 1079 CrossRef CAS;
(c) C. B. Aakeroy, N. R. Champness and C. Janiak, CrystEngComm, 2010, 12, 22 RSC.
-
(a)
G. R. Desiraju, Crystal Design: Structure and Functions (Perspectives in Supramolecular Chemistry), John Wiley & sons, England, 2003 CrossRef;
(b)
Organic Crystal Engineering: Frontiers in Crystal Engineering, ed. E. R. T. Tiekink, J. J. Vittal and M. J. Zaworotko, John Wiley & Sons, Ltd., 2010 Search PubMed.
-
G. R. Desiraju, J. J. Vittal and A. Ramanan, Crystal Engineering: A Textbook, World Scientific Pub. Co. Inc., Singapore, 2011 Search PubMed.
-
(a) D. Braga, F. Grepioni and G. R. Desiraju, Chem. Rev., 1998, 98, 1375 CrossRef CAS PubMed;
(b) J. Y. Lu, Coord. Chem. Rev., 2003, 246, 327 CrossRef CAS; A. J. Blake, N. R. Champness, P. Hubberstey, W.-S. Li, M. A. Withersby and M. Schröder, Coord. Chem. Rev., 1999, 183, 117 Search PubMed;
(c) D. Braga, Acc. Chem. Res., 2000, 33, 601 CrossRef CAS PubMed;
(d) S. R. Batten, Curr. Opin. Solid State Mater. Sci., 2001, 5, 107 CrossRef CAS;
(e) L. Brammer, Chem. Soc. Rev., 2004, 33, 476 RSC;
(f) L. Carlucci, G. Ciani and D. M. Proserpio, Coord. Chem. Rev., 2003, 246, 247 CrossRef CAS;
(g) R. Robson, Dalton Trans., 2008, 5113 RSC;
(h)
S. R. Batten, S. M. Neville and D. R. Turner, Coordination Polymers: Design, Analysis and Application, Royal Society of Chemistry, Cambridge, 2008 Search PubMed.
- B. F. Hoskins and R. Robson, J. Am. Chem. Soc., 1990, 112, 1546 CrossRef CAS.
-
(a) O. R. Evans and W. B. Lin, Acc. Chem. Res., 2002, 35, 511 CrossRef CAS PubMed;
(b) C. Wang, T. Zhang and W. Lin, Chem. Rev., 2012, 112, 1084 CrossRef CAS PubMed;
(c) C. Wang, D. Liu and W. Lin, J. Am. Chem. Soc., 2013, 135, 13222 CrossRef CAS PubMed;
(d) H. Reinsch, M. A. van der Veen, B. Gil, B. Marszalek, T. Verbiest, D. de Vos and N. Stock, Chem. Mater., 2013, 25, 17 CrossRef CAS;
(e) D.-S. Liu, Y. Sui, W.-T. Chen and P. Feng, Cryst. Growth Des., 2015, 15, 4020 CrossRef CAS.
-
(a) M. Eddaoudi, J. Kim, N. Rosi, D. Vodak, J. Wachter, M. O'Keefe and O. M. Yaghi, Science, 2002, 295, 469 CrossRef CAS PubMed;
(b) N. Rosi, J. Eckert, M. Eddaoudi, D. T. Vodak, J. Kim, M. O'Keefe and O. M. Yaghi, Science, 2003, 300, 1127 CrossRef CAS PubMed;
(c) J. R. Li, R. J. Kuppler and H. C. Zhou, Chem. Soc. Rev., 2009, 38, 1477 RSC;
(d) J. Liu, P. K. Thallapally, B. P. McGrail, D. R. Brown and J. Liu, Chem. Soc. Rev., 2012, 41, 2308 RSC;
(e) H. Furukawa, K. E. Cordova, M. O'Keeffe and O. M. Yaghi, Science, 2013, 341, 1230444 CrossRef PubMed.
-
(a) B. Kesanli and W. Lin, Coord. Chem. Rev., 2003, 246, 305 CrossRef CAS and references cited therein;
(b) J. Lee, O. K. Farha, J. Roberts, K. A. Scheidt, S. T. Nguyen and J. T. Hupp, Chem. Soc. Rev., 2009, 38, 1450 RSC.
-
(a) L. E. Kreno, K. Leong, O. K. Farha, M. Allendorf, R. P. Van Duyne and J. T. Hupp, Chem. Rev., 2012, 112, 1105 CrossRef CAS PubMed;
(b) Y. Yu, J.-P. Ma, C.-W. Zhao, J. Yang, X.-M. Zhang, Q.-K. Liu and Y.-B. Dong, Inorg. Chem., 2015, 54, 11590 CrossRef CAS PubMed;
(c) X. Li, L. Yang, L. Zhao, X.-L. Wang, K.-Z. Shao and Z.-M. Su, Cryst. Growth Des., 2016, 16, 4374 CrossRef CAS;
(d) H. Zhang, J. Yang, Y.-Y. Liu, S. Song and J.-F. Ma, Cryst. Growth Des., 2016, 16, 3244 CrossRef CAS;
(e) S. L. Jackson, A. Rananaware, C. Rix, S. V. Bhosale and K. Latham, Cryst. Growth Des., 2016, 16, 3067 CrossRef CAS;
(f) B. Wang, X.-L. Lv, D. Feng, L.-H. Xie, J. Zhang, M. Li, Y. Xie, J.-R. Li and H.-C. Zhou, J. Am. Chem. Soc., 2016, 138, 6204 CrossRef CAS PubMed.
-
(a) J. J. Vittal, Coord. Chem. Rev., 2007, 251, 1781 CrossRef CAS;
(b) I. G. Georgiev and L. R. MacGillivray, Chem. Soc. Rev., 2007, 36, 1239 RSC;
(c) L. R. Macgillivray, G. S. Papaefstathiou, T. Friscic, T. D. Hamilton, D. K. Bucar, Q. Chu, D. B. Varshney and I. G. Georgiev, Acc. Chem. Res., 2008, 41, 280 CrossRef CAS PubMed;
(d) W. Yuan, T. Friščič, D. Apperley and S. L. James, Angew. Chem., Int. Ed., 2010, 49, 3916 CrossRef CAS PubMed;
(e) G. K. Kole and J. J. Vittal, Chem. Soc. Rev., 2013, 42, 1755 RSC.
-
(a) N. C. Gianneschi, M. S. Masar III and C. A. Mirkin, Acc. Chem. Res., 2005, 38, 825 CrossRef CAS PubMed;
(b) N. B. Debata, D. Tripathy and D. K. Chand, Coord. Chem. Rev., 2012, 256, 1831 CrossRef CAS;
(c) A. Schmidt, A. Casini and F. E. Kühn, Coord. Chem. Rev., 2014, 275, 19 CrossRef CAS;
(d) M. Han, D. M. Engelhard and G. H. Clever, Chem. Soc. Rev., 2014, 43, 1848 RSC;
(e) L. Chen, Q. Chen, M. Wu, F. Jiang and M. Hong, Acc. Chem. Res., 2015, 48, 201 CrossRef CAS PubMed;
(f) T. R. Cook and P. J. Stang, Chem. Rev., 2015, 115, 7001 CrossRef CAS PubMed.
-
(a) J. S. Field, L. P. Ledwaba, O. Q. Munro and D. R. McMillin, CrystEngComm, 2008, 10, 740 RSC;
(b) D. L. Reger, A. Debreczeni, B. Reinecke, V. Rassolov, M. D. Smith and R. F. Semeniuc, Inorg. Chem., 2009, 48, 8911 CrossRef CAS PubMed;
(c) D. L. Reger, A. Debreczeni and M. D. Smith, Inorg. Chem., 2011, 50, 11754 CrossRef CAS PubMed;
(d) X. Zhang, Z. Chi, Y. Zhang, S. Liu and J. Xu, J. Mater. Chem. C, 2013, 1, 3376 RSC;
(e) C. Jobbágy, M. Molnár, P. Baranyai, A. Hamza, G. Pálinkás and A. Deák, CrystEngComm, 2014, 16, 3192 RSC;
(f) N. Kitani, N. Kuwamura, T. Tsukuda, N. Yoshinari and T. Konno, Chem. Commun., 2014, 50, 13529 RSC;
(g) T. Seki, T. Ozaki, T. Okura, K. Asakura, A. Sakon, H. Uekusa and H. Ito, Chem. Sci., 2015, 6, 2187 RSC.
-
(a) D. Braga, F. Grepioni, P. Sabatino and G. R. Desiraju, Organometallics, 1994, 13, 3532 CrossRef CAS;
(b) G. R. Desiraju, J. Mol. Struct., 1996, 374, 191 CrossRef CAS;
(c) K. Biradha, D. Braga, F. Grepioni and G. R. Desiraju, Organometallics, 1996, 15, 1284 CrossRef CAS;
(d) D. Braga, F. Grepioni and G. R. Desiraju, J. Organomet. Chem., 1997, 548, 33 CrossRef CAS;
(e) D. Braga, F. Grepioni and G. R. Desiraju, Chem. Rev., 1998, 98, 1375 CrossRef CAS PubMed;
(f) D. Braga and F. Grepioni, Coord. Chem. Rev., 1999, 183, 19 CrossRef CAS;
(g) D. Braga, L. Maini, M. Polito, L. Scaccianoce, G. Cojazzi and F. Grepioni, Coord. Chem. Rev., 2001, 216–217, 225 CrossRef CAS;
(h) R. Bertani, P. Sgarbossa, A. Venzo, F. Lelj, M. Amati, G. Resnati, T. Pilati, P. Metrangolo and G. Terraneo, Coord. Chem. Rev., 2010, 254, 677 CrossRef CAS.
-
(a) B. Moulton and M. Zaworotko, Chem. Rev., 2001, 101, 1629 CrossRef CAS PubMed;
(b)
M. Schröder and N. R. Champness, in Encyclopedia of Supramolecular Chemistry, ed. J. L. Atwood and J. W. Steed, Marcel Dekker Inc., 2004, p. 1420 Search PubMed.
- C. R. Groom, I. J. Bruno, M. P. Lightfoot and S. C. Ward, Acta Crystallogr., Sect. B: Struct. Sci., Cryst. Eng. Mater., 2016, 72, 171 CrossRef CAS PubMed.
-
(a) M. Fujita, Chem. Soc. Rev., 1998, 27, 417 RSC;
(b) S. Leininger, B. Olenyuk and P. J. Stang, Chem. Rev., 2000, 100, 853 CrossRef CAS PubMed;
(c) B. J. Holliday and C. A. Mirkin, Angew. Chem., Int. Ed., 2001, 40, 2022 CrossRef CAS;
(d) M. Fujita, M. Tominaga, A. Hori and B. Therrien, Acc. Chem. Res., 2005, 38, 369 CrossRef CAS PubMed;
(e) B. H. Northrop, Y.-R. Zheng, K.-W. Chi and P. J. Stang, Acc. Chem. Res., 2009, 42, 1554 CrossRef CAS PubMed;
(f) R. Chakrabarty, P. S. Mukherjee and P. J. Stang, Chem. Rev., 2011, 111, 6810 CrossRef CAS PubMed.
- M. C. Naranthatta, D. Das, D. Tripathy, H. S. Sahoo, V. Ramkumar and D. K. Chand, Cryst. Growth Des., 2012, 12, 6012 CAS.
- D. Tripathy, V. Ramkumar and D. K. Chand, Cryst. Growth Des., 2013, 13, 3763 CAS.
- M. C. Naranthatta, S. Bandi, R. Jagan and D. K. Chand, Cryst. Growth Des., 2016, 16, 6722 CAS.
- A. Hori, H. Kataoka, A. Akasaka, T. Okano and M. Fujita, J. Polym. Sci., Part A: Polym. Chem., 2003, 41, 3478 CrossRef CAS.
-
(a) G. Ma, Y. S. Yung, D. S. Chung and J. I. Hong, Tetrahedron Lett., 1999, 40, 531 CrossRef CAS;
(b) D. K. Chand, K. Biradha, M. Kawano, S. Sakamoto, K. Yamaguchi and M. Fujita, Chem. – Asian J., 2006, 1, 82 CrossRef CAS PubMed;
(c) P. Diaz, J. A. Tovilla, P. Ballester, J. Benet-Buchholz and R. Vilar, Dalton Trans., 2007, 3516 RSC.
-
(a) M. Fujita, O. Sasaki, T. Mitsuhashi, T. Fujita, J. Yazaki, K. Yamaguchi and K. Ogura, Chem. Commun., 1996, 1535 RSC;
(b) S. B. Lee, S. Hwang, D. S. Chung, H. Yun and J.-I. Hong, Tetrahedron Lett., 1998, 39, 873 CrossRef CAS;
(c) K. Uehara, K. Kasai and N. Mizuno, Inorg. Chem., 2007, 46, 2563 CrossRef CAS PubMed.
-
(a) Z. Qin, M. C. Jennings and R. J. Puddephatt, Chem. Commun., 2002, 354 RSC;
(b) K. Uehara, H. Nakao, R. Kawamoto, S. Hikichi and N. Mizuno, Inorg. Chem., 2006, 45, 9448 CrossRef CAS PubMed;
(c) K. Uehara, K. Kasai and N. Mizuno, Inorg. Chem., 2010, 49, 2008 CrossRef CAS PubMed;
(d) S. Guha, F. S. Goodson, R. J. Clark and S. Saha, CrystEngComm, 2012, 14, 1213 RSC;
(e) Y. Dong, D. T. J. Loong, A. K. L. Yuen, R. J. Black, S. O'Malley, J. K. Clegg, L. F. Lindoy and K. A. Jolliffe, Supramol. Chem., 2012, 24, 508 CrossRef CAS;
(f) K. Uehara, T. Oishi, T. Hirose and N. Mizuno, Inorg. Chem., 2013, 52, 11200 CrossRef CAS PubMed.
-
(a) O. Piovesana, C. Bellitto, A. Flamini and P. F. Zanazzi, Inorg. Chem., 1979, 18, 2258 CrossRef CAS;
(b) H.-K. Yip, T.-F. Lai and C.-M. Che, J. Chem. Soc., Dalton Trans., 1991, 1639 RSC;
(c) B.-H. Xia, C.-M. Che and Z.-Y. Zhou, Chem. – Eur. J., 2003, 9, 3055 CrossRef CAS;
(d) S. Clement, S. M. Aly, D. Bellows, D. Fortin, C. Strohmann, L. Guyard, A. S. Abd-El-Aziz, M. Knorr and P. D. Harvey, Inorg. Chem., 2009, 9, 4118 CrossRef PubMed;
(e) J. E. Bercaw, A. C. Durrell, H. B. Gray, J. C. Green, N. Hazari, J. A. Labinger and J. R. Winkler, Inorg. Chem., 2010, 49, 1801 CrossRef CAS PubMed;
(f) N. Marino, C. H. Fazen, J. D. Blakemore, C. D. Incarvito, N. Hazari and R. P. Doyle, Inorg. Chem., 2011, 50, 2507 CrossRef CAS PubMed;
(g) J. Luo, J. R. Khusnutdinova, N. P. Rath and L. M. Mirica, Chem. Commun., 2012, 48, 1532 RSC.
-
(a) T. Murahashi and H. Kurosawa, Coord. Chem. Rev., 2002, 231, 207 CrossRef CAS;
(b) V. K. Jain and L. Jain, Coord. Chem. Rev., 2005, 249, 3075 CrossRef CAS.
-
(a) C. A. Hunter and J. K. M. Sanders, J. Am. Chem. Soc., 1990, 112, 5525 CrossRef CAS;
(b) C. A. Hunter, Chem. Soc. Rev., 1994, 23, 101 RSC;
(c) C. Janiak, J. Chem. Soc., Dalton Trans., 2000, 3885 RSC.
- J. A. R. Navarro, M. Angustias Romero and J. M. Salas, J. Chem. Soc., Dalton Trans., 1997, 1001 RSC.
- E. Gao, M. Zhu, H. Yin, L. Liu, Q. Wu and Y. Sun, J. Inorg. Biochem., 2008, 102, 1958 CrossRef CAS PubMed.
- B. Oskui and W. S. Sheldrick, Eur. J. Inorg. Chem., 1999, 1325 CrossRef CAS.
- B. Oskui, M. Mintert and W. S. Sheldrick, Inorg. Chim. Acta, 1999, 287, 72 CrossRef CAS.
- J. Gao, T. Riis-Johannesen, R. Scopelliti, X. Qian and K. Severin, Dalton Trans., 2010, 39, 7114 RSC.
- S.-Y. Yu, M. Fujita and K. Yamaguchi, J. Chem. Soc., Dalton Trans., 2001, 3415 RSC.
- K. Ohno, T. Sugaya, M. Kato, N. Matsumoto, R. Fukano, Y. Ogino, S. Kaizaki, T. Fujihara and A. Nagasawa, Cryst. Growth Des., 2014, 14, 3675 CAS.
- W. Micklitz, W. S. Sheldrick and B. Lippert, Inorg. Chem., 1990, 29, 211 CrossRef CAS.
- W.-Z. Shen, G. Trotscher-Kaus and B. Lippert, Dalton Trans., 2009, 8203 RSC.
- R. A. Adrian, S. Zhu, D. R. Powell, G. A. Broker, E. R. T. Tiekink and J. A. Walmsley, Dalton Trans., 2007, 4399 RSC.
- S. Chan and W.-T. Wong, J. Organomet. Chem., 1995, 489, C78 CrossRef CAS.
- N. R. Conley, L. A. Labios, D. M. Pearson, C. C. L. McCrory and R. M. Waymouth, Organometallics, 2007, 26, 5447 CrossRef CAS.
- M. Abul-Haj, M. Quiros and J. M. Salas, Polyhedron, 2004, 23, 2373 CrossRef CAS.
- D. M. Pearson, N. R. Conley and R. M. Waymouth, Organometallics, 2011, 30, 1445 CrossRef CAS.
- C. Borriello, R. Centore and G. Roviello, Inorg. Chem. Commun., 2005, 8, 755 CrossRef CAS.
- M. Juribasic, M. Curic, K. Molcanov, D. Matkovic-Calogovic and D. Babic, Dalton Trans., 2010, 39, 8769 RSC.
- T. Murahashi, T. Okuno, T. Nagai and H. Kurosawa, Organometallics, 2002, 21, 3679 CrossRef CAS.
- M. Yu, L.-F. Wang, H.-P. Huang, S.-Y. Yu and Y.-Z. Li, Chin. J. Inorg. Chem., 2006, 22, 941 CAS.
- Y. Yamada and K. Okamoto, Chem. Lett., 1999, 28, 315 CrossRef.
- Y. Yamada, K. Fujisawa and K. Okamoto, Bull. Chem. Soc. Jpn., 2000, 73, 2067 CrossRef CAS.
- H.-P. Huang, Q. Wu and G.-Q. Mei, Acta Crystallogr., Sect. E: Struct. Rep. Online, 2006, 62, m3465 CAS.
- J. Tong, S.-Y. Yu and H. Li, Chem. Commun., 2012, 48, 5343 RSC.
- M. A. Haj, M. Quiros and J. M. Salas, J. Chem. Soc., Dalton Trans., 2002, 4740 RSC.
- E. Alonso, J. Forniés, C. Fortuño, A. Martín, G. M. Rosair and A. J. Welch, Inorg. Chem., 1997, 36, 4426 CrossRef CAS PubMed.
- A. Klein and R. Lepski, Z. Anorg. Allg. Chem., 2009, 635, 878 CrossRef CAS.
- S. Iwatsuki, T. Itou, H. Ito, H. Mori, K. Uemura, Y. Yokomori, K. Ishihara and K. Matsumoto, Dalton Trans., 2006, 1497 RSC.
- K. Matsumoto, H. Moriyama and K. Suzuki, Inorg. Chem., 1990, 29, 2096 CrossRef CAS.
- B.-C. Tzeng, C.-S. Ding, T.-Y. Chang, C.-C. Hu and G.-H. Lee, CrystEngComm, 2012, 14, 8228 RSC.
- T. Murahashi, T. Okuno, K. Usui, K. Takase, T. Otani, S. Ogoshi and H. Kurosawa, Dalton Trans., 2011, 40, 2383 RSC.
- R. W. Troff, R. Hovorka, T. Weilandt, A. Lützen, M. Cetina, M. Nieger, D. Lentz, K. Rissanen and C. A. Schalley, Dalton Trans., 2012, 41, 8410 RSC.
- H.-P. Huang, S.-H. Lu, S.-Y. Yu, Y.-Z. Li, Q. Jiao and Y.-J. Pan, Inorg. Chem. Commun., 2005, 8, 656 CrossRef CAS.
- J. Vicente, J.-A. Abad, M. López-Sáez, P. G. Jones and D. Bautista, Chem. – Eur. J., 2010, 16, 661 CrossRef CAS PubMed.
- H.-P. Huang and L.-X. Liu, Acta Crystallogr., Sect. E: Struct. Rep. Online, 2007, 63, m1875 CAS.
- B.-C. Tzeng, W.-F. Fu, C.-M. Che, H.-Y. Chao, K.-K. Cheung and S.-M. Peng, J. Chem. Soc., Dalton Trans., 1999, 1017 RSC.
- H.-P. Huang, Q. Wu, L.-X. Liu and Q.-F. Song, Acta Crystallogr., Sect. E: Struct. Rep. Online, 2007, 63, m458 CAS.
- M. Fujita, S.-Y. Yu, T. Kusukawa, H. Funaki, K. Ogura and K. Yamaguchi, Angew. Chem., Int. Ed., 1998, 37, 2082 CrossRef CAS.
-
S. Yu, Private Communication, 2014 Search PubMed.
- H.-P. Huang, H.-W. Ma and Y.-Z. Li, Acta Crystallogr., Sect. E: Struct. Rep. Online, 2007, 63, m1089 CAS.
- S. J. Park, D. M. Shin, S. Sakamoto, K. Yamaguchi, Y. K. Chung, M. S. Lah and J.-I. Hong, Chem. Commun., 2003, 998 RSC.
- S. Ghosh, R. Chakrabarty and P. S. Mukherjee, Dalton Trans., 2008, 1850 RSC.
- R. Ahlrichs, M. Ballauff, K. Eichkorn, O. Hanemann, G. Kettenbach and P. Klufers, Chem. – Eur. J., 1998, 4, 835 CrossRef CAS.
- L.-Y. Yao, L. Qin, T.-Z. Xie, Y.-Z. Li and S.-Y. Yu, Inorg. Chem., 2011, 50, 6055 CrossRef CAS PubMed.
- Y. M. Na, T. H. Hoh, B. J. Ha, J. Hong and O.-S. Jung, Bull. Korean Chem. Soc., 2009, 30, 573 CrossRef CAS.
- A. Khutia, P. J. S. Miguel and B. Lippert, Chem. – Eur. J., 2011, 17, 4205 CrossRef CAS PubMed.
- J. Vicente, R. V. Shenoy, E. Martínez-Viviente and P. G. Jones, Inorg. Chem., 2011, 50, 7189 CrossRef CAS PubMed.
- J. Ruiz, M. Teresa Martínez, F. Florenciano, V. Rodríguez and G. López, Inorg. Chem., 2003, 42, 3650 CrossRef CAS PubMed.
- J. Vicente, R. V. Shenoy and E. Martínez-Viviente, Organometallics, 2009, 28, 6101 CrossRef CAS.
- H. Shen, I. M. Steele and R. F. Jordan, Acta Crystallogr., Sect. C: Cryst. Struct. Commun., 2003, 59, m405 Search PubMed.
- G.-Q. Mei, K.-L. Huang, H.-P. Huang and Y.-Z. Li, Acta Crystallogr., Sect. E: Struct. Rep. Online, 2006, 62, m2368 CAS.
- G.-Q. Mei, K.-L. Huang and H.-P. Huang, Acta Crystallogr., Sect. E: Struct. Rep. Online, 2006, 62, m2743 CAS.
- P. Klufers and T. Kunte, Chem. – Eur. J., 2003, 9, 2013 CrossRef CAS PubMed.
- P. Klufers and T. Kunte, Eur. J. Inorg. Chem., 2002, 1285 CrossRef CAS.
- T. Schwarz, D. Hess and P. Klufers, Dalton Trans., 2010, 39, 5544 RSC.
- T. Allscher, X. Kastele, G. Kettenbach, P. Klufers and T. Kunte, Chem. – Asian J., 2007, 2, 1037 CrossRef CAS PubMed.
- S. Ghosh and P. S. Mukherjee, Organometallics, 2007, 26, 3362 CrossRef CAS.
- D. Tripathy, V. Ramkumar and D. K. Chand, Cryst. Growth Des., 2013, 13, 3763 CAS.
- N. B. Debata, D. Tripathy, V. Ramkumar and D. K. Chand, Tetrahedron Lett., 2010, 51, 4449 CrossRef CAS.
- R. A. Adrian, R. E. Benson, L. M. Daniels, E. R. T. Tiekink and J. A. Walmsley, Acta Crystallogr., Sect. E: Struct. Rep. Online, 2006, 62, m601 CAS.
- A. V. Eremin, D. D. Vaulina, M. A. Stepanova, V. G. Antonov, A. N. Belyaev and S. A. Simanova, Russ. J. Gen. Chem., 2011, 81, 194 Search PubMed.
- M. Yu, Y.-Z. Li, L.-F. Wang, H.-P. Huang and S.-Y. Yu, Chin. J. Inorg. Chem., 2006, 22, 963 CAS.
- A. E. Lapshin and O. V. Magdysyuk, Glass Phys. Chem., 2011, 37, 206 CrossRef CAS.
- A. E. Lapshin and O. V. Magdysyuk, Glass Phys. Chem., 2009, 35, 627 CrossRef CAS.
- R. A. Adrian, G. A. Broker, E. R. T. Tiekink and J. A. Walmsley, Inorg. Chim. Acta, 2008, 361, 1261 CrossRef CAS PubMed.
- J. Garcia-Anton, J. Pons, X. Solans, M. Font-Bardia and J. Ros, Inorg. Chim. Acta, 2004, 357, 571 CrossRef CAS.
- A. Singh and P. R. Sharp, Dalton Trans., 2005, 2080 RSC.
- A. Singh, U. Anandhi, M. A. Cinellu and P. R. Sharp, Dalton Trans., 2008, 2314 RSC.
- P. Brandi-Blanco, P. J. S. Miguel and B. Lippert, Dalton Trans., 2011, 40, 10316 RSC.
- T. Allscher, X. Kastele, G. Kettenbach, P. Klufers and T. Kunte, Chem. – Asian J., 2007, 2, 1037 CrossRef CAS PubMed.
- M. Fujita, S. Nagao, M. Iida, K. Ogata and K. Ogura, J. Am. Chem. Soc., 1993, 115, 1574 CrossRef CAS.
- R. Krieglstein, D. K. Breitinger and G. Liehr, Eur. J. Inorg. Chem., 2001, 3067 CrossRef CAS.
- T. Z. Xie, C. Guo, S. Y. Yu and Y. J. Pan, Angew. Chem., Int. Ed., 2012, 51, 117 Search PubMed.
- D. Abella, V. Blanco, E. Pía, M. Chas, C. Platas-Iglesias, C. Peinador and J. M. Quintela, Chem. Commun., 2008, 2879 RSC.
- C. Peinador, V. Blanco and J. M. Quintela, J. Am. Chem. Soc., 2009, 131, 920 CrossRef CAS PubMed.
- V. Blanco, M. D. García, C. Peinador and J. M. Quintela, Chem. Sci., 2011, 2, 2407 RSC.
- V. Blanco, M. Chas, D. Abella, E. Pia, C. Platas-Iglesias, C. Peinador and J. M. Quintela, Org. Lett., 2008, 10, 409 CrossRef CAS PubMed.
- V. Blanco, A. Gutiérrez, C. Platas-Iglesias, C. Peinador and J. M. Quintela, J. Organomet. Chem., 2009, 74, 6577 CAS.
- M. Chas, D. Abella, V. Blanco, E. Pía, G. Blanco, A. Fernandez, C. Platas-Iglesias, C. Peinador and J. M. Quintela, Chem. – Eur. J., 2007, 13, 8572 CrossRef CAS PubMed.
- G. Sanchez, J. L. Serrano, J. Garcia, G. Lopez, J. Perez and E. Molins, Inorg. Chim. Acta, 1999, 287, 37 CrossRef CAS.
- E. Sinn, C. M. Flynn Junior and R. B. Martin, J. Am. Chem. Soc., 1978, 100, 489 CrossRef CAS.
- A. Schneider, E. Freisinger, B. Beck and B. Lippert, J. Chem. Soc., Dalton Trans., 2000, 837 RSC.
- M. Fujita, M. Aoyagi and K. Ogura, Inorg. Chim. Acta, 1996, 246, 53 CrossRef CAS.
- V. Blanco, M. Chas, D. Abella, C. Peinador and J. M. Quintela, J. Am. Chem. Soc., 2007, 129, 13978 CrossRef CAS PubMed.
- C. Peinador, E. Pía, V. Blanco, M. D. García and J. M. Quintela, Org. Lett., 2010, 12, 1380 CrossRef CAS PubMed.
- J. Ahn, K. H. Park, T. H. Noh and O.-S. Jung, Inorg. Chem. Commun., 2011, 14, 1868 CrossRef CAS.
- J. Ahn, S. M. Kim, K. H. Park, T. H. Noh and O.-S. Jung, Dalton Trans., 2011, 40, 8520 RSC.
- R. S. Forgan, D. C. Friedman, C. L. Stern, C. J. Bruns and J. F. Stoddart, Chem. Commun., 2010, 46, 5861 RSC.
- R. Schneider, M. W. Hosseini, J.-M. Planeix, A. De Cian and J. Fischer, Chem.
Commun., 1998, 1625 RSC.
- D. Tripathy, H. S. Sahoo, V. Ramkumar and D. K. Chand, RSC Adv., 2014, 4, 18595 RSC.
- V. Blanco, D. Abella, E. Pía, C. Platas-Iglesias, C. Peinador and J. M. Quintela, Inorg. Chem., 2009, 48, 4098 CrossRef CAS PubMed.
- W.-Z. Shen, D. Gupta and B. Lippert, Inorg. Chem., 2005, 44, 8249 CrossRef CAS PubMed.
- K. Uehara, T. Ohishi, T. Hirose and N. Mizuno, Inorg. Chem., 2013, 52, 11200 CrossRef CAS PubMed.
- T.-Z. Xie, C. Guo, S.-Y. Yu and Y.-J. Pan, Angew. Chem., Int. Ed., 2012, 51, 1177 CrossRef CAS PubMed.
- P. Klufers and T. Kunte, Angew. Chem., Int. Ed., 2001, 40, 4210 CrossRef CAS.
Footnote |
† Electronic supplementary information (ESI) available. See DOI: 10.1039/c7ce00654c |
|
This journal is © The Royal Society of Chemistry 2017 |
Click here to see how this site uses Cookies. View our privacy policy here.