DOI:
10.1039/D3SU00175J
(Paper)
RSC Sustain., 2023,
1, 1765-1772
Highly efficient synthesis of sustainable bisphenols from hydroxycinnamic acids†
Received
5th June 2023
, Accepted 9th August 2023
First published on 11th August 2023
Abstract
Three stilbene based bisphenols: (E)-4,4′-(ethene-1,2-diyl)diphenol (1), (E)-4,4′-(ethene-1,2-diyl)bis(2-methoxyphenol) (2), and (E)-4,4′-(ethene-1,2-diyl)bis(2,6-dimethoxyphenol) (3) were synthesized from p-coumaric, ferulic, and sinapic acid, respectively. This was accomplished with a high-yielding two-step approach, which combined catalyst-free thermal decarboxylation of the cinnamic acids followed by olefin metathesis. The thermal decarboxylation proceeded in quantitative yield merely by refluxing the cinnamic acids in DMF under a nitrogen atmosphere. The resulting vinyl phenols were briefly isolated and then immediately subjected to olefin metathesis with a Grubbs–Hoveyda 2nd generation catalyst, which resulted in direct precipitation of the bisphenols from the reaction mixture. Overall yields of 1, 2, and 3 were 92, 88, and 85%, respectively, starting from the cinnamic acids. The compounds were isolated as pure solids without the need for crystallization or column chromatography. Bisphenol 1 was isomerized to the cis-isomer by irradiation with UV light. The resulting 80
:
20 cis
:
trans mixture exhibited a glass transition at low temperatures and a decreased melting temperature. To further expand the suite of bisphenols that can be produced from cinnamic acids, p-coumaric acid was directly converted into a 4-vinylphenol dimer by thermal decarboxylation/dimerization in a biphasic aqueous base/toluene solution to yield (E)-4,4′-(but-1-ene-1,3-diyl)diphenol (4). The ability to efficiently produce bisphenols from abundant, low-cost, and easily sourced bio-based substrates holds great promise for the development of more sustainable polymers.
Sustainability spotlight
Bisphenol A (BPA) is an important industrial chemical building block for polycarbonates, coatings, and structural composite materials. However, the derivation of this molecule from petrochemical sources and the negative health impacts of BPA necessitate a transition to more sustainable and less toxic molecules that can be derived from biomass substrates using efficient synthetic methods. To accomplish this goal, we developed an atom economic process for the conversion of hydroxycinnamic acids (readily obtained from lignin) to bisphenols through a two-step method consisting of catalyst-free decarboxylation followed by olefin metathesis. This work aligns with several UN sustainable development goals including: health/well-being (SDG 3), sustainable industrialization (SDG 9), responsible production (SDG 12), climate change (SDG 13) and sustainable terrestrial ecosystems (SDG 14).
|
Introduction
Bisphenols, such as bisphenol A (BPA; 2,2-bis(4-hydroxyphenyl)propane), are commodity chemicals used in the synthesis of polyethers, polycarbonates, and epoxy resins.1,2 However, most bisphenol-based polymers are made from petroleum sources and not widely recycled, which leads to significant negative impacts on the environment.3,4 Further, the synthesis of BPA-like bisphenols results in the formation of isomeric and oligomeric products and requires several equivalents of phenol.5,6 This unsustainable and low atom-economy synthetic route, along with the documented health risks of BPA and similar bisphenols,7–9 has inspired the study of lower toxicity, sustainable alternatives that can be derived from biomass feedstocks in an efficient manner.2,10
Processing biomass to access platform chemicals is a key strategy for producing sustainable materials. Important feedstocks such as fatty acids, phenols, and hydrocarbons can be produced through the valorization or fermentation of biomass substrates and used for the preparation of fuels, polymers, and composite materials.11–13 A number of studies have demonstrated that bio-based bisphenols are useful synthons for the production of sustainable thermoplastics and thermosetting resins.14–17 This previous work includes studies on cyanate ester resins and polycarbonates based on bisphenols derived from vanillin, eugenol, and resveratrol.16,18–23 Recently, several groups have reported bio-based bisphenols with little to no estrogenic effects when compared to BPA.24–29 The presence of sterically hindering functional groups (e.g. methoxy) ortho to the phenolic –OH groups appears to reduce estrogenic effects by preventing binding at the active sites of estrogen receptors.26
Hydroxycinnamic acids such as p-coumaric, ferulic, and sinapic acid are phenolic compounds that can be isolated from lignin and used as valuable synthons for the preparation of bisphenols and polymeric materials.30–32 These α,β-unsaturated carboxylic acid functionalized phenols are ubiquitous components of the cell walls of plants and have previously been utilized to generate polymers with remarkable thermal properties,33,34 biodegradability,35,36 and liquid crystallinity.36–38 Hydroxycinnamic acids can be efficiently extracted from woody biomass with purities of up to 99%. Extraction can be conducted through alkaline39–42 or enzymatic hydrolysis43,44 followed by solvent extraction,45,46 adsorption onto nanomaterials or membranes,47,48 or counter-current chromatography (Fig. 1).49,50 Decarboxylation of these hydroxycinnamic acids followed by dimerization of the subsequent vinyl phenols is an intriguing approach for the formation of sustainable bisphenols. However, procedures for synthesizing bisphenols from hydroxycinnamic acid bio-feedstocks typically require metal catalysts51,52 or enzymes53–56 for the initial decarboxylation step. Few examples of catalyst-free thermal decarboxylation of cinnamic acids exist. However, recently, Yang and coworkers synthesized various functionalized 4-vinylphenols from hydroxycinnamic acids by heating dilute solutions of the acids in N,N-dimethylformamide (DMF), above the boiling point, in sealed reactors.57 This approach eliminated the need for catalysts or other additives, but may be difficult to scale-up due to the use of low concentrations and elevated pressures.
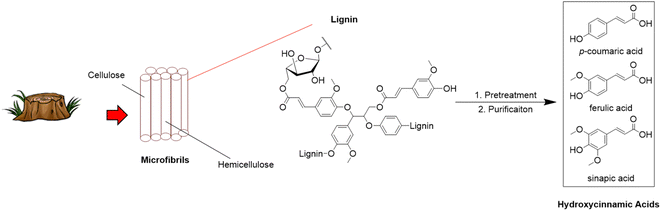 |
| Fig. 1 Extraction of hydroxycinnamic acids from biomass-derived lignin. | |
Dimerization of 4-vinylphenols to yield bisphenols can be accomplished through Ru-catalyzed olefin metathesis.56,58 Our group has previously employed this approach for the synthesis of high-performance renewable thermosets and thermoplastics from eugenol.16 Recently, Baraibar and coworkers developed a one-pot cascade reaction to synthesize bisphenols directly from hydroxycinnamic acids. The decarboxylation step was accomplished with a phenolic acid decarboxylase derived from Bacillus subtilis.56 The Ru-catalyst loading for the dimerization step was quite high (5%), which would ultimately limit sustainability and scalability due to the cost and scarcity of ruthenium. The scalability of the reported approach was further hindered by the need for flash chromatography to purify the bisphenol products. Finally, the enzymatic approach failed to catalyze the decarboxylation of the highly-substituted sinapic acid.
To develop an efficient and sustainable pathway for the synthesis of bisphenols from naturally abundant hydroxycinnamic acid feedstocks, this work describes the catalyst-free thermal decarboxylation of the acids followed by Ru-catalyzed olefin metathesis to afford bisphenol compounds in outstanding yields (Scheme 1a). In addition, a second thermal dimerization route was studied for p-coumaric acid that eliminated the need for a metal catalyst (Scheme 1b). To further diversify the products obtainable from hydroxcinnamic acids, we studied the photochemical isomerization of the trans-stilbene isomer derived from p-coumaric acid to generate the cis-isomer (Scheme 1c). We also attempted the direct synthesis of the cis-isomer from 4-vinylphenol using a Z-selective olefin metathesis catalyst (Scheme 1d).
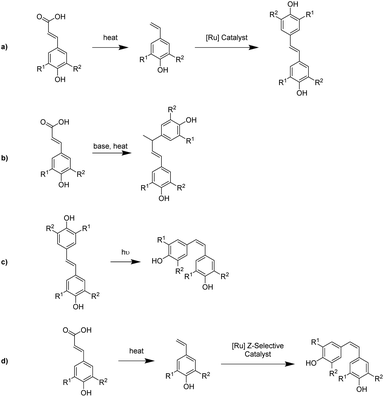 |
| Scheme 1 Synthesis of bio-based bisphenols from hydroxycinnamic acids through (a) catalyst-free decarboxylation and Ru-catalysis, (b) thermal dimerization, (c) photoisomerization of trans-bisphenols to cis-isomers, and (d) direct conversion of vinyl phenols to cis-isomers using a Z-selective catalyst. | |
Experimental details
General considerations
Unless otherwise noted, all reagents were purchased and used as received from the manufacturer without further purification. 1H and 13C NMR spectra were recorded on a JEOL 400 MHz spectrometer. All NMR chemical shifts are reported in ppm downfield from tetramethylsilane and are referenced relative to the NMR solvent according to the literature values: 1H NMR (CDCl3 = 7.26 ppm, DMSO-d6 = 2.50 ppm, (CD3)2CO = 2.05 ppm); 13C NMR (CDCl3 = 77.16 ppm, DMSO-d6 = 39.52 ppm, (CD3)2CO = 29.84 ppm). Olefin metathesis reactions were prepared and performed under an inert atmosphere of N2 using either a Schlenk line or glovebox.
Synthesis of (E)-4,4′-(ethene-1,2-diyl)diphenol from p-coumaric acid (1)
In a 250 mL round bottom flask, p-coumaric acid (50 g, 0.3 mol) was dissolved in 100 mL of DMF. The flask was fitted with a condenser and heated to reflux with vigorous stirring for 6 h. The flask was then cooled to ambient temperature and the solution poured into water (1 L). This mixture was then extracted with 3 × 200 mL EtOAc and washed with water (100 mL) followed by a 5% LiCl solution (2 × 200 mL) to remove excess DMF. The organic layer was dried over anhydrous MgSO4, and concentrated under reduced pressure to yield a yellow solid. In a nitrogen-filled glovebox, Hoveyda–Grubbs Catalyst® M720 (1.91 g, 1 mol%) and toluene (160 mL) were added to the residue. The resulting dark red solution was then transferred to a Schlenk line and stirred at 80 °C for 18 h. The product rapidly started precipitating out of solution. After the reaction was complete, the solid was collected on a frit, rinsed with hexanes, and dried under reduced pressure to afford a tan powder (29.70 g, 0.140 mol, 92% yield). The NMR data were consistent with literature reports.59 mp. 284–290 °C. 1H NMR (400 MHz, DMSO-d6) δ 9.46 (s, 2H, Ar-OH), 7.34 (d, J = 8.6 Hz, 4H, Ar-H), 6.86 (s, 2H, C(sp2)-H), 6.74 (d, J = 8.6 Hz, 4H, Ar-H), 13C NMR (100 MHz, DMSO-d6) δ 157.3, 129.1, 127.9, 125.7, 116.0.
Synthesis of (E)-4,4′-(ethene-1,2-diyl)bis(2-methoxyphenol) (2) from ferulic acid
In a similar manner to the procedure described above, ferulic acid (5 g, 0.026 mol) was decarboxylated in DMF to yield a yellow oil. In an N2 filled glovebox, Hoveyda–Grubbs Catalyst® M720 (0.161 g, 1 mol%) and toluene (30 mL) were added to the residue. The metathesis reaction was conducted as described above to afford 3.08 g of product (88%) as a tan solid. The NMR data were consistent with literature reports.60 mp. 217–220 °C. 1H NMR (400 MHz, DMSO-d6) δ 9.03 (s, 2H, Ar-OH), 7.14 (s, 2H, Ar-H), 6.93 (d, J = 9.5 Hz, 4H, Ar-H), 6.75 (d, J = 7.7 Hz, 2H, 2× C(sp2)-H), 3.82 (s, 6H, OCH3), 13C NMR (100 MHz, DMSO-d6) 146.8, 145.4, 130.3, 126.6, 120.2, 114.6, 108.1, 56.0.
Synthesis of (E)-4,4′-(ethene-1,2-diyl)bis(2,6-dimethoxyphenol) (3) from sinapic acid
In similar manner to the procedure described above, sinapic acid (5 g, 0.022 mol) was decarboxylated in refluxing DMF (20 mL). After the standard workup, the resulting vinylphenol was isolated as a yellow oil. In an N2-filled glovebox, Hoveyda–Grubbs Catalyst® M720 (0.140 g, 1 mol%) and toluene (30 mL) were added to the residue. The metathesis reaction was conducted as described above to yield 3.15 g of the product (85%) as a tan solid. The NMR data were consistent with literature reports.61 mp. 256–261 °C. 1H NMR (400 MHz, DMSO-d6) δ 8.40 (s, 2H, Ar-OH), 6.99 (s, 2H, C(sp2)-H), 6.84 (s, 4H, Ar-H), 3.81 (s, 12H, -OCH3), 13C NMR (100 MHz, DMSO-d6) δ 153.3, 134.6, 133.4, 128.1, 103.6, 79.8, 77.4, 59.2, 55.9.
Synthesis of (E)-4,4′-(but-1-ene-1,3-diyl)diphenol (4)
To a 250 mL round-bottomed flask equipped with a reflux condenser, 4-hydroxycinnamic acid (4.92 g, 0.03 mol), toluene (75 mL), and NaOH (1.26 g, 0.0315 mol) in DI water (45 mL) were added under nitrogen. The bilayered solution was stirred vigorously and refluxed for 24 hours. After allowing the mixture to cool, the aqueous phase was neutralized and extracted with ethyl acetate (3 × 100 mL). The organic phase was collected, dried with anhydrous MgSO4, filtered, and the solvent removed under reduced pressure to obtain a viscous oil containing the 4-hydroxystyrene dimer along with some oligomeric material (1.95 g, 54% yield). The crude material was then purified via column chromatography (Rf: 0.48, 1
:
1 EA
:
Hex) to afford the product as a white solid. The NMR data were consistent with literature reports.62 mp. 210–214 °C. 1H NMR (400 MHz, (CD3)2CO) δ 8.24 (s, 1H, OH), 8.04 (s, 1H, OH), 7.21 (d, J = 8.4 Hz, 2H, Ar-H), 7.06 (d, J = 8.3 Hz, 2H, Ar-H), 6.73 (dd, J = 8.5, 3.3 Hz, 4H, Ar-H), 6.31–6.15 (m, 2H, C(sp2)-H), 3.48 (m, 1H, CH), 1.32 (d, J = 7.3 Hz, 3H, CH3) 13C NMR (100 MHz, DMSO-d6) δ 157.1, 156.0, 136.5, 133.0, 130.4, 128.7, 128.3, 127.7, 127.6, 115.8, 115.6, 44.5, 22.2.
Photochemical isomerization of 1
500 mg of 1 were added to a 100 mL quartz flat-bottom flask. Dry MeCN (50 mL) was then added and the mixture sparged with N2 for 45 min. The flask was then placed in a Luzchem® LZC-4V photoreactor fitted with fourteen 355 nm, 8 W fluorescent lamps and irradiated with stirring for 2 h. The reaction mixture was then filtered and the filtrate concentrated under reduced pressure to yield a viscous oil. Approximately 3 mL of MeCN were added to the residual oil, which resulted in dissolution of most of the product along with precipitation of 1 as a tan solid. The solid was collected on a fine fritted funnel and the filtrate was then concentrated under reduced pressure to yield an 8
:
2 mixture of cis
:
trans1 as a viscous brown oil (455 mg, 91% yield). mp. 256–259 °C. 1H NMR of the cis-isomer (400 MHz, (CD3)2CO) δ 8.35 (s, 2H, Ar-OH), 7.08 (d, J = 8.54 Hz, 4H, Ar-H), 6.69 (d, J = 8.5 Hz, 4H, Ar-H), 6.35 (s, 2H, C(sp2)-H).
Results and discussion
We began our study with p-coumaric acid, an unsubstituted hydroxycinnamic acid, as a model substrate for optimization of reaction conditions. Unlike previous work, the decarboxylation of p-coumaric acid was not conducted in a sealed tube but instead was conducted under atmospheric pressure in a round-bottom flask equipped with a condenser.57p-Coumaric acid was dissolved in DMF and heated to reflux. After one hour, we observed a 41% conversion of the hydroxycinnamic acid. Running the reaction for 4 hours resulted in a 56% conversion and 6 hours yielded full conversion of the starting material.
After a standard workup, crude 4-vinylphenol was obtained as an off-white solid contaminated with residual DMF. Uninhibited 4-vinylphenol partially polymerized overnight, even at reduced temperature (4 °C), which greatly reduced the yield for the subsequent homometathesis step (Table 1, entry 1). To remedy this issue, the vinyl phenol was immediately allowed to react with Grubbs 2nd generation catalyst M204 (1 mol%) in toluene at 80 °C to obtain the desired bisphenol [(E)-4,4′-(ethene-1,2-diyl)diphenol] (1) in 29% yield. The product directly precipitated from the reaction solution and could be isolated in high purity without recrystallizing the product or subjecting it to column chromatography (Table 1, entry 2). Scale-up of the reaction to 50 g of p-coumaric acid resulted in a similar (30%) yield of the bisphenol (Table 1, entry 3). These initial results were promising, but the modest yield of the bisphenol was of concern due to the low carbon efficiency and sustainability of the process.
Table 1 Optimization of reaction conditions for the synthesis of bisphenols from hydroxycinnamic acids
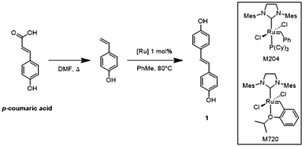
|
Entry |
Scale of p-coumaric acid |
5% LiCl (aq) wash |
Ru-catalyst |
Catalyst loading (%) |
Yield of 1 |
Vinylphenol left overnight in the solid phase.
|
1a |
5 g |
No |
M204 |
1 |
Trace |
2 |
5 g |
No |
M204 |
1 |
29% |
3 |
50 g |
No |
M204 |
1 |
30% |
4 |
50 g |
Yes |
M720 |
1 |
92% |
5 |
5 g |
Yes |
M720 |
0.1 |
65% |
Several studies have shown that DMF, even in small quantities, can be problematic for [Ru]-catalyzed olefin metathesis due to coordination of DMF to the metal center and subsequent inhibition of the catalyst.63 To mitigate this issue, the organic layer from the decarboxylation step was carefully washed with a 5% aqueous LiCl solution to drive off any residual DMF. To further enhance the yield of the product, the second generation Hoveyda–Grubbs Catalyst® M720 (1 mol%), a more stable catalyst, was used for the metathesis reaction. This allowed us to obtain a 92% overall yield of 1 (from p-coumaric acid) at the 50 g scale (Table 1, entry 4). In a similar fashion to the initial reactions, the product readily precipitated from the reaction mixture and was isolated simply by filtration followed by solvent washing. Reducing the catalyst loading to 0.1% resulted in a 65% yield of bisphenol (Table 1, entry 5), representing a minimum turnover number (TON) of 650. Previous work has shown that catalyst loadings as low as 0.009 mol%, an order-of-magnitude lower than demonstrated here, are effective for the synthesis of trans-stilbene from styrene.64,65 In the current case, the presence of acidic phenolic groups and the purity of the substrate limit the TON. Protection of the phenolic groups and/or purification of the vinyl phenol through column chromatography would likely allow for an increased TON, but at the expense of additional synthetic complexity as well as increased solvent, reagent, and energy use, which would dramatically reduce the sustainability of our approach.
Application of the optimized reaction conditions to ferulic acid resulted in an 88% yield of (E)-4,4′-(ethene-1,2-diyl)bis(2-methoxyphenol) (2) (Scheme 2). These conditions were also effective for the decarboxylation/metathesis of the stubborn sinapic acid, affording an 85% overall yield of (E)-4,4′-(ethene-1,2-diyl)bis(2,6-dimethoxyphenol) (3) (Scheme 2). Suitable crystals for X-ray analysis of 3 were obtained via slow evaporation of dichloromethane. The X-ray structure (Fig. 2) confirmed a planar molecule with the expected trans orientation. Close non-bonded interactions designated as non-classical hydrogen bonds (C–H–π) were observed between alkene and phenyl hydrogens and the oxygens of the hydroxyl and methoxy groups of a neighboring molecule (Fig. S7†).
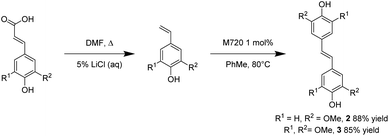 |
| Scheme 2 Synthesis of bisphenols from sinapic and ferulic acid using optimized reaction conditions. | |
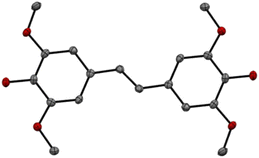 |
| Fig. 2 X-ray structure of 3 with thermal ellipsoids at 50% probability. | |
Differential Scanning Calorimetry (DSC) analysis of the bisphenols revealed melting points of 280 °C, 215 °C, and 250 °C for compounds 1, 2, and 3 respectively (Fig. S1–S3†). These melting points were confirmed using a Mel-Temp apparatus. High melting points of bisphenols can be detrimental to the processability of derivative thermosetting resins. One way to decrease the melting point is through isomerization of the trans-stilbene moiety to the cis-isomer.66 This can be accomplished through irradiation of the compound with UV light.67,68 To this end, we subjected 1 in dry, degassed MeOH to 355 nm light in a photoreactor for 24 h to obtain a mixture of the cis- and trans-bisphenol. Under these conditions, several diastereomeric dimers were formed by [2 + 2]-cycloaddition (Scheme 3) and observed as a series of doublets in the 1H NMR spectrum of the mixture (Fig. S18†). It has previously been reported that cyclobutanes and phenanthrenes can be made from stilbenes when irradiated in methanol for long periods of time.69,70 To avoid these side reactions, acetonitrile was used as the solvent and the photochemical reaction was limited to two hours. This resulted in an 8
:
2 cis
:
trans mixture (Scheme 3) with no detectable formation of cyclobutane-containing products. Thermal analysis of this material revealed a complex DSC with an apparent glass transition at 3 °C and a depressed melting point compared to 1 (Fig. S5†). It's unclear how this difference will impact the properties of derivative polymers, but one would expect it to have a positive effect on processability. Efforts to obtain a pure cis isomer from the 8
:
2 product mixture via column chromatography or fractional crystallization were unsuccessful. In an effort to establish a more direct route to the cis-isomer, we attempted the metathesis reaction of 4-vinylphenol with a Z-selective Grubbs catalyst, M2001. Unfortunately, the catalyst decomposed under the standard reaction conditions and none of the cis-isomer was produced. This result was attributed to catalyst sensitivity to the hydroxyl groups of the vinyl phenol.
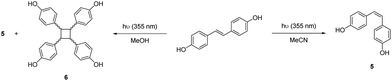 |
| Scheme 3 Photoisomerization of 1 to cis isomer 5. | |
To further expand on the suite of bisphenols accessible from hydroxycinnamic acids, we explored a catalyst-free pathway utilizing thermal decarboxylation/dimerization to generate (E)-4,4′-(but-1-ene-1,3-diyl)diphenol (4) from p-coumaric acid (Scheme 1b), R1 = R2 = H. Initially, an overnight neat thermal dimerization at 120 °C in the presence of a radical inhibitor was attempted from pure 4-vinylphenol isolated as described above. However, the vinyl phenol underwent spontaneous polymerization to generate poly(4-vinylphenol) (PVP) (Scheme 4, Fig. S16 and S17†),71,72 which plays an important role in the electronics industry and is utilized in dielectric, photoresist, and energy storage based applications.73 DSC of the PVP showed a Tg of 170 °C while gel permeation chromatography (GPC) indicated an Mw of 16
570 with a dispersity index (Mw/Mn) of 2.0. In an attempt to increase the selectivity to dimer, a modified metal-free regioselective dimerization procedure was then attempted utilizing a catalytic amount of a Brønsted acid in a ketone-based solvent system.62 4-Vinylphenol generated from p-coumaric acid was dissolved in either 3-pentanone or cyclopentanone (1
:
1) with a catalytic amount of p-toluene sulfonic acid (PTSA) (1
:
12). The reaction was conducted at various times and temperatures ranging from 1–24 hours and room temperature to 120 °C, but consistently yielded a polymerized product upon workup.
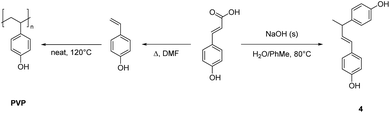 |
| Scheme 4 Thermal dimerization of p-coumaric acid. | |
Previous studies by Hosoda et al. demonstrated decarboxylative coupling of 4-hydroxycinnamic acids in the presence of base catalysts, using a biphasic (water/toluene) solvent system.74 Using a similar approach, p-coumaric acid was heated overnight in a biphasic aqueous sodium hydroxide/toluene mixture to yield the dimer as a white solid (Scheme 4). This coupling can be accomplished in a single step under moderate reaction conditions, but suffers from a modest yield and the need to purify the product by either column chromatography or crystallization. The three-carbon bridge between the aromatic rings could reduce the Tg of derivative thermosetting resins, but the greater flexibility of the linkage may allow for the isolation of more processable monomers.
Conclusions
This work describes efficient methods for the conversion of hydroxycinnamic acids to a family of bisphenols. The ability to derive the starting materials from abundant biomass sources opens the door to large scale production of sustainable building blocks that can be used for a variety of applications including coatings, structural composites, electronic devices, food packaging and optical devices. In addition to the sustainability of the approach, bisphenols derived from ferulic and sinapic acid are expected to have reduced estrogenic effects due to the methoxy groups ortho to the phenolic –OH groups. Future work on these materials should include the synthesis and characterization of various thermosetting resins and thermoplastic polymers generated from the bisphenols as well as efforts to reduce catalyst loadings for bisphenol synthesis and/or the exploration of metathesis catalysts based on cheaper and more abundant transition metals. To further enhance the sustainability of our approach, it would be interesting to explore alternatives to DMF and toluene for the synthesis of the bisphenols. In particular, the use of a bio-based solvent (e.g. 2-methyltetrahydrofuran) for the metathesis reactions would be a simple approach to reduce the carbon intensity of the process. Other topics may include the study of more efficient, catalyst-free routes to the thermal dimer as well as hydrogenated bisphenols derived from bisphenols 1–4. Studies along these lines are ongoing in our laboratory.
Author contributions
Cristian Zavala: investigation, methodology, writing-original draft. Natalie Vest: investigation. Joshua Baca: investigation, writing-review and editing. Derek Zhang: investigation. Randall McClain: investigation. Benjamin Harvey: conceptualization, supervision, writing-review and editing.
Conflicts of interest
There are no conflicts to declare.
Acknowledgements
The authors gratefully acknowledge funding from the Office of Naval Research and support for N.A.V. from the Department of Defense (DoD) SMART program. We also thank Dr Lawrence Baldwin for conducting differential scanning calorimetry experiments.
Notes and references
- D. Chen, K. Kannan, H. Tan, Z. Zheng, Y.-L. Feng, Y. Wu and M. Widelka, Bisphenol Analogues Other Than BPA: Environmental Occurrence, Environ. Sci. Technol., 2016, 50(11), 5438–5453 CrossRef CAS PubMed
.
- F. Liguori, C. Moreno-Marraodan and P. Barbaro, Biomass-derived chemical substitutes forbisphenol A: recent advancements in catalytic synthesis, Chem. Soc. Rev., 2020, 49, 6329–6363 RSC
.
- S. Suh and J. Zheng, Strategies to reduce the global carbon footprint of plastics, Nat. Clim. Change, 2019, 9, 374–378 CrossRef
.
- K. Ragaert, L. Delva and K. Van Greem, Mechanical and chemical recycling of solid plastic waste, Waste Manag., 2017, 69, 24–58 CrossRef CAS PubMed
.
- M. Terasaki, M. Nomachi, J. S. Edmonds and M. Morita, Impurities in industrial grade 4,4′-isopropylidene diphenol (bisphenol A): possible implications for estrogenic activity, Chemosphere, 2004, 55(6), 927–931 CrossRef CAS PubMed
.
- J. M. Pahigian and Y. Zuo, Occurrence, endocrine-related bioeffects and fate of bisphenol A chemical degradation intermediates and impurities: a review, Chemosphere, 2018, 207, 469–480 CrossRef CAS PubMed
.
- V. Mustieles, S. C. D'Cruz, S. Courderq, A. Rodriguez-Carillo, J.-B. Fini, T. Hofer, I.-L. Steffensen, H. Dirven, R. Barouki, N. Olea, M. F. Fernandez and A. David, Bisphenol A and its analogues: A comprehensive review to identify, Environ. Int., 2020, 144, 105811 CrossRef CAS PubMed
.
- J. G. Hengstler, H. Foth, T. Gebel, P. J. Kramer, W. Lilienblum, H. Schweinfurth, W. Volkel, K. M. Wollin and U. Gundert-Remy, Critical evaluation of key evidence on the human health hazards of exposure to bisphenol A, Crit. Rev. Toxicol., 2011, 41(4), 263–291 CrossRef CAS PubMed
.
- M. S. Muhamad, M. R. Salim, W. J. Lau and Z. Yusop, A review on bisphenol A occurrences, health effects and treatment process via membrane technology for drinking water, Environ. Sci. Pollut. Res., 2016, 23, 11549–11567 CrossRef CAS PubMed
.
- F. A. M. M. Goncalves, M. Santos, T. Cernadas, P. Ferreira and P. Alves, Advances in the development of bio based epoxy resins: insight into more sustainable materials and future applications, Int. Mater. Rev., 2022, 67(2), 119–149 CrossRef CAS
.
- C. Okkerse and H. Van Bekkum, From Fossil to Green, Green Chem., 1999, 1, 107–114 RSC
.
- C.-C. Chen, L. Dai, L. Ma and R.-T. Guo, Enzymatic degradation of plant biomass and synthetic polymers, Nat. Rev. Chem, 2020,(4), 114–126 CrossRef PubMed
.
-
M. Jawaid, P. M. Tahir and N. Saba, Lignocellulosic Fibre and Biomass-Based Composite Materials: Processing, Properties and Applications, Woodhead Publishing, 2017 Search PubMed
.
- N. Ramdani, H. Zaimeche and M. Derradji, Biobased thermally-stable aromatic cyanate ester thermosets: a review, React. Funct. Polym., 2021, 168, 105037 CrossRef CAS
.
- H. A. Meylemans, T. J. Groshens and B. G. Harvey, Synthesis of Renewable Bisphenols from Creosol, ChemSusChem, 2012, 5, 206–1210 CrossRef CAS PubMed
.
- B. G. Harvey, C. M. Sahagun, A. J. Guenthner, T. J. Groshens, L. R. Cambrea, J. T. Reams and J. M. Mabry, A High-Performance Renewable Thermosetting Resin Derived from Eugenol, ChemSusChem, 2014, 7(7), 1964–1969 CrossRef CAS PubMed
.
- B. G. Harvey, A. J. Guenthner, W. W. Lai, H. A. Meylemans, M. C. Davis, L. R. Cambrea, J. T. Reams and K. R. Lamison, Effects of o-Methoxy Groups on the Properties and Thermal Stability of Renewable High-Temperature Cyanate Ester Resins, Macromolecules, 2015, 48(10), 3173–3179 CrossRef CAS
.
- H. A. Meylemans, B. G. Harvey, J. T. Reams, A. J. Guenthner, L. R. Cambrea, T. J. Groshens, L. C. Baldwin, M. D. Garrison and J. M. Mabry, Synthesis, Characterization, and Cure Chemistry of RenewableBis(cyanate) Esters Derived from 2-Methoxy-4-Methylphenol, Biomacromolecules, 2013, 14, 771–780 CrossRef CAS PubMed
.
- B. G. Harvey, A. J. Guenthner, H. A. Meylemans, S. R. L. Haines, K. R. Lamison, T. J. Groshens, L. R. Cambrea, M. C. Davis and W. W. Lai, Renewable thermosetting resins and thermoplastics from vanillin, Green Chem., 2015, 17(2), 1249–1258 RSC
.
- J. J. Cash, M. C. Davis, M. D. Ford, T. J. Groshens, A. J. Guenthner, B. G. Harvey, K. R. Lamison, J. M. Mabry, H. A. Meylemans, J. T. Reams and C. M. Sahagun, High Tg thermosetting resins from resveratrol, Polym. Chem., 2013, 4, 3859–3865 RSC
.
- M. D. Garrison and B. G. Harvey, Structure-property relationships of cis-resveratrol cyanate ester blends, Polymer, 2021, 213, 123194 CrossRef CAS
.
- B. G. Harvey, A. J. Guenthner, G. R. Yandek, L. R. Cambrea, H. A. Meylemans, L. C. Baldwin and J. T. Reams, Synthesis and characterization of a renewable cyanate ester/polycarbonate network derived from eugenol, Polymer, 2014, 55(20), 5073–5079 CrossRef CAS
.
- M. D. Garrison, M. A. Savolainen, A. P. Chafin, J. E. Baca, A. M. Bons and B. G. Harvey, Synthesis and Characterization of High-Performance, Bio-Based Epoxy–Amine Networks Derived from Resveratrol, ACS Sustainable Chem. Eng., 2020, 8(37), 14137–14149 CrossRef CAS
.
- M. D. Garrison, P. J. Storch, W. S. Eck, V. H. Adams, P. W. Fedick and B. G. Harvey, BPA-free high-performance sustainablepolycarbonates derived from non-estrogenicbio-based phenols, Green Chem., 2021, 23, 8016–8029 RSC
.
- Y. Sun, L. Sun, L. Hong, J. Li, S. Tang and C. Zhao, Bio-based Non-estrogenic Dimethacrylate Dental Composite from Cloves, J. Dent. Res., 2022, 101(13), 1613–1619 CrossRef CAS PubMed
.
- S. F. Koelewijn, S. Van de Bosch, T. Renders, W. Schutyser, B. Lagrain, M. Smet, J. Thomas, W. Dehaen, P. Van Puyvelde, H. Witters and B. F. Sels, Sustainable bisphenols from renewable softwood lignin feedstock for polycarbonates and cyanate ester resins, Green Chem., 2017, 19(11), 2561–2570 RSC
.
- H. Hong, B. G. Harvey, G. R. Palmese, J. F. Stanzione, H. W. Ng, S. Sakkiah, W. Tong and J. M. Sadler, Experimental Data Extraction and in Silico Prediction of the Estrogenic Activity of Renewable Replacements for Bisphenol A, Int. J. Environ. Res. Public Health, 2016, 13(7), 705 CrossRef PubMed
.
- A. Maiorana, A. F. Reano, R. Centore, M. Grimaldi, P. Balaguer, F. Allais and R. A. Gross, Structure property relationships of bio based n-alkyl bisferulate epoxy resins, Green Chem., 2016, 18(18), 4961–4973 RSC
.
- M. Janvier, L. Hollande, A. S. Jaufurally, M. Pernes, R. Ménard, M. Grimaldi, J. Beaugrand, P. Balaguer, P.-H. Ducrot and F. Allais, Syringaresinol: A Renewable and Safer Alternative to Bisphenol A for Epoxy-Amine Resins, ChemSusChem, 2017, 10(4), 738–746 CrossRef CAS PubMed
.
-
C. M. Fellow, T. C. Brown and W. O. S. Doherty, Lignocellulosics as a Renewable Feedstock for Chemical Industry: Chemicals from Lignin, in Green Chemistry for Environmental Remediation, 2011, pp. 561–610 Search PubMed
.
- I. Barbara, A. L. Flourat and F. Allais, Renewable polymers derived from ferulic acid and biobased diols via ADMET, Eur. Polym. J., 2015, 62, 236–243 CrossRef CAS
.
- A. L. Flourat, J. Combes, C. Bailly-Maitre-Grand, K. Magnien, A. Haudrechy, J.-H. Renault and F. Allais, Accessing p-Hydroxycinnamic Acids: Chemical Synthesis, Biomass Recovery, or Engineered Microbial Production?, ChemSusChem, 2021, 14(1), 118–129 CrossRef CAS PubMed
.
- L. Mialon, A. G. Pemba and S. A. MIller, Biorenewable polyethylene terephthalate mimics derived from lignin and acetic acid, Green Chem., 2010, 12(10), 1704–1706 RSC
.
- A. G. Pemba, M. Rostagno, T. A. Lee and S. A. Miller, Cyclic and spirocyclic polyacetal ethers from lignin-based aromatics, Polym. Chem., 2014, 5(9), 3214–3221 RSC
.
- M. A. Ouimet, J. Griffin, A. L. Carbone-Howell, W.-H. Wu, N. D. Stebbins and K. E. Uhrich, Biodegradable Ferulic Acid-Containing Poly(anhydride-ester): Degradation Products with Controlled Release and Sustained Antioxidant Activity, Biomacromolecules, 2013, 14(3), 854–861 CrossRef CAS PubMed
.
- J. Du, Y. Fang and Y. Zheng, Synthesis, characterization and biodegradation of biodegradable-cum-photoactive liquid-crystalline copolyesters derived from ferulic acid, Polymer, 2007, 48(19), 5541–5547 CrossRef CAS
.
- J. Stumpe, A. Ziegler, M. Berghahn and H. R. Kricheldorf, Photoreactive Cholesteric Polyesters Derived from 4-Carboxycinnamic Acid and Novel Chiral Spacers, Macromolecules, 1995, 28(15), 5306–5311 CrossRef CAS
.
- Q. Zhao and W. Wu, Syntheses and photoluminescence properties of UV photocrosslinkable polyesters based on fluorene, Polymer, 2009, 50(4), 998–1004 CrossRef CAS
.
- A. U. Buranov and G. Mazza, Extraction and purification of ferulic acid from flax shives, wheat and corn bran by alkaline hydrolysis and pressurised solvents, Food Chem., 2009, 115, 1542–1548 CrossRef CAS
.
- M. Chadni, A. Flourat, V. Reungoat, L. M. M. Mouterde, F. Allais and I. Ioannou, Selective Extraction of Sinapic Acid Derivatives from Mustard Seed Meal by Acting on pH: Toward a High Antioxidant Activity Rich Extract, Molecules, 2021, 26(1), 212 CrossRef CAS PubMed
.
- V. I. Timokhin, M. Regner, A. H. Motagamwala, C. Stener, S. D. Karlen, J. A. Dumesic and J. Ralph, Production of p-Coumaric Acid from Corn GVL-Lignin, ACS Sustainable Chem. Eng., 2020, 8(47), 17427–17438 CrossRef CAS
.
- S. I. Mussato, G. Dragone and I. C. Roberto, Ferulic and p-coumaric acids extraction by alkaline hydrolysis of brewer's spent grain, Ind. Crops Prod., 2007, 25(2), 231–237 CrossRef
.
- I. Antonopoulou, E. Sapountzaki, U. Rova and P. Christakopoulos, Ferulic Acid From Plant Biomass: A Phytochemical With Promising Antiviral Properties, Front. Nutr., 2022, 8, 777576 CrossRef PubMed
.
- L. Quinn, S. G. Gray, S. Meaney, S. Finn, P. McLoughlin and M. Hayes, Extraction and Quantification of Sinapinic Acid from Irish Rapeseed Meal and Assessment of Angiotensin-I Converting Enzyme (ACE-I) Inhibitory Activity, J. Agric. Food Chem., 2017, 65(32), 6886–6892 CrossRef CAS PubMed
.
- J. Salgado, B. Max, R. Rodriguez-Solana and J. Dominguez, Purification of ferulic acid solubilized from agroindustrial wastes and further conversion into 4-vinyl guaiacol by Streptomyces setonii using solid state fermentation, Ind. Crops Prod., 2012, 39, 52–61 CrossRef CAS
.
- H. Sibhatu, S. Anuradha Jabasingh, A. Yiman and S. Ahmed, Ferulic acid production from brewery spent grains, an agro-industrial waste, LWT--Food Sci. Technol., 2021, 135, 110009 CrossRef CAS
.
- J. Domingos, A. Teixeira, S. Dupoiron, F. Allais and M. Lameloise, Simultaneous recovery of ferulic acid and sugars from wheat bran enzymatic hydrolysate by diananofiltration, Sep. Purif. Technol., 2020, 242, 116755 CrossRef CAS
.
- P. Ideia, I. Sousa-Ferreira and P. C. Castilho, A Novel and Simpler Alkaline Hydrolysis Methodology for Extraction of Ferulic Acid from Brewer's Spent Grain and its (Partial) Purification through Adsorption in a Synthetic Resin, Foods, 2020, 9(5), 600 CrossRef CAS PubMed
.
- Z. Liu, J. Wang, P. Shen, C. Wang and Y. Shen, Microwave-assisted extraction and high-speed counter-current chromatography purification of ferulic acid from Radix Angelicae sinensis, Sep. Purif. Technol., 2006, 52(1), 18–21 CrossRef CAS
.
- Y. Tang, J. Hao, C. Fan and X. Cao, Preparative separation of high-purity trans- and cis-ferulic acid from wheat bran by pH-zone-refining counter-current chromatography, J. Chromatogr. A, 2021, 1636, 461772 CrossRef CAS PubMed
.
- R. Bernini, E. Mincione, M. Barontini, G. Provenzano and L. Setti, Obtaining 4-vinylphenols by decarboxylation of natural 4-hydroxycinnamic acids under microwave irradiation, Tetrahedron, 2007, 63(39), 9663–9667 CrossRef CAS
.
- S. Hashimoto, S. Michiyo and T. Shiro, The in Vitro Metabolism of 3-(1-Hydroxy-2-piperidinoethyl)-5-phenyl-isoxazole Citrate (31252-S) with Rabbit Liver Homogenate, Chem. Pharm. Bull., 1976, 24(8), 1757–1764 CrossRef CAS PubMed
.
- H. Rodriguez, J. M. Landete, J. A. Curiel, B. de las Rivas, J. M. Mancheno and R. Munoz, Characterization of the p -Coumaric Acid Decarboxylase from Lactobacillus plantarum CECT 748 T, J. Agric. Food Chem., 2008, 56(9), 3068–3072 CrossRef CAS PubMed
.
- K. L. Ferguson, N. Arunrattanamook and E. M. G. Marsh, Mechanism of the Novel Prenylated Flavin-Containing Enzyme Ferulic Acid Decarboxylase Probed by Isotope Effects and Linear Free-Energy Relationships, Biochemistry, 2016, 55(20), 2857–2863 CrossRef CAS PubMed
.
- G. A. Aleku, C. Prause, R. T. Bradshaw-Allen, K. Plasch, S. M. Glueck, S. S. Bailey, A. P. Payne, D. A. Parker, K. Faber and D. Leys, Terminal Alkenes from Acrylic Acid Derivatives via Non-Oxidative Enzymatic Decarboxylation by Ferulic Acid Decarboxylases, ChemCatChem, 2018, 310(17), 3736–3745 CrossRef PubMed
.
- A. Gomez Baraibar, D. Reichert, C. Mugge, S. Seger, H. Groger and R. Kourist, A One-Pot Cascade Reaction Combining an EncapsulatedDecarboxylase with a Metathesis Catalyst for the Synthesis of Bio-Based Antioxidants, Angew. Chem., Int. Ed., 2016, 55(47), 14823–14827 CrossRef CAS PubMed
.
- Q. Yang, Y. Li, H. Liu, E. Wang, M. Peng, T. Deng, X. Pan, L. Zhongsheng, Y. Yan, L. Yang and X. Yang, Catalyst-free decarboxylation of 4-hydroxycinnamic acids:efficient synthesis of4-vinylphenols, R. Soc. Open Sci., 2022, 9, 220014 CrossRef CAS PubMed
.
- K. Ferre-Filmon, L. Delaude, A. Demonceau and A. F. Noels, Stereoselective Synthesis of (E)-Hydroxystilbenoids by Ruthenium-Catalyzed Cross-Metathesis, Eur. J. Org Chem., 2005, 15, 3319–3325 CrossRef
.
- B. Song, G. Liu, R. Xu, S. Yin, Z. Wang and X. Zhang, Interfacial Self-Organization of Bolaamphiphiles Bearing Mesogenic Groups: Relationships between the Molecular Structures and Their Self-Organized Morphologies, Langmuir, 2008, 24(8), 3734–3739 CrossRef CAS PubMed
.
- J. Hitce, M. Crutizat, C. Bourdon, A. Vivès, X. Marat and M. Dalko-Csiba, Flash-metathesis for the coupling of sustainable (poly)hydroxyl β-methylstyrenes from essential oils, Green Chem., 2015, 17(7), 3756–3761 RSC
.
- S. Li and K. Lundquist, Stereoisomers of 3,3′-dimethoxy-4,4′-stilbenequinone, Holzforschung, 2005, 59(3), 282–284 CAS
.
- F. R. Fehn, S. Schwarz and R. Kempe, Regioselective Heterodimerization of Styrenes with Diarylethene and Vinylnaphthalene by a Catalyst System Consisting of Simple Acid and a Ketone, ChemistrySelect, 2017, 2, 3289 CrossRef
.
-
B. Grubbs, P. Wheeler, B. Tran, D. Stoianova, J. Phillips, D. Pederson and A. Johns, Olefin Metethesis Application Guide Materia, 2016, https://www.materia-inc.com/assets/guides/Materia_Metathesis_Application_Guide.pdf Search PubMed
.
- T. J. Bannin, P. P. Datta, E. T. Kiesewetter and M. K. Kiesewetter, Synthesizing Stilbene by Olefin Metathesis Reaction Using Guided Inquiry To Compare and Contrast Wittig and Metathesis Methodologies, J. Chem. Educ., 2019, 96(1), 143–147 CrossRef CAS
.
- P. Sabila, Styrene Cross-metathesis Using Low Catalyst Concentrations, Univers. J. Chem., 2015, 3(3), 87–90 Search PubMed
.
-
J. March, Advanced Organic Chemistry, John Wiley & Sons, 3rd edn, 1986 Search PubMed
.
- H. Latva-Maenpaa, R. Wufu, D. Mulat, T. Sarjala, P. Saranpaa and K. Wahala, Stability and Photoisomerization of Stilbenes Isolated from the Bark of Norway Spruce Roots, Molecules, 2021, 26(4), 1036 CrossRef CAS PubMed
.
- L. R. Cambrea, M. C. Davis, M. D. Garrison, T. J. Groshens, R. E. Lyon and N. Safronava, Processable cyanate ester resin from Cis resveratrol, J. Polym. Sci., Part A: Polym. Chem., 2017, 55(6), 971–980 CrossRef CAS
.
- B. Danylec, E. M. Campi, C. M. Forsyth, R. I. Boysen and M. T. W. Hearn, Photochemically Induced Solid State Dimerisation of Resveratrol Analogues: A Greener Synthetic Process, Aust. J. Chem., 2020, 73(12), 1260–1270 CrossRef CAS
.
- A. Francioso, A. Boffi, C. Villani, L. Manzi, M. D'Erme, A. Macone and L. Mosca, Isolation and Identification of 2,4,6-Trihydroxyphenanthrene as a Byproduct of trans-Resveratrol Photochemical Isomerization and Electrocyclization, J. Org. Chem., 2014, 79(19), 9381–9384 CrossRef CAS PubMed
.
- K. S. Khuong, W. H. Jones, W. A. Pryor and K. N. Houk, The Mechanism of the Self-Initiated Thermal Polymerization of Styrene. Theoretical Solution of a Classic Problem, J. Am. Chem. Soc., 2005, 127(4), 1265–1277 CrossRef CAS PubMed
.
- F. R. Mayo, The dimerization of styrene, J. Am. Chem. Soc., 1968, 90(5), 1289–1295 CrossRef CAS
.
- S. Choi, S. Song, T. Kim, J. C. Shin, J. W. Jo, S. K. Park and Y. H. Kim, Self-Aligned Top-Gate Metal-Oxide Thin-Film Transistors Using a Solution-Processed Polymer Gate Dielectric, Micromachines, 2020, 11(12), 1035 CrossRef PubMed
.
-
A. Hosada, H. Mori, Y. Miyake, Y. Maeda, T. Kobata and H. Taniguchi, Hydroxystyrene dimer derivative, method for producing the same, chain transfer agent, and method for polymerizing radically polymerizable monomer, Japanese patent, JP2010222285A, 2010
.
|
This journal is © The Royal Society of Chemistry 2023 |
Click here to see how this site uses Cookies. View our privacy policy here.