DOI:
10.1039/D1OB01003D
(Review Article)
Org. Biomol. Chem., 2021,
19, 6746-6760
Silicon compounds as stoichiometric coupling reagents for direct amidation
Received
24th May 2021
, Accepted 18th July 2021
First published on 22nd July 2021
Abstract
Despite being one of the most frequently carried out chemical reactions in industry, there is currently no amidation protocol that is regarded as safe, high yielding, environmentally friendly and inexpensive. The direct amidation of a carboxylic acid with an amine is viewed as an inherently good solution for developing such a protocol. Since the 1960s, there has been a gradual development in the use of silicon reagents for direct amidation. This review covers the methods published to April 2021 for silicon reagent mediated direct amidation of a carboxylic acid with an amine. This review also covers the use of polymeric SiO2 to promote direct amidation.
1. Introduction
The ubiquity of amide bonds cannot be overestimated.1 Their key role in the function of life, along with the estimation that amide bond formation accounts for 25% of all reactions carried out in drug discovery and development,2 makes amides one of the most important functional groups known to chemists. Accordingly many efficient and inventive protocols have been developed to form this bond that have been reviewed many times.3–10 Notwithstanding this state of affairs, it is surprising that amidation is still a reaction considered non-ideal, as outlined by the 2007 ACS Green Chemistry Institute (GCI) Pharmaceutical Roundtable, which voted that an aim to develop amide formation avoiding poor atom economy reagents should be the top priority for the chemical research community.11 In 2018, the ACS GCI Pharmaceutical Roundtable redefined the goal to develop general methods for catalytic/sustainable (direct) amide or peptide formation, reflecting the unsolved nature of sustainable amidation.12 Accordingly, much current interest is directed at the development of direct amidations of carboxylic acids with amines.13–17
A further challenge to amidation, other than the environmental impact, is scope, where the ease of direct amidation between various carboxylic acids and amines varies considerably. Ideally, a given amidation protocol, whether catalytic or stoichiometric, should be applicable to couple a wide variety of acid and amine substrates.
Over the past 50 years, various silicon reagents have been reported for direct amidations. This review summarises these protocols in terms of scope – including peptide bond formation. Heterogenous silicon protocols utilizing silica as a catalyst for direct amidation, are also described. The coupling reagents are grouped according to the leaving group at silicon, starting with those with Si–Cl bonds as these were the first type to be developed for amidation, and progressing through those with Si–H, Si–N and Si–O bonds. In the case of HSi(OCH(CF3)2)3 with two different reactive Si–X bonds it is grouped with the silanes on the basis of its reactivity. A table of comparison of the reagents (including hazards) is also presented in the review with the aim of being a quick referral tool for the reader.
2. Reagents with Si–Cl bonds
2.1. Tetrachlorosilane
The first reported use of a stoichiometric silicon-based reagent (SR) for direct amidation of a carboxylic acid with an amine was in 1969.18 Chan et al. demonstrated that the use of silicon tetrachloride (SR1) as the reagent in pyridine as the solvent (and also as an acid scavenger) provided amide products (Scheme 1A). A key benefit of this protocol is that the chlorosilane SR1 is destroyed in the work-up and converted into silica, which is easily removed.
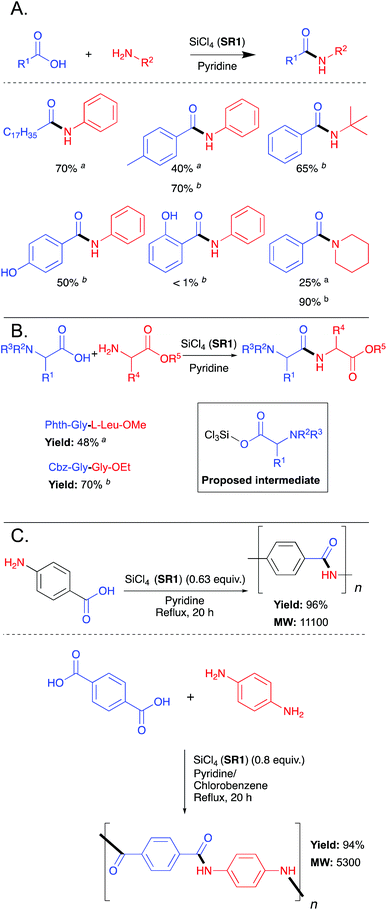 |
| Scheme 1 (A) Selected examples of SiCl4 (SR1) mediated amide bond formation.18 Reaction conditions: a25 °C, 10 h; breflux, 1 h. (B) Selected examples of SiCl4 mediated formation of dipeptides.19 Reaction conditions: a110 °C, 30 min; b60 °C, 85 min. (C) SiCl4 mediated polymerisations.20 See Table 1, entry 1 for full substrate scope. | |
Table 1 Comparison of substrate scope, cost and hazard codes for stoichiometric silicon reagents
|
|
|
|
Aliphatic carboxylic acid+ |
Aromatic Carboxylic acid+ |
Aniline+ |
|
|
|
Entry |
Silicon reagent |
Presynthesis required? |
Acid : amine : silicon ratio [solvent] |
1° amine |
Cyclic 2° amine |
Acyclic 2° amine |
1° amine |
Cyclic 2° amine |
Acyclic 2° amine |
RCO2H |
ArCO2H |
Peptide |
Pricea |
Hazard statementb |
Prices from Sigma https://www.sigmaaldrich.com/united-kingdom.html (accessed 11/05/2021) based on the largest available pack size.
H225 – Highly flammable liquid and vapour; H226 – Flammable liquid and vapor; H301 – Toxic if swallowed or if inhaled; H302 – Harmful if swallowed; H310 – Fatal if contact with skin; H311 – Toxic in contact with skin; H314 – Causes severe skin burns and eye damage; H315 – Causes skin irritation; H318 – Causes serious eye damage; H319 – Causes serious eye irritation; H330 – Fatal if inhaled; H331 – Toxic if inhaled; H332 – Harmful if swallowed or if inhaled; H335 – May cause respiratory irritation; H412 – Harmful to aquatic life with long lasting effect.
|
118,19 |
|
NO |
1.0 : 1.0 : 0.75 [Pyridine] |
ND |
ND |
ND |
60% (25 °C) |
90% (115 °C) |
ND |
70% (115 °C) |
70% (115 °C) |
Range of amino acids (protected) (60 °C – reflux) |
£0.11 per g |
H301, H331, H314, H335 |
221,22 |
|
NO |
1.0 : 1.1 : 3.0 [Pyridine] |
ND |
ND |
ND |
ND |
ND |
ND |
ND |
ND |
Range of amino acids (unprotected) (rt) |
£0.06 per mL |
H225, H302, H314, H331 |
323 |
MePhSiCl2SR3 |
NO |
1.1 : 1.1 : 1.0 + additive [THF] |
26% (rt −60 °C) |
ND |
ND |
ND |
ND |
ND |
ND |
ND |
ND |
£0.62 per mL |
H314 |
423 |
Ph2SiCl2SR4 |
NO |
1.1 : 1.1 : 1.0 + additive [THF] |
75% (rt −60 °C) |
ND |
ND |
ND |
ND |
ND |
ND |
ND |
ND |
£0.69 per mL |
H310, H314 |
523 |
|
YES |
1.1 : 1.1 : 1.0 + additives [THF] |
99% (rt −60 °C) |
96% (rt – 60 °C) |
84% (rt – 60 °C) |
ND |
96% (rt – 60 °C) |
ND |
ND |
ND |
Range of amino acids (protected) (rt −60 °C) |
NA |
NA |
623 |
|
YES |
1.1 : 1.1 : 1.0 + additives [THF] |
ND |
ND |
ND |
ND |
ND |
ND |
ND |
ND |
Small range of amino acids (protected) (rt −60 °C) |
NA |
NA |
723 |
|
YES |
1.1 : 1.1 : 1.0 + additives [THF] |
ND |
ND |
ND |
ND |
ND |
ND |
ND |
ND |
Range of amino acids (protected) (rt −60 °C) |
NA |
NA |
823 |
|
YES |
1.1 : 1.1 : 1.0 + additives [THF] |
ND |
ND |
ND |
ND |
ND |
ND |
ND |
ND |
Small range of amino acids (protected) (rt −60 °C) |
NA |
NA |
923 |
|
YES |
1.1 : 1.1 : 1.0 + additives [THF] |
ND |
ND |
ND |
ND |
ND |
ND |
ND |
ND |
Small range of amino acids (protected) (rt −60 °C) |
NA |
NA |
1023 |
|
YES |
1.1 : 1.1 : 1.0 + additives [THF] |
ND |
ND |
ND |
ND |
ND |
ND |
ND |
ND |
Small Range of amino acids (protected) (rt −60 °C) |
NA |
NA |
1125 |
|
NO |
1.0 : 1.0 : 3.0 [DMF] |
88% (rt) |
89% (rt) |
94% (rt) |
85% (rt) |
98% (rt) |
0% (rt) |
ND |
0% (rt) |
Limited range (protected) |
£5.16 per g |
H225, H302, H332, H315, H319, H335 |
1226 |
|
1.0 : 1.0 : 1.5 [MeCN/CH2Cl2] |
ND |
99% (20 °C) |
Weinreb amide – 74% (20 °C) |
ND |
93% (20 °C) |
Weinreb amide –86% (20 °C) |
61% (20 °C) |
ND |
Range of amino acids (protected) |
£5.16 per g |
|
1331 |
|
NO |
1.0 : 1.0 : 1.0 [MeCN] (some require additives) |
80% (80 °C) |
88% (80 °C) |
94% (80 °C) |
74% (80 °C) |
67% (80 °C) |
ND (80 °C) |
46% (80 °C) |
ND |
Range of amino acid (protected) (+additives) |
£3.01 per g |
H315 |
1433 |
|
1.0 : 1.0 : 1.0 + additives [MeCN] |
91% (100 °C) |
98% (100 °C) |
53% (100 °C) |
87% (100 °C) |
ND |
ND |
28% (100 °C) |
ND |
Single example (protected amino acid) (100 °C) |
£3.01 per g |
H315 |
1531 |
Me2PhSiH SR13 |
NO |
1.0 : 1.0 : 1.0 [MeCN] |
32% (80 °C) |
ND |
ND |
ND |
ND |
ND |
ND |
ND |
ND |
£2.04 per g |
H226, H318 |
1631 |
Ph3SiH SR14 |
NO |
1.0 : 1.0 : 1.0 [MeCN] |
50% (80 °C) |
ND |
ND |
ND |
ND |
ND |
ND |
ND |
ND |
£2.18 per g |
H315, H319 |
1731 |
Et2SiH2SR15 |
NO |
1.0 : 1.0 : 1.0 [MeCN] |
14% (80 °C) |
ND |
ND |
ND |
ND |
ND |
ND |
ND |
ND |
£8.12 per mL |
H225 |
1831 |
MePhSiH2SR16 |
NO |
1.0 : 1.0 : 1.0 [MeCN] |
76% (80 °C) |
ND |
ND |
ND |
ND |
ND |
ND |
ND |
ND |
£5.52 per mL |
H226, H315, H319, H335 |
1935,36 |
|
YES |
1.0 : 1.0 : 1.0 (with catalyst) CH2Cl2 |
>99% (with catalyst) (rt) |
ND |
ND |
96% (with catalyst) (rt) |
ND |
ND |
ND |
ND |
Range of amino acids (protected,35 unprotected36) |
NA |
NA |
2039 |
|
NO |
1.0 : 1.0 : 1.0/2.0 [No solvent] |
85% (110 °C) |
83% (110 °C) |
ND |
ND |
83% (110 °C) |
ND |
ND |
ND |
Single example (protected amino acid) (110 °C) |
£0.14 per mL |
H225, H302, H332, H311, H412 |
2140 |
|
YES |
1.0 : 1.2 : 1.2 [THF] |
88% (rt) |
96% (rt) |
96% (rt) |
71% (rt) |
82% (rt) |
ND |
53% (50 °C) |
ND |
ND |
NA |
NA |
2240 |
|
YES |
1.0 : 1.2 : 0.6 [THF] |
90% (rt) |
96% (rt) |
92% (rt) |
76% (rt) |
87% (rt) |
ND |
71% (50 °C) |
ND |
ND |
NA |
NA |
2341 |
|
YES |
1.2 : 1.0 : 0.6 [THF] |
98% (rt) |
97% (rt) |
93% (rt) |
84% (rt) |
85% (rt) |
ND |
86% (rt) |
ND |
ND |
NA |
NA |
2442 |
|
YES |
1.0 : 1.2 : 1.0 [THF] |
92% (rt) |
92% (rt) |
83% (rt) |
72% (rt) |
ND |
ND |
97% (rt – 50 °C) |
81% (rt) |
ND |
NA |
NA |
2544 |
|
NO |
1.0 : 1.0 : 2.0 [Toluene] |
100% (reflux) |
98% (reflux) |
81% (reflux) |
98% (reflux) |
98% (reflux) |
88% (reflux) |
84% (reflux) |
100% (reflux) |
Single example (reflux) |
£0.34 per g |
H226, H315, H318, H330 |
2643 |
|
1.0 : 1.0 : 1.0 [MeCN] |
22% (110 °C) |
ND |
ND |
ND |
ND |
ND |
ND |
ND |
ND |
|
|
2742 |
|
1.0 : 1.2 : 1.0 [THF] |
9% (rt) |
ND |
ND |
ND |
ND |
ND |
ND |
ND |
ND |
|
|
2842 |
|
NO |
1.0 : 1.2 : 1.0 [THF] |
8% (rt) |
ND |
ND |
ND |
ND |
ND |
ND |
ND |
ND |
£0.08 per mL |
H226, H319, H332, H335 |
2944 |
|
1.0 : 1.0 : 2.0 [Toluene] |
85% (110 °C) |
ND |
ND |
ND |
ND |
ND |
ND |
ND |
ND |
|
|
3042 |
Si(OCH2(CF3)4SR25 |
NO |
1.0 : 1.2 : 1.0 [THF] |
99% (rt) |
ND |
69% (rt) |
ND |
ND |
ND |
ND |
ND |
ND |
NA |
NA |
3142 |
Si(OCH2CF2CF2CF3)4SR26 |
NO |
1.0 : 1.2 : 1.0 [THF] |
99% (rt) |
ND |
83% (rt) |
ND |
ND |
ND |
ND |
ND |
ND |
NA |
NA |
3226 |
Me2Si(OAc)2SR27 |
NO |
1.0 : 1.0 [THF] |
ND |
0% (rt) |
ND |
ND |
ND |
ND |
ND |
ND |
ND |
£4.80 per mL |
H314 |
3326 |
MeSi(OAc)3SR28 |
NO |
1.0 : 1.0 [THF] |
ND |
57% (rt) |
ND |
ND |
ND |
ND |
ND |
ND |
ND |
£1.00 per g |
H302, H314 |
3426 |
Si(OAc)4SR29 |
NO |
1.0 : 1.0 [THF] |
99% (rt) |
99% (rt) |
99% (rt) |
ND |
ND |
ND |
ND |
ND |
ND |
£1.89 per g |
H314 |
In 1971, Chan et al. attempted to expand the scope of this method by applying it to the synthesis of dipeptides.19 However, initial attempts to couple an N-protected amino acid directly with an amino ester failed, where the N-protected amino acid was completely recovered while the amino ester underwent polymerisation. Chan et al. proposed that a silylester would be the reactive intermediate and could be formed in situ by initial addition of the N-protected amino acid followed by the addition of the amino ester, thus preventing undesirable polymerisation. Accordingly, a series of dipeptides (Scheme 1B) were made by allowing N-protected amino acids to react with SiCl4 prior to addition of an amino ester.19 It was noted that tetrabenzyloxy silane was a by-product with Cbz protected compounds but that other protecting groups such as phthaloyl (Phth), benzoyl (Bz) and acetyl (Ac) were tolerated. Significant racemisation was found by analysing the optical rotation of the peptide products. From the reaction of Bz-L-Leu with Gly-OEt, the optically inactive 4-isobutyl-2-phenyloxazolone was isolated and was proposed as the mode of racemisation.
In 1985, Heitz et al. showed that aromatic polyamides could be prepared via direct amidation polycondensation reactions mediated by tetrachlorosilane (SR1), and to date these constitute the only reported examples utilizing a silicon-based reagent for polyamide formation (Scheme 1C).20
2.2. Alkylchlorosilanes
In 2002, Leeuwen et al. demonstrated that dimethyldichlorosilane (SR2) could be utilised in a simultaneous protection-activation strategy for the direct amidation of unprotected amino acids through the presumed intermediacy of a cyclic silane (Scheme 2A).21 Pyridine was again employed as the solvent and as an acid scavenger, and the use of primary amines with the amino group attached to a primary carbon atom were required for good yield. This dichlorodialkylsilane-mediated amidation strategy was also applicable to N-alkylamino acids, β-amino acids and also for the regioselective direct amidation of aspartic acid (Scheme 2B). In 2015 Fedorov et al. extended the scope to include 2° cyclic amines as nucleophiles.22
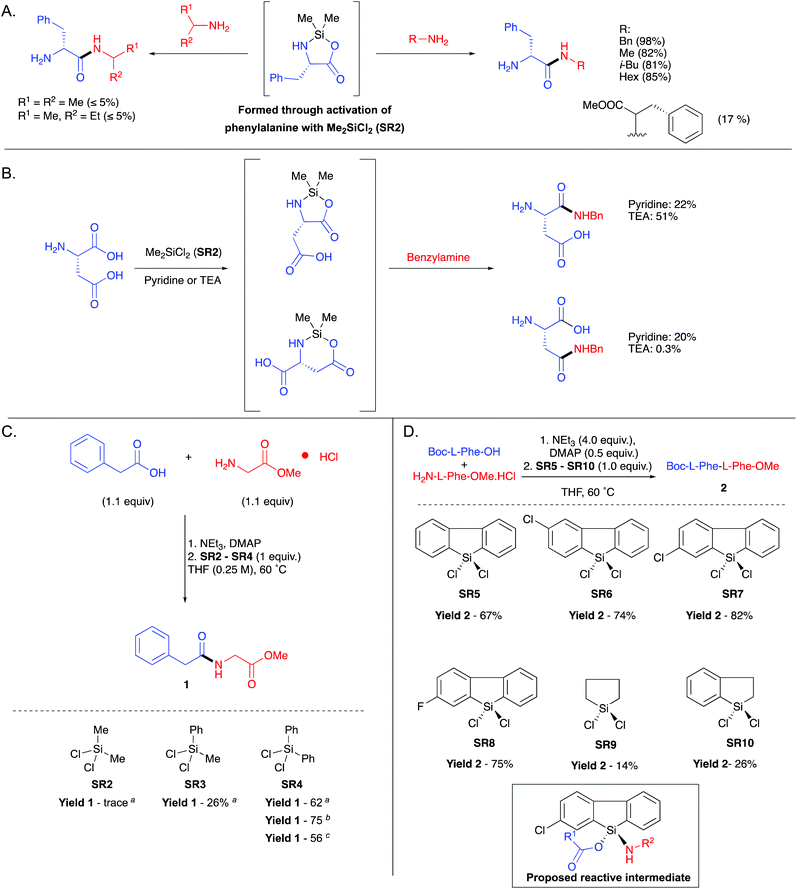 |
| Scheme 2 (A) Selected examples of Me2SiCl2 (SR2) mediated amide formation.21 See Table 1, entry 2 for full substrate scope. (B) Regioselective direct amidation of aspartic acid with Me2SiCl2. (C) Screen of silicon chloride reagents SR2–SR4 for the formation of 1; a5 equivalents of NEt3; b5 equivalents of NEt3 and 0.5 equivalents of DMAP; c5 equivalents of NEt3 and 1 equivalent of DMAP.23 (D) Screen of 9-silafluorenyl and related chloride species SR5–SR10 for optimum amidation protocol. See Table 1, entries 5–10 for full substrate scope. | |
2.3. Arylchlorosilanes
In 2016, Charette et al. reported conformationally flat and rigid 9-silafluorenyl dichlorides (SR5–SR10) (Scheme 2D) as superior reagents to phenyltrichlorosilane (SR4) for the direct amidation of N-Boc protected amino acids with amino esters (Scheme 2C).23 Notably, no epimerisation was observed in these amidations, and the method could be extended to the formation of tripeptides. A ligation mechanism is proposed. Charette et al. provided experimental evidence for the mechanism of their protocol, with a computational study being carried out by Jiang et al.24 The coupling competency of SR7 was demonstrated over range of different amino acid substrates.
3. Reagents with Si–H bonds
3.1. Phenylsilane
In 2006, Ruan et al. were the first to demonstrate the use of PhSiH3 (SR11) as a coupling reagent for direct amide bond formation (Scheme 3A).25 This protocol was also successful for the synthesis of solid supported amides (Scheme 3B).
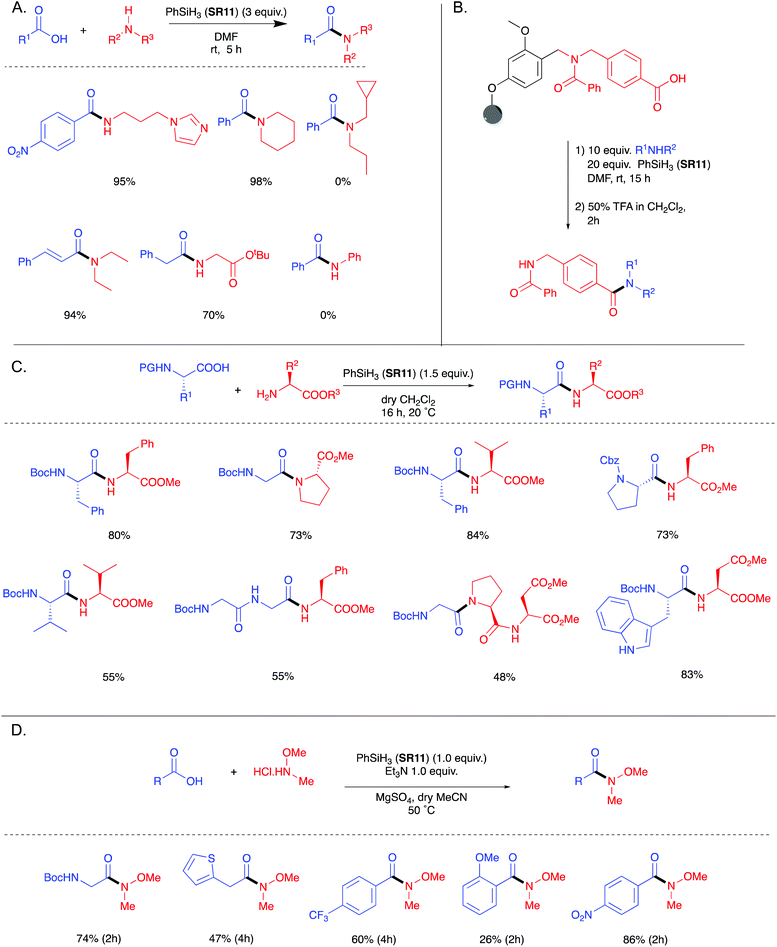 |
| Scheme 3 (A) General scheme for PhSiH3 (SR11) mediated amide bond formation and selected examples showing the scope of the reaction.25 (B) PhSiH3 mediated amidation and subsequent acid-mediated cleavage of a solid supported acid.25 (C) Selected examples showing the scope of peptide bond formation using PhSiH3.26 (D) Formation of various Weinreb amides via PhSiH3 mediated amidation.26 See Table 1, entries 11 and 12 for full substrate scope. | |
In 2020 Blanchet et al. re-examined the use of PhSiH3 (SR11) and confirmed its utility for the formation of amides when using acetonitrile as the reaction solvent.26 In contrast to the work by Ruan et al. which employed 3 equivalents of silane SR11, it was found that the use of 1.5 equivalents of the silane was optimal.
The scope of this protocol using PhSiH3 was expanded to form Cbz-, Boc- and Fmoc-protected dipeptides and tripeptides without any detectable epimerisation (Scheme 3C). Weinreb amide formation was also reported (Scheme 3D).
As silanes have precedent27 for the chemoselective reduction of phosphine oxides, a catalytic Staudinger ligation (Scheme 4) was developed in which phenylsilane (SR11) was employed as the stoichiometric reductant.28 This catalytic Staudinger ligation was competent for the formation of amides, as well as lactams and peptides, notably without racemisation. Denton demonstrated that the silane cannot be simply regarded as an innocent terminal reductant in these processes,29 and subsequently White and Mecinović provided further mechanistic insight.30
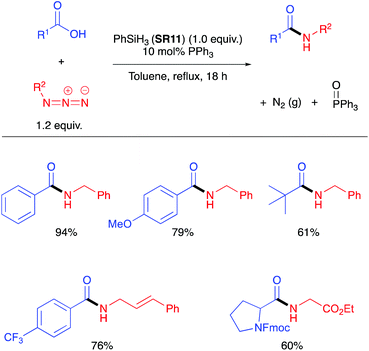 |
| Scheme 4 Catalytic Staudinger ligation to form amides with selected examples of scope of the reaction.28 | |
3.2. Diphenylsilane
In 2017, Charette et al. reported the use of various aryl and alkyl silanes as reagents for direct amidation, with diphenylsilane (SR12) being the most effective under the conditions examined.31 Charette found that SR12 was a superior coupling reagent to Me2PhSiH (SR13), Ph3SiH (SR14), Et2SiH2 (SR15) and MePhSiH2 (SR16).
Successful direct amidation with SR12 was demonstrated for various combinations of aromatic and aliphatic carboxylic acids with primary and secondary amines (Scheme 5). Dipeptides, tripeptides and lactam of various ring sizes were also successfully formed. The stoichiometric by-products of these amidations are reported as hydrogen gas and an insoluble siloxane, which facilitates product isolation. Recent computational studies on the system suggest that these reactions proceed via nucleophilic attack of the amine on an acyloxysilane formed from the silane and the carboxylic acid with liberation of hydrogen.32 Very recently, Adler et al. have reported the beneficial effect of added N-methylpyrrolidine for Ph2SiH2 mediated direct amidations,33 as well as a Glorius robustness study for this protocol.34
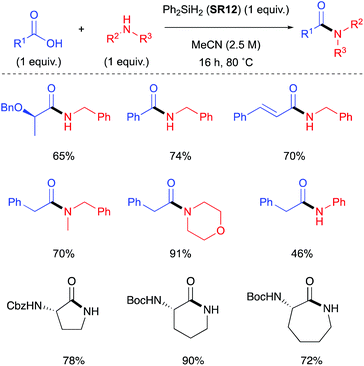 |
| Scheme 5 Selected examples of amides and lactams formed using Ph2SiH2 (SR12) mediated amidation.31 See Table 1, entry 13 for full substrate scope. | |
3.3. HSi(OCH(CF3)2)3
In 2020 Muramatsu and Yamamoto published a direct amidation protocol utilizing HSi(OCH(CF3)2)3 (SR17) as a coupling reagent35 for the epimerisation-free formation of dipeptides under ambient conditions (Scheme 6A). As for the other silanes described above, hydrogen gas and a siloxane are generated as the stoichiometric by-products. 29Si NMR studies are reported to elucidate the mechanistic course of the reaction (Scheme 6B). The use of catalytic quantities of aminosilane 8 allowed the further development of a high yielding reaction protocol using the ideal 1
:
1
:
1 quantities of acid:amine:reagent (Scheme 7). It is important to note that although the protocol has a catalytic aspect, a stoichiometric coupling reagent is still required to promote the bond formation.
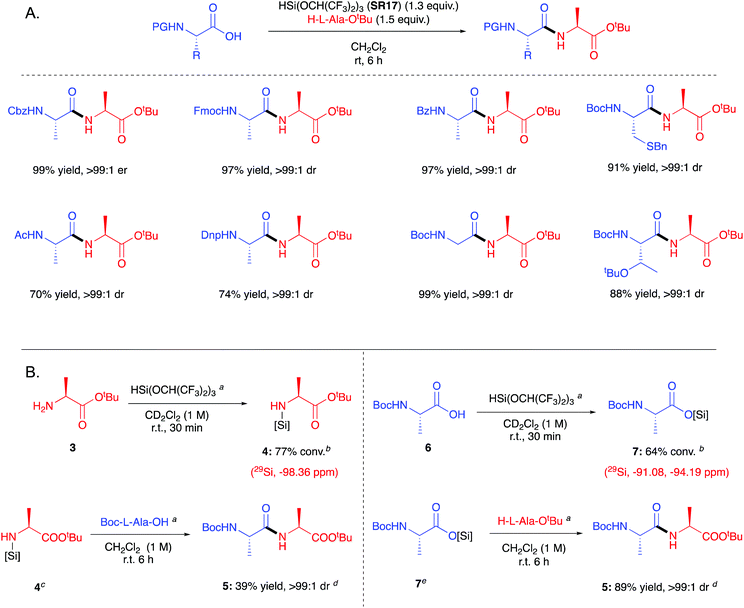 |
| Scheme 6 (A) Example of scope of peptides formed with HSi(OCH(CF3)2)3 (SR17).35 See Table 1, entry 19 for full substrate scope. (B) Example of mechanistic studies carried out by Yamamoto.35 [Si] = Si(OCH(CF3)2)3; a1 equivalent added; bconversion determined by 1H NMR spectroscopy; c4 was formed in situ by reaction of 3 with HSi(OCH(CF3)2)3 in CH2Cl2 for 1 h (rt); ddrs determined by 1H NMR spectroscopy; e7 was formed in situ by reaction of 6 with HSi(OCH(CF3)2)3 in CH2Cl2 for 12 h (rt).35 | |
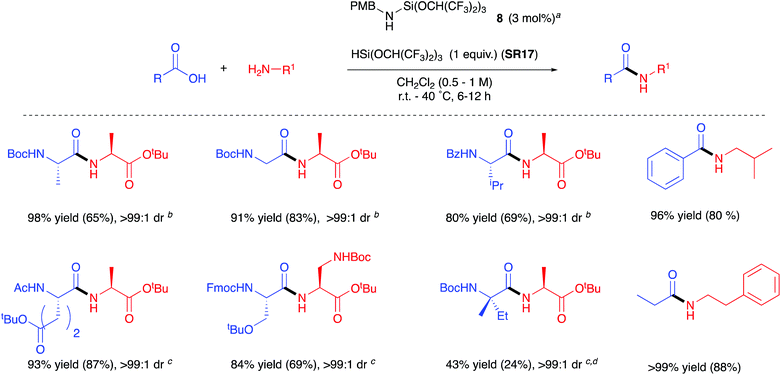 |
| Scheme 7 Scope of dipeptides formed using Yamamoto's catalytic amidation protocol.35 Yield in parenthesis is for when aminosilane catalyst is not added. aSilylamine formed in situ from silylation of p-methoxybenzylamine with HSi(OCH(CF3)2)3 (1 equiv.); bdrs provided by HPLC; cdrs provided by 1H NMR spectroscopy; dHSi(OCH2CF3)3 used instead of HSi(OCH(CF3)2)3. See Table 1, entry 19 for full substrate scope. | |
Yamamoto et al. have since extended their protocol utilizing SR17 in combination with N-(tert-butyldimethylsilyl)-N-methyltrifluoroacetamide (MTBSTFA) for the one-pot formation of dipeptides between unprotected amino acids.36
3.4. (EtO)2MeSiH
In 2014, Mecinović et al. reported the use of (EtO)2MeSiH as a stoichiometric reducing agent for PPh3 catalysed direct amidation of unactivated carboxylic acids and amines.37
3.5. Poly(methylhydroxysilane)
In 2017, Mecinović et al. published on poly(methylhydroxysilane) as a superstoichiometric reducing agent in an organophosphorus catalysed direct amidation between a carboxylic acid and an amine.38 A silyl ester intermediate was not detected as a reactive intermediate, and it was concluded that the silane's role is purely as a reductant.
4. Reagents with Si–N bonds
4.1. Hexamethyldisilazane
In 1999 Chou et al. demonstrated that the use of neat HMDS (SR18) coupled carboxylic acids and piperazine (Scheme 8A).39 A base wash and subsequent chromatography resulted in pure amide products. Direct amidation of N-Boc-Ala-OH with piperazine was also demonstrated, albeit with partial epimerisation. The intermedicacy of a silyl ester was implicated by stepwise heating of an acid with HMDS followed by addition of an amine (Scheme 8B).
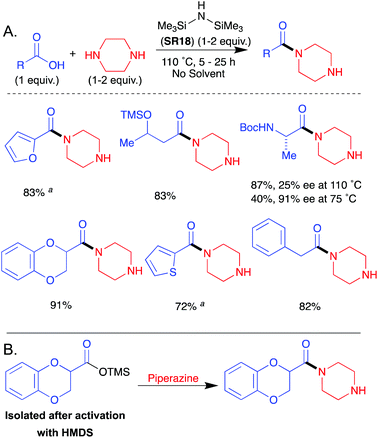 |
| Scheme 8 (A) Representative scope of (Me3Si)2NH (SR18) mediated amidation reaction.39a5–9% diamide formation was observed. (B) Formation of a silylester followed by amide formation. See Table 1, entry 20 for full substrate scope. | |
4.2. Imidazolesilanes
In 2005, Mukaiyama et al. demonstrated that imidazole-1-ylsilanes SR19 and SR20 (Scheme 9) – prepared by trans-silylation of silylchlorides with silylimidazoles – were efficient stoichiometric coupling reagents for direct amidation at ambient temperature.40
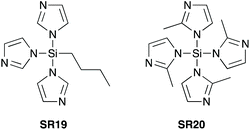 |
| Scheme 9 Silylimidazoles prepared from trimethylsilylimidazole precursors.40 | |
The work-up protocol post-amidation uses hydrolysis of the silane and subsequent polycondensation to provide an insoluble siloxane which could be readily removed by filtration and extraction with EtOAc (this is only applicable to Method B) (Scheme 10).
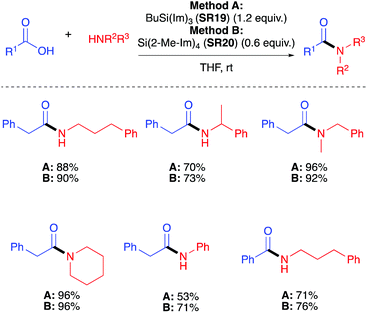 |
| Scheme 10 Selected amide products by direct amidation using BuSi(Im)3 (SR19) and Si(2-Me-Im)4 (SR20).40 See Table 1, entries 21 and 22 for full substrate scope. | |
5. Reagents with Si–O bonds
5.1. Tetrakis(pyridine-2-yloxy)silane
In a second report in 2005, Mukaiyama et al. demonstrated the use of tetrakis(pyridine-2-yloxy) silane (SR21) as a reagent for direct amidation to give amides in high yield at room temperature.41 The amides were delivered pure after sequential filtration, and acid–base washes (Scheme 11). As for the synthesis of imidazol-1-ylsilanes,40 tetrakis(pyridine-2-yloxy)silane (SR21) was prepared by trans-silylation.
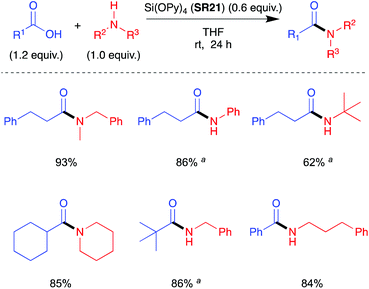 |
| Scheme 11 Selected amide products by direct amidation using Si(OPy)4 (SR21); a1.5 equiv. of carboxylic acid was used. See Table 1, entry 23 for full substrate scope.41 | |
5.2. Tetrakis(1,1,1,3,3,3-hexafluoro-2-propoxy)silane
In a third communication in 2005,42 Mukaiyama et al. screened (Scheme 12) various alkoxy silanes for their amide coupling abilities, and identified tetrakis(1,1,1,3,3,3-hexafluoro-2-propoxy)silane (SR22) as the most competent coupling reagent for direct amide bond formation at room temperature. The incorporation of fluorine substituents was found to be beneficial compared to alkoxy groups, and the branched nature of the hexafluoroisopropanol grouping prevented competitive ester formation. Pure amides were obtained after a simple acid–base wash regime. The reagent was reported to be prepared in a glove box.
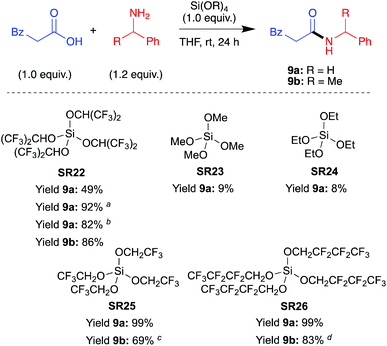 |
| Scheme 12 Screen of various alkoxysilanes SR22–26 for amidation.42aSi[OCH(CF3)2]4 (0.5 equiv.) was used; bSi[OCH(CF3)2]4 (0.25 equiv.) was used; c2,2,2-Trifluoroethyl ester was afforded as by-product (23% yield); d2,2,3,3,4,4,4-Heptafluorobutyl ester was afforded as a by-product (8% yield). See Table 1, entries 24, 27, 28, 30 and 31 for full substrate scope. | |
5.3. Tetramethyl orthosilicate
Mukaiyama found that simple alkoxy silanes such as tetramethyl orthosilicate (TMOS – CAUTION: fatal if inhaled, GHS: H330 – SR23) and tetraethyl orthosilicate (TEOS – SR24) gave only low conversion when used as stoichiometric coupling reagents for amidation at room temperature in THF.42 Sheppard also found that SR23 gave low conversion when used as a stoichiometric coupling reagent in refluxing acetonitrile.43 However, Braddock et al. successfully developed a TMOS based protocol in refluxing toluene to afford high yields of pure amide products from aliphatic and aromatic carboxylic acids with primary, cyclic & acyclic secondary amines and anilines as well as other challenging amidations (Scheme 13).44 A work-up procedure based on annihilation of the silicon reagent provided the pure products without the need for chromatographic purification. A one mole scale amidation was demonstrated with competitive process mass intensity, and a putative reactive silyl ester intermediated was observed in situ by 1H, 13C and 29Si NMR methods. TMOS (SR23) has since been demonstrated successfully for the direct amidation of carboxylic acids with pentafluorosulfanyl aniline.45
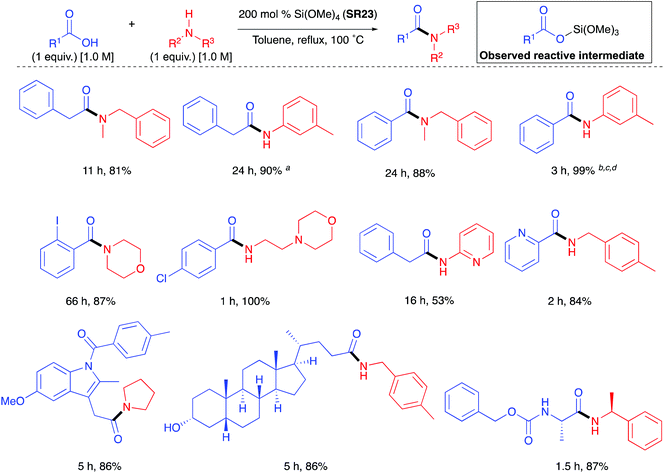 |
| Scheme 13 Selected examples of amides formed via TMOS (SR23) mediated coupling.44a[acid] = 0.5 M, [amine] = 0.5 M; b250 mol% TMOS, N2, [acid] = 2.0 M, [amine] = 2.0 M; cWith 4 Å molecular sieves suspended in the head space; d2 equiv. of BzOH. See Table 1, entry 25 for full substrate scope. | |
5.4. Silicon tetraacetate
Blanchet et al. screened a series of commercially available silyl acetates: Me2Si(OAc)2 (SR27), MeSi(OAc)3 (SR28), Si(OAc)4 (SR29), to determine their ability to acetylate morpholine stoichiometrically.26 The results showed that the Si(OAc)4 provided complete conversion to 4-acetylmorpholine after only 10 minutes at 0 °C in tetrahydrofuran solvent. However, after 5 h MeSi(OAc)3 provided only 57% yield and Me2Si(OAc)2 gave no conversion. It was found that Si(OAc)4 was able to acetylate a range of amines (Scheme 14). Chan had previously shown that Si(OAc)4 (SR29) was competent at acylating aniline when the reaction was carried out in refluxing toluene.19
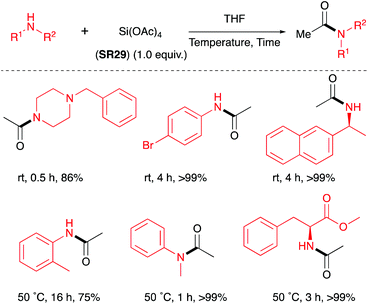 |
| Scheme 14 Selected examples of amides formed by acetylation using Si(OAc)4 (SR29).26 See Table 1, entry 34 for full substrate scope. | |
6. Heterogenous silicon reagents
In addition to the homogenous, stoichiometric silicon reagents described above there are several reports of the use of heterogenous silicas to promote effective amidation. These studies concluded that silanol groups on the surface of silicas promote the adsorption of amine and acid substrates and enable direct amidation, often with high yields.46
Clark et al. discovered that (Kieselgel 60, K60) could be used to promote formation of a range of alkyl and aryl amides but that pre-drying of the silica at 700 °C, was required (Scheme 15).47 Silica gel (K60) has also been used by Zhang et al. as an effective coupling catalyst to provide a range of different amides without requiring the pre-treatment of the silica.47,48 Gamba-Sánchez et al. applied microwaves to promote amidations without the requirement for pre-treatment of the silica.49 They found that high selectivity could be achieved with carboxylic acids providing higher yields with primary amines over cyclic secondary and aromatic amines, and also showing preference for the reaction of the amine functionality in binucleophilic substrates, such as ethanolamine.49
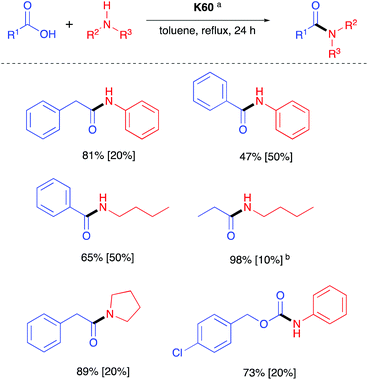 |
| Scheme 15 Selected examples of amides formed via K60 mediated coupling.47 Catalyst loading in square-brackets. aActivated by heating at 700 °C. bIsolated after 12 h. | |
Other mesoporous silicas, such as the more expensive MCM-41, have been shown by Komura et al. to promote amidation of fatty acids and long chain amines.50 The MCM-41 promoted a greater conversion of palmitic acid and n-hexylamine in refluxing toluene than either the activated47 or non-activated48 silica, with no loss in catalytic activity when recycled.50 Komura also reported the use of mesoporous silica SBA-15 as a heterogenous catalyst.51 Work by Fontaine et al. improved the catalytic activity of SBA-15 by enriching the material with Lewis acidic metals such as Ti and Zr.52 When the surface silanols of the catalyst are protected with tetramethyldisilazane (TMDS) the catalytic activity drops to 9%, consistent with the silanol groups being required for catalytic activity. SBA-15 silica with surface aminopropyl groups was also found to act as formylating agent for a series of amines and alcohols.53 Another mesoporous silica, OSU-6, has been shown to catalyse amidation for a range of substrates when a neat mixture of acid and amine are mixed with the catalyst in a heated sealed pressure tube.54
The (K60) silica47,48 utilized by Clark and Zhang is significantly cheaper than OSU-6, MCM-41, and SBA-15, but the more expensive silicas do not require pre-activation.
7. Conclusion
It is not surprising, that considering the worldwide demand for amides, the expectations for novel reagents for direct amidation of carboxylic acids are high. A chemist developing a novel coupling reagent with the aim of industrial implementation will need to ensure that their protocol is high yielding for a wide scope of reactants, is environmentally friendly, safe to use, and commercially available and affordable. This review shows that the silicon reagents presented above are competent at promoting amide bond formation by direct amidation of carboxylic acids with varied substrate scope. However, there is still need for improvement. A combination of broad scope, safety, environmental impact and affordability has not yet been achieved by any one of the reagents presented in this review. In terms of safety, many of the compounds (TMOS, SiCl4 and PhSiH3) display hazard symbols that make them uninviting to the industrial chemist. Many of the protocols presented still utilize a chromatographic purification of the amide product which does not meet the demands for an environmentally friendly amidation. The beneficial properties of several of the silicon reagents that allows for the simple conversion to insoluble siloxanes and subsequent removal by filtration, or annihilation by aqueous work-up, should be pursued and exploited with the aim of reducing process mass intensities. Notably, the majority of reagents presented are commercially available making them attractive to the synthetic community. However, the search remains for a silicon-based reagent that meets all these highly demanding criteria.55 Moreover, the advent of a silicon-based catalyst for direct amidation would be a further advance and is overdue.
Conflicts of interest
There are no conflicts to declare.
Acknowledgements
We thank the EPSRC Centre for Doctoral Training in Next Generation Synthesis and Reaction Technology (Imperial College London, EP/S023232/1) for a studentship to J. J. D.
Notes and references
-
A. Greenberg, C. M. Breneman and J. F. Liebm, The amide linkage: selected structural aspects in chemistry, biochemistry, and materials science, Wiley-Interscience, New York, 2002 Search PubMed.
- J. Boström, D. G. Brown, R. J. Young and G. M. Keserü, Nat. Rev. Drug Discovery, 2018, 17, 709–727 CrossRef PubMed.
- E. Valeur and M. Bradley, Chem. Soc. Rev., 2009, 38, 606–631 RSC.
- V. R. Pattabiraman and J. W. Bode, Nature, 2011, 480, 471–479 CrossRef CAS PubMed.
- J. R. Dunetz, J. Magano and G. A. Weisenburger, Org. Process Res. Dev., 2016, 20, 140–177 CrossRef CAS.
- A. Ojeda-Porras and D. Gamba-Sánchez, J. Org. Chem., 2016, 81, 11548–11555 CrossRef CAS PubMed.
- R. M. De Figueiredo, J. S. Suppo and J. M. Campagne, Chem. Rev., 2016, 116, 12029–12122 CrossRef CAS PubMed.
- E. Massolo, M. Pirola and M. Benaglia, Eur. J. Org. Chem., 2020, 4641–4651 CrossRef CAS.
- A. S. Santos, A. M. S. Silva and M. M. B. Marques, Eur. J. Org. Chem., 2020, 2501–2516 CrossRef CAS.
- M. Todorovic and D. M. Perrin, Pept. Sci., 2020, 112, e24210 CAS.
- D. J. C. Constable, P. J. Dunn, J. D. Hayler, G. R. Humphrey, J. L. Leazer, R. J. Linderman, K. Lorenz, J. Manley, B. A. Pearlman, A. Wells, A. Zaks and T. Y. Zhang, Green Chem., 2007, 9, 411–420 RSC.
- M. C. Bryan, P. J. Dunn, D. Entwistle, F. Gallou, S. G. Koenig, J. D. Hayler, M. R. Hickey, S. Hughes, M. E. Kopach, G. Moine, P. Richardson, F. Roschangar, A. Steven and F. J. Weiberth, Green Chem., 2018, 20, 5082–5103 RSC.
- H. Charville, D. Jackson, G. Hodges and A. Whiting, Chem. Commun., 2010, 46, 1813–1823 RSC.
- R. M. Lanigan and T. D. Sheppard, Eur. J. Org. Chem., 2013, 7453–7465 CrossRef CAS.
- H. Lundberg, F. Tinnis, N. Selander and H. Adolfsson, Chem. Soc. Rev., 2014, 43, 2714–2742 RSC.
- X. Wang, Nat. Catal., 2019, 2, 98–102 CrossRef.
- M. T. Sabatini, L. T. Boulton, H. F. Sneddon and T. D. Sheppard, Nat. Catal., 2019, 2, 10–17 CrossRef CAS.
- T.-H. Chan and L. T. L. Wong, J. Org. Chem., 1969, 34, 2766–2767 CrossRef CAS.
- T.-H. Chan and L. T. L. Wong, J. Org. Chem., 1971, 36, 850–853 CrossRef CAS.
- P. Strohriegl, W. Heitz and G. Weber, Makromol. Chem., Rapid Commun., 1985, 6, 111–118 CrossRef CAS.
- S. H. Van Leeuwen, P. J. L. M. Quaedflieg, Q. B. Broxterman and R. M. J. Liskamp, Tetrahedron Lett., 2002, 43, 9203–9207 CrossRef CAS.
- A. N. Balaev, V. N. Osipov, K. A. Okhmanovich and V. E. Fedorov, Pharm. Chem. J., 2015, 49, 334–339 CrossRef CAS.
- S. J. Aspin, S. Taillemaud, P. Cyr and A. B. Charette, Angew. Chem., Int. Ed., 2016, 55, 13833–13837 CrossRef CAS PubMed.
- Y.-Y. Jiang, L. Zhu, Y. Liang, X. Man and S. Bi, J. Org. Chem., 2017, 82, 9087–9096 CrossRef CAS PubMed.
- Z. Ruan, R. M. Lawrence and C. B. Cooper, Tetrahedron Lett., 2006, 47, 7649–7651 CrossRef CAS.
- E. Morisset, A. Chardon, J. Rouden and J. Blanchet, Eur. J. Org. Chem., 2020, 388–392 CrossRef CAS.
- K. L. Marsi, J. Org. Chem., 1974, 39, 265–267 CrossRef.
- A. D. Kosal, E. E. Wilson and B. L. Ashfeld, Angew. Chem., Int. Ed., 2012, 51, 12036–12040 CrossRef CAS PubMed.
- K. G. Andrews and R. M. Denton, Chem. Commun., 2017, 53, 7982–7985 RSC.
- P. B. White, S. J. Rijpkema, R. P. Bunschoten and J. Mecinović, Org. Lett., 2019, 21, 1011–1014 CrossRef CAS PubMed.
- M. Sayes and A. B. Charette, Green Chem., 2017, 19, 5060–5064 RSC.
- B. Hu, Y.-Y. Jiang, P. Liu, R.-X. Zhang, Q. Zhang, T.-T. Liu and S. Bi, Org. Biomol. Chem., 2019, 17, 9232–9242 RSC.
- M. C. D'Amaral, N. Jamkhou and M. J. Adler, Green Chem., 2021, 23, 288–295 RSC.
- K. D. Collins and F. Glorius, Nat. Chem., 2013, 5, 597–601 CrossRef CAS PubMed.
- W. Muramatsu, C. Manthena, E. Nakashima and H. Yamamoto, ACS Catal., 2020, 10, 9594–9603 CrossRef CAS.
- W. Muramatsu and H. Yamamoto, J. Am. Chem. Soc., 2021, 143, 6792–6796 CrossRef CAS PubMed.
- D. C. Lenstra, F. P. J. T. Rutjes and J. Mecinović, Chem. Commun., 2014, 50, 5763 RSC.
- D. F. J. Hamstra, D. C. Lenstra, T. J. Koenders, F. P. J. T. Rutjes and J. Mecinović, Org. Biomol. Chem., 2017, 15, 6426–6432 RSC.
- W. C. Chou, M. C. Chou, Y. Y. Lu and S. F. Chen, Tetrahedron Lett., 1999, 40, 3419–3422 CrossRef CAS.
- T. Tozawa, Y. Yamane and T. Mukaiyama, Chem. Lett., 2005, 34, 734–735 CrossRef CAS.
- T. Tozawa, Y. Yamane and T. Mukaiyama, Chem. Lett., 2005, 34, 1334–1335 CrossRef CAS.
- T. Tozawa, Y. Yamane and T. Mukaiyama, Chem. Lett., 2005, 34, 1586–1587 CrossRef CAS.
- P. Starkov and T. D. Sheppard, Org. Biomol. Chem., 2011, 9, 1320–1323 RSC.
- D. C. Braddock, P. D. Lickiss, B. C. Rowley, D. Pugh, T. Purnomo, G. Santhakumar and S. J. Fussell, Org. Lett., 2018, 20, 950–953 CrossRef CAS PubMed.
- H. G. Hiscocks, D. L. Yit, G. Pascali and A. T. Ung, Monatsh. Chem., 2021, 152, 449–459 CrossRef CAS.
- A. Rimola, M. Fabbiani, M. Sodupe, P. Ugliengo and G. Martra, ACS Catal., 2018, 8, 4558–4568 CrossRef CAS.
- J. W. Comerford, J. H. Clark, D. J. MacQuarrie and S. W. Breeden, Chem. Commun., 2009, 2562–2564 RSC.
- X.-D. Yang, X.-H. Zeng, Y.-H. Zhao, X.-Q. Wang, Z.-Q. Pan, L. Li and H.-B. Zhang, J. Comb. Chem., 2010, 12, 307–310 CrossRef CAS PubMed.
- A. Ojeda-Porras, A. Hernández-Santana and D. Gamba-Sánchez, Green Chem., 2015, 17, 3157–3163 RSC.
- K. Komura, Y. Nakano and M. Koketsu, Green Chem., 2011, 13, 828–831 RSC.
- M. Tamura, D. Murase and K. Komura, Synthesis, 2015, 47, 769–776 CrossRef CAS.
- M. V. Zakharova, F. Kleitz and F. G. Fontaine, Dalton Trans., 2017, 46, 3864–3876 RSC.
- R. Malakooti, S. Sobhani, N. Razavi, S. Shafiei and R. Mokhtari, Collect. Czech. Chem. Commun., 2011, 76, 1979–1990 CrossRef CAS.
- B. Nammalwar, N. P. Muddala, F. M. Watts and R. A. Bunce, Tetrahedron, 2015, 71, 9101–9111 CrossRef CAS.
- For a one-pot, two-step amidation of a carboxylic acid and amine using Ph3SiSH as a stoichiometric coupling reagent see: A. A. Lamar and L. S. Liebeskind, Tetrahedron Lett., 2015, 56, 6034–6037 CrossRef CAS.
|
This journal is © The Royal Society of Chemistry 2021 |
Click here to see how this site uses Cookies. View our privacy policy here.