DOI:
10.1039/D2QM00806H
(Research Article)
Mater. Chem. Front., 2022,
6, 3382-3390
Highly efficient thermally activated delayed fluorescence emitter based on the 5H-benzo[d]benzo[4,5]imidazo[1,2-a]imidazole donor†
Received
10th August 2022
, Accepted 19th September 2022
First published on 21st September 2022
Abstract
In this study, a donor–π-acceptor-type thermally activated delayed fluorescence (TADF) emitter (BzITz) was designed and synthesized using 5H-benzo[d]benzo[4,5]imidazo[1,2-a]imidazole as a fused rigid electron donor, and a benzonitrile merged triazine unit as an electron acceptor. The effects of the fused rigid donor unit on the photophysical and electroluminescence properties of the TADF emitter were thoroughly explored. Compared with a reported emitter based on carbazole in a previous study, photophysical analysis results showed that BzITz exhibited a relatively small singlet–triplet energy splitting, a short delayed fluorescence lifetime, and a high photoluminescence quantum yield. Consequently, an organic light-emitting diode fabricated using the BzITz emitter exhibited an improved device performance with an external quantum efficiency of 24.0%, a current efficiency of 49.4 cd A−1, and a low-efficiency roll-off. These findings show that the use of fused rigid donors can play a critical role in the design of efficient TADF emitters.
Introduction
Compared with traditional display technologies, organic light-emitting diodes (OLEDs) offer unprecedented advantages such as high contrast, lightweight and ultrathin properties, flexibility, wide viewing angles, and low-cost device production. However, designing highly emissive and stable emitters for OLEDs remains a significant challenge.1–7
Ordinary fluorescent materials have been used extensively as conventional OLED emitters, but their device performance cannot exceed the theoretical maximum exciton utilization efficiency of 25% because the yielded ratio of singlet and triplet excitons is 1
:
3 under electrical excitation conditions.8,9 Conversely, because phosphorescent materials exhibit a strong spin–orbit coupling effect, the internal quantum efficiency (IQE) of the organic electroluminescence (EL) of phosphorescent materials can theoretically reach 100%.10,11 Although phosphorescent materials exhibit excellent EL characteristics with unitary IQE and significant external quantum efficiency (EQE), the use of precious rare-earth metals limits their practical applications.5,12–17 Recently, the development of emitters through thermally activated delayed fluorescence (TADF) has attracted significant attention, and TADF materials are termed as third-generation OLED emitters.18–21 These materials are regarded as being highly efficient since they can achieve 100% IQE using triplet excitons through triplet-to-singlet thermal upconversion from the lowest triplet excited state (T1) to the lowest singlet excited state (S1) via reverse intersystem crossing (RISC).18–20,22–24 Luminescent materials with a small S1–T1 energy splitting (ΔEST) can be obtained through a specific molecular design that involves minimal overlap between the highest occupied molecular orbital (HOMO) and the lowest unoccupied molecular orbital (LUMO) because of spatial separation of the electron-donating and electron-withdrawing units, enabling the thermal upconversion of electrons from the triplet to singlet excited states via RISC.25–29 Consequently, TADF materials are being explored intensively for next-generation OLEDs, to overcome current technical limitations such as the device lifetime and color purity. In recent years, many researchers have developed highly efficient TADF materials with enhanced device performances using diverse acceptor and donor moieties.30,31
Donor–π–acceptor structures with twisted geometries have been used extensively to develop TADF materials.32,33 In this framework, donor and acceptor moieties play a key role in determining the TADF properties via management of the HOMO and LUMO, which affects important TADF parameters such as the photoluminescence quantum yield (PLQY), delayed fluorescence lifetime, and ΔEST.18 Several donor and acceptor moieties have been demonstrated as efficient and RISC-enhancing building blocks of TADF emitters. However, the exploration of more donor and acceptor moieties is necessary to improve the device performance of TADF OLEDs. In particular, new donor moieties without carbazole units need to be investigated to expand the material-design platform of TADF emitters.
In this study, we designed and synthesized a high-performance TADF emitter with a simple molecular skeleton of donor–π-acceptor architecture. The synthesized TADF emitter, 2-(5H-benzo[d]benzo[4,5]imidazo[1,2-a]imidazol-5-yl)-5-(4,6-diphenyl-1,3,5-triazin-2-yl)benzonitrile (BzITz), comprises a fused rigid donor 5H-benzo[d]benzo[4,5]imidazo[1,2-a]imidazole (BzI) and a triazine acceptor. The effects of the BzI donor on the photophysical and electrochemical properties were studied in detail. The use of this fused rigid donor decreased ΔEST, shortened the delayed fluorescence lifetime, and improved the PLQY of the emitter. Furthermore, the potential of this TADF material in multilayered OLED devices was explored. The device demonstrated an excellent performance with an EQE of 24.0% and a low-efficiency roll-off compared with other reported carbazole-based TADF emitters.
Results and discussion
Design strategy and synthesis
Our study aims to examine the effects of the carbazole-free fused rigid donor moiety on the physiochemical and EL properties of the designed TADF emitter. The synthesized TADF emitter was BzITz with a 5H-benzo[d]benzo[4,5]imidazo[1,2-a]imidazole (BzI) donor. The BzI donor was chosen because it has a high triplet energy and a strong electron-donating character. It can alter the lowest singlet and triplet excited states, playing an important role in controlling the ΔEST and thus the TADF mechanism of the synthesized material. A benzonitrile linker was introduced between the donor and the triazine acceptor, not only to facilitate the RISC process but also to increase the HOMO and LUMO overlap for efficient emission. Scheme 1 illustrates the chemical structure of the emitter and its synthetic procedures. Intermediate 1 was synthesized according to the reported procedures with some modifications.34 Intermediate 2 was synthesized according to the procedure reported in our previous study.35 In a potassium phosphate-mediated N-arylation reaction, the above-mentioned intermediates 1 and 2 were reacted together to yield BzITz. The chemical structure of the emitter was confirmed through 1H and 13C nuclear magnetic resonance (NMR) spectroscopy and mass analysis. The emitter was purified via vacuum train sublimation before being assembled into an OLED device.
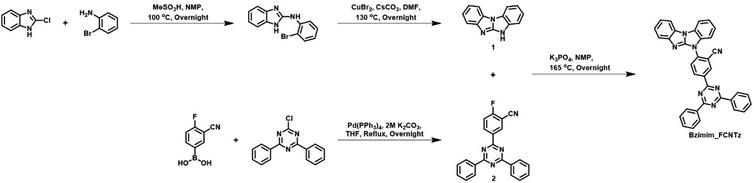 |
| Scheme 1 Synthesis of the BzITz emitter. | |
Theoretical calculations
To gain insight into the electronic structure, physical properties, and orbital distributions (HOMO and LUMO) of the target molecule, we performed theoretical calculations on the unconstrained geometry of BzITz using the global hybrid functional B3LYP and the 6-31G* basis set of the Gaussian 16 program. Fig. 1 shows the optimized geometries for BzITz and its HOMO/LUMO distributions. The dihedral angle between the BzI donor and the adjacent benzonitrile spacer was 54.6° because of the steric hindrance caused by the cyano group on the benzonitrile spacer. This is useful for separating HOMOs and LUMOs and for lowering the ΔEST. As shown in Fig. 1, the HOMO is mainly localized on the BzI donor, whereas the LUMO is primarily delocalized on the diphenyltriazine acceptor with a small contribution from the two peripheral phenyl rings on triazine, the bridged benzonitrile spacer, and the nitrogen atom of the BzI donor. The LUMO electron density appears to be relatively low on the peripheral phenyl rings of diphenyltriazine because the electron-withdrawing cyano group tends to pull the electron density over the benzonitrile spacer, where it is attached. The calculated HOMO–LUMO energy gap (Eg) was 3.26 eV, derived from the HOMO of −5.54 eV and the LUMO of −2.28 eV. A moderate HOMO level similar to that of carbazole was observed. Time-dependent density functional theory (DFT) computations were performed to calculate the energies of the S1 and T1 states. Because of the spatially partitioned HOMO and LUMO, BzITz is expected to have a minimal ΔEST. The energies for the S1 and T1 states were 2.82 and 2.58 eV, respectively, resulting in a ΔEST of 0.24 eV. These findings suggest that BzITz would trigger effective RISC for TADF emission.
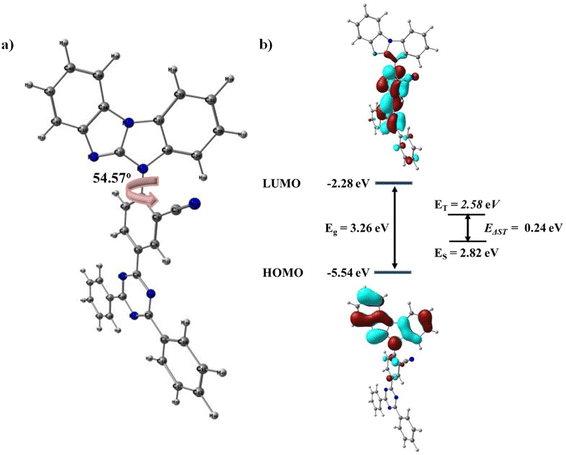 |
| Fig. 1 (a) Optimized geometry and (b) frontier molecular orbital distributions of the compounds, estimated using the global hybrid functional B3LYP and the 6-31G* basis set on the Gaussian 16 program. | |
Furthermore, the natural transition orbital (NTO) distributions for BzITz were calculated using the long-range corrected ωB97XD functional with the 6-31+G* basis set. Fig. S2 (ESI†), displays the highest occupied natural transition orbital (HONTO) and the lowest unoccupied natural transition orbital (LUNTO) for the singlet and triplet excited states. In the singlet excited state, the HONTO and LUNTO were extensively separated with slight overlap, indicating that the singlet emission can be interpreted as charge-transfer (CT) emission. On the other hand, as ascertained from the orbital pictures of the triplet excited state, the HONTO and LUNTO showed large overlapping over the phenyl ring of the benzonitrile unit and also separation, suggesting a mixed transition with CT and local-emission (LE) character. The mixed nature of the triplet excited state would be advantageous in increasing the RISC rate.36
Electrochemical properties
Cyclic voltammetry experiments were conducted on the emitter in dichloromethane using 0.1 M tetrabutylammonium perchlorate as the supporting electrolyte to investigate the emitter's redox characteristics. Oxidation potentials were calibrated using an Fc/Fc+ redox couple, and Table 1 presents the results. With reference to ferrocene (Fc), the HOMO and LUMO energy levels of the emitter were determined from the onset potentials of the oxidation and reduction waves, respectively. The corresponding HOMO and LUMO energy levels for the emitter are −6.11 and −3.09 eV, respectively, and hence the resulting HOMO–LUMO gap is 3.02 eV. BzI demonstrated a robust donor character with a small oxidation potential because of the low electronegativity of nitrogen in BzI.
Table 1 Photophysical and electrochemical data of the emitter
|
λ
abs
(nm) |
λ
emi
(nm) |
E
S
/ETd (eV) |
ΔESTe (eV) |
HOMO/LUMOf (eV) |
E
g
(eV) |
PLQYh (%) |
Measured in THF solution at RT.
Measured in toluene solution at RT.
Calculated from the onset of solid PL at RT.
Estimated from the onset value of phosphorescence spectra in toluene at 77 K.
Calculated singlet–triplet energy gap.
HOMO and LUMO estimated from cyclic voltammograms using HOMO = −(4.8 + Eox); LUMO = HOMO − E0-0.
Electrochemical bandgap obtained from the intersection of the absorption and emission spectra of THF solution.
Measured for 20 wt% doped films in DPEPO.
Under air.
Under nitrogen.
|
BzITz
|
365, 274 |
461 |
3.05/2.88 |
0.17 |
−6.11/−3.09 |
3.02 |
98.8i/99.2j |
Photophysical properties
The optical properties of the emitter were measured through steady-state ultraviolet-visible (UV-vis) absorption and photoluminescence (PL) spectra. Fig. 2 shows the normalized absorption spectrum recorded in tetrahydrofuran (1.0 × 10−5 M) and the PL spectrum recorded in toluene (1.0 × 10−5 M) at room temperature (RT). The BzITz emitter exhibited two independent absorption bands in the range of 250–400 nm, where the short-wavelength band observed below ∼300 nm is assigned to the localized π–π* electronic transitions of the aromatic segments, whereas the structureless broadband absorption at 365 nm is attributed to ICT from the BzI donor fragment to the diphenyltriazine acceptor fragment. The PL spectrum also exhibits single broadband emission at 461 nm, indicating that the singlet transition is initiated by CT states, as predicted by their spatially separated frontier molecular orbitals (Fig. 2). Furthermore, the emission profiles of the emitter were recorded in various solvents of varying polarity to better understand the CT nature of the excited state (Fig. S1, ESI†). As the solvent polarity was increased from non-polar toluene (Tol) to polar tetrahydrofuran (THF), the emission spectrum of the emitter became gradually red-shifted. Generally, a red-shifted broad emission in polar solvents suggests CT-excited states of the emitter.37 Therefore, BzITz can be regarded as a CT-based emitter.
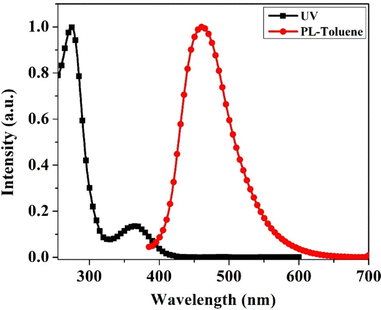 |
| Fig. 2 Absorption spectrum (black) of the emitter recorded in THF solution and emission spectrum (red) recorded in toluene solution at RT. | |
The solid PL, low-temperature fluorescence (LTFL), and low-temperature phosphorescence (LTPL) spectra of BzITz were recorded at ambient temperature and at 77 K, respectively, to evaluate its light-emitting features, such as the S1 and T1 state energies. By doping with the emitter in a bis[2-(diphenylphosphino)phenyl]ether oxide (DPEPO) host at a concentration of 20 wt%, solid PL was measured (Fig. 3a). BzITz exhibited a broad CT emission in the blue region with a peak at 467 nm. The phosphorescence spectrum of the emitter was also broad, indicating that its lowest triplet state is a CT state (Fig. 3b).38–41 The onset wavelengths from these LTFL and LTPL spectra were used to estimate the energy of the S1 and T1 states. The energies of S1 and T1 were 3.05 and 2.88 eV, respectively, providing a ΔEST of 0.17 eV. The emitter's small ΔEST value may efficiently promote T1 to S1 exciton upconversion through the RISC process.19 The BzI donor generated a significant CT character in the emitter, lowering the singlet energy. Conversely, the triplet energy was maintained by the significant twisting between the donor and acceptor resulting from steric interactions between the cyano group on the benzonitrile spacer and the BzI donor. These results were consistent with the theoretical calculations.
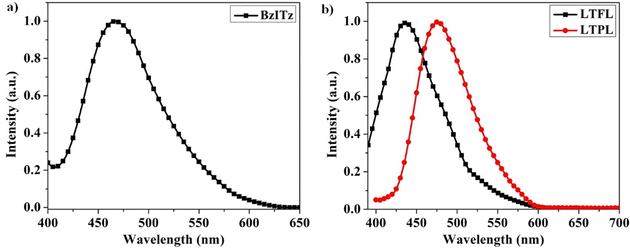 |
| Fig. 3 (a) Emission spectrum of the emitter recorded in a 20 wt% doped DPEPO film, and (b) fluorescence (black) and phosphorescence (red) spectra of the emitter recorded in the THF matrix at 77 K. | |
Transient PL measurements of BzITz were conducted using 20 wt% emitter-doped films in a DPEPO host at RT in a vacuum to gain insight into the emitter's delayed fluorescence behavior. Fig. 4 shows the transient PL decay curve, and Table 2 provides the corresponding data. The emitter-doped film exhibited prompt and delayed components with lifetimes of 16.6 ns and 7.93 μs, respectively. The emitter's delayed fluorescence is attributed to the RISC process, where triplet excitons are thermally upconverted into singlet excitons. Furthermore, the PLQY values for the prompt (ΦF) and delayed (ΦTADF) components were calculated by measuring the absolute PLQY using an integrating sphere as 65.9% and 33.3%, respectively.42 The total PLQY was 99.2% in a nitrogen atmosphere. The rate constants for prompt fluorescence (kp), delayed fluorescence (kd), intersystem crossing (ISC; kISC), RISC (kRISC), and the radiative decay for singlet (ksr), and non-radiative decay (ksnr) were calculated,43,44 and Table 2 presents the results. Because of the small ΔEST, the emitter had a lower ksnr value than ksr through an efficient upconversion process. The small ΔEST and low ksnr enhanced the PLQY of BzITz, implying that it has a substantial delayed-fluorescence contribution in light emission for use as a TADF emitter.
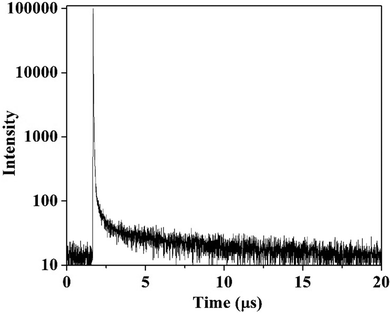 |
| Fig. 4 Transient PL decay profiles of the 20 wt% emitter-doped DPEPO film. | |
Table 2 Transient PL quantum yields and rate constants of BzITz
t
p
(ns) |
k
p
(107) |
t
d
(μs) |
k
d
(105) |
Φ
F
(%) |
Φ
TADF
(%) |
k
ISC
(107 s−1) |
k
RISC
(105 s−1) |
k
sr eh (107 s−1) |
k
snr ei (105 s−1) |
Prompt (p) and delayed (d) fluorescence lifetime.
Rate constant for prompt and delayed fluorescence lifetime.
Prompt and
delayed PLQY measured in DPEPO (20% dopant) thin film and calculated from the total PLQY and the proportion of the integrated area of the individual components in the transient spectra to the total integrated area.e
Calculated using the equations reported in the literature.43
Rate constant for ISC.
Rate constant for RISC.
Radiative decay rate constant for a singlet.
Non-radiative decay rate constant for a singlet.
|
16.6 |
6.02 |
7.93 |
1.26 |
65.9 |
33.3 |
2.02 |
1.90 |
3.97 |
3.20 |
Electroluminescence properties
The EL performance of a vacuum-processed multilayer OLED device was investigated using BzITz as a dopant in the DPEPO host matrix. The OLED device configuration used was indium tin oxide (20 nm)/PEDOT:PSS (40 nm)/TAPC (10 nm)/mCP (10 nm)/EML (25 nm, 10 wt%)/TSPO1 (5 nm)/TPBi (20 nm)/LiF (1.5 nm)/Al (200 nm). To evaluate the doping-concentration effect, the doping concentration of BzITz was varied from 10% to 40%. Diphenylphosphine oxide-4-(triphenylsilyl)phenyl (TSPO1) and 1,3-bis(N-carbazolyl)benzene (mCP) were used as electron-transporting and hole-transporting exciton blocking layers, respectively. Di-[4-(N,N-ditolylamino)phenyl]cyclohexane (TAPC) and LiF were used as hole-transport and electron-injection layers, respectively. BzITz-doped (10, 20, 30, or 40 wt%) DPEPO layers were used as the emission layers. To maintain the excitons confined in the dopant, high-triplet-energy DPEPO was chosen as the host. Fig. 5 depicts the device structure, energy-level alignment diagram, and chemical structures of the organic materials used in the device. Fig. 6 shows current density–voltage–luminance, EQE–luminance, and EL plots of the BzITz devices. Table 3 summarizes the detailed device parameters.
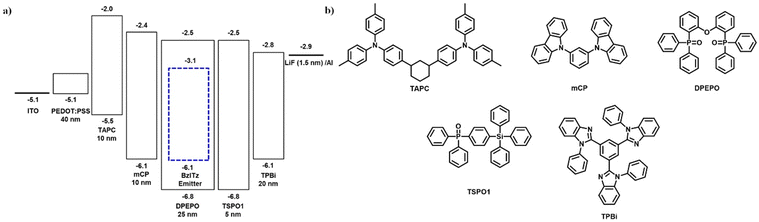 |
| Fig. 5 (a) Device structure and energy-level alignment of the materials, and (b) chemical structure of the materials. | |
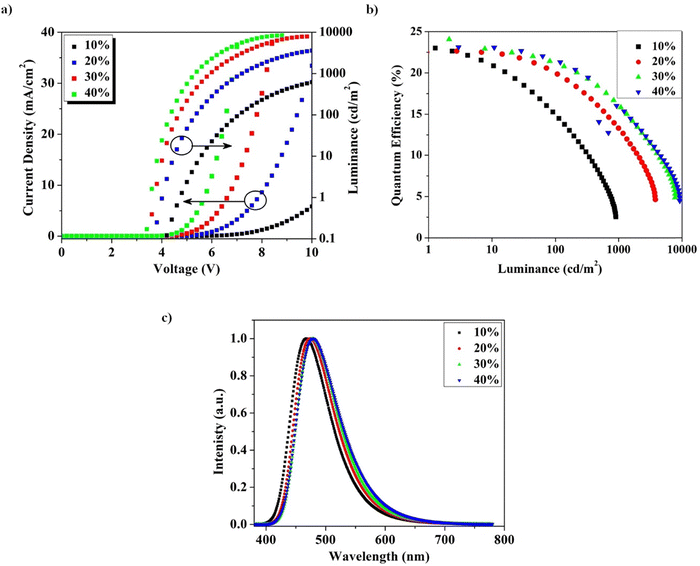 |
| Fig. 6 (a) J–V–L plot, (b) EQE vs. luminance plot, and (c) EL spectra of the emitter. | |
Table 3 EL properties of the emitter
Dopant |
Concentration (wt%) |
Voltage (V) |
EQE (%) |
Current efficiency (cd A−1) |
Power efficiency (lm W−1) |
CIE (x, y) |
Max |
@100 cd m−2 |
Max |
@100 cd m−2 |
Max |
@100 cd m−2 |
BzITz
|
10 |
9.4 |
23.0 |
15.0 |
37.7 |
24.1 |
27.9 |
10.7 |
0.17, 0.22 |
20 |
6.5 |
22.6 |
20.0 |
43.4 |
37.7 |
35.7 |
22.1 |
0.17, 0.27 |
30 |
5.4 |
24.0 |
21.2 |
49.4 |
43.1 |
40.8 |
29.1 |
0.18, 0.30 |
40 |
5.2 |
23.1 |
21.7 |
41.4 |
32.6 |
50.1 |
46.6 |
0.19, 0.33 |
As shown in Fig. 6a, the current density and luminance of the device gradually increase as the doping concentration is increased from 10% to 40%.35,45–47 This is explained by the dopant-aided charge injection and hopping mechanisms. As the dopant has a deep LUMO and a shallow HOMO relative to the DPEPO host, direct charge trapping in the dopant would be the dominant EL process in these devices. The energy-level diagram shows that the significant HOMO gap between mCP and DPEPO disrupted the hole injection and transport. Consequently, the dopant plays a crucial role as a hole-trapping and -transporting medium in the device. Therefore, the current density and luminance of the BzITz devices increase with increasing doping concentration.48
Fig. 6b shows the EQE vs. L plots of the BzITz devices at various doping concentrations. The optimized device has a maximum EQE of 24.0% at a doping concentration of 30 wt%. Notably, when the doping concentration was increased from 30% to 40%, the BzITz device did not show any substantial decrease in EQE, showing that the concentration quenching effect is insignificant in the BzITz emitter. In general, the distance between emitters causes the concentration quenching effect because the prospect of exciton collision and quenching increases with the doping concentration. However, it was not severe with BzITz because of its sterically hindered design, which reduced strong intermolecular interactions and hence minimized concentration quenching.49 This is further indirectly supported by the weak redshift in the EL spectrum with the doping concentration. The high EQE can be attributed to the emitter's high PLQY and high upconversion efficiency, which are aided by a small ΔEST and short td. As upconversion efficiency is critical to the EQE of the device because of the large population of triplet excitons in the EL mechanism, BzITz attained a high EQE by ensuring the efficient upconversion of triplet excitons into the singlet state. As shown in Fig. 6c, the EL spectra of the BzITz devices exhibited sky-blue emission similar to the PL spectra with CIE color coordinates of (0.18, 0.30). In addition, the emitter exhibited a small redshift of ∼12 nm when the doping concentration was increased from 10 to 40 wt% because of the use of a fused BzI donor. This small bathochromic shift observed in the EL spectra with an increasing doping concentration is attributed to strong intermolecular interactions at high doping ratios, which is common in host–guest-type OLEDs.35,50 The devices did not produce any other emissions from the host material or any other layers, implying that energy was transferred efficiently from the host to the guest. Table 4 illustrates the comparison of the device performance for some previously reported non-rigid donors35,51,52 and fused rigid donors51–53 used in TADF emitters. In particular, compared with TADF emitters that have the same backbone structure, except for the donor, the EQE was significantly improved. Therefore, it can be concluded that the BzITz emitter showed promising device performance characteristics, indicating that such fused rigid donors can be used as potential donors for developing highly efficient TADF emitters.
Table 4 Comparison of EL properties of reported emitters
Emitter |
EQE (%) |
Ref. |
TrzCz-CN |
14.4 |
51
|
BFICNTrz, X = O |
6.4 |
35
|
BTICNTrz, X = S |
15.2 |
BTITrz, X = S |
20.7 |
52
|
BFITrz, X = O |
12.0 |
DICzCNTrz |
21.4 |
53
|
CzDICzTrz |
19.9 |
54
|
Conclusion
In summary, we have designed and synthesized a rigid BzI-donor-based sky-blue TADF emitter of BzITz with a diphenyltriazine acceptor and a benzonitrile spacer. The BzITz TADF emitter exhibited a small ΔEST of 0.16 eV and a high PLQY of 99.2%. According to the results, BzITz exhibited a high maximum EQE of 24.0% with a small concentration-quenching effect. Compared with the carbazole-derived donor, the BzI donor offered a high EQE because of its high PLQY, indicating that the fused rigid unit can be used as an effective donor for developing efficient TADF emitters.
Experimental section
All synthesis-related information is provided in the ESI.†
A device structure of indium tin oxide (20 nm)/PEDOT:PSS (40 nm)/TAPC (10 nm)/mCP (10 nm)/EML (25 nm, 10 wt%)/TSPO1 (5 nm)/TPBi (20 nm)/LiF (1.5 nm)/Al (200 nm) was used to test the TADF emitter. Vacuum deposition of the organic materials was used to form the thin film of organic materials at a vacuum pressure of 10−7 torr. Measurement of the electrical and optical performances of the devices was conducted using a Keithley 2400 source measurement and CS 2000 spectroradiometer system.
Conflicts of interest
There are no conflicts to declare.
Acknowledgements
This work was supported by MOTIE (20012556).
References
- C. W. Tang and S. A. Vanslyke, Organic electroluminescent diodes, Appl. Phys. Lett., 1987, 51, 913–915 CrossRef CAS.
- J. H. Burroughes, D. D. C. Bradley, A. R. Brown, R. N. Marks, K. Mackay, R. H. Friend, P. L. Burns and A. B. Holmes, Light-emitting diodes based on conjugated polymers, Nature, 1990, 347, 539–541 CrossRef CAS.
- J. R. Sheats, H. Antoniadis, M. Hueschen, W. Leonard, J. Miller, R. Moon, D. Roitman and A. Stocking, Organic electroluminescent devices, Science, 1996, 273, 884–888 CrossRef CAS PubMed.
- H. J. Jang, J. Y. Lee, J. Kim, J. Kwak and J. H. Park, Progress of display performances: AR, VR, QLED, and OLED, J. Inf. Disp., 2020, 21, 1–9 CrossRef CAS.
- Z. Yang, Z. Mao, Z. Xie, Y. Zhang, S. Liu, J. Zhao, J. Xu, Z. Chi and M. P. Aldred, Recent advances in organic thermally activated delayed fluorescence materials, Chem. Soc. Rev., 2017, 46, 915–1016 RSC.
- J. M. Teng, Y. F. Wang and C. F. Chen, Recent progress of narrowband TADF emitters and their applications in OLEDs, J. Mater. Chem. C, 2020, 8, 11340–11353 RSC.
- V. V. Patil, H. Lee, I. Kim, K. H. Lee, W. J. Chung, J. Kim, S. Park, H. Choi, W. J. Son and S. O. Jeon, Purely Spin-Vibronic Coupling Assisted Triplet to Singlet Up-Conversion for Real Deep Blue Organic Light-Emitting Diodes with Over 20% Efficiency and y Color Coordinate of 0.05, Adv. Sci., 2021, 8, 2101137 CrossRef CAS.
- L. J. Rothberg and A. J. Lovinger, Status of and prospects for organic electroluminescence, J. Mater. Res., 1996, 11, 3174–3187 CrossRef CAS.
- R. H. Friend, R. W. Gymer, A. B. Holmes, J. H. Burroughes, R. N. Marks, C. Taliani, D. D. C. Bradley, D. A. Dos Santos, J. L. Bredas, M. Logdlund and W. R. Salaneck, Electroluminescence in conjugated polymers, Nature, 1999, 397, 121–128 CrossRef CAS.
- M. A. Baldo, D. F. O’Brien, D. Y. You, A. Shoustikov, S. Sibley, M. E. Thompson and S. R. Forrest, Highly efficient phosphorescent emission from organic electroluminescent devices, Nature, 1998, 395, 151–154 CrossRef CAS.
- Y. X. Wang, J. H. Yun, L. Wang and J. Y. Lee, High Triplet Energy Hosts for Blue Organic Light-Emitting Diodes, Adv. Funct. Mater., 2021, 31, 2008332 CrossRef CAS.
- S. K. Jeon, H. Lee, K. S. Yook and J. Y. Lee, Recent Progress of the Lifetime of Organic Light-Emitting Diodes Based on Thermally Activated Delayed Fluorescent Material, Adv. Mater., 2019, 31, 1803524 CrossRef PubMed.
- D. R. Lee, M. Kim, S. K. Jeon, S. H. Hwang, C. W. Lee and J. Y. Lee, Design strategy for 25% external quantum efficiency in green and blue thermally activated delayed fluorescent devices, Adv. Mater., 2015, 27, 5861–5867 CrossRef CAS PubMed.
- J. H. Kim, J. H. Yun and J. Y. Lee, Recent progress of highly efficient red and near-infrared thermally activated delayed fluorescent emitters, Adv. Opt. Mater., 2018, 6, 1800255 CrossRef.
- M. Y. Wong and E. Zysman-Colman, Purely Organic Thermally Activated Delayed Fluorescence Materials for Organic Light-Emitting Diodes, Adv. Mater., 2017, 29, 1605444 CrossRef.
- C. Li, R. S. Nobuyasu, Y. Wang, F. B. Dias, Z. Ren, M. R. Bryce and S. Yan, Solution-Processable Thermally Activated Delayed Fluorescence White OLEDs Based on Dual-Emission Polymers with Tunable Emission Colors and Aggregation-Enhanced Emission Properties, Adv. Opt. Mater., 2017, 5, 1700435 CrossRef.
- X. Cai and S.-J. Su, Marching Toward Highly Efficient, Pure-Blue, and Stable Thermally Activated Delayed Fluorescent Organic Light-Emitting Diodes, Adv. Funct. Mater., 2018, 28, 1802558 CrossRef.
- A. Endo, M. Ogasawara, A. Takahashi, D. Yokoyama, Y. Kato and C. Adachi, Thermally activated delayed fluorescence from Sn4+-porphyrin complexes and their application to organic light-emitting diodes – A novel mechanism for electroluminescence, Adv. Mater., 2009, 21, 4802–4806 CrossRef CAS PubMed.
- H. Uoyama, K. Goushi, K. Shizu, H. Nomura and C. Adachi, Highly efficient organic light-emitting diodes from delayed fluorescence, Nature, 2012, 492, 234–238 CrossRef CAS.
- Q. Zhang, B. Li, S. Huang, H. Nomura, H. Tanaka and C. Adachi, Efficient blue organic light-emitting diodes employing thermally activated delayed fluorescence, Nat. Photonics, 2014, 8, 326–332 CrossRef CAS.
- H. J. Jang, J. Y. Lee, G. W. Baek, J. Kwak and J. H. Park, Progress in the development of the display performance of AR, VR, QLED and OLED devices in recent years, J. Inf. Disp, 2022, 23, 1–17 CrossRef.
- B. D’Andrade, Applied physics: molecules that convert heat into light, Nature, 2012, 492, 197 CrossRef PubMed.
- Q. Zhang, J. Li, K. Shizu, S. Huang, S. Hirata, H. Miyazaki and C. Adachi, Design of efficient thermally activated delayed fluorescence materials for pure blue organic light emitting diodes, J. Am. Chem. Soc., 2012, 134, 14706–14709 CrossRef CAS PubMed.
- S. Y. Byeon, D. R. Lee, K. S. Yook and J. Y. Lee, Recent Progress of Singlet-Exciton-Harvesting Fluorescent Organic Light-Emitting Diodes by Energy Transfer Processes, Adv. Mater., 2019, 31, 1803714 CrossRef PubMed.
- G. Mehes, H. Nomura, Q. Zhang, T. Nakagawa and C. Adachi, Enhanced Electroluminescence Efficiency in a Spiro-Acridine Derivative through Thermally Activated Delayed Fluorescence, Angew. Chem., Int. Ed., 2012, 51, 11311–11315 CrossRef CAS PubMed.
- J. Li, T. Nakagawa, J. MacDonald, Q. Zhang, H. Nomura, H. Miyazaki and C. Adachi, Highly Efficient Organic Light-Emitting Diode Based on a Hidden Thermally Activated, Adv. Mater., 2013, 25, 3319–3323 CrossRef CAS PubMed.
- H. Wang, L. Xie, Q. Peng, L. Meng, Y. Wang, Y. Yi and P. Wang, Novel thermally activated delayed fluorescence materials-thioxanthone derivatives and their applications for highly efficient OLEDs, Adv. Mater., 2014, 26, 5198–5204 CrossRef CAS PubMed.
- S. Y. Lee, T. Yasuda, Y. S. Yang, Q. Zhang and C. Adachi, Luminous butterflies: Efficient exciton harvesting by benzophenone derivatives for full-color delayed fluorescence OLEDs, Angew. Chem., Int. Ed., 2014, 53, 6402–6406 CrossRef CAS PubMed.
- S. Hirata, Y. Sakai, K. Masui, H. Tanaka, S. Y. Lee, H. Nomura, N. Nakamura, M. Yasumatsu, H. Nakanotani, Q. Zhang, K. Shizu, H. Miyazaki and C. Adachi, Highly efficient blue electroluminescence based on thermally activated delayed fluorescence, Nat. Mater., 2015, 14, 330–336 CrossRef CAS PubMed.
- S. H. Han, J. H. Jeong, J. W. Yoo and J. Y. Lee, Ideal blue thermally activated delayed fluorescence emission assisted by a thermally activated delayed fluorescence assistant dopant through a fast reverse intersystem crossing mediated cascade energy transfer process, J. Mater. Chem. C, 2019, 7, 3082–3089 RSC.
- K. M. Youn, H. Lee, H. J. Yoo, Y. H. Jung, J. Do Park, H. Jeong, J. Lee, J. Y. Lee and J. H. Kwon, High triplet energy bipolar host materials with the combination of dibenzofuran and benziimidazobenzoimidazole moieties for blue thermally activated delayed fluorescence emitter, J. Mater. Chem. C, 2020, 8, 13811–13818 RSC.
- M. Godumala, S. Choi, M. J. Cho and D. H. Choi, Thermally activated delayed fluorescence blue dopants and hosts: from the design strategy to organic light-emitting diode applications, J. Mater. Chem. C, 2016, 4, 11355–11381 RSC.
- D. H. Kim, K. Inada, L. Zhao, T. Komino, N. Matsumoto, J. C. Ribierre and C. Adachi, Organic light emitting diodes with horizontally oriented thermally activated delayed fluorescence emitters, J. Mater. Chem. C, 2017, 5, 1216–1223 RSC.
- W. Chung and J. Y. Lee, The role of the bulky blocking unit of the fluorescent emitter in efficient green hyper-fluorescent organic light-emitting diodes, J. Inf. Disp., 2021, 22, 49–54 CrossRef CAS.
- R. K. Konidena, K. H. Lee, J. Y. Lee and W. P. Hong, Triggering Thermally Activated Delayed Fluorescence by Managing the Heteroatom in Donor Scaffolds: Intriguing Photophysical and Electroluminescence Properties, Chem. – Asian J., 2019, 14, 2251–2258 CrossRef CAS PubMed.
- H. L. Lee, K. H. Lee, J. Y. Lee and H. J. Lee, Molecular design opening two emission pathways for high efficiency and long lifetime of thermally activated delayed fluorescent organic light-emitting diodes, J. Mater. Chem. C, 2021, 9, 7328–7335 RSC.
- R. K. Konidena, K. R. Justin Thomas, A. Pathak, D. K. Dubey, S. Sahoo and J.-H. Jou, Tuning the Photophysical and Electroluminescence Properties in Asymmetrically Tetrasubstituted Bipolar Carbazoles by Functional Group Disposition, ACS Appl. Mater. Interfaces, 2018, 10, 24013–24027 CrossRef CAS PubMed.
- D. R. Lee, S. H. Hwang, S. K. Jeon, C. W. Lee and J. Y. Lee, Benzofurocarbazole and benzothienocarbazole as donors for improved quantum efficiency in blue thermally activated delayed fluorescent devices, Chem. Commun., 2015, 51, 8105–8107 RSC.
- L.-S. Cui, H. Nomura, Y. Geng, J. U. Kim, H. Nakanotani and C. Adachi, Controlling Singlet Triplet Energy Splitting for Deep-Blue Thermally Activated Delayed Fluorescence Emitters, Angew. Chemie, 2017, 129, 1593–1597 CrossRef.
- C. H. Ryoo, I. Cho, J. Han, J. H. Yang, J. E. Kwon, S. Kim, H. Jeong, C. Lee and S. Y. Park, Structure-Property Correlation in Luminescent Indolo[3,2-b]indole (IDID) Derivatives: Unraveling the Mechanism of High Efficiency Thermally Activated Delayed Fluorescence (TADF), ACS Appl. Mater. Interfaces, 2017, 9, 41413–41420 CrossRef CAS PubMed.
- W. Huang, M. Einzinger, T. Zhu, H. S. Chae, S. Jeon, S. G. Ihn, M. Sim, S. Kim, M. Su, G. Teverovskiy, T. Wu, T. Van Voorhis, T. M. Swager, M. A. Baldo and S. L. Buchwald, Molecular Design of Deep Blue Thermally Activated Delayed Fluorescence Materials Employing a Homoconjugative Triptycene Scaffold and Dihedral Angle Tuning, Chem. Mater., 2018, 30, 1462–1466 CrossRef CAS.
- K. Masui, H. Nakanotani and C. Adachi, Analysis of exciton annihilation in high-efficiency sky-blue organic light-emitting diodes with thermally activated delayed fluorescence, Org. Electron., 2013, 14, 2721–2726 CrossRef CAS.
- G. H. Kim, R. Lampande, J. B. Im, J. M. Lee, J. Y. Lee and J. H. Kwon, Controlling the exciton lifetime of blue thermally activated delayed fluorescence emitters using a heteroatom-containing pyridoindole donor moiety, Mater. Horiz., 2017, 4, 619–624 RSC.
- H. Noda, H. Nakanotani and C. Adachi, Excited state engineering for efficient reverse intersystem crossing, Sci. Adv., 2018, 4, 1–8 Search PubMed.
- T. B. Fleetham, L. Huang, K. Klimes, J. Brooks and J. Li, Tetradentate Pt(II) Complexes with 6-Membered Chelate Rings: A New Route for Stable and Efficient Blue Organic Light Emitting Diodes, Chem. Mater., 2016, 28, 3276–3282 CrossRef CAS.
- Y. J. Kang, J. H. Yun, S. H. Han and J. Y. Lee, Benzofuroacridine and benzothienoacridine as new
donor moieties for emission color management of thermally activated delayed fluorescent emitters, J. Mater. Chem. C, 2019, 7, 4573–4580 RSC.
- J. G. Yu, S. H. Han, H. L. Lee, W. P. Hong and J. Y. Lee, A novel molecular design employing a backbone freezing linker for improved efficiency, sharpened emission and long lifetime in thermally activated delayed fluorescence emitters, J. Mater. Chem. C, 2019, 7, 2919–2926 RSC.
- H. L. Lee, K. H. Lee, J. Y. Lee and W. P. Hong, Management of thermally activated delayed fluorescence using a secondary electron accepting unit in thermally activated delayed fluorescent emitters, J. Mater. Chem. C, 2019, 7, 6465–6474 RSC.
- X. Z. Xiao-Chun Fan, K. Wang, Y.-Z. Shi, D.-M. Sun, J.-X. Chen, F. Huang, H. Wang, J. Yu and C.-S. Lee, Thermally activated delayed fluorescence materials for nondoped organic light-emitting diodes with. nearly 100% exciton harvest, SmartMat, 2022, 1–11 Search PubMed.
- M. Kim, S. K. Jeon, S. H. Hwang and J. Y. Lee, Stable blue thermally activated delayed fluorescent organic light-emitting diodes with three times longer lifetime than phosphorescent organic light-emitting diodes, Adv. Mater., 2015, 27, 2515–2520 CrossRef CAS PubMed.
- H. Li, J. Li, D. Liu and Y. Mei, Mechanism evolution from normal fluorescence to thermally activated delayed fluorescence and color tuning over visible light range: Effect of intramolecular charge transfer strength, Dyes Pigm., 2020, 183, 108732 CrossRef CAS.
- R. K. Konidena, K. H. Lee and J. Y. Lee, 6H-Benzo[4,5]thieno[2,3-b] indole as a novel donor for efficient thermally activated delayed fluorescence emitters with EQEs over 20%, J. Mater. Chem. C, 2019, 7, 13912–13919 CAS.
- R. K. Konidena, K. H. Lee, J. Y. Lee and W. P. Hong, 15H-Diindolo[2,3-b1′,2′,3′-lm]carbazole: a novel rigid donor for highly efficient thermally activated delayed fluorescence emitters, J. Mater. Chem. C, 2019, 7, 8037–8044 RSC.
- R. K. Konidena, K. H. Lee and J. Y. Lee, Molecular design featuring carbazole-decorated 15H-diindolo[2,3-b:1′,2′,3′-lm] carbazole for improved efficiency and lifetime of thermally activated delayed fluorescence emitters, J. Mater. Chem. C, 2020, 8, 2491–2499 RSC.
|
This journal is © the Partner Organisations 2022 |