DOI:
10.1039/C4QO00001C
(Research Article)
Org. Chem. Front., 2014,
1, 235-239
Ru(II)-catalyzed rearrangement of the allenic sulfide bearing propargyl moiety: efficient formation of benzene derivatives†‡
Received
2nd January 2014
, Accepted 21st January 2014
First published on 7th February 2014
Abstract
[RuCl2(p-cymene)]2 efficiently catalyzes the rearrangement of allenic sulfides bearing the propargyl moiety to afford 1,2,4-trisubstituted benzene derivatives. The alkyne-Ru(II) carbene [2 + 2] cycloaddition/ring opening process has been proposed in the reaction mechanism.
Introduction
Transition-metal-catalyzed reactions of allene derivatives have attracted great attention in recent years.1,2 Many synthetically useful transformations have been developed in this area. In a few cases a metal carbene has been proposed as a reactive intermediate in these transformations.3 Recently, we have reported the highly efficient reaction of [RuCl2(p-cymene)]2- or PtCl2-catalyzed rearrangement of β-carbonyl allenic sulfides, which gives furan as products (Scheme 1A and B).4 The reaction is assumed to proceed through 1,4-sulfanyl group migration, which leads to the formation of a metal carbene intermediate (Scheme 1A). The metal carbene intermediate is then trapped intramolecularly by carbonyl oxygen to afford the furan derivatives as final products.
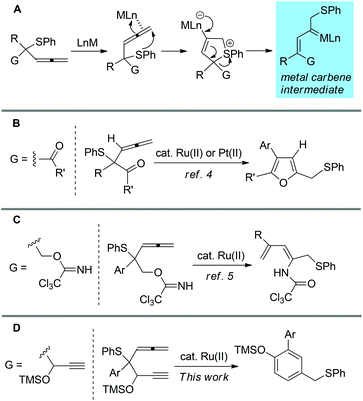 |
| Scheme 1 Transformations based on metal carbene intermediate generated from transition-metal-catalyzed rearrangement of allenyl sulfide. | |
Inspired by this work, we further conceived that replacing the carbonyl group with nucleophilic functional group G in such a system might lead to novel transformations of the metal-carbene intermediate. In this context, we have previously reported Ru(II)-catalyzed reaction of 2-aryl-2-(phenythio) penta-3,4-dienyl 2,2,2-trichloro acetimidates, which leads to a novel rearrangement (Scheme 1C).5 In this paper, we further demonstrate that Ru(II)-catalyzed reaction of β-ethynyl allenic sulfides leads to the formation of benzene derivatives (Scheme 1D). An alkyne-Ru(II) carbene [2 + 2] cycloaddition/ring opening process has been proposed for the reaction mechanism.
Results and discussion
We discovered this type of transformation firstly from investigating the reaction of β-ethynyl allenic sulfides.6 When β-ethynyl allenic sulfide 1 was catalyzed by 5 mol% [RuCl2(p-cymene)2] in DCE at 60 °C, two products 2 and 3, which are different in the position of acetyl substituents on the benzene ring, were obtained in 90% yield in 1 h (eqn (1)). The reaction shows no selectivity, with the ratio of 2 and 3 being 1
:
1. | 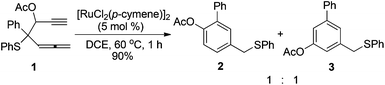 | (1) |
We proposed a mechanism for the formation of 2 and 3 as shown in Scheme 2. Similar to the reaction we have published previously,4,5 intramolecular attack of the phenylsulfanyl group on the Ru(II)-activated allene moiety triggers sequential rearrangement to form Ru(II) carbene intermediate C. The Ru(II) carbene interacts with the tethered alkyne moiety and generates a new Ru(II) carbene intermediate E. This process is presumably proceeding via an alkene–alkyne metathesis-like pathway through intermediate D.7 Notably, Padwa and coworkers have reported a series of studies on cyclization of the initially formed metal carbenoid onto the tethered alkyne unit to give a new metal carbenoid which could lead to diverse transformations such as 1,2-migration, cyclopropanation, ylide formation with carbonyl, C–H insertion.8 In Padwa's system, the initially formed metal carbene species are generated from the transition-metal-catalyzed decomposition of diazo compounds. Finally, the 1,2-H migration or 1,2-OAc migration of the Ru(II) carbene intermediate gives product 2 or 3, respectively.
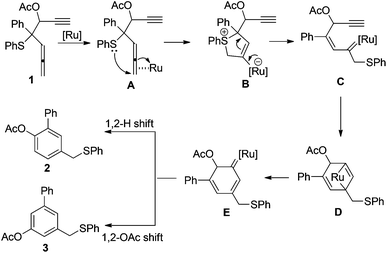 |
| Scheme 2 Mechanistic hypothesis. | |
The Ru(II)-catalyzed transformation of 1 to benzene products 2 and 3 was highly efficient; it was completed in 1 hour with high yield. Encouraged by this result, we proceeded to investigate more β-ethynyl allenic sulfide substrates. According to the reaction mechanism proposed in Scheme 2, the final step in the catalytic cycle is the competitive 1,2-H shift or 1,2-OAc shift of the Ru-carbene intermediate. We have conceived that by replacing the OAc with a group of low 1,2-migratory aptitude the 1,2-H shift may become predominant. For this purpose, we synthesized β-ethynyl allenic sulfide 4a, in which the OAc is replaced with the TMS group. To our delight, the Ru(II)-catalyzed reaction of 4a under the same reaction conditions gave only 1,2-H migration products 5a and 6a in 81% and 17% yield respectively (Scheme 1A). The phenol product 6a was formed by the deprotection of the TMS group of 5a during the isolation process. To simplify the reaction, we decided to deprotect the TMS group of 5ain situ upon the completion of Ru(II)-catalyzed transformation of 4a. After screening of some TMS deprotection conditions, we found that adding 1.0 equiv. of TFA to the crude product of the Ru(II)-catalyzed transformation dissolved in MeOH gave phenol 6a in 91% isolated yield (Scheme 3B). The structure of 6a was further confirmed by X-ray analysis (Fig. 1).9,10
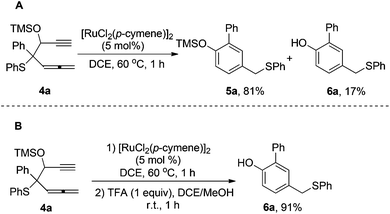 |
| Scheme 3 [RuCl2(p-cymene)]2-catalyzed reaction of 4a. | |
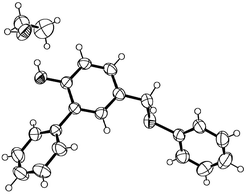 |
| Fig. 1 ORTEP representation of 6a·EtOH. | |
Next, we proceeded to synthesize a series of β-ethynyl allenic sulfides 4a–j for studying the substrate scope of this novel Ru(II)-catalyzed rearrangement. As outlined in Scheme 4, the nucleophilic addition of ethynylmagnesium to aldehydes 7a–j afforded the corresponding alcohol 8a–j. Treatment of the alcohols with trimethylsilyl chloride gave the desired β-ethynyl allenic sulfides 4a–j as diastereomeric mixtures in each case.
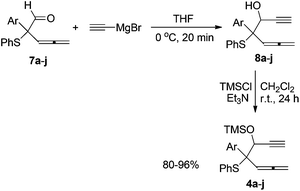 |
| Scheme 4 Preparation of β-ethynyl allenic sulfides 4a–j. | |
With the β-ethynyl allenic sulfide substrates in hand, we proceeded to investigate the reaction of a series of β-ethynyl allenic sulfides 4a–j, under the conditions described in Scheme 3. The Rh(II)-catalyzed reaction and the deprotection of the TMS group of the crude products in situ with TFA afforded the corresponding phenol products 6a–j (Scheme 5). Most of the substrates employed in this study gave the corresponding phenol products in good yields. The only exception is when the Ar is a 1-naphthyl group. In this case, the yield of the corresponding product 6i is slightly diminished.
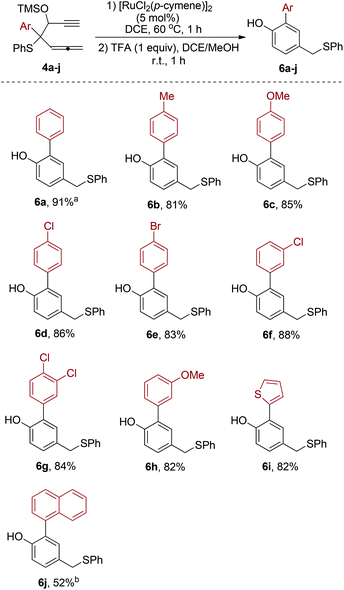 |
| Scheme 5 [RuCl2(p-cymene)]2-catalyzed reaction of 4a–j. aIsolated yields. bThe first step needs 2 h for completion of the reaction. | |
Furthermore, we proceeded to extend the substrate scope to allenic sulfides bearing the cyclic moiety. Unfortunately, the Ru(II)-catalyzed reaction of 9 under the same reaction conditions was sluggish and gave a complex mixture after heating for 2 days (Scheme 6A). However, the reaction with allenic sulfide 10, which bears OAc substituent, gave the expected benzene derivative 11 in 50% yield (Scheme 6B). Notably, only the product attributed to 1,2-OAc migration is formed.
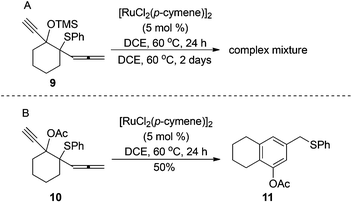 |
| Scheme 6 Ru(II)-catalyzed reaction with allenic sulfides 9 and 10. | |
Conclusions
In summary, we have reported a novel Ru(II)-catalyzed rearrangement of allenic sulfides bearing the propargyl moiety. The reaction affords 1,2,4-trisubstituted benzene derivatives in good yields.11 This rearrangement further demonstrates the generality of the Ru-carbene generation through the rearrangement of the allenic sulfide system. Further transformations may be expected based on this type of rearrangement and studies along this line are ongoing in our laboratory.
Experimental section
General
All reactions were performed under a nitrogen atmosphere in a flame-dried reaction flask. All solvents were distilled prior to use. Toluene and THF were distilled over sodium, and DCE was distilled over NaH. For chromatography, 200–300 mesh silica gel (Yantai, China). 1H and 13C NMR spectra were recorded at 300 MHz (or 200 MHz) and 75 MHz (or 50 MHz) with a Varian Mercury 300 spectrometer. Chemical shifts are reported in ppm using tetramethylsilane as an internal standard. IR spectra were recorded using a Nicolet 5MX-S infrared spectrometer. Mass spectra were obtained on a VG ZAB-HS mass spectrometer. For the preparation of substrates 4a–j and 10, see the ESI.‡
General procedure for [RuCl2(p-cymene)]2-catalyzed reaction of 4a–j and the subsequent deprotection of the TMS group
Under a nitrogen atmosphere, [RuCl2(p-cymene)]2 (0.025 mmol) and 1-ethynyl-2-phenylthio-3,4-pentadienyl trimethylsilyl ether 4a–j (0.5 mmol) were mixed in anhydrous DCE (5 mL) in a 25 mL round-bottomed flask. The reaction was continued at 60 °C (oil-bath) until completion as judged by TLC. The reaction system was cooled down to room temperature, and methanol (5 mL) and TFA (0.5 mmol) were then added to this solution. The reaction was continued at room temperature for about 1 h. Removal of the solvent in vacuo gave a crude residue, which was purified by a silica gel column. Elution with petroleum ether–ethyl acetate (15
:
1) afforded pure phenol product 6a–j.
(2-Phenyl-4-phenylthiomethyl)phenyl acetate (2) and (3-phenyl-5-phenylthiomethyl) phenyl acetate (3) (a 1
:
1 inseparable mixture).
IR (film) 3063, 2913, 1764, 1209, 1193, 764, 738, 691 cm−1; 1H NMR (CDCl3, 300 MHz) δ 2.04 (s, 3H), 2.27 (s, 3H), 4.11 (s, 2H), 4.12 (s, 2H), 7.03–7.50 (m, 26H); 13C NMR (CDCl3, 75 MHz) δ 20.7, 21.0, 38.5, 38.9, 119.1, 120.6, 122.8, 125.0, 126.5, 126.5, 127.0, 127.4, 127.7, 128.2, 128.7, 128.7, 128.7, 128.8, 128.8, 130.1, 130.2, 131.2, 134.7, 135.5, 135.7, 135.9, 137.2, 139.5, 139.8, 142.7, 146.7, 151.0, 169.2. EI-MS (m/z, relative intensity): 334 (M+, 22), 292 (7), 225 (17), 183 (100), 109 (4), 77 (4), 43 (14). HRMS calcd for C21H18O2S [M+] 334.1028; found: 334.1032.
(2-Phenyl-4-phenylthiomethyl)phenyl trimethylsilyl ether (5a).
IR (film) 3061, 2954, 1479, 1261, 1253, 909, 843, 736, 701 cm−1; 1H NMR (CDCl3, 300 MHz) δ 0.04 (s, 9H), 4.12 (s, 2H), 6.84 (d, J = 8.1 Hz, 1H), 7.15–7.50 (m, 12H); 13C NMR (CDCl3, 75 MHz) δ 0.016, 38.73, 120.60, 126.38, 126.77, 127.78, 128.79, 129.52, 129.89, 130.20, 130.61, 131.25, 133.05, 136.31, 138.66, 151.39. EI-MS (m/z, relative intensity): 364 (M+, 6), 349 (3), 255 (100), 73 (55). HRMS calcd for C22H24OSSi [M+] 364.1317; found: 364.1304.
(2-Phenyl-4-phenylthiomethyl)phenol (6a).
IR (film) 3531, 3055, 1584, 1485, 1272, 1173, 764, 739, 700, 691 cm−1; 1H NMR (CDCl3, 300 MHz) δ 4.09 (s, 2H), 5.21 (s, 1H), 6.90 (d, J = 8.1 Hz, 1H), 7.14–7.50 (m, 12H); 13C NMR (CDCl3, 75 MHz) δ 38.47, 115.90, 126.30, 127.86, 128.80, 128.95, 129.17, 129.49, 129.93, 130.66, 136.27, 136.72, 151.48. EI-MS (m/z, relative intensity): 292 (M+, 7), 183 (100), 165 (3), 109 (5), 77 (6). HRMS calcd for C19H16OS [M+] 292.0922; found: 292.0921.
[2-(4-Methylphenyl)-4-phenylthiomethyl]phenol (6b).
IR (film) 3530, 3440, 3022, 2919, 1584, 1489, 1481, 1269, 1187, 824, 739, 690 cm−1; 1H NMR (CDCl3, 300 MHz) δ 2.39(s, 3H), 5.23 (s, 1H), 6.88 (d, J = 8.1 Hz, 1H), 7.11–7.33 (m, 11H); 13C NMR (CDCl3, 75 MHz) δ 21.15, 38.52, 115.79, 126.28, 127.91, 128.80, 129.28, 129.46, 129.91, 129.94, 130.60, 133.70, 136.38, 137.70, 151.58. EI-MS (m/z, relative intensity): 306 (M+, 6), 197 (100), 181 (6), 109 (5). HRMS calcd for C20H18OS [M+] 306.1078; found: 306.1074.
[2-(4-Methoxyphenyl)-4-phenylthiomethyl]phenol (6c).
IR (film) 3531, 3409, 2916, 1608, 1515, 1491, 1277, 1246, 1178, 1026, 908, 834, 734, 690 cm−1; 1H NMR (CDCl3, 300 MHz) δ 3.80 (s, 3H), 4.06 (s, 2H), 5.33 (s, 1H), 6.84–6.87 (m, 1H), 6.95–6.98 (m, 2H), 7.10–7.33 (m, 9H); 13C NMR (CDCl3, 75 MHz) δ 38.46, 55.24, 114.52, 115.76, 126.23, 127.66, 128.74, 128.92, 129.05, 129.41, 129.87, 130.11, 130.64, 136.36, 151.61, 159.18. EI-MS (m/z, relative intensity): 322 (M+, 8), 213 (100), 197 (5), 181 (8), 169 (5), 109 (6). HRMS calcd for C20H18O2S [M+] 322.1028; found: 322.1034.
[2-(4-Chlorophenyl)-4-phenylthiomethyl]phenol (6d).
IR (film) 3539, 3399, 3056, 1584, 1482, 1089, 1014, 833, 740, 691 cm−1; 1H NMR (CDCl3, 300 MHz) δ 4.07 (s, 2H), 5.08 (s, 1H), 6.84 (d, J = 8.4 Hz, 1H), 7.08–7.42 (m, 11H); 13C NMR (CDCl3, 75 MHz) δ 38.47, 116.15, 126.42, 126.86, 128.82, 129.13, 129.70, 129.88, 138.08, 130.37, 130.64, 133.76, 135.32, 136.12, 151.39. EI-MS (m/z, relative intensity): 326 (M+, 8), 217 (100), 181 (17), 152 (11), 109 (8). HRMS calcd for C19H15OS35Cl [M+] 326.0532; found: 326.0540.
[2-(4-Bromolphenyl)-4-phenylthiomethyl]phenol (6e).
IR (film) 3537, 3415, 2917, 1584, 1481, 1172, 1072, 1009, 830, 739, 690 cm−1; 1H NMR (CDCl3, 300 MHz) δ 4.06 (s, 2H), 5.11 (s, 1H), 6.84 (d, J = 8.4 Hz, 1H), 7.08–7.30 (m, 9H), 7.56 (d, J = 8.4 Hz, 2H); 13C NMR (CDCl3, 75 MHz) δ 38.47, 116.17, 121.92, 126.41, 126.86, 128.81, 129.72, 129.90, 130.07, 130.58, 130.68, 132.06, 135.81, 136.10, 151.34. EI-MS (m/z, relative intensity): 370 (M+, 7), 261 (100), 181 (53), 152 (19), 128 (10), 109 (17). HRMS calcd for C19H15OS79Br [M+] 370.0027; found: 370.0029.
[2-(3-Chlorophenyl)-4-phenylthiomethyl]phenol (6f).
IR (film) 3541, 3411, 3058, 1594, 1564, 1477, 1174, 783, 739, 691 cm−1; 1H NMR (CDCl3, 300 MHz) δ 4.08 (s, 2H), 5.05 (s, 1H), 6.88 (d, J = 8.4 Hz, 1H), 7.08–7.41 (m, 11H); 13C NMR (CDCl3, 75 MHz) δ 38.54, 116.22, 126.53, 126.63, 127.07, 127.78, 128.84, 129.22, 129.87, 129.91, 130.11, 130.28, 130.66, 134.81, 136.00, 138.79, 151.41. EI-MS (m/z, relative intensity): 326 (M+, 8), 217 (100), 181 (16), 152 (12), 109 (9). HRMS calcd for C19H15OS35Cl [M+] 326.0532; found: 326.0529.
[2-(4-Dichlorophenyl)-4-phenylthiomethyl]phenol (6g).
IR (film) 3547, 3384, 1584, 1550, 1471, 1133, 1029, 825, 739, 690 cm−1; 1H NMR (CDCl3, 300 MHz) δ 4.06 (s, 2H), 4.73 (s, 1H), 6.82 (d, J = 8.1 Hz, 1H), 7.06–7.50 (m, 9H); 13C NMR (CDCl3, 75 MHz) δ 38.54, 116.38, 125.75, 126.63, 128.36, 128.88, 130.05, 130.18, 130.39, 130.61, 130.64, 131.02, 131.73, 132.84, 135.87, 137.13, 151.39. EI-MS (m/z, relative intensity): 360 (M+, 6), 251 (100), 215 (18), 181 (11), 149 (20), 109 (22). HRMS calcd for C19H14OS35Cl2 [M+] 360.0142; found: 360.0142.
[2-(3-Methoxyphenyl)-4-phenylthiomethyl]phenol (6h).
IR (film) 3426, 3058, 2940, 2835, 1598, 1583, 1480, 1220, 1163, 1034, 823, 778, 740, 691 cm−1; 1H NMR (CDCl3, 300 MHz) δ 3.83 (s, 3H), 4.09 (s, 2H), 5.31 (s, 1H), 6.89–6.99 (m, 4H), 7.14–7.38 (m, 8H); 13C NMR (CDCl3, 75 MHz) δ 38.48, 55.27, 113.60, 114.37, 115.90, 121.03, 126.32, 127.73, 128.78, 129.49, 129.57, 129.99, 130.29, 130.46, 136.27, 138.09, 151.51, 160.17. EI-MS (m/z, relative intensity): 322 (M+, 7), 213 (100), 181 (9), 109 (5). HRMS calcd for C20H18O2S [M+] 322.1028; found: 322.1034.
[2-4-Phenylthiomethyl-(2-thiophenyl)]phenol (6i).
IR (film) 3515, 1583, 1494, 1479, 1439, 840, 739, 691 cm−1; 1H NMR (CDCl3, 300 MHz) δ 4.06 (s, 2H), 5.57 (s, 1H), 6.86 (d, J = 8.4 Hz, 1H), 7.10–7.37 (m, 10H); 13C NMR (CDCl3, 75 MHz) δ 38.41, 116.20, 120.81, 125.97, 126.21, 126.42, 127.71, 128.82, 129.63, 129.70, 130.03, 130.27, 136.08, 138.41, 151.46. EI-MS (m/z, relative intensity): 298 (M+, 11), 206 (42), 189 (100), 135 (16), 109 (11), 43 (16). HRMS calcd for C17H14OS2 [M+] 298.0486; found: 298.0484.
[2-(1-Naphthyl)-4-phenylthiomethyl]phenol (6j).
IR (film) 3533, 3056, 1585, 1492, 1182, 908, 804, 784, 738, 690 cm−1; 1H NMR (CDCl3, 300 MHz) δ 4.10 (s, 2H), 4.82 (s, 1H), 6.98 (d, J 8.1 Hz, 1H), 7.14–7.56 (m, 12H), 7.89–7.92 (m, 2H); 13C NMR (CDCl3, 75 MHz) δ 38.55, 115.73, 125.54, 125.66, 126.02, 126.32, 126.38, 126.75, 128.10, 128.41, 128.77, 128.82, 129.31, 129.98, 130.22, 131.62, 131.70, 133.65, 133.87, 136.11, 152.23. EI-MS (m/z, relative intensity): 342 (M+, 8), 233 (100), 215 (14), 203 (18), 189 (13), 179 (30), 165 (14), 152 (25). HRMS calcd for C23H18OS [M+] 342.1076; found: 342.1074.
(3-Phenylthiomethyl-5,6,7,8-tetrahydrogen)-1-naphthyl acetate (11).
IR (film) 2932, 1763, 1205, 1039, 738, 691 cm−1; 1H NMR (CDCl3, 300 MHz) δ 1.75 (s, 4H), 2.28 (s, 3H), 2.52 (s, 2H), 2.72 (s, 2H), 4.05 (s, 2H), 6.81 (s, 1H), 6.92 (s, 1H), 7.18–7.33 (m, 5H); 13C NMR (CDCl3, 75 MHz) δ 20.75, 22.32, 22.52, 23.06, 29.31, 38.37, 119.25, 126.19, 127.22, 128.34, 128.80, 129.49, 135.17, 136.46, 139.22, 148.97, 169.26. EI-MS (m/z, relative intensity): 312 (M+, 20), 270 (2), 203 (42), 161 (100), 147 (20), 91 (23), 43 (47). HRMS calcd for C19H20O2S [M+] 312.1184; found: 312.1172.
Notes and references
- For recent monographs, see:
(a)
Modern Allene Chemistry, ed. N. Krause and A. S. K. Hashmi, Wiley-VCH, Weinheim, Germany, 2004, vol. 1–2 Search PubMed;
(b)
S. Ma, Palladium-Catalyzed Two- or Three-Component Cyclization of Functionalized Allenes in Palladium in Organic Synthesis, ed. J. Tsuji, Springer, Berlin, Germany, 2005, p. 183 Search PubMed;
(c)
Science of Synthesis, ed. N. Krause, Thieme, Stuttgart, Germany, 2007, vol. 44 Search PubMed.
- For reviews on allenes, see:
(a) R. Zimmer, C. Dinesh, E. Nandanan and F. A. Khan, Chem. Rev., 2000, 100, 3067 CrossRef CAS PubMed;
(b) A. S. K. Hashmi, Angew. Chem., Int. Ed., 2000, 39, 3590 CrossRef CAS;
(c) J. A. Marshall, Chem. Rev., 2000, 100, 3163 CrossRef CAS PubMed;
(d) X. Lu, C. Zhang and Z. Xu, Acc. Chem. Res., 2001, 34, 535 CrossRef CAS PubMed;
(e) R. Bates and V. Satcharoen, Chem. Soc. Rev., 2002, 31, 12 RSC;
(f) S. Ma, Acc. Chem. Res., 2003, 36, 701 CrossRef CAS PubMed;
(g) L. Sydnes, Chem. Rev., 2003, 103, 1133 CrossRef CAS PubMed;
(h) M. Tius, Acc. Chem. Res., 2003, 36, 284 CrossRef CAS PubMed;
(i) L. L. Wei, H. Xiong and R. P. Hsung, Acc. Chem. Res., 2003, 36, 773 CrossRef CAS PubMed;
(j) L. Brandsma and N. A. Nedolya, Synthesis, 2004, 735 CrossRef CAS PubMed;
(k) S. Ma, Chem. Rev., 2005, 105, 2829 CrossRef PubMed;
(l) S. Ma, Aldrichimica Acta, 2007, 40, 91 CAS;
(m) M. Brasholz, H.-U. Reissig and R. Zimmer, Acc. Chem. Res., 2009, 42, 45 CrossRef CAS PubMed;
(n) S. Ma, Acc. Chem. Res., 2009, 42, 1679 CrossRef CAS PubMed;
(o) C. Aubert, L. Fensterbank, P. Garcia, M. Malacria and A. Simonneau, Chem. Rev., 2011, 111, 1954 CrossRef CAS PubMed;
(p) S. Yu and S. Ma, Angew. Chem., Int. Ed., 2012, 51, 3074 CrossRef CAS PubMed.
-
(a) A. S. K. Hashmi, Angew. Chem., Int. Ed. Engl., 1995, 34, 1581 CrossRef;
(b) A. S. K. Hashmi, T. L. Ruppert, T. Knöfel and J. W. Bats, J. Org. Chem., 1997, 62, 7295 CrossRef CAS PubMed;
(c) A. W. Sromek, M. Rubina and V. Gevorgyan, J. Am. Chem. Soc., 2005, 127, 10500 CrossRef CAS PubMed;
(d) A. S. Dudnik and V. Gevorgyan, Angew. Chem., Int. Ed., 2007, 46, 5195 CrossRef CAS PubMed;
(e) N. Nishina and Y. Yamamoto, Angew. Chem., Int. Ed., 2006, 45, 3314 CrossRef CAS PubMed;
(f) X. Huang and L. Zhang, J. Am. Chem. Soc., 2007, 129, 6398 CrossRef CAS PubMed.
- L. Peng, X. Zhang, M. Ma and J. Wang, Angew. Chem., Int. Ed., 2007, 46, 1905 CrossRef CAS PubMed.
- L. Peng, X. Zhang, J. Ma and J. Wang, J. Organomet. Chem., 2011, 696, 118 CrossRef CAS PubMed.
- J. Ma, L. Peng, X. Zhang, Z. Zhang, M. Campbell and J. Wang, Chem.–Asian J., 2010, 5, 2214 CrossRef CAS PubMed.
- For a related example, see: M. Kim, R. L. Miller and D. Lee, J. Am. Chem. Soc., 2005, 127, 12818 CrossRef CAS PubMed.
-
(a) A. Padwa, K. E. Krumpe and J. M. Kassir, J. Org. Chem., 1992, 57, 4940 CrossRef CAS;
(b) A. Padwa and F. R. Kinder, J. Org. Chem., 1993, 58, 21 CrossRef CAS;
(c) A. Padwa and M. D. Weingarten, J. Org. Chem., 2000, 65, 3722 CrossRef CAS PubMed;
(d) A. Padwa and C. S. Straub, Org. Lett., 2000, 2, 2093 CrossRef CAS PubMed;
(e) A. Padwa, J. Organomet. Chem., 2001, 617–618, 3 CrossRef CAS.
- CCDC 969849 contains the supplementary crystallographic data for this paper.
- L. Peng, X. Zhang, J. Ma, Z. Zhong and J. Wang, Org. Lett., 2007, 9, 1445 CrossRef CAS PubMed.
- For a recent example of transition-metal-catalyzed arene synthesis, see: L. Xu, R. Yu, Y. Wang, J. Chen and Z. Yang, J. Org. Chem., 2013, 78, 5744 CrossRef CAS PubMed.
Footnotes |
† Dedicated to Professor Max Malacria on the occasion of his 65th birthday. |
‡ Electronic supplementary information (ESI) available: Preparation of substrates, characterization data, 1H, 13C NMR, MS and IR spectra. CCDC 969849. For ESI and crystallographic data in CIF or other electronic format see DOI: 10.1039/c4qo00001c |
|
This journal is © the Partner Organisations 2014 |