DOI:
10.1039/D3AN00051F
(Paper)
Analyst, 2023,
148, 2012-2020
Determination of the quality of lipoproteins by Raman spectroscopy in obese and healthy subjects†
Received
10th January 2023
, Accepted 11th March 2023
First published on 28th March 2023
Abstract
Lipoproteins (LPs) are multimolecular complexes of lipids and proteins responsible for transporting fatty acids, cholesterol, and micronutrients (carotenoids) through the body. The quantification of triglycerides and cholesterol carried by lipoproteins is a leading clinical parameter to assess the increased risk of cardiovascular events. However, in recent times, the study of the overall “quality” of lipoproteins, defined by their biochemical composition and oxidation state, has emerged as necessary to improve the definition of the cardiovascular risk. In this work, we present Raman spectroscopy (RS) as an effective method to immediately detect the functional groups relative to the principal biochemical components and the level of unsaturated lipids present in LPs. Furthermore, we show how RS can reveal the differences in the biochemical composition and oxidation state of LPs extracted from a cohort of obese patients (Ob) and a control group of healthy subjects (HC). In particular, RS revealed how low-density lipoproteins (LDLs) from obese patients are enriched in triglycerides and more oxidized than those from the control group, while high-density lipoproteins (HDLs) from Ob patients were depleted in cholesterol and phospholipids. RS analysis also allowed the study of the relationship between the levels of carotenoids present in the different classes of LPs highlighting how this parameter depends on the disease severity. Overall, these results demonstrated that RS is a viable approach for quickly and effectively gaining information on LPs’ biochemical composition and oxidation state, providing an immediate measure of their quality. Besides, RS further proved the role of LPs in obesity and metabolic dysfunctions.
Introduction
Lipoproteins (LPs) are multimolecular complexes consisting of a hydrophobic lipid core covered by an envelope of amphipathic molecules, which play a crucial role in the transport of triglycerides (TGs) and cholesterol throughout the body.1 Currently, the amount of cholesterol carried by LPs is one of the main parameters used in clinical practice to assess the risk of cardiovascular events.2 In particular, an elevated level of cholesterol in low-density lipoproteins (LDL-C) is known as a significant risk factor for coronary heart disease and stroke as it causes the build-up of fatty deposits within blood vessels, reducing or blocking the flow of blood to the heart.3 In contrast, a high level of cholesterol in high-density lipoproteins (HDL-C) is commonly associated with a decreased cardiovascular disease (CVD) risk, particularly in healthy individuals with no prior history of cardiovascular events.4 This simplified view of the relationship between cholesterol levels in LPs and CVD has been recently questioned by multiple clinical trials, and a more complex U-shape relationship between HDL-C and CVD emerged, driven by the relationship between the free cholesterol transfer to high-density lipoproteins (HDLs) and the triglyceride lipolysis.5 In fact, reducing LDL-C levels by means of standard therapy with statins in primary and secondary cardiovascular prevention has significantly reduced the risk of CVD.6,7 However, negative findings from numerous randomized clinical trials have challenged the concept that elevated HDL-C levels would translate into a clinical benefit.8 The most known of these trials was ILLUMINATE which investigated the use of Torcetrapib, a cholesterol ester transfer protein (CETP) inhibitor.9 Despite the detected increase in HDL-C levels, this trial was terminated prematurely due to the increased risk of cardiovascular events and mortality in patients.9 Similar results were obtained by trials using other CETP inhibitors as Dalcetrapib and Evacetrapib.10,11 As CETP inhibitors did not show any benefit related to HDL-C elevation, the study of the correlation between the risk of cardiovascular events associated with other LP alterations gained traction.8 The importance of defining not only the quantity of cholesterol transported by LPs but also the overall “quality” of LPs, defined by their biochemical composition and oxidation state, has thus emerged as a new clinical need for improving the definition of the cardiovascular risk.12–14 It is known that in many pathological conditions, the composition of the LPs is altered.8 Obesity, a pandemic of the modern world, is intimately associated with metabolic dysfunctions. Obese subjects are characterized by high levels of serum TG and low HDL-C, mainly driven by insulin resistance and pro-inflammatory adipokines.15 Recent evidence also suggests that obesity can alter proteins’ and lipids’ composition and the function of LPs, converting these particles into dysfunctional ones.8,16 In addition, the oxidation state of LPs is known to be an essential factor in obesity and other pathological contexts, as the level of antioxidants transported by LPs seems to be linked to their functionality.14,17,18
So far, the quality analysis of the LPs has been lacking a generally accepted gold standard method, and several functional, chromatographic, and biochemical assays have been used depending on the specific biomolecule or property to be studied.19,20 In this context, RS seems to be an ideal tool for studying the quality of LPs. RS is an optical method able to provide information on the functional chemical groups present in a sample simply by its illumination with a monochromatic laser light source.21 In particular, RS is emerging as a powerful analytical tool for the analysis of lipids, with an applicative focus on the quality control of edible oils. RS can quickly provide semi-quantitative indications of the levels of carotenoids, antioxidant micronutrients, by looking at the very characteristic bands in the region of 1162 and 1525 cm−1.22,23 Furthermore, the ratiometric analysis of Raman spectra can provide multiple information on the levels of cis and trans double bonds and thus on the overall level of lipid unsaturation.24 For example, the ratio between the band at 1439 cm−1 and the one at 1665 cm−1 was suggested by multiple authors as a measure of the cis double bonds present.25,26 At the same time, trans double bonds can be inferred from the intensity of the peaks at 965 cm−1.24,27 In previous work, Ricciardi and co-workers demonstrated that RS analysis allows obtaining simultaneously multiple information about the relative contents of cholesterol, phosphatidylcholine, triglycerides, and proteins present in LPs.23 Overall, these data suggested that RS might be a suitable tool to study the quality of LPs in an easy, affordable, and fast way, providing information both on the relative amounts of biomolecules present and on their oxidation state in a single measure.
Starting from these premises, this study aimed at investigating RS's ability to provide information on the quality of the main classes of LPs: Very Low Density Lipoproteins (VLDLs), Low Density Lipoproteins (LDLs), and High Density Lipoproteins (HDLs) extracted by ultracentrifugation from the human plasma of obese patients (Ob). A matched control group of healthy subjects (HC) was also enlisted in order to compare the quality of LPs extracted, thus proving the clinical potential of RS. RS data on the biochemical composition of LPs were also compared with those obtained by dosing cholesterol, TG and phospholipids using standard biochemical assays to effectively characterize the quality of LPs in selected patients.
Experimental
Study population
From 2019 to 2022, patients referring to the O.U. of Internal Medicine and Endocrinology of ICS Maugeri (Pavia, Italy) and S.C. General Surgery, Hospital Galmarini (Tradate, Italy), were screened for eligibility. A total of 39 Ob patients with BMI ≥ 30 kg m−2 were recruited. Among the Ob patients, a subgroup had a diagnosis of further metabolic dysfunctions: 11 suffer from type two diabetes Mellitus (Ob + T2DM), and 15 suffer from metabolic syndrome (Ob + SM). Diagnosis of T2DM and SM was set according to the American Diabetes Association criteria28 and NCEP ATP III definition29 respectively. All subjects enrolled had no prior history of statin use, as they might affect the LPs composition. A control group, formed by healthy volunteers free from any significant disease [HC, n = 26] with BMI < 25 kg m−2, was also enrolled. Blood samples were collected under fasting conditions. Anthropometric parameters (body weight, BMI, waist and hip circumference), and lipid profile (total cholesterol, HDL-C, TG) were collected for each patient. LDL-C was calculated using the Friedewald formula. Glycemic profiles were collected for Ob patients to discriminate those suffering from T2DM from those not suffering from T2DM.
The study was conducted according to the principles expressed in the Declaration of Helsinki and approved by the ethical committee of ICS Maugeri IRCCS (2407 CE). Written informed consent was obtained from all the individual participants before their inclusion in the study. The main characteristics of the two groups are reported in Table 1. The characteristics of the different subgroups of Ob (Ob; Ob + SM; Ob + T2DM) are reported in the ESI as Table S1.†
Table 1 Clinical characteristics of the enrolled cohorts
Parameter |
HC |
Ob |
*p < 0.01; and **p < 0.0005. |
Number |
26 (12 F; 14 M) |
39 (17 F;22 M) |
Age, years |
43.9 ± 13.4 |
48.3± 10.1 |
Weight, kg |
66.6 ± 10.3 |
118.2 ± 24.5** |
BMI, kg m−2 |
23.0 ± 2.4 |
41.3 ± 8.1** |
Waist circumference, cm |
77.8 ± 9.6 |
124.4 ± 14.9** |
Hips circumference, cm |
97.3 ± 10.3 |
128.5 ± 17.6** |
Total cholesterol, mg dl−1 |
160.1 ± 22.9 |
194.1 ± 27.6* |
HDL-C, mg dl−1 |
64.8 ± 13.9 |
45.9 ± 10.5** |
LDL-C, mg dl−1 |
85.7 ± 16 |
136.6 ± 27.5** |
Triglycerides, mg dl−1 |
67.4 ± 19.8 |
133.9 ± 50.9** |
Glycemia, mg dl−1 |
— |
103.5 ± 23.8 |
Preparation of plasma samples
Peripheral venous blood was collected from each subject in EDTA-coated vacutainer tubes. Plasma samples were obtained by blood centrifugation at 3000 rpm for 20 min at 4 °C and aliquots were stored in sterile cryovials at −80 °C until use.
Lipoprotein isolation
LPs were isolated from plasma by ultracentrifugation using a multistep discontinuous KBr density gradient by adapting procedure 16 with reference to an “Optima Max” tabletop ultracentrifuge (Beckman Coulter).30,31 To remove albumin completely, the HDL fraction (density, 1.063–1.210 g ml−1) was subjected to a second centrifugation, as described in procedure 15 in ref. 30. After separation, LPs were dialyzed overnight in 10 mM phosphate buffered saline pH 7.4 to eliminate KBr and stored at −80 °C until use.
Lipoprotein characterization
Lipids were extracted following the Folch procedure.32 The phospholipid content was determined in the extract using the Bartlett assay33 while the levels of cholesterol and triacylglycerols in the lipid extracts were determined using commercially available kits (Scalvo Diagnostic) according to the manufacturers’ protocols.34 All the measurements were performed at least in duplicate.
Raman spectra acquisition and analysis
Raman spectra were recorded using an inVia Reflex confocal Raman microscope (Renishaw plc, Wotton-under-Edge, UK) equipped with a laser light source operating at 633 nm. The software WIRE® 5 (Renishaw, UK) was used for spectral acquisition following the protocol previously developed and described in ref. 23. Briefly, a 4 μL drop of LP suspension was dropped on the surface of Raman grade CaF2 discs (Crystran, UK) and dried for 20 min at room temperature. Raman spectra were collected using a He–Ne laser with 100% power, a 1200 L mm−1 grating and a 100× objective (Leica). Spectra were acquired in two regions: between 400 and 1800 cm−1 (low-frequency region) and between 2600 and 3200 cm−1 (high-frequency region). For each sample, five different spectra were collected at different positions of the drop.
Cosmic rays were removed using the function in the proprietary WIRE® software. The baseline is subtracted to remove the background using an Asymmetric Least Squared Smoothing Baseline algorithm (parameters: asymmetric factor = 0.002; threshold = 0.02; smoothing factor = 5; the number of iterations = 12). Normalization of the spectra was performed by dividing each value of the acquired spectra by the intensity of the peak at 1439 cm−1 (CH2 scissoring) for the low-frequency region and by the intensity of the peak at 2894 cm−1 (C–H stretching of CH2) for the high-frequency region. These peaks were chosen with reference to the CH2 group present both in lipids and proteins (main constituents of LPs) and appeared constant in the acquired dataset.
The average of the five spectra obtained from each LP extracted from subjects was considered as the final spectrum representative of the subject.
Statistical analysis of Raman bands
For the statistical analysis of data, we first verified the normal distribution by applying the Shapiro–Wilk tests to each group. Secondly, we performed an appropriate parametric [t-test] or non-parametric [Mann–Whitney test] test to compare mean values between groups depending on the normal/not normal distribution of data. To reduce the influence of noise on the data analysis, the peak intensity was defined as the average value of a band around the mentioned peak. The exact peak assignment of the bands considered is shown in the ESI (Table S1†). Statistical significance was set at p < 0.05.
Results and discussion
As reported in the literature, the structural composition of LPs is altered in many pathological conditions. RS has been extensively used to provide information on the functional chemical groups present in the sample analyzed. Thanks to this approach, we evaluate the quality of LPs (VLDLs, LDLs, and HDLs) extracted by ultracentrifugation from the plasma of Ob patients (Ob, n = 39) compared to a control group (HC, n = 26). The Ob and HC subjects were similar in age. As expected, the two groups had significant differences in all anthropometric measurements (weight, BMI, waist, and hip circumference). As well as, the total cholesterol, TG, and LDL-C were significantly higher in the Ob patients than in HC, while HDL-C levels were significantly lower (Table 1).
Raman spectra were acquired in the fingerprint region between 400 and 1800 cm−1 and in the CH region between 2600 and 3200 cm−1. The peaks at 1439 cm−1 and 2984 cm−1, relative to the CH2 groups, were selected as reference points for the spectra normalization. As the ratio between the bands of CH2 and other bands is commonly used to obtain structural information on lipids, this choice allowed us to immediately derive data about LPs unsaturation.26 Furthermore, by normalizing for a fixed point of the spectra, it was possible to avoid the interference that the highly variable bands of carotenoids have on the determination of the reference value, that occurs when other common normalization methods (such as 0–1; or vector normalization) are used, and that makes the comparison between different samples and between different classes of LPs impossible.
Fig. 1 reports the average normalized Raman spectra of LPs from Ob patients and HC and the differential spectrum obtained by subtracting Ob from HC. In all the classes of LPs studied, it was possible to identify all the peaks linked to the biochemical composition of LPs. In particular, it was possible to identify the peaks relative to cholesterol (700 cm−1), phosphatidylcholine (717 cm−1), TG (1300 cm−1) and the ratio between lipids and proteins (2851/2930 cm−1). Other peaks present are related to unsaturated lipids (960 cm−1, 1271 cm−1, 1656 cm−1) and carotenoids (1000 cm−1, 1160 cm−1 and 1525 cm−1) providing particularly informative data on the oxidation state of LPs. All the mentioned peaks were present in all the LPs considered and revealed a typical LP pattern. The peak intensity, however, differed in Ob and HC, reflecting variations in the composition between the classes of LPs and in the quality of LPs.
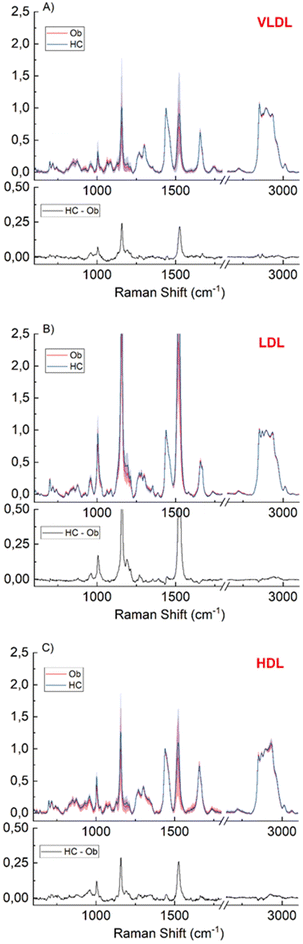 |
| Fig. 1 Raman spectra acquired in the low and high frequency regions on VLDLs (A), LDLs (B) and HDLs (C) extracted from HC [HC; n = 26] and Ob patients [Ob; n = 39] with the differential spectrum between the two groups considered. | |
Raman analysis of the LP composition
RS allowed the study of spectral differences among Ob and HC patients in the peaks related to the biochemical component present in the three classes of LPs considered (VLDLs, LDLs, and HDLs) as shown in Fig. 2.
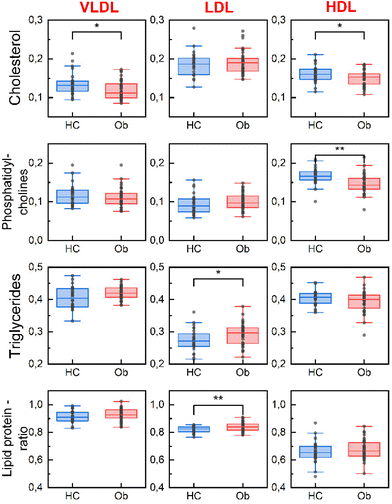 |
| Fig. 2 Box plots showing the different Raman intensities of selected biochemical related bands in Ob patients (n = 39) vs. HC (n = 26). Data are shown as box and whisker plots. Each data point represents an individual subject analyzed. Each box represents the area between the 25th and 75th percentiles (interquartile range, IQR). The lines inside the boxes represent the median values. Whiskers extend to the lowest and highest values within 1.5 times the IQR from the box. Statistical significance: *p < 0.05; **p < 0.01; and ***p < 0.0005. | |
Less intense peaks of cholesterol at 700 cm−1 were detected in the VLDLs and HDLs of Ob compared with HC. LDLs, in contrast, did not show any differences in this peak. This seems in accordance with the lower levels of HDL-C characterizing Ob and provides more information on the balance between the cholesterol transported by the different LPs.
A statistically significant difference is also observed in the peak related to phosphatidylcholine at 717 cm−1. This peak is less intense in the HDLs of the Ob patients compared to HC.
The peak relative to TG at 1300 cm−1 remains unchanged between the two groups in the VLDLs and HDLs but was found to be more intense in the LDLs of Ob compared to HC. This result was confirmed by the study of the ratio between lipids and proteins in the high frequency region related to CH2, determining an increased amount of the lipid component in LDLs.
Biochemical analysis of the LPs composition
In order to be able to confirm the structural alteration in the lipid balance identified by RS in the LDL and HDL fractions, for a subgroup of subjects, the results were compared with the one obtained using a set of biochemical assays commonly employed to quantify cholesterol, TG, and phospholipids.34 As RS does not provide information on the absolute amount of lipids present but only on their relative amount, the results obtained from the biochemical assays are expressed in terms of percentage of each component over the total amount of lipids detected. This allowed us to better understand the relationship between the different classes of lipids observed in RS and the ones obtained using the standard assays. Biochemical assays confirmed an increment of the TG detected in Ob's LDLs (Ob: 40.5% ± 7.1 vs. HC: 34.1% ± 5.3; p = 0.002) and a reduction in the percentage of cholesterol (Ob: 22.1% ± 5.1 vs. HC: 27.3% ± 9.1; p = 0.006) in the Ob's HDLs. Phospholipids did not change in the HDLs of Ob compared to HC (Fig. 3). Although some differences are thus observed between the results obtained using biochemical assays and RS, this study confirmed the actual presence of alterations in the biochemical composition of LDLs and HDLs.
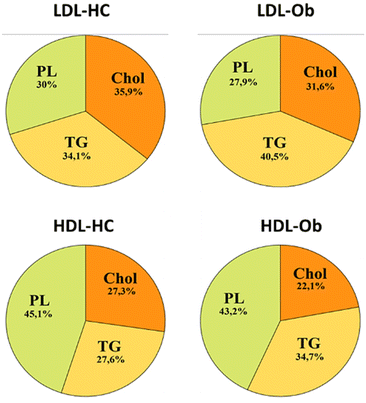 |
| Fig. 3 Mean composition of LDL (HC n = 16; Ob n = 37) and HDL (HC n = 25; Ob n = 35) as measured by using the commercially available enzymatic kits. Chol = cholesterol; TGs: triglycerides; and PLs: phospholipids. | |
The differences obtained between the phosphatidylcholine quantified at RS and the phospholipids detected by the enzymatic assay might be explained by the fact that the two methods are not based on the quantification of the same functional group, as the biochemical assay is based on the measure of the phosphorous contained herein. The RS peak at 717 cm−1 here used is instead related to the C–N+(CH3)3 bond characteristic of choline.
Finally, it must be noticed that while RS was done directly on the LPs extracted by ultracentrifugation, the biochemical assays required a further extraction of the lipids using the Folch approach, which is time-consuming and can introduce bias due to the extraction process. Besides, RS provided a simultaneous determination of all the parameters considered, while biochemical assays were required to perform a specific assessment for each component studied, resulting in longer times.
Raman analysis of LPs oxidation
The oxidation state of LPs is also emerging as an essential factor in pathological contexts as the level of antioxidants in LPs seems to be linked to their functionality.14,17,18 A detailed analysis of the peaks related to the oxidation state of LPs, relative to lipid unsaturation (960 cm−1, 1271 cm−1, 1656 cm−1) and carotenoids (1525 cm−1) confirmed the presence of significant differences between the two groups (Fig. 4). The LDLs and HDLs of Ob were more oxidized and carried a lower amount of micronutrients (carotenoids).
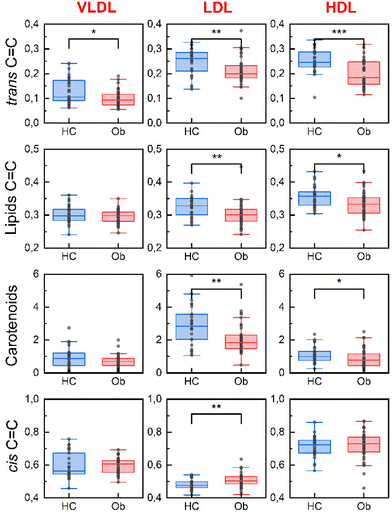 |
| Fig. 4 Box plots showing the different Raman intensities of selected oxidation related bands in Ob patients (n = 39) vs. HC (n = 26). Data are shown as box and whisker plots. Each data point represents an individual subject analyzed. Each box represents the area between the 25th and 75th percentiles (interquartile range, IQR). The lines inside the boxes represent the median values. Whiskers extend to the lowest and highest values within 1.5 times the IQR from the box. Statistical significance: *p < 0.05; **p < 0.01; and ***p < 0.0005. | |
Ob LDL and HDL particles showed less intense peaks of C
C lipids at 1271 cm−1 compared to HC. Similarly, a less intense trans C
C fatty acid peak at 960 cm−1 was detected in all the LPs classes of Ob compared with HC. In contrast, the peak of cis C
C fatty acids at 1656 cm−1 increased in the LDLs of Ob patients compared to HC, indicating a shift in the lipid composition.35
Carotenoid peaks are more intense in LDLs compared to the other classes of LPs confirming their antioxidant role described in the literature.36 It is however also known that HDLs are involved in the transport of these anti-oxidants from the liver to other peripheral tissues such as the retina37 and the clinical relevance of this parameter must still be fully understood. The levels of carotenoids (here measured by RS as a peak at 1525 cm−1) were found to be lower in the LDLs and HDLs of Ob compared to HC.
As mentioned, by normalizing the data for the peak relative to the CH2 group at 1439 cm−1 it is possible to infer also absolute information on the number of double bonds contained herein. While it is not possible by RS to obtain a complete profile of the unsaturation of the different classes of lipids, the marked presence of multiple peaks relative to lipid unsaturation suggests that most of the lipids contained in LPs had some level of unsaturation.26,38
It is also remarkably observed that while the level of double bonds measured at 1271 cm−1 is rather constant in all the LPs, LDLs showed a marked lower peak of cis double bonds at 1656 cm−1 when compared with VLDLs and HDLs,39 suggesting that cholesterol esters might be relatively more saturated than TG.
Correlation between the composition of LPs and the disease state of obese subjects
The RS analysis of LPs allowed us also to study the relationship between the levels of specific biomolecules present in the different classes of LPs and to infer information on the disease state. Some Ob subjects enrolled in the study also suffered from further metabolic dysfunctions: metabolic syndrome (Ob + SM; n = 15) and type 2 diabetes mellitus (Ob + T2DM; n = 11). The clinical characteristics of the different subpopulations of Ob patients are reported in Table S2.†
We thus looked at the relationship between the biochemical components characterizing the different classes of LPs, all acquired with the same parameters previously described, in all the subpopulations considered (Ob; Ob + SM; Ob + T2DM), and in the controls. The results showed a correlation between the carotenoids in LDLs and HDLs.
However, this relationship's slope changed and decreased as the disease progressed toward more compromised metabolic dysfunctions (Fig. 5). The intensities observed of the relationship between carotenoids in LDLs and HDLs have a slope of 1.65 ± 0.35 in HC; 1.45 ± 0.28 in Ob; 0.98 ± 0.34; and only 0.68 in Ob + T2DM, with a clinical progression that is not immediately noticeable just by looking at the levels of carotenoids present in the two classes of LPs. This result agrees with the literature, supporting a dysfunctional LP's proinflammatory/prooxidant role in different pathological conditions such as SM and T2DM.14,40–42
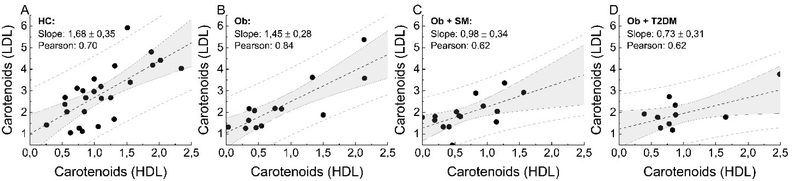 |
| Fig. 5 Intensity of the peak at 1525 cm−1 relative to carotenoids present in the LDLs and HDLs of HC (A); Ob (B); Ob + SM (C) and Ob + T2DM (D). Each dot represents one subject; black dashed lines represent the best linear fit of the data of each plot; the grey area represents the 95% confidence bands of the fit; the area between the dashed grey lines represents the 95% prediction bands for new data points. | |
Conclusions
Recently, to improve the definition of the cardiovascular risk, the importance of defining not only the quantity of LPs but also their “quality” has emerged.
In this work, we demonstrated the suitability of RS, combined with a ratiometric analysis of spectra, to obtain multiple information on the profiles of the main classes of LPs in a fast and easy way for a better comprehension of different metabolic conditions. LPs were extracted by ultracentrifugation from the plasma of Ob and HC. RS was used to identify immediately and without the need to use any further preparation or extraction procedure an altered biochemical composition and oxidation state in LPs from the Ob group. This provides an immediate advantage of RS over the traditionally used biochemical assays. In particular, RS highlighted that Ob's LDLs had marked lower levels of carotenoids and unsaturated lipids, while Ob's HDLs were characterized by a different balance in the lipid components (cholesterol, phospholipids, and lipid/protein ratio).
Overall, this work unravels the effectiveness of the use of RS as a tool for the study of the quality of LPs and paves the road toward a more comprehensive understanding of the role of LPs in metabolic dysfunctions and a better definition of the CVD risk.
Author contributions
RC, FC, and CM: study concept and design; FM and AR: selection and recruitment of subjects included in the study; AB: sample collection and preparation; AB, RC, and CM: statistical analysis; AB: acquisition of Raman spectra; AB, SM, RC, CM, FM, and MT: analysis and interpretation of data; AB and CM contributed to manuscript writing and figure preparation. FM, RC, SM, MT, and FC contributed to manuscript writing, editing, and critical review. All authors have given approval to the final version of the manuscript.
Conflicts of interest
The authors declare that they have no known competing financial interests or personal relationships that could have appeared to influence the work reported in this paper. All authors have given approval to the final version of the manuscript.
Acknowledgements
This work was partially funded by the Italian Ministry of Health, Ricerca Corrente 2021. The graphical abstract was composed using BioRender. Project funded under the National Recovery and Resilience Plan (NRRP), Mission 4 Component 2 Investment 1.3 - Call for tender No. 341 of 15 March 2022 of Italian Ministry of University and Research funded by the European Union – NextGenerationEU; Award Number: Project code PE00000003, Concession Decree No. 1550 of 11 October 2022 adopted by the Italian Ministry of University and Research, CUP D93C22000890001, Project title “ON Foods - Research and innovation network on food and nutrition Sustainability, Safety and Security – Working ON Foods”.
References
-
K. R. Feingold, Introduction to Lipids and Lipoproteins, in Endotext, ed. K. R. Feingold, B. Anawalt, A. Boyce, G. Chrousos, W. W. de Herder, K. Dhatariya, K. Dungan, J. M. Hershman, J. Hofland, S. Kalra, G. Kaltsas, C. Koch, P. Kopp, M. Korbonits, C. S. Kovacs, W. Kuohung, B. Laferrère, M. Levy, E. A. McGee, R. McLachlan, J. E. Morley, M. New, J. Purnell, R. Sahay, F. Singer, M. A. Sperling, C. A. Stratakis, D. L. Trence and D. P. Wilson, MDText.com, Inc., South Dartmouth (MA), 2000 Search PubMed.
- M. R. Langlois, B. G. Nordestgaard, A. Langsted, M. J. Chapman, K. M. Aakre, H. Baum, J. Borén, E. Bruckert, A. Catapano, C. Cobbaert, P. Collinson, O. S. Descamps, C. J. Duff, A. von Eckardstein, A. Hammerer-Lercher, P. R. Kamstrup, G. Kolovou, F. Kronenberg, S. Mora, K. Pulkki, A. T. Remaley, N. Rifai, E. Ros, S. Stankovic, A. Stavljenic-Rukavina, G. Sypniewska, G. F. Watts, O. Wiklund and P. Laitinen, Quantifying Atherogenic Lipoproteins for Lipid-Lowering Strategies: Consensus-Based Recommendations from EAS and EFLM, Clin. Chem. Lab. Med., 2020, 58(4), 496–517, DOI:10.1515/cclm-2019-1253.
- J. Borén, M. J. Chapman, R. M. Krauss, C. J. Packard, J. F. Bentzon, C. J. Binder, M. J. Daemen, L. L. Demer, R. A. Hegele, S. J. Nicholls, B. G. Nordestgaard, G. F. Watts, E. Bruckert, S. Fazio, B. A. Ference, I. Graham, J. D. Horton, U. Landmesser, U. Laufs, L. Masana, G. Pasterkamp, F. J. Raal, K. K. Ray, H. Schunkert, M.-R. Taskinen, B. van de Sluis, O. Wiklund, L. Tokgozoglu, A. L. Catapano and H. N. Ginsberg, Low-Density Lipoproteins Cause Atherosclerotic Cardiovascular Disease: Pathophysiological, Genetic, and Therapeutic Insights: A Consensus Statement from the European Atherosclerosis Society Consensus Panel, Eur. Heart J., 2020, 41(24), 2313–2330, DOI:10.1093/eurheartj/ehz962.
- The Emerging Risk Factors Collaboration, Major Lipids, Apolipoproteins, and Risk of Vascular Disease, J. Am. Med. Assoc., 2009, 302(18), 1993, DOI:10.1001/jama.2009.1619.
- H. Taheri, K. B. Filion, S. B. Windle, P. Reynier and M. J. Eisenberg, Cholesteryl Ester Transfer Protein Inhibitors and Cardiovascular Outcomes: A Systematic Review and Meta-Analysis of Randomized Controlled Trials, Cardiology, 2020, 145(4), 236–250, DOI:10.1159/000505365.
- S. E. Nissen, S. J. Nicholls, I. Sipahi, P. Libby, J. S. Raichlen, C. M. Ballantyne, J. Davignon, R. Erbel, J. C. Fruchart, J.-C. Tardif, P. Schoenhagen, T. Crowe, V. Cain, K. Wolski, M. Goormastic, E. M. Tuzcu and ASTEROID Investigators for the, Effect of Very High-Intensity Statin Therapy on Regression of Coronary Atherosclerosis: The ASTEROID Trial, J. Am. Med. Assoc., 2006, 295(13), 1556, DOI:10.1001/jama.295.13.jpc60002.
- K. M. Ali, A. Wonnerth, K. Huber and J. Wojta, Cardiovascular Disease Risk Reduction by Raising HDL Cholesterol - Current Therapies and Future Opportunities: Cardiovascular Risk and HDL Cholesterol, Br. J. Pharmacol., 2012, 167(6), 1177–1194, DOI:10.1111/j.1476-5381.2012.02081.x.
- S. T. Chiesa and M. Charakida, High-Density Lipoprotein Function and Dysfunction in Health and Disease, Cardiovasc. Drugs Ther., 2019, 33(2), 207–219, DOI:10.1007/s10557-018-06846-w.
- P. J. Barter, M. Caulfield, M. Eriksson, S. M. Grundy, J. J. P. Kastelein, M. Komajda, J. Lopez-Sendon, L. Mosca, J.-C. Tardif, D. D. Waters, C. L. Shear, J. H. Revkin, K. A. Buhr, M. R. Fisher, A. R. Tall and B. Brewer, Effects of Torcetrapib in Patients at High Risk for Coronary Events, N. Engl. J. Med., 2007, 357(21), 2109–2122, DOI:10.1056/NEJMoa0706628.
- G. G. Schwartz, A. G. Olsson, M. Abt, C. M. Ballantyne, P. J. Barter, J. Brumm, B. R. Chaitman, I. M. Holme, D. Kallend, L. A. Leiter, E. Leitersdorf, J. J. V. McMurray, H. Mundl, S. J. Nicholls, P. K. Shah, J.-C. Tardif and R. S. Wright, Effects of Dalcetrapib in Patients with a Recent Acute Coronary Syndrome, N. Engl. J. Med., 2012, 367(22), 2089–2099, DOI:10.1056/NEJMoa1206797.
- A. M. Lincoff, S. J. Nicholls, J. S. Riesmeyer, P. J. Barter, H. B. Brewer, K. A. A. Fox, C. M. Gibson, C. Granger, V. Menon, G. Montalescot, D. Rader, A. R. Tall, E. McErlean, K. Wolski, G. Ruotolo, B. Vangerow, G. Weerakkody, S. G. Goodman, D. Conde, D. K. McGuire, J. C. Nicolau, J. L. Leiva-Pons, Y. Pesant, W. Li, D. Kandath, S. Kouz, N. Tahirkheli, D. Mason and S. E. Nissen, Evacetrapib and Cardiovascular Outcomes in High-Risk Vascular Disease, N. Engl. J. Med., 2017, 376(20), 1933–1942, DOI:10.1056/NEJMoa1609581.
- K.-H. Cho, The Current Status of Research on High-Density Lipoproteins (HDL): A Paradigm Shift from HDL Quantity to HDL Quality and HDL Functionality, Int. J. Mol. Sci., 2022, 23(7), 3967, DOI:10.3390/ijms23073967.
- H. Thakkar, V. Vincent, A. Sen, A. Singh and A. Roy, Changing Perspectives on HDL: From Simple Quantity Measurements to Functional Quality Assessment, J. Lipids, 2021, 2021, 5585521, DOI:10.1155/2021/5585521.
- A. Bonizzi, G. Piuri, F. Corsi, R. Cazzola and S. Mazzucchelli, HDL Dysfunctionality: Clinical Relevance of Quality Rather Than Quantity, Biomedicines, 2021, 9(7), 729, DOI:10.3390/biomedicines9070729.
- L. Montefusco, F. D’Addio, C. Loretelli, M. Ben Nasr, M. Garziano, A. Rossi, I. Pastore, L. Plebani, M. E. Lunati, A. M. Bolla, M. D. Porta, G. Piuri, F. Rocchio, A. Abdelsalam, E. Assi, M. Barichella, A. Maestroni, V. Usuelli, L. Loreggian, F. Muzio, G. V. Zuccotti, R. Cazzola and P. Fiorina, Anti-Inflammatory Effects of Diet and Caloric Restriction in Metabolic Syndrome, J. Endocrinol. Invest., 2021, 44(11), 2407, DOI:10.1007/s40618-021-01547-y.
- M. Zocchi, M. Della Porta, F. Lombardoni, R. Scrimieri, G. V. Zuccotti, J. A. Maier and R. Cazzola, A Potential Interplay between HDLs and Adiponectin in Promoting Endothelial Dysfunction in Obesity, Biomedicines, 2022, 10(6), 1344, DOI:10.3390/biomedicines10061344.
- A. V. Poznyak, N. G. Nikiforov, A. M. Markin, D. A. Kashirskikh, V. A. Myasoedova, E. V. Gerasimova and A. N. Orekhov, Overview of OxLDL and Its Impact on Cardiovascular Health: Focus on Atherosclerosis, Front. Pharmacol., 2021, 11, 613780, DOI:10.3389/fphar.2020.613780.
- F. Ito and T. Ito, High-Density Lipoprotein (HDL) Triglyceride and Oxidized HDL: New Lipid Biomarkers of Lipoprotein-Related Atherosclerotic Cardiovascular Disease, Antioxidants, 2020, 9(5), 362, DOI:10.3390/antiox9050362.
- A. Hafiane and J. Genest, High Density Lipoproteins: Measurement Techniques and Potential Biomarkers of Cardiovascular Risk, BBA Clin., 2015, 3, 175–188, DOI:10.1016/j.bbacli.2015.01.005.
- Y. Hirowatari, H. Yoshida, H. Kurosawa, K. Doumitu and N. Tada, Measurement of Cholesterol of Major Serum Lipoprotein Classes by Anion-Exchange HPLC with Perchlorate Ion-Containing Eluent, J. Lipid Res., 2003, 44(7), 1404–1412, DOI:10.1194/jlr.D300003-JLR200.
- H. J. Butler, L. Ashton, B. Bird, G. Cinque, K. Curtis, J. Dorney, K. Esmonde-White, N. J. Fullwood, B. Gardner, P. L. Martin-Hirsch, M. J. Walsh, M. R. McAinsh, N. Stone and F. L. Martin, Using Raman Spectroscopy to Characterize Biological Materials, Nat. Protoc., 2016, 11(4), 664–687, DOI:10.1038/nprot.2016.036.
- R. Withnall, B. Z. Chowdhry, J. Silver, H. G. M. Edwards and L. F. C. de Oliveira, Raman Spectra of Carotenoids in Natural Products, Spectrochim. Acta, Part A, 2003, 59(10), 2207–2212, DOI:10.1016/S1386-1425(03)00064-7.
- A. Ricciardi, G. Piuri, M. D. Porta, S. Mazzucchelli, A. Bonizzi, M. Truffi, M. Sevieri, R. Allevi, F. Corsi, R. Cazzola and C. Morasso, Raman Spectroscopy Characterization of the Major Classes of Plasma Lipoproteins, Vib. Spectrosc., 2020, 109, 103073, DOI:10.1016/j.vibspec.2020.103073.
- B. Muik, B. Lendl, A. Molina-Díaz and M. J. Ayora-Cañada, Direct Monitoring of Lipid Oxidation in Edible Oils by Fourier Transform Raman Spectroscopy, Chem. Phys. Lipids, 2005, 134(2), 173–182, DOI:10.1016/j.chemphyslip.2005.01.003.
- J. R. de Wolf, A. Lenferink, A. Lenferink, C. Otto and N. Bosschaart, Evaluation of the Changes in Human Milk Lipid Composition and Conformational State with Raman Spectroscopy during a Breastfeed, Biomed. Opt. Express, 2021, 12(7), 3934, DOI:10.1364/BOE.427646.
- L. E. Jamieson, A. Li, K. Faulds and D. Graham, Ratiometric Analysis Using Raman Spectroscopy as a Powerful Predictor of Structural Properties of Fatty Acids, R. Soc. Open Sci., 2018, 5(12), 181483, DOI:10.1098/rsos.181483.
- H. Yang and J. Irudayaraj, Comparison of Near-Infrared, Fourier Transform-Infrared, and Fourier Transform-Raman Methods for Determining Olive Pomace Oil Adulteration in Extra Virgin Olive Oil, J. Am. Oil Chem. Soc., 2001, 78(9), 889, DOI:10.1007/s11746-001-0360-6.
- American Diabetes Association, Diagnosis and Classification of Diabetes Mellitus, Diabetes Care, 2014, 37(Supplement_1), S81–S90, DOI:10.2337/dc14-S081.
- Expert Panel on Detection, Evaluation, and Treatment of High Blood Cholesterol in Adults. Executive Summary of the Third Report of the National Cholesterol Education Program (NCEP) Expert Panel on Detection, Evaluation, and Treatment of High Blood Cholesterol in Adults (Adult Treatment Panel III), JAMA, J. Am. Med. Assoc., 2001, 285(19), 2486–2497, DOI:10.1001/jama.285.19.2486.
-
B. H. Chung, J. P. Segrest, M. J. Ray, J. D. Brunzell, J. E. Hokanson, R. M. Krauss, K. Beaudrie and J. T. Cone, [8] Single Vertical Spin Density Gradient Ultracentrifugation, in Methods in Enzymology, Elsevier, 1986, vol. 128, pp. 181–209. DOI:10.1016/0076-6879(86)28068-4.
- R. Cazzola, G. Cervato and B. Cestaro, Variability in α-Tocopherol Antioxidant Activity in the Core and Surface Layers of Low-and High-Density Lipoproteins, J. Nutr. Sci. Vitaminol., 1999, 45(1), 39–48, DOI:10.3177/jnsv.45.39.
- J. Folch, M. Lees and G. H. Sloane Stanleya, A Simple method for the isolation and purification of total lipides from animal tissues, J. Biol. Chem., 1957, 226(1), 497–509 CrossRef CAS PubMed.
- G. R. Bartlett, Phosphorus Assay in Column Chromatography, J. Biol. Chem., 1959, 234(3), 466–468 CrossRef CAS PubMed.
- R. Cazzola, E. Cassani, M. Barichella and B. Cestaro, Impaired Fluidity and Oxidizability of HDL Hydrophobic Core and Amphipathic Surface in Dyslipidemic Men, Metabolism, 2013, 62(7), 986–991, DOI:10.1016/j.metabol.2013.01.012.
- C. Chatgilialoglu, C. Ferreri, M. Melchiorre, A. Sansone and A. Torreggiani, Lipid Geometrical Isomerism: From Chemistry to Biology and Diagnostics, Chem. Rev., 2014, 114(1), 255–284, DOI:10.1021/cr4002287.
- N. Singh, N. Singh, S. K. Singh, A. K. Singh, D. Kafle and N. Agrawal, Reduced Antioxidant Potential of LDL Is Associated With Increased Susceptibility to LDL Peroxidation in Type II Diabetic Patients, Int. J. Endocrinol. Metab., 2012, 10(4), 582–586, DOI:10.5812/ijem.5029.
- E. H. Harrison, Mechanisms of Transport and Delivery of Vitamin A and Carotenoids to the Retinal Pigment Epithelium, Mol. Nutr. Food Res., 2019, 63(15), 1801046, DOI:10.1002/mnfr.201801046.
- K. Czamara, K. Majzner, M. Z. Pacia, K. Kochan, A. Kaczor and M. Baranska, Raman Spectroscopy of Lipids: A Review: Raman Spectroscopy of Lipids, J. Raman Spectrosc., 2015, 46(1), 4–20, DOI:10.1002/jrs.4607.
- M. Melchiorre, A. Torreggiani, C. Chatgilialoglu and C. Ferreri, Lipid Markers of “Geometrical” Radical Stress: Synthesis of Monotrans Cholesteryl Ester Isomers and Detection in Human Plasma, J. Am. Chem. Soc., 2011, 133(38), 15184–15190, DOI:10.1021/ja205903h.
- C. E. Kosmas, D. Silverio, A. Sourlas, P. D. Montan and E. Guzman, Dysfunctional High-Density Lipoprotein and Atherogenesis, Vessel Plus, 2019, 3, 2, DOI:10.20517/2574-1209.2018.79.
- P. Holvoet, D. D. Keyzer and D. Jacobs Jr., Oxidized LDL and the Metabolic Syndrome, Future Lipidol., 2008, 3(6), 637–649, DOI:10.2217/17460875.3.6.637.
- N. Hui, P. J. Barter, K.-L. Ong and K.-A. Rye, Altered HDL Metabolism in Metabolic Disorders: Insights into the Therapeutic Potential of HDL, Clin. Sci., 2019, 133(21), 2221–2235, DOI:10.1042/CS20190873.
|
This journal is © The Royal Society of Chemistry 2023 |