DOI:
10.1039/C9OB01559K
(Review Article)
Org. Biomol. Chem., 2020,
18, 606-617
Recent trends in catalytic sp3 C–H functionalization of heterocycles†
Received
12th July 2019
, Accepted 18th September 2019
First published on 8th January 2020
Abstract
Heterocycles are a ubiquitous substructure in organic small molecules designed for use in materials and medicines. Recent work in catalysis has focused on enabling access to new heterocycle structures by sp3 C–H functionalization on alkyl side-chain substituents—especially at the heterobenzylic position—with more than two hundred manuscripts published just within the last ten years. Rather than describing in detail each of these reports, in this mini-review we attempt to highlight gaps in existing techniques. A semi-quantitative overview of ongoing work strongly suggests that several specific heterocycle types and bond formations outside of C–C, C–N, and C–O have been almost completely overlooked.
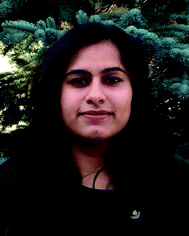 Milanpreet Kaur | Milanpreet Kaur was born in Yamuna Nagar (Haryana), India. She completed both her B.Sc. (2015) and M.Sc. (2017) in Chemistry at Hindu College, University of Delhi. Currently, she is completing her graduate studies under the supervision of Prof. Jeffrey Van Humbeck, Department of Chemistry, University of Calgary, Canada. Her research interest is focused on designing a new catalytic system for site selective C–H functionalization. |
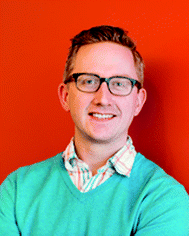 Jeffrey F. Van Humbeck | Jeff Van Humbeck is a native of Calgary, Alberta, Canada, where he completed his undergraduate education at the University of Calgary before venturing south of the 49th parallel. His PhD was conducted under the supervision of Prof. David W. C. MacMillan at Princeton University, studying the mechanism of interaction between organocatalytic reactive intermediates and transition metals. Next, he changed fields and coasts, joining the laboratory of Prof. Jeffrey R. Long at the University of California, Berkeley, where he investigated the use of porous organic polymers in gas purification, nuclear fuel processing, and as ion-conducting solid electrolytes. Jeff began his independent career at MIT in July 2014, where his group made their first discoveries in site-selective C–H functionalization. In January 2018, Jeff returned to Canada to take up a position at the University of Calgary. |
Introduction
Scope and goals of this mini-review
Herein, we present an overview of 206 recent papers (2010 or later) that encompass 4904 discrete catalytic reactions.1–206 The reader may be curious to know what inspired this undertaking, and why this particular set of publications was chosen. One area of our laboratory investigates copper-8 and iron-catalyzed26 sp3 C–H functionalization of azaheterocycle substrates. After establishing our particular mechanistic approach with some initial success, the natural question arose: what next?
To help guide our future work, we wanted a semi-quantitative overview of ongoing work in the area of sp3 C–H functionalization of heterocycles, broadly defined. We were most interested to learn if specific reaction types or substrate classes are currently poorly served by existing technology. While numerous high-quality reviews on C–H functionalization have been published recently,207–214 such resources are naturally focused on what has been achieved and not what is missing. These are important resources, but did not answer our key question.
Our strategy to create an overview that would highlight current gaps was this: first, we divided the major topics of interest—reaction mechanism, bond formation, and heterocycle type—into predefined categories that are listed within. Next, we set out to find a broadly representative set of publications we could use to roughly enumerate how thoroughly any particular [mechanism, bond formation, and heterocycle] combination had been investigated. This was sensibly achieved with a broad SciFinder™ search, followed by reasonable filtering of the results as described in Fig. 1. We first looked for any C–H functionalization of an alkyl group attached to a heterocycle that fit three criteria: (i) having a publication year or 2010 or later, (ii) in an academic journal, and which (iii) has a catalyst as recognized by the search tools. Our search initially focused on only carbon substituents at the position adjacent to the heterocycle, to avoid massive quantities of trivial reactions such as the oxidation of an alcohol to an aldehyde. However, if a manuscript featured a mixture of examples, where in some cases all ‘R’ substituents were carbon and in other cases some ‘R’ substituents were heteroatoms, both were included in the statistics we detail below.
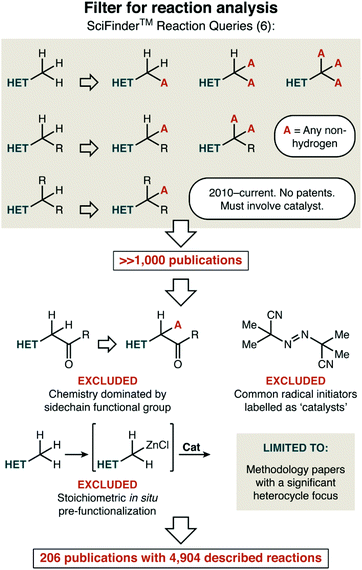 |
| Fig. 1 Data gathering strategy using the SciFinder™ database. HET = any heterocycle. In our search, R = any carbon. However, we did not exclude results where R = heteroatom, if those reactions were discovered by coincidence. | |
This search delivered a number of publications that was well beyond our ability to analyse. Upon slightly closer inspection, though, there were a number of further filters that could be applied so that the focus would truly rest on recent catalyst development in C–H functionalization. First, we excluded transformations that are much better described as another reaction type. For example, we decided not to consider aldol reactions of ketones that simply happen to have heterocycle substituents. Similarly, we felt that publications reporting traditional radical reactions (e.g. halogenation using N-bromosuccinimide) using common radical initiators as catalysts (e.g. AIBN; azobisisobutyrylnitrile) do not reflect ongoing work in catalyst development in this area. Next, we also excluded reactions that involved stoichiometric in situ activation of the heterocycle (e.g. such as deprotonation with a strong base) prior to a catalytic transformation taking place.
Finally, we also restricted our analysis to manuscripts that had a significant heterocycle focus and/or scope. This excluded target-oriented synthesis that might feature a single reaction of the type we were interested in, and most mechanistic studies of reactions in this class were also excluded. More difficult decisions had to be made around broadly applicable C–H functionalization chemistry. Should a paper that features fifty all-carbon alkyl aromatic substrates and only five alkyl heterocycles be considered? Although a rather qualitative filter, we gave our honest best effort to answer that exact question: does this publication have a significant heterocycle focus and/or scope. These features—focus and scope—do not necessarily occur together. In some cases, the focus of the paper was on other substrate classes, but the sheer number of heterocycles reported justified its inclusion. In others, while only a handful of substrates were reported, the focus was evident as every example was a heterocycle.
What results is a set of 206 papers that is by no means exhaustive and was not chosen by random sampling. Chemical reaction indexes such as SciFinder™ also do not perfectly categorize reactions. We can say this with certainty, as we discovered that our own publications did not appear in our initial searches. As such, we do not suggest taking any of the conclusions below as being decimal-point accurate.
By any reasonable count, however, the 4904 unique reactions contained in these papers represent a significant fraction of academic work done in the last decade. In any place where we think that our publication-selection strategy has falsely resulted in interesting trends, we have tried to alert the reader. Most importantly, we believe that some of the trends observed when analysing these data are so suggestive that they are almost unquestionably significant. There are clearly areas of heterocycle space that need much better service from catalysis. We hope that this mini-review will serve to encourage laboratories other than our own to address some of these challenges.
Results and discussion
Manuscript-level analysis: reaction type
The most important deconstruction of the data we gathered is that based on the heterocycle structure, which is presented later on. To appreciate some of our structure-based analysis, a starting set of expectations is necessary. First, we present some manuscript-level analysis of the paper set that can provide the necessary context for structure-based analysis.
As shown in Fig. 2, we classified papers based on the bond formation pursued, and further sub-divided based on the structure of the reaction partner. Carbon–carbon bond formations predominate, comprising 145 of 206 reports. Carbon–nitrogen and carbon–oxygen bonds are described at a relatively similar rate—27 and 22 times, respectively—with both being reported more often than all other types of bonds combined (i.e. ‘C-Other’).
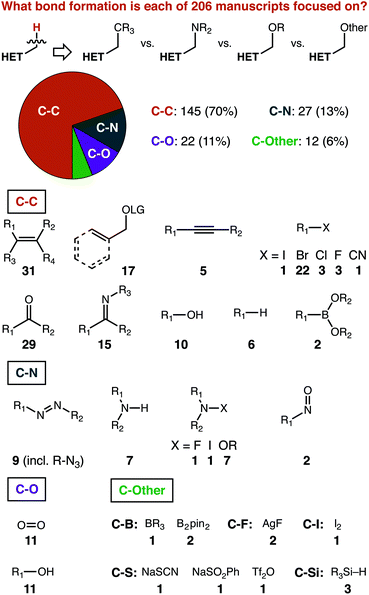 |
| Fig. 2 Division of manuscripts by the type of bond formed, further sub-divided by the chemical structure of the reaction partner. HET = any heterocycle. OLG = a competent leaving group connected through a C–O bond, such as acetate. pin = pinacolato. Tf = trifluoromethanesulfonyl. Bolded numbers refer to the number of manuscripts published where the shown structure was the most common reaction partner. | |
To us, the relative ranking of C–C, C–N, and C–O bonds makes sense for a specific reason. The sub-division based on the reaction partner shows that there is simply a much greater diversity of possible coupling partners that can be used to make C–C bonds, as compared to C–N or C–O. Carbon–carbon multiple bonds, whether of an alkene, alkyne, or activated with an oxygen-based leaving group (both benzylic and allylic), represent almost a quarter of the papers in our set (53 of 206). The carbon–heteroatom double bonds of carbonyls and imines have been explored to nearly the same extent (44 of 206). Carbon–bromine bonds are ideal substrates for transition-metal catalysed pathways (22 of 206), with a variety of other partners each contributing a handful of further examples. Reagents for carbon–nitrogen and carbon–oxygen bond formation are dominated by the element/element multiple bonded species (i.e. N
N and O
O, 20 of 49) and the element-hydrogen bond (i.e. R2N–H and RO–H, 18 of 49).
Arguably, then, the relative lack of other bond forming processes reported is surprising. The diversity of possible coupling partners to make C–X bonds is certainly greater than that incorporated into our data set.
Manuscript-level analysis: reaction mechanism
For a similar reason, having an idea of the distribution of mechanisms that were reported within our data set is important for understanding structure-based analysis. Our definitions for each mechanism type are presented in Fig. 3, and the results of this analysis are presented in Fig. 4. We define mechanisms according to the following definitions. ‘Organometallic’ feature metal–carbon bonds, either on the heterocycle or on the reaction partner it encounters. ‘Lewis Acid’ and ‘Brønsted Acid’ encompasses heteroatom coordination of either the heterocycle, or its reaction partner, with the difference between them being the traditional definition of a Brønsted acid being a proton exclusively. ‘Redox’ catalysts can engage in single electron transfer with either the heterocycle or its reaction partner, or can include two-electron redox processes, as would be demonstrated by the halogen redox cycle shown in Fig. 3.93 We used ‘Organocatalyst’ to encompass any organic catalyst not acting by a classical Brønsted acid or base pathway (e.g. enamine/iminium catalysis, counterion catalysis, etc.). ‘Radical’ catalysts act to create a carbon-centered radical on the sp3 functionalized carbon of the heterocycle. Brønsted base catalysts are as classically defined. A handful of manuscripts leveraged multiple/synergistic catalyst pathways and could not be assigned to only one of these groups.
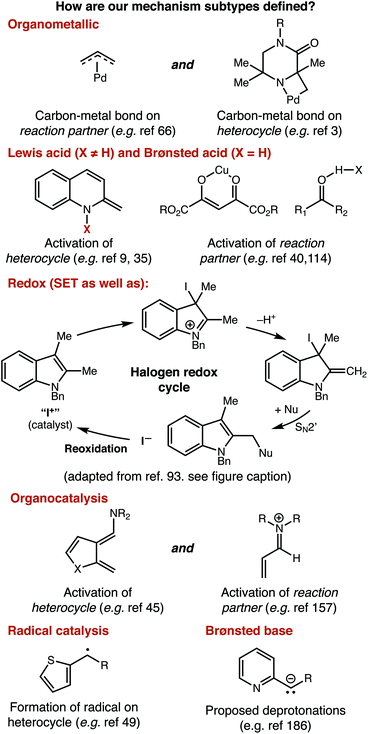 |
| Fig. 3 Definition of mechanisms as used throughout this manuscript. An abbreviated mechanistic cycle for halogen redox processes is adapted with permission from ref. 93. Copyright 2018 American Chemical Society. | |
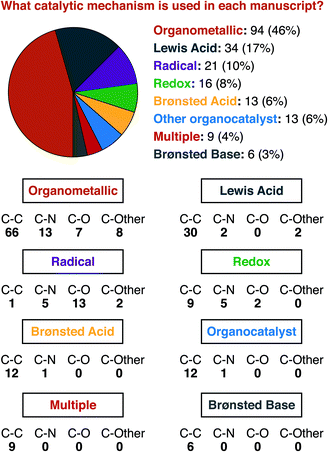 |
| Fig. 4 Division of manuscripts by the reported mechanism, further divided by bond formation. The numbers in bold under each bond type refer to the number of manuscripts published focusing on that transformation. | |
Organometallic mechanisms represent nearly half of our set. We ascribe this behaviour to two factors. First, as we will show below, one major class of heterocycle substrates is serviced almost entirely by organometallic pathways, which certainly affects the manuscript-level analysis. Second, organometallic pathways can be seen as being the most versatile mechanism class. Five of the seven other possible mechanisms (i.e. Lewis Acid, Brønsted Acid, Brønsted Base, Organocatalyst, and Multiple) deliver predominantly carbon–carbon bonds. 69 of 75 manuscripts using these mechanisms deliver C–C bonds. Radical mechanisms, conversely, deliver almost exclusively carbon–heteroatom bonds (20 of 21). Organometallic mechanisms have delivered essentially any type of desired new bond, across a wide range of substrate types, and hence have contributed the greatest share of current work.
Structure-level analysis: reaction distribution by structure type
Before any counting began, we divided all heterocycles into eight groups, with no expectation as to how common they would be in the data (Fig. 5). The first division was by the ring size and aromatic nature: 6-membered aromatic vs. 5-membered aromatic vs. all other aromatics and saturated. Both 6- and 5-membered rings were sub-divided into 3 sections each. 6-Membered rings were divided based on whether the C–H bond that is broken is in ‘resonant’ communication with the heteroatoms in the ring system. That is: if an arbitrary reactive intermediate (i.e. anion, cation, radical) were placed on the sp3 side-chain, could resonance structures be drawn that place this reactive intermediate on any of the heteroatoms in the ring system? By this definition, 4-alkylpyridines are ‘6-Resonant’, while 3-alkylpyridines are ‘6-Non-Resonant’. We considered extended heterocycles to be in the same category as their parent heterocycle: 2-alkylpyridines and 2-alkylquinolines are both considered ‘6-Resonant’. We expected that 8-substituted quinolines (and related structures) specifically deserved their own category, given their widespread use as model substrates for method development. A similar split was made for 5-membered rings. 2-Alkylimidazole would be considered ‘5-Resonant’, while 4-alkylimidazole would be considered ‘5-Non-Resonant’. We expected that five-membered rings featuring a single heteroatom (e.g. furan, thiophene, indole, etc.) would have unique chemistry and deserved their own category. If the C–H bond being broken is found on the all-carbon ring of an extended heterocycle (e.g. 5-, 6-, or 7-alkylquinolines), then that was to be considered an example of ‘Remote’ C–H functionalization. All other heterocycles encountered were grouped into a final category.
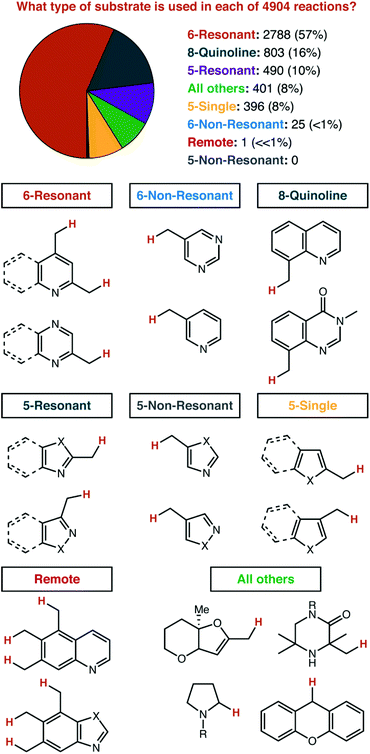 |
| Fig. 5 Classification of heterocycles according to the ring structure and position of alkyl substitution. | |
We found the results to be striking. Three of our eight pre-set categories were essentially absent from 206 manuscripts and 4904 reactions. Zero examples of five-membered rings functionalized at a non-resonant position were observed, other than reactions listed as ‘trace’ yield, which were not included. Only one single example of remote functionalization was observed as a major product (i.e. excluding the minor component of product mixtures) and is shown in Fig. 6.
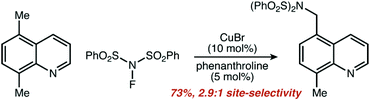 |
| Fig. 6 Single example of a reaction from our data set that fit the criteria for remote functionalization. Adapted with permission from ref. 195. Copyright 2016 Royal Society of Chemistry. | |
Non-resonant 6-membered substrates were nearly rare, accounting for only ∼0.5% of reported reactions. A further investigation of the eight papers which report these substrates illustrates just how unusual it is for a catalytic reaction to be specific for these positions.16,47,49,88,103,130,134,135,140 One strategy to activate these positions is demonstrated by four publications from the Walsh laboratory (ref. 16, 103, 134 and 135), and describe the production of di- and tri-arylmethane products through palladium or nickel catalysis. In three of those four reports, reactive positions adjacent to all-carbon aromatics are also demonstrated as competent coupling partners (Fig. 7). Given that basic heterocycles such as pyridine often inhibit transition metal catalysis, it is a very noteworthy positive feature of these systems that heterocycles are well-tolerated. From a mechanistic perspective, we would describe such reactions as being heterocycle compatible, but not necessarily heterocycle selective. Four other manuscripts report only heterocycle substrates, with a mixture of resonant and non-resonant positions activated. These reports may, indeed, be heterocycle selective—but are not necessarily selective for non-resonant positions. In fact, we found only one manuscript where the paper focused exclusively on non-resonant positions. In that specific case, Höke, Herdtweck, and Bach reported the use of a highly specific hydrogen-bonding interaction between a rhodium paddlewheel catalyst flanked with chiral lactams and a 3-alkylquinolone substrate as a mechanism to access these positions selectively.47
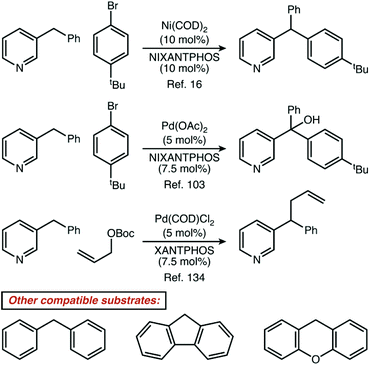 |
| Fig. 7 Heterocycle tolerant cross-coupling reactions developed by Walsh. | |
At first glance, ‘All Others’ seems to be underrepresented, and here we will highlight a very significant effect of our literature filter described in the introduction. The actual search terms entered into SciFinder™ do not include sp3 functionalization inside the heterocycle ring itself. Yet, substrates such as N-Boc-pyrrolidine and xanthene make up a significant fraction of the reactions classified as ‘All Others’. These reactions were discovered from their inclusion in manuscripts that also feature substrates which fit our search terms. So, this number is unquestionably under reported, and would have been much greater if we had exhaustively searched for sp3 C–H functionalizations inside heterocycle rings. We did not pursue that strategy, as we felt as though it was essentially a different review topic. The types of strategies pursued for functionalization adjacent to an aromatic heterocycle and within a saturated heterocycle ring are likely very different. We did not exclude these reactions from our analysis though—we thought it might provide some qualitative context, as long as the readers were properly warned to take those numbers to be very rough indeed.
Even with some prior expectations, we were surprised by the high number of 8-alkylquinolines reported. We had expected to see this well-established tool for organometallic C–H activation chemistry well-represented. To support that assumption, we next detailed the distribution of reaction mechanisms used for each substrate class that had a significant number of examples (Fig. 8).
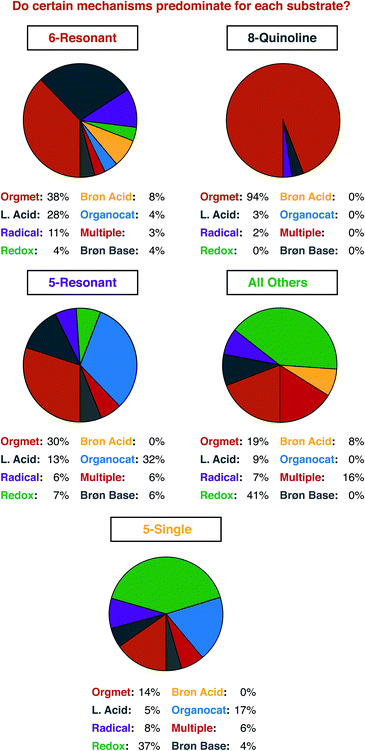 |
| Fig. 8 Relationship between the heterocycle structure and mechanism class. | |
Three observations seem most important to us from this analysis. First, it is indeed true that 8-alkylquinoline is used almost exclusively to investigate organometallic C–H activation pathways. Second, there was a potentially significant difference in the number of organocatalytic pathways reported for 5- and 6-membered resonant substrates. Third, and perhaps naturally, single-heteroatom 5-membered substrates (e.g. indole, thiophene, furan, etc.) are more often subject to redox catalysis than resonant/quinoline substrates. For the reasons mentioned just above, we did not further analyse the ‘All Other’ group, but it is included here for the curious reader. The high number of reports using 8-substituted quinolines is driven primarily by rhodium and palladium catalysis, which make up 27 of the 32 papers that feature organometallic mechanisms, with cobalt, ruthenium and iridium making up the remainder (Fig. 9).
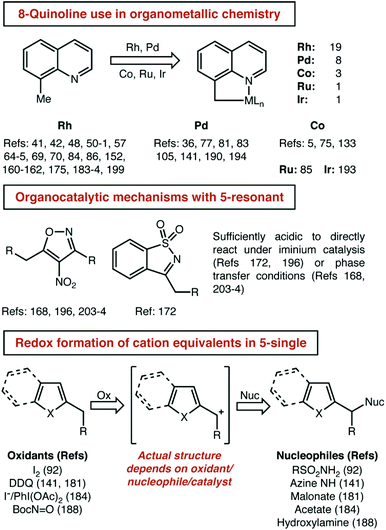 |
| Fig. 9 Structural features that lead to the observed difference in the distribution of the reaction mechanism. | |
With regard to the issue of the relatively high number of organocatalytic pathways reported for 5-membered resonant heterocycles, that result seems to be a somewhat coincidental matter of two particular substrate structures. Four of the five papers that report these mechanisms use the same—highly acidic—heterocycle, a 4-nitroisoxazole. The final report also features a highly acidified substrate, a cyclic sulfonylimine. The highly acidic nature of these substrates allows for sufficient tautomerization and/or deprotonation to occur in the absence of a strong base so that iminium and phase transfer catalysis can be used. With regards to the use of single-heteroatom 5-membered substrates, again, our expectations were met. The ability of these species to generate benzylic cation equivalents—with various precise structures—that can couple with nucleophiles is not shared by the relatively electron poor substrates found in other classes.
Conclusions
Missing chemistries: do they really matter?
Without question, in our eyes the most important result of this analysis is the near-complete dearth of catalytic chemistry that is being targeted at non-resonant and remote aromatic positions. Whatever systematic effects our publication filter strategy may have, it is unquestionably true that reactions on these substrates are orders-of-magnitude less common than the other structure types. Such a statement leads to a natural question, however: does it really matter? We think that compelling arguments can be made on either side, and we will give our balanced thoughts here. The reader can decide for themselves whether more effort is needed in these areas.
Is this a chicken-or-the-egg issue? Are remotely alkylated quinolines particularly difficult to make, and so are not available substrates? Are thiazoles substituted at non-resonant positions not useful for pharmaceutical or materials applications, and so synthetic routes to form them are not valuable? Also, one could point to the fact that we excluded very well established radical initiators like AIBN from our search. Perhaps traditional reaction platforms serve these substrates perfectly well.
While we lack the omnipotence to answer these questions authoritatively, we can again look at the data (Fig. 10). We chose 5-, 6-, and 7-substituted quinolines and 4-, and 5-substituted thiazoles as representative heterocycles. We used the single C–H functionalization of a methylene carbon atom as a representative transformation. In this case, we had no restriction on the publication year or the nature of the catalyst used, which should thus include traditional chemistries like AIBN/NBS bromination.
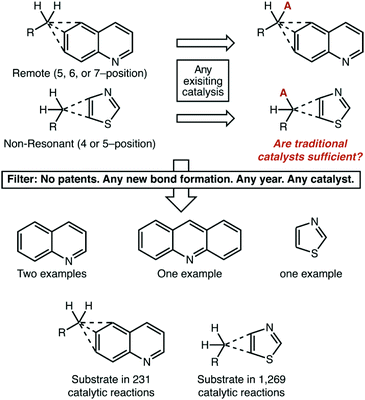 |
| Fig. 10 Analysis of remote and non-resonant C–H activation with no restrictive filters for publication year or catalyst structure. | |
It is unquestionably true that these alkylheterocycles are relatively rare. They have been reported as product structures in just over 13
000 reactions published in academic journals. Following from this, they have been reported as the substrate of a catalytic reaction only 1500 times. Even still, of these 1500 reactions only four involved C–H functionalization—excluding two reactions where the desired products were the minor component of a regioisomeric mixture. All four reactions were radical brominations. The absence of evidence is not evidence of absence, however. We do not see any fundamental reason why many existing reaction platforms could not be adapted for these systems.
Even still, we argue in favour of increased investigation in these areas for two reasons. Fundamentally, it seems hard to argue against investigation of a reasonable substrate class where no single modern catalytic approach has been applied. It may be the case that absolutely nothing novel or interesting is encountered, and a catalyst that is able to react with 2-ethylpyridine also suffices for 6-ethylquinoline and 4-ethylthiazole. It is hard to put too much weight on this argument, however, given that it would also be an argument for ending research in synthetic methodology nearly entirely.
Second, we believe that as target-oriented research attempts to use C–H functionalization in more and more complex settings, issues of site-selectivity are going to become increasingly relevant.215–226 Consider complex small molecules investigated in pharmaceutical applications, such as verucerfont or nizatidine (Fig. 11). If C–H functionalization for the synthesis of potential metabolites or for attachment to a polymer nanoparticle was desired, how could the site of reaction be controlled? Developing such technology for the synthetic community will require accessing non-resonant and remote positions, as well as positions adjacent to heterocycles that are currently not well explored. This is an area where traditional chemistry will also come up short. While some particular feature of the substrate structure might guide NBS bromination to a single position, that will only provide access to the inherently most reactive site. It does not seem at all apparent how inherently less reactive positions could be selectively accessed without the use of catalysis. To our eye, this seems to be a problem well suited for the application of supramolecular chemistry. Could heterocycle coordination and C–H activation occur at two different locations in a catalyst scaffold—held in precise relative positions—to break bonds that are inaccessible to current catalysts? Or, will the development of a successful system avoid heterocycle coordination entirely? Perhaps other non-covalent interactions could guide the reaction towards unusual sites. We hope that research groups active in this area of C–H functionalization will consider investigating more substrates that are dramatically underreported in their ongoing work.
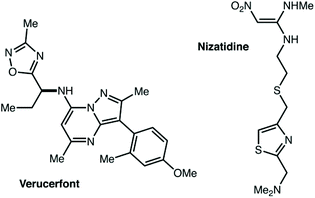 |
| Fig. 11 Complex alkylheterocycles that will require significant catalyst development to enable site-selective C–H functionalization. | |
Conflicts of interest
There are no conflicts to declare.
Acknowledgements
Funding for this work was provided by the NSERC of Canada through the Discovery Grant program, and the University of Calgary through start-up funds.
Notes and references
- K. Ablajan, G. B. Panetti, X. Yang, B.-S. Kim and P. J. Walsh, Adv. Synth. Catal., 2017, 359, 1927–1932 CrossRef CAS PubMed.
- H. Akutsu, K. Nakashima, S.-I. Hirashima, M. Kitahara, Y. Koseki and T. Miura, Tetrahedron Lett., 2017, 58, 4759–4762 CrossRef CAS.
- T. A. Alanine, S. Stokes, C. A. Roberts and J. S. Scott, Org. Biomol. Chem., 2018, 16, 53–56 RSC.
- S. K. Arupula, S. Guin, A. Yadav, S. M. Mobin and S. Samanta, J. Org. Chem., 2018, 83, 2660–2675 CrossRef CAS PubMed.
- N. Barsu, M. A. Rahman, M. Sen and B. Sundararaju, Chem. – Eur. J., 2016, 22, 9135–9138 CrossRef CAS PubMed.
- I. Bassoude, S. Berteina-Raboin, S. Massip, J.-M. Leger, C. Jarry, E. M. Essassi and G. Guillaumet, Eur. J. Org. Chem., 2012, 2012, 2572–2578 CrossRef CAS.
- T. Beisel, J. Kirchner, T. Kaehler, J. Knauer, Y. Soltani and G. Manolikakes, Org. Biomol. Chem., 2016, 14, 5525–5528 RSC.
- K. W. Bentley, K. A. Dummit and J. F. Van Humbeck, Chem. Sci., 2018, 9, 6440–6445 RSC.
- D. Best, S. Kujawa and H. W. Lam, J. Am. Chem. Soc., 2012, 134, 18193–18196 CrossRef CAS PubMed.
- B. Blank and R. Kempe, J. Am. Chem. Soc., 2010, 132, 924–925 CrossRef CAS PubMed.
- M. Blocker, S. Immaneni and A. Shaikh, Tetrahedron Lett., 2014, 55, 5572–5575 CrossRef CAS.
- J. Bojanowski and A. Albrecht, ChemistrySelect, 2019, 4, 4588–4592 CrossRef CAS.
- T. Brandhofer, A. Özdemir, A. Gini and O. G. Mancheño, Chem. – Eur. J., 2019, 25, 4077–4086 CrossRef CAS PubMed.
- C. Bruneau and R. Gramage-Doria, Adv. Synth. Catal., 2016, 358, 3847–3856 CrossRef CAS.
- P. M. Burton and J. A. Morris, Org. Lett., 2010, 12, 5359–5361 CrossRef CAS PubMed.
- X. Cao, S.-C. Sha, M. Li, B.-S. Kim, C. Morgan, R. Huang, X. Yang and P. J. Walsh, Chem. Sci., 2016, 7, 611–618 RSC.
- V. Ceban, P. Putaj, M. Meazza, M. B. Pitak, S. J. Coles, J. Vesely and R. Rios, Chem. Commun., 2014, 50, 7447–7450 RSC.
- S. Chatterjee, P. Bhattacharjee, J. Temburu, D. Nandi and P. Jaisankar, Tetrahedron Lett., 2014, 55, 6680–6683 CrossRef CAS.
- C. Chaudhari, S. M. A. H. Siddiki and K.-I. Shimizu, Tetrahedron Lett., 2013, 54, 6490–6493 CrossRef CAS.
- S. S. Chavan, M. Y. Pathan, S. H. Thorat, R. Gonnade and S. A. R. Mulla, RSC Adv., 2015, 5, 81103–81107 RSC.
- S. S. Chavan, M. Y. Pathan and S. A. R. Mulla, RSC Adv., 2015, 5, 103091–103094 RSC.
- X. Chen, M. Liu, Y. Liu, Y. Chen, C.-T. Au and S.-F. Yin, Asian J. Org. Chem., 2018, 7, 1825–1829 CrossRef CAS.
- Y. Chen, S. Wang, Q. Jiang, C. Cheng, X. Xiao and G. Zhu, J. Org. Chem., 2018, 83, 716–722 CrossRef CAS PubMed.
- L. Cheng, Y. Sun, W. Wang, C. Yao and T.-J. Li, J. Org. Chem., 2019, 84, 3074–3082 CrossRef CAS PubMed.
- B. S. Cho and Y. K. Chung, Chem. Commun., 2015, 51, 14543–14546 RSC.
- J. C. Cooper, C. Luo, R. Kameyama and J. F. Van Humbeck, J. Am. Chem. Soc., 2018, 140, 1243–1246 CrossRef CAS PubMed.
- J. De Houwer, K. A. Tehrani and B. U. W. Maes, Angew. Chem., Int. Ed., 2012, 51, 2745–2748 CrossRef CAS PubMed.
- D.-Q. Dong, X. Gao, L.-X. Li, S.-H. Hao and Z.-L. Wang, Res. Chem. Intermed., 2018, 44, 7557–7567 CrossRef CAS.
- S. Duan, M. Li, X. Ma, W. Chen, L. Li, H. Zhang, X. Yang and P. J. Walsh, Adv. Synth. Catal., 2018, 360, 4837–4842 CrossRef CAS.
- T.-Y. Feng, H.-X. Li, D. J. Young and J.-P. Lang, J. Org. Chem., 2017, 82, 4113–4120 CrossRef CAS PubMed.
- Y. Fukumoto, M. Hirano, N. Matsubara and N. Chatani, J. Org. Chem., 2017, 82, 13649–13655 CrossRef CAS PubMed.
- Y. Fukumoto, M. Hirano and N. Chatani, ACS Catal., 2017, 7, 3152–3156 CrossRef CAS.
- F. Gao, B.-S. Kim and P. J. Walsh, Chem. Sci., 2016, 7, 976–983 RSC.
- G. Gao, Y. Fu, M. Li, B. Wang, B. Zheng, S. Hou and P. J. Walsh, Adv. Synth. Catal., 2017, 359, 2890–2894 CrossRef CAS PubMed.
- X. Gao, F. Zhang, G. Deng and L. Yang, Org. Lett., 2014, 16, 3664–3667 CrossRef CAS PubMed.
- D. N. Garad and S. B. Mhaske, J. Org. Chem., 2017, 82, 10470–10478 CrossRef CAS PubMed.
- V. B. Graves and A. Shaikh, Tetrahedron Lett., 2013, 54, 695–698 CrossRef CAS.
- B.-T. Guan, B. Wang, M. Nishiura and Z. Hou, Angew. Chem., Int. Ed., 2013, 52, 4418–4421 CrossRef CAS PubMed.
- S. Guha and G. Sekar, Chem. – Eur. J., 2018, 24, 14171–14182 CrossRef CAS PubMed.
- C. Guo, J. Song, S.-W. Luo and L.-Z. Gong, Angew. Chem., Int. Ed., 2010, 49, 5558–5562 CrossRef CAS PubMed.
- S. Han, J. Park, S. Kim, S. H. Lee, S. Sharma, N. K. Mishra, Y. H. Jung and I. S. Kim, Org. Lett., 2016, 18, 4666–4669 CrossRef CAS PubMed.
- S. Han, W. Ma, Z. Zhang, L. Liu, M. Tang and J. Li, Asian J. Org. Chem., 2017, 6, 1014–1018 CrossRef CAS.
- C. He and M. J. Gaunt, Angew. Chem., Int. Ed., 2015, 54, 15840–15844 CrossRef CAS PubMed.
- J. He, L. G. Hamann, H. M. L. Davies and R. E. J. Beckwith, Nat. Commun., 2015, 6, 5943 CrossRef PubMed.
- X.-L. He, H.-R. Zhao, C.-Q. Duan, X. Han, W. Du and Y.-C. Chen, Chem. – Eur. J., 2018, 24, 6277–6281 CrossRef CAS PubMed.
- M. Hirano, Y. Fukumoto, N. Matsubara and N. Chatani, Chem. Lett., 2018, 47, 385–388 CrossRef CAS.
- T. Höke, E. Herdtweck and T. Bach, Chem. Commun., 2013, 49, 8009–8011 RSC.
- W. Hou, Y. Yang, Y. Wu, H. Feng, Y. Li and B. Zhou, Chem. Commun., 2016, 52, 9672–9675 RSC.
- D. P. Hruszkewycz, K. C. Miles, O. R. Thiel and S. S. Stahl, Chem. Sci., 2017, 8, 1282–1287 RSC.
- X.-H. Hu, X.-F. Yang and T.-P. Loh, ACS Catal., 2016, 6, 5930–5934 CrossRef CAS.
- X. Huang and J. You, Chem. Lett., 2015, 44, 1685–1687 CrossRef CAS.
- X. Huang, Y.-H. Wen, F.-T. Zhou, C. Chen, D.-C. Xu and J.-W. Xie, Tetrahedron Lett., 2010, 51, 6637–6640 CrossRef CAS.
- M. Itoh, K. Hirano, T. Satoh and M. Miura, Org. Lett., 2014, 16, 2050–2053 CrossRef CAS PubMed.
- Z. Jamal, Y.-C. Teo and L.-K. Wong, Eur. J. Org. Chem., 2014, 2014, 7343–7346 CrossRef CAS.
- Z. Jamal and Y.-C. Teo, RSC Adv., 2015, 5, 26949–26953 RSC.
- Z. Jamal, Y.-C. Teo and G. S. Lim, Tetrahedron, 2016, 72, 2132–2138 CrossRef CAS.
- T. Jeong, N. K. Mishra, P. Dey, H. Oh, S. Han, S. H. Lee, H. S. Kim, J. Park and I. S. Kim, Chem. Commun., 2017, 53, 11197–11200 RSC.
- H. Jiang, H. Chen, A. Wang and X. Liu, Chem. Commun., 2010, 46, 7259–7261 RSC.
- K. Jiang, D. Pi, H. Zhou, S. Liu and K. Zou, Tetrahedron, 2014, 70, 3056–3060 CrossRef CAS.
- J.-J. Jin, H.-Y. Niu, G.-R. Qu, H.-M. Guo and J. S. Fossey, RSC Adv., 2012, 2, 5968–5971 RSC.
- J.-J. Jin, D.-C. Wang, H.-Y. Niu, S. Wu, G.-R. Qu, Z.-B. Zhang and H.-M. Guo, Tetrahedron, 2013, 69, 6579–6584 CrossRef CAS.
- J. Jin and D. W. C. MacMillan, Angew. Chem., Int. Ed., 2015, 54, 1565–1569 CrossRef CAS PubMed.
- S. Kayal and S. Mukherjee, Org. Lett., 2017, 19, 4944–4947 CrossRef CAS PubMed.
- J. H. Kim, S. Greßies, M. Boultadakis-Arapinis, C. Daniliuc and F. Glorius, ACS Catal., 2016, 6, 7652–7656 CrossRef CAS.
- S. Kim, S. Han, J. Park, S. Sharma, N. K. Mishra, H. Oh, J. H. Kwak and I. S. Kim, Chem. Commun., 2017, 53, 3006–3009 RSC.
- M. Kljajic, J. G. Puschnig, H. Weber and R. Breinbauer, Org. Lett., 2017, 19, 126–129 CrossRef CAS PubMed.
- H. Komai, T. Yoshino, S. Matsunaga and M. Kanai, Org. Lett., 2011, 13, 1706–1709 CrossRef CAS PubMed.
- H. Komai, T. Yoshino, S. Matsunaga and M. Kanai, Synthesis, 2012, 2185–2194 CAS.
- L. Kong, X. Zhou, Y. Xu and X. Li, Org. Lett., 2017, 19, 3644–3647 CrossRef CAS PubMed.
- L. Kong, B. Liu, X. Zhou, F. Wang and X. Li, Chem. Commun., 2017, 53, 10326–10329 RSC.
- D. Kowalczyk and Ł. Albrecht, Adv. Synth. Catal., 2018, 360, 406–410 CrossRef CAS.
- A. Kumar and R. D. Shukla, Green Chem., 2015, 17, 848–851 RSC.
- G. S. Kumar, J. W. Boyle, C. Tejo and P. W. H. Chan, Chemi. – Asian J., 2016, 11, 385–389 CrossRef CAS PubMed.
- J. Kumar, A. Gupta and S. Bhadra, Org. Biomol. Chem., 2019, 17, 3314–3318 RSC.
- R. Kumar, R. Kumar, D. Chandra and U. Sharma, J. Org. Chem., 2019, 84, 1542–1552 CrossRef CAS PubMed.
- K. Kumari, B. K. Allam and K. N. Singh, RSC Adv., 2014, 4, 19789–19793 RSC.
- V. G. Landge, M. K. Sahoo, S. P. Midya, G. Jaiswal and E. Balaraman, Dalton Trans., 2015, 44, 15382–15386 RSC.
- A. I. Lansakara, D. P. Farrell and F. C. Pigge, Org. Biomol. Chem., 2014, 12, 1090–1099 RSC.
- C. Le, Y. Liang, R. W. Evans, X. Li and D. W. C. MacMillan, Nature, 2017, 547, 79 CrossRef CAS PubMed.
- M. Lee and M. S. Sanford, Org. Lett., 2017, 19, 572–575 CrossRef CAS PubMed.
- S. J. Lee, A. F. Brooks, N. Ichiishi, K. J. Makaravage, A. V. Mossine, M. S. Sanford and P. J. H. Scott, Chem. Commun., 2019, 55, 2976–2979 RSC.
- J. Li, C. Wu, B. Zhou and P. J. Walsh, J. Org. Chem., 2018, 83, 2993–2999 CrossRef CAS.
- L. Li, F. Zhang, G.-J. Deng and H. Gong, Org. Lett., 2018, 20, 7321–7325 CrossRef CAS PubMed.
- B. Liu, T. Zhou, B. Li, S. Xu, H. Song and B. Wang, Angew. Chem., Int. Ed., 2014, 53, 4191–4195 CrossRef CAS PubMed.
- B. Liu, B. Li and B. Wang, Chem. Commun., 2015, 51, 16334–16337 RSC.
- B. Liu, P. Hu, X. Zhou, D. Bai, J. Chang and X. Li, Org. Lett., 2017, 19, 2086–2089 CrossRef CAS PubMed.
- D. Liu, C. Liu, H. Li and A. Lei, Angew. Chem., Int. Ed., 2013, 52, 4453–4456 CrossRef CAS PubMed.
- J. Liu, X. Zhang, H. Yi, C. Liu, R. Liu, H. Zhang, K. Zhuo and A. Lei, Angew. Chem., Int. Ed., 2015, 54, 1261–1265 CrossRef CAS PubMed.
- J. Liu, H.-X. Zheng, C.-Z. Yao, B.-F. Sun and Y.-B. Kang, J. Am. Chem. Soc., 2016, 138, 3294–3297 CrossRef CAS PubMed.
- J.-Y. Liu, H.-Y. Niu, S. Wu, G.-R. Qu and H.-M. Guo, Chem. Commun., 2012, 48, 9723–9725 RSC.
- M. Liu, T. Chen and S.-F. Yin, Catal. Sci. Technol., 2016, 6, 690–693 RSC.
- X.-J. Liu and S.-L. You, Angew. Chem., Int. Ed., 2017, 56, 4002–4005 CrossRef CAS PubMed.
- X. Liu, Y. Zhou, Z. Yang, Q. Li, L. Zhao and P. Liu, J. Org. Chem., 2018, 83, 4665–4673 CrossRef CAS PubMed.
- X.-L. Liu, D.-H. Jing, Z. Yao, W.-H. Zhang, X.-W. Liu, Z.-J. Yang, Z. Zhao, Y. Zhou and X.-N. Li, Tetrahedron Lett., 2015, 56, 5637–5645 CrossRef CAS.
- Z. Liu, M. Li, B. Wang, G. Deng, W. Chen, B.-S. Kim, H. Zhang, X. Yang and P. J. Walsh, Org. Chem. Front., 2018, 5, 1870–1876 RSC.
- C. C. J. Loh, M. Schmid, B. Peters, X. Fang and M. Lautens, Angew. Chem., Int. Ed., 2016, 55, 4600–4604 CrossRef CAS.
- M. J. Lopez, A. Kondo, H. Nagae, K. Yamamoto, H. Tsurugi and K. Mashima, Organometallics, 2016, 35, 3816–3827 CrossRef CAS.
- M. Loubidi, A. Moutardier, J. F. Campos and S. Berteina-Raboin, Tetrahedron Lett., 2018, 59, 1050–1054 CrossRef CAS.
- B. Lu, Q. Lu, S. Zhuang, J. Cheng and B. Huang, RSC Adv., 2015, 5, 8285–8288 RSC.
- Y. Luo, H.-L. Teng, M. Nishiura and Z. Hou, Angew. Chem., Int. Ed., 2017, 56, 9207–9210 CrossRef CAS PubMed.
- I. S. Makarov, T. Kuwahara, X. Jusseau, I. Ryu, A. T. Lindhardt and T. Skrydstrup, J. Am. Chem. Soc., 2015, 137, 14043–14046 CrossRef CAS PubMed.
- D. Mao, G. Hong, S. Wu, X. Liu, J. Yu and L. Wang, Eur. J. Org. Chem., 2014, 2014, 3009–3019 CrossRef CAS.
- J. Mao, K. Eberle, J. Zhang, C. Rodríguez-Escrich, Z. Xi, M. A. Pericàs and P. J. Walsh, Tetrahedron Lett., 2015, 56, 3604–3607 CrossRef CAS PubMed.
- J. B. McManus, N. P. R. Onuska and D. A. Nicewicz, J. Am. Chem. Soc., 2018, 140, 9056–9060 CrossRef CAS PubMed.
- K. B. McMurtrey, J. M. Racowski and M. S. Sanford, Org. Lett., 2012, 14, 4094–4097 CrossRef CAS PubMed.
- A. McNally, C. K. Prier and D. W. C. MacMillan, Science, 2011, 334, 1114 CrossRef CAS PubMed.
- M. Meazza, V. Ceban, M. B. Pitak, S. J. Coles and R. Rios, Chem. – Eur. J., 2014, 20, 16853–16857 CrossRef CAS PubMed.
- M. Meazza, F. Tur, N. Hammer and K. A. Jørgensen, Angew. Chem., Int. Ed., 2017, 56, 1634–1638 CrossRef CAS PubMed.
- T. Mita, Y. Ikeda, K. Michigami and Y. Sato, Chem. Commun., 2013, 49, 5601–5603 RSC.
- T. Mukai, K. Hirano, T. Satoh and M. Miura, Org. Lett., 2010, 12, 1360–1363 CrossRef CAS PubMed.
- R. Murakami, K. Sano, T. Iwai, T. Taniguchi, K. Monde and M. Sawamura, Angew. Chem., Int. Ed., 2018, 57, 9465–9469 CrossRef CAS PubMed.
- A. Muthukumar and G. Sekar, Org. Biomol. Chem., 2017, 15, 691–700 RSC.
- S. Nagaraju, N. Satyanarayana, B. Paplal, A. K. Vasu, S. Kanvah, B. Sridhar, P. Sripadi and D. Kashinath, RSC Adv., 2015, 5, 94474–94478 RSC.
- R. Niu, J. Xiao, T. Liang and X. Li, Org. Lett., 2012, 14, 676–679 CrossRef CAS PubMed.
- A. Noble and D. W. C. MacMillan, J. Am. Chem. Soc., 2014, 136, 11602–11605 CrossRef CAS PubMed.
- Y. Obora, S. Ogawa and N. Yamamoto, J. Org. Chem., 2012, 77, 9429–9433 CrossRef CAS PubMed.
- X. Pang, L. Xiang, J. Ma, X. Yang and R. Yan, RSC Adv., 2016, 6, 111713–111717 RSC.
- L. Pawar and F. C. Pigge, Tetrahedron Lett., 2013, 54, 6067–6070 CrossRef CAS.
- D. Pi, K. Jiang, H. Zhou, Y. Sui, Y. Uozumi and K. Zou, RSC Adv., 2014, 4, 57875–57884 RSC.
- C. K. Prier and D. W. C. MacMillan, Chem. Sci., 2014, 5, 4173–4178 RSC.
- B. Qian, S. Guo, C. Xia and H. Huang, Adv. Synth. Catal., 2010, 352, 3195–3200 CrossRef CAS.
- B. Qian, S. Guo, J. Shao, Q. Zhu, L. Yang, C. Xia and H. Huang, J. Am. Chem. Soc., 2010, 132, 3650–3651 CrossRef CAS PubMed.
- B. Qian, P. Xie, Y. Xie and H. Huang, Org. Lett., 2011, 13, 2580–2583 CrossRef CAS PubMed.
- B. Qian, D. Shi, L. Yang and H. Huang, Adv. Synth. Catal., 2012, 354, 2146–2150 CrossRef CAS.
- B. Qian, L. Yang and H. Huang, Tetrahedron Lett., 2013, 54, 711–714 CrossRef CAS.
- J. Rana, R. Babu, M. Subaramanian and E. Balaraman, Org. Chem. Front., 2018, 5, 3250–3255 RSC.
- D. Ren, L. Xu, L. Wang and S.-S. Li, Org. Lett., 2019, 21, 627–631 CrossRef CAS PubMed.
- L. Ren, L. Wang, Y. Lv, G. Li and S. Gao, Org. Lett., 2015, 17, 2078–2081 CrossRef CAS PubMed.
- R. L. Reyes, T. Iwai, S. Maeda and M. Sawamura, J. Am. Chem. Soc., 2019, 141, 6817–6821 CrossRef CAS PubMed.
- A. R. Rivero, B.-S. Kim and P. J. Walsh, Org. Lett., 2016, 18, 1590–1593 CrossRef CAS PubMed.
- M. Rueping and N. Tolstoluzhsky, Org. Lett., 2011, 13, 1095–1097 CrossRef CAS PubMed.
- R. Sarkar, S. Mitra and S. Mukherjee, Chem. Sci., 2018, 9, 5767–5772 RSC.
- M. Sen, B. Emayavaramban, N. Barsu, J. R. Premkumar and B. Sundararaju, ACS Catal., 2016, 6, 2792–2796 CrossRef CAS.
- S.-C. Sha, J. Zhang, P. J. Carroll and P. J. Walsh, J. Am. Chem. Soc., 2013, 135, 17602–17609 CrossRef CAS PubMed.
- S.-C. Sha, H. Jiang, J. Mao, A. Bellomo, S. A. Jeong and P. J. Walsh, Angew. Chem., Int. Ed., 2016, 55, 1070–1074 CrossRef CAS PubMed.
- M. Shang, J. Z. Chan, M. Cao, Y. Chang, Q. Wang, B. Cook, S. Torker and M. Wasa, J. Am. Chem. Soc., 2018, 140, 10593–10601 CrossRef CAS PubMed.
- V. Sharma, J. Kaur and S. S. Chimni, Eur. J. Org. Chem., 2018, 2018, 3489–3495 CrossRef CAS.
- M. H. Shaw, V. W. Shurtleff, J. A. Terrett, J. D. Cuthbertson and D. W. C. MacMillan, Science, 2016, 352, 1304 CrossRef CAS PubMed.
- Y.-B. Shen, S.-S. Li, Y.-M. Sun, L. Yu, Z.-H. Hao, Q. Liu and J. Xiao, J. Org. Chem., 2019, 84, 2779–2785 CrossRef CAS PubMed.
- M. Shigeno, K. Nakaji, K. Nozawa-Kumada and Y. Kondo, Org. Lett., 2019, 21, 2588–2592 CrossRef CAS PubMed.
- A. Shrestha, M. Lee, A. L. Dunn and M. S. Sanford, Org. Lett., 2018, 20, 204–207 CrossRef CAS PubMed.
- C. Song, X. Dong, H. Yi, C.-W. Chiang and A. Lei, ACS Catal., 2018, 8, 2195–2199 CrossRef CAS.
- G. Song, Y. Su, X. Gong, K. Han and X. Li, Org. Lett., 2011, 13, 1968–1971 CrossRef CAS PubMed.
- H. Sterckx, J. De Houwer, C. Mensch, W. Herrebout, K. A. Tehrani and B. U. W. Maes, Beilstein J. Org. Chem., 2016, 12, 144–153 CrossRef CAS PubMed.
- H. Sterckx, C. Sambiagio, V. Médran-Navarrete and B. U. W. Maes, Adv. Synth. Catal., 2017, 359, 3226–3236 CrossRef CAS.
- Y.-L. Su, Z.-Y. Han, Y.-H. Li and L.-Z. Gong, ACS Catal., 2017, 7, 7917–7922 CrossRef CAS.
- Q. Sun, P. Xie, D. Yuan, Y. Xia and Y. Yao, Chem. Commun., 2017, 53, 7401–7404 RSC.
- H. Suzuki, R. Igarashi, Y. Yamashita and S. Kobayashi, Angew. Chem., Int. Ed., 2017, 56, 4520–4524 CrossRef CAS PubMed.
- N. Takemura, Y. Kuninobu and M. Kanai, Org. Biomol. Chem., 2014, 12, 2528–2532 RSC.
- D. Talwar, A. Gonzalez-de-Castro, H. Y. Li and J. Xiao, Angew. Chem., Int. Ed., 2015, 54, 5223–5227 CrossRef CAS PubMed.
- Z. Tan, H. Jiang and M. Zhang, Chem. Commun., 2016, 52, 9359–9362 RSC.
- C. Tang, M. Zou, J. Liu, X. Wen, X. Sun, Y. Zhang and N. Jiao, Chem. – Eur. J., 2016, 22, 11165–11169 CrossRef CAS PubMed.
- Z. Tashrifi, K. Rad-Moghadam, M. Mehrdad, M. Soheilizad, B. Larijani and M. Mahdavi, Tetrahedron Lett., 2018, 59, 1555–1559 CrossRef CAS.
- B. M. Trost, D. A. Thaisrivongs and J. Hartwig, J. Am. Chem. Soc., 2011, 133, 12439–12441 CrossRef CAS PubMed.
- H. Tsurugi, K. Yamamoto and K. Mashima, J. Am. Chem. Soc., 2011, 133, 732–735 CrossRef CAS PubMed.
- M. Vellakkaran, J. Das, S. Bera and D. Banerjee, Chem. Commun., 2018, 54, 12369–12372 RSC.
- S. Vera, Y. Liu, M. Marigo, E. C. Escudero-Adán and P. Melchiorre, Synlett, 2011, 489–494 CAS.
- S. V. N. Vuppalapati and Y. R. Lee, Tetrahedron, 2012, 68, 8286–8292 CrossRef CAS.
- F.-F. Wang, C.-P. Luo, G. Deng and L. Yang, Green Chem., 2014, 16, 2428–2431 RSC.
- H. Wang, G. Tang and X. Li, Angew. Chem., Int. Ed., 2015, 54, 13049–13052 CrossRef CAS PubMed.
- N. Wang, R. Li, L. Li, S. Xu, H. Song and B. Wang, J. Org. Chem., 2014, 79, 5379–5385 CrossRef CAS PubMed.
- X. Wang, D.-G. Yu and F. Glorius, Angew. Chem., Int. Ed., 2015, 54, 10280–10283 CrossRef CAS PubMed.
- Y. Wang, X. Li, F. Leng, H. Zhu, J. Li, D. Zou, Y. Wu and Y. Wu, Adv. Synth. Catal., 2014, 356, 3307–3313 CrossRef CAS.
- Y.-F. Wang, F.-L. Zhang and S. Chiba, Synthesis, 2012, 44, 1526–1534 CrossRef CAS.
- Z.-L. Wang, RSC Adv., 2015, 5, 5563–5566 RSC.
- J. Wu, D. Wang, X. Chen, Q. Gui, H. Li, Z. Tan, G. Huang and G. Wang, Org. Biomol. Chem., 2017, 15, 7509–7512 RSC.
- X. Wu, H.-J. Zhu, S.-B. Zhao, S.-S. Chen, Y.-F. Luo and Y.-G. Li, Org. Lett., 2018, 20, 32–35 CrossRef CAS PubMed.
- X. Xia, Q. Zhu, J. Wang, J. Chen, W. Cao, B. Zhu and X. Wu, J. Org. Chem., 2018, 83, 14617–14625 CrossRef CAS PubMed.
- F. Xiao, C. Liu, S. Yuan, H. Huang and G.-J. Deng, J. Org. Chem., 2018, 83, 10420–10429 CrossRef CAS PubMed.
- M. Xiao, D. Ren, L. Xu, S.-S. Li, L. Yu and J. Xiao, Org. Lett., 2017, 19, 5724–5727 CrossRef CAS PubMed.
- J. Xie, M. Rudolph, F. Rominger and A. S. K. Hashmi, Angew. Chem., Int. Ed., 2017, 56, 7266–7270 CrossRef CAS PubMed.
- X.-F. Xiong, H. Zhang, J. Peng and Y.-C. Chen, Chem. – Eur. J., 2011, 17, 2358–2360 CrossRef CAS PubMed.
- H. Xu, L. Laraia, L. Schneider, K. Louven, C. Strohmann, A. P. Antonchick and H. Waldmann, Angew. Chem., Int. Ed., 2017, 56, 11232–11236 CrossRef CAS PubMed.
- L. Xu, H. Chen, J. Liu, L. Zhou, Q. Liu, Y. Lan and J. Xiao, Org. Chem. Front., 2019, 6, 1162–1167 RSC.
- S.-Y. Yan, P.-X. Ling and B.-F. Shi, Adv. Synth. Catal., 2017, 359, 2912–2917 CrossRef CAS.
- J. Yang, J. Hu, Y. Huang, X. Xu and F. Qing, Chin. J. Chem., 2017, 35, 867–870 CrossRef CAS.
- L. Yang, X. Shi, B.-Q. Hu and L.-X. Wang, Asian J. Org. Chem., 2016, 5, 494–498 CrossRef CAS.
- W. Yang, S. Ye, Y. Schmidt, D. Stamos and J.-Q. Yu, Chem. – Eur. J., 2016, 22, 7059–7062 CrossRef CAS PubMed.
- X. Yang, B.-S. Kim, M. Li and P. J. Walsh, Org. Lett., 2016, 18, 2371–2374 CrossRef CAS PubMed.
- S. Yaragorla, G. Singh and R. Dada, Tetrahedron Lett., 2015, 56, 5924–5929 CrossRef CAS.
- S. Yaragorla, R. Dada and G. Singh, Synlett, 2016, 912–918 CAS.
- J.-B. Yu, Y. Zhang, Z.-J. Jiang and W.-K. Su, J. Org. Chem., 2016, 81, 11514–11520 CrossRef CAS PubMed.
- S. Yu, G. Tang, Y. Li, X. Zhou, Y. Lan and X. Li, Angew. Chem., Int. Ed., 2016, 55, 8696–8700 CrossRef CAS PubMed.
- S. Yu, Y. Li, L. Kong, X. Zhou, G. Tang, Y. Lan and X. Li, ACS Catal., 2016, 6, 7744–7748 CrossRef CAS.
- H. Zaimoku, T. Hatta, T. Taniguchi and H. Ishibashi, Org. Lett., 2012, 14, 6088–6091 CrossRef CAS PubMed.
- D.-D. Zhai, X.-Y. Zhang, Y.-F. Liu, L. Zheng and B.-T. Guan, Angew. Chem., Int. Ed., 2018, 57, 1650–1653 CrossRef CAS PubMed.
- G. Zhang, T. Irrgang, T. Dietel, F. Kallmeier and R. Kempe, Angew. Chem., Int. Ed., 2018, 57, 9131–9135 CrossRef CAS PubMed.
- J. Zhang, S.-C. Sha, A. Bellomo, N. Trongsiriwat, F. Gao, N. C. Tomson and P. J. Walsh, J. Am. Chem. Soc., 2016, 138, 4260–4266 CrossRef CAS PubMed.
- J. Zhang, S. T. Kohlbouni and B. Borhan, Org. Lett., 2019, 21, 14–17 CrossRef CAS PubMed.
- S. Zhang, F. Luo, W. Wang, X. Jia, M. Hu and J. Cheng, Tetrahedron Lett., 2010, 51, 3317–3319 CrossRef CAS.
- S. Zhang, B.-S. Kim, C. Wu, J. Mao and P. J. Walsh, Nat. Commun., 2017, 8, 14641 CrossRef PubMed.
- S. Zhang, B. Hu, Z. Zheng and P. J. Walsh, Adv. Synth. Catal., 2018, 360, 1493–1498 CrossRef CAS PubMed.
- T. Zhang, X. Hu, X. Dong, G. Li and H. Lu, Org. Lett., 2018, 20, 6260–6264 CrossRef CAS PubMed.
- W. Zhang, S. Ren, J. Zhang and Y. Liu, J. Org. Chem., 2015, 80, 5973–5978 CrossRef CAS PubMed.
- X. Zhang, R. Wu, W. Liu, D.-W. Qian, J. Yang, P. Jiang and Q.-Z. Zheng, Org. Biomol. Chem., 2016, 14, 4789–4793 RSC.
- Y. Zhang, B. Wei, H. Lin, W. Cui, X. Zeng and X. Fan, Adv. Synth. Catal., 2015, 357, 1299–1304 CrossRef CAS.
- Y. Zhang, B.-W. Wei, W.-X. Wang, L.-L. Deng, L.-J. Nie, H.-Q. Luo and X.-L. Fan, RSC Adv., 2017, 7, 1229–1232 RSC.
- D. Zhao, T. Wang, Q. Shen and J.-X. Li, Chem. Commun., 2014, 50, 4302–4304 RSC.
- H. Zhao, X. Zhou, B. Li, X. Liu, N. Guo, Z. Lu and S. Wang, J. Org. Chem., 2018, 83, 4153–4159 CrossRef CAS.
- J. Zheng, X. Fan, B. Zhou, Z. H. Li and H. Wang, Chem. Commun., 2016, 52, 4655–4658 RSC.
- B.-H. Zhou, C. Wu, X.-X. Chen, H.-X. Huang, L.-L. Li, L.-M. Fan and J. Li, Tetrahedron Lett., 2017, 58, 4157–4161 CrossRef CAS.
- Y. Zhou, Z. Tang and Q. Song, Chem. Commun., 2017, 53, 8972–8975 RSC.
- B. Zhu, R. Lee, Y. Yin, F. Li, M. L. Coote and Z. Jiang, Org. Lett., 2018, 20, 429–432 CrossRef CAS.
- B. Zhu, F. Li, B. Lu, J. Chang and Z. Jiang, J. Org. Chem., 2018, 83, 11350–11358 CrossRef CAS PubMed.
- Z.-Q. Zhu, P. Bai and Z.-Z. Huang, Org. Lett., 2014, 16, 4881–4883 CrossRef CAS PubMed.
- K. J. Stowers, K. C. Fortner and M. S. Sanford, J. Am. Chem. Soc., 2011, 133, 6541–6544 CrossRef CAS PubMed.
- J. He, M. Wasa, K. S. L. Chan, O. Shao and J. Q. Yu, Chem. Rev., 2017, 117, 8754–8786 CrossRef CAS PubMed.
- K. Murakami, S. Yamada, T. Kaneda and K. Itami, Chem. Rev., 2017, 117, 9302–9332 CrossRef CAS PubMed.
- C. G. Newton, S. G. Wang, C. C. Oliveira and N. Cramer, Chem. Rev., 2017, 117, 8908–8976 CrossRef CAS PubMed.
- L. Ping, D. S. Chung, J. Bouffard and S. G. Lee, Chem. Soc. Rev., 2017, 46, 4299–4328 RSC.
- Y. Qin, L. H. Zhu and S. Z. Luo, Chem. Rev., 2017, 117, 9433–9520 CrossRef CAS PubMed.
- R. Shang, L. Ilies and E. Nakamura, Chem. Rev., 2017, 117, 9086–9139 CrossRef CAS PubMed.
- J. Twilton, C. Le, P. Zhang, M. H. Shaw, R. W. Evans and D. W. C. MacMillan, Nat. Rev. Chem., 2017, 1, 19 CrossRef.
- H. Yi, G. T. Zhang, H. M. Wang, Z. Y. Huang, J. Wang, A. K. Singh and A. W. Lei, Chem. Rev., 2017, 117, 9016–9085 CrossRef CAS PubMed.
-
M. Yamaguchi and K. Manabe, in Site-Selective Catalysis, ed. T. Kawabata, Springer Int Publishing Ag, Cham, 2016, vol. 372, pp. 1–25 Search PubMed.
- C. R. Shugrue and S. J. Miller, Chem. Rev., 2017, 117, 11894–11951 CrossRef CAS PubMed.
- O. Robles and D. Romo, Nat. Prod. Rep., 2014, 31, 318–334 RSC.
- T. Newhouse and P. S. Baran, Angew. Chem., Int. Ed., 2011, 50, 3362–3374 CrossRef CAS PubMed.
- S. R. Neufeldt and M. S. Sanford, Acc. Chem. Res., 2012, 45, 936–946 CrossRef CAS PubMed.
- J. C. Lewis, P. S. Coelho and F. H. Arnold, Chem. Soc. Rev., 2011, 40, 2003–2021 RSC.
- H. M. L. Davies and D. Morton, Chem. Soc. Rev., 2011, 40, 1857–1869 RSC.
-
M. Canta, M. Rodriguez and M. Costas, in Site-Selective Catalysis, ed. T. Kawabata, Springer Int Publishing Ag, Cham, 2016, vol. 372, pp. 27–54 Search PubMed.
- D. C. Blakemore, L. Castro, I. Churcher, D. C. Rees, A. W. Thomas, D. M. Wilson and A. Wood, Nat. Chem., 2018, 10, 383–394 CrossRef CAS PubMed.
- M. Bietti, Angew. Chem., Int. Ed., 2018, 57, 16618–16637 CrossRef CAS PubMed.
- D. Vidal, G. Olivo and M. Costas, Chem. – Eur. J., 2018, 24, 5042–5054 CrossRef CAS PubMed.
- M. C. White and J. P. Zhao, J. Am. Chem. Soc., 2018, 140, 13988–14009 CrossRef CAS PubMed.
Footnote |
† Electronic supplementary information (ESI) available: One Excel file containing counting statistics for each manuscript included. See DOI: 10.1039/c9ob01559k |
|
This journal is © The Royal Society of Chemistry 2020 |
Click here to see how this site uses Cookies. View our privacy policy here.