DOI:
10.1039/D2QO00416J
(Research Article)
Org. Chem. Front., 2022,
9, 3540-3545
Photocatalytic access to aromatic keto sulfonyl fluorides from vinyl fluorosulfates†
Received
14th March 2022
, Accepted 9th May 2022
First published on 11th May 2022
Abstract
We present herein an efficient photocatalytic transformation of vinyl fluorosulfates to aromatic β-keto sulfonyl fluorides with 1 mol% of iridium catalyst under the irradiation of 3 W blue LEDs. Preliminary mechanistic studies proposed a direct radical fragmentation and recombination of vinyl fluorosulfates through a free fluorosulfonyl radical (FSO2˙). This methodology provides a facile approach to aromatic β-keto sulfonyl fluorides, featuring sustainable conditions and a broad substrate scope (32 examples) with 33%–90% isolated yields.
Introduction
Sulfonyl fluorides are valuable motifs not only in organic synthesis but also in chemical biology,1 medicine2 and materials science.3 Due to their special stability and reactivity pattern, sulfonyl fluorides have been widely utilized in Sulfur(VI) Fluoride Exchange (SuFEx) as the latest reaction for click chemistry, which is pioneered by Sharpless and applied widely to many research fields.4 Thus, there are increasing demands to develop efficient methods for the synthesis of various sulfonyl fluoride compounds, especially with highly-valued functionalities.
β-Keto sulfonyl fluorides have drawn special attention as they are highly functionalized with both fluorosulfonyl motifs and carbonyl groups, enabled with further possibility of post-functionalizations. Yet, compared to the well-developed methods to synthesize aliphatic5 or aromatic6 sulfonyl fluorides, strategies to prepare β-keto sulfonyl fluorides remain less explored. Early in 1990, Seppelt and co-workers reported a useful synthesis of β-keto sulfonyl fluoride through a multiple-step procedure with ketene (g) and SF5Cl (g) (Scheme 1a).7 Recently, a facile strategy to synthesize β-keto sulfonyl fluoride that relied on F–Cl exchange between β-keto sulfonyl chloride and KH2F was demonstrated by Hirai and co-workers (Scheme 1b).8 Notably, a novel synthetic method involving the formation of the FSO2 radical was developed most recently on the basis of an electrochemical oxidative process.9 The Huang and Liao groups demonstrated that β-keto sulfonyl fluorides can be synthesized through oxofluorosulfonylation of alkynes with sulfuryl chlorofluoride as the radical fluorosulfonyl source under electrochemical conditions with air as the oxidant (Scheme 1c). While impressive progress has been made to develop novel and diverse synthetic tools for the generation of β-keto sulfonyl fluorides, many of the reported examples generally require harsh conditions or usage of gaseous starting materials. Therefore, the development of new and efficient methods to synthesize β-keto fluorosulfones still remains highly desirable, especially with a broader substrate scope, milder reaction conditions and easier handling.
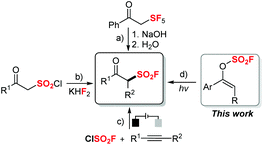 |
| Scheme 1 Synthetic approaches to β-keto sulfonyl fluorides. | |
Vinyl fluorosulfates, as versatile substrates, have been used in transition-metal-catalyzed cross-coupling processes to form C–C bonds.10 Most recently, Michaudel and co-workers reported an elegant modular synthesis of β-keto sulfonamides from alkenyl sulfamates which are rapidly generated through SuFEx chemistry with vinyl fluorosulfates.11 However, to the best of our knowledge, radical transformations directly with vinyl fluorosulfates is seldom reported. Meanwhile, a sustainable photoredox strategy has witnessed remarkable development during the last few decades and been applied in many research fields.12 Inspired by all these advances and in connection with our research interest in the synthesis of β-functionalized ketones from enolates via a radical fragmentation/recombination strategy,13 we envisioned that an efficient approach to various aromatic β-keto sulfonyl fluorides might be realized through photocatalytic radical rearrangement of vinyl fluorosulfates (Scheme 1d). Herein, we report our efforts on this approach.
Results and discussion
We initially investigated suitable reaction conditions by using vinyl fluorosulfate (1a) as the model substrate. The use of 1 mol% of [Ir(dFCF3ppy)2bbpy]PF6 (Ir 1) as the photocatalyst, 30 W blue LEDs as the light source, and ethyl ether as the solvent at room temperature for 12 hours successfully delivered 6% 19F NMR yield of the target product 2a along with 69% of unreacted 1a (Table 1, entry 1). We then carried out further solvent screening. While reactions in ethyl acetate or toluene generated 2a almost equally (33% in EA and 30% in toluene), toluene was chosen to be the optimal solvent as the substrate 1a is more stable in toluene. By applying toluene as the preferred solvent, several photoredox catalysts, such as transition-metal catalysts (Ir or Ru) and organophotocatalysts (4-CzIPN or 9-fluorenone), were then screened. [Ir(dFCF3ppy)2dtbbpy]PF6 (Ir 3) emerged as the leading catalyst that improved the yield of 2a to 48%. Upon careful evaluation of other parameters such as light sources, additives (K2CO3, Na2CO3, Et3N and 4 Å MS), the reaction time and the reaction concentration (see the ESI† for details), the optimized conditions were as follows: 1a (0.2 mmol), Ir 3 (1 mol%) and 4 Å MS (44 mg) irradiated using 3 W blue LEDs at room temperature for 12 h (Table 1, entry 15). The desired product 2a was generated in a good isolated yield of 81%, and the starting vinyl fluorosulfate 1a was almost consumed.
Table 1 Optimization of reaction conditionsa
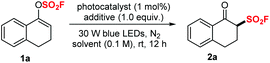
|
Entry |
Photocatalyst |
Solvent |
Additive |
2a/1a b (%) |
Reaction conditions: 1a (0.2 mmol), photocatalyst (0.002 mmol, 1 mol%) and additive in solvent (2 mL), irradiated using 30 W blue LEDs at room temperature for 12 h under N2. Ir 1: [Ir(dFCF3ppy)2bbpy]PF6; Ir2: fac-Ir(ppy)3; Ir 3: [Ir(dFCF3ppy)2dtbbpy]PF6.
Determined by crude 19F NMR analysis; isolated yield in parentheses.
Irradiated using 3 W blue LEDs.
4 Å MS (44 mg) as an additive.
|
1 |
Ir 1
|
Et2O |
— |
6/69 |
2 |
Ir 1
|
THF |
— |
18/54 |
3 |
Ir 1
|
DMF |
— |
<5/51 |
4 |
Ir 1
|
EA |
— |
33/36 |
5 |
Ir 1
|
Toluene |
— |
30/63 |
6 |
Ir 2
|
Toluene |
— |
<5/93 |
7 |
Ir 3
|
Toluene |
— |
48/21 |
8 |
Ru(bpy)3Cl2 |
Toluene |
— |
<5/96 |
9 |
4-CzIPN |
Toluene |
— |
21/60 |
10 |
9-Fluorenone |
Toluene |
— |
33/60 |
11c |
Ir 3
|
Toluene |
— |
48/39 |
12c |
Ir 3
|
Toluene |
Na2CO3 |
78/<5 |
13c |
Ir 3
|
Toluene |
K2CO3 |
51/24 |
14c |
Ir 3
|
Toluene |
Et3N |
<5/99 |
15c,d |
Ir 3
|
Toluene |
4 Å MS |
84(81)/<5 |
With the optimized conditions in hand, we proceeded to investigate the broad applicability of this new approach for a library of vinyl fluorosulfates. As shown in Table 2, a variety of aromatic vinyl fluorosulfates with diverse electronic and steric properties have displayed satisfactory to good reactivity. Functional groups such as halogens, CF3 and CN at the 3-, 4- or 5-position of the aromatic rings were well tolerated and the corresponding products were generated in 57%–87% yields (Table 2, 2b–2e, 2i–2p and 2s–2w). Substrates with alkyl, phenyl, OCF3 or OSO2F groups on aromatic rings also reacted smoothly to deliver the corresponding products in good yields of 49%–79% (Table 2, 2f–2i, 2k, 2q, 2r, 2x and 2y). Notably, substrate 1j, bearing the dimethylamino group at the 4-position on the aromatic ring, is unsuitable for this transformation, and no desired product was formed with 60% of 1j remaining. To our delight, substrate 1z, which possessed an F group at the sterically hindered 6-position of the aromatic ring, underwent this transformation with a moderate yield of 33%. However, the reaction was totally hindered with substrate 1aa harbouring a methyl group at the 6-position of the aromatic ring, and we reasoned that hydrogen abstraction at the benzylic C–H position was probably the main cause. Then we focused on the modifications to the enol motifs. To our delight, substrate 1ab bearing a β-methyl group at the enol motif could render the expected product 2ab in a synthetically useful yield of 51% with dr of 1
:
1.5. 1H-Inden-3-yl fluorosulfonates performed as good candidates, giving the desired products (2ac 90% and 2ad 76%) without loss of efficiencies. Moreover, our attempt to construct 4-oxochromane-3-sulfonyl fluoride was also successful and the target β-keto sulfonyl fluoride 2ae was formed in 57% yield. However, to our great disappointment, substrates derived from acyclic ketone (1af) or aliphatic ketone (1ag) could not generate the desired products. Additionally, substrates with sterically hindered substituents (1ah–1ak) were amenable for the transformation and the corresponding products were obtained in synthetically useful yields (40%–65%).
Table 2 Scope of substratesa
Reaction conditions: 1 (0.2 mmol), [Ir(dFCF3ppy)2dtbbpy]PF6 (0.002 mmol, 1 mol%) and 4 Å MS (44 mg) in toluene (2 mL), irradiated using 3 W blue LEDs at room temperature for 12 h under N2; isolated yields.
Irradiated for 24 h.
Na2CO3 (0.2 mmol, 1.0 equiv.) instead of 4 Å MS (44 mg).
THF as the solvent.
|
|
After the successful preparation of 2a on an 8 mmol scale with a slightly decreased yield (1.26 g, 69%) (Scheme 2a), we then investigated the diversification of 2a through SuFEx click reactions. As illustrated in Scheme 2b and c, β-keto sulfonyl fluoride 2a readily underwent SuFEx with methanol or 3-butynol, affording the corresponding sulfonate esters 3a and 3b in good to quantitative yields (95% and 72%). Furthermore, sulfonamides 3c (92%, Scheme 2d), 3d (55%, Scheme 2e) and 3e (79%, Scheme 2f) were generated smoothly through S–N bond formation reactions between 2a and a primary amine (benzyl amine) or secondary amines (N-methyl allyl amine or morphine).
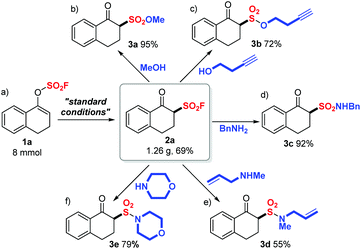 |
| Scheme 2 Gram-scale synthesis and derivatizations of 2a. | |
To gain some mechanistic insights into this transformation, we carried out several experiments. Control experiments indicated that light irradiation and a photocatalyst were essential for the success of this transformation. In the absence of an additive, a much lower yield was obtained. Notably, 1a decomposed readily with extra addition of H2O (10 mol%); therefore, the desired product 2a was formed in a dramatically decreased yield of 27%. According to these mechanistic results, we assumed that 4 Å MS could act partially as a drying agent that prevented vinyl fluorosulfates 1 from decomposition, and 4 Å MS could also work as a weak base to accelerate the transformation of vinyl fluorosulfates 1 to β-keto sulfonyl fluorides 2. A typical radical scavenger TEMPO (1.0 equiv.) was introduced to the standard reaction system with 1a and completely inhibited the reaction, suggesting the involvement of a radical mechanism (Scheme 3a). We then performed a crossover experiment by applying an equimolar amount of 1b and 5 to the standard conditions (Scheme 3b). Four possible crossover products (2b, 2a, 6 and 7) were detected by crude 19F NMR in a ratio of 1
:
1.3
:
0.5
:
0.6, suggesting the involvement of a free radical procedure.
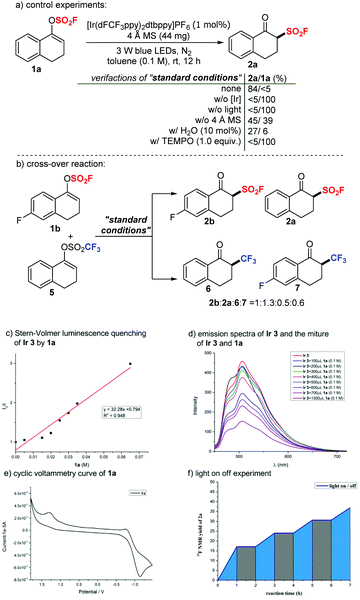 |
| Scheme 3 Mechanistic studies. | |
To further clarify the mechanism, we applied DFT calculations on the triplet energy of vinyl fluorosulfate (1a) (for more details, see the ESI†). The calculated triplet energy of 1a is 134 kJ mol−1 which is much lower than the reported ET of Ir 3 (251 kJ mol−1).14a,c Therefore, a possibility of energy transfer between the initiator and the substrate is supported. We then carried out the fluorescence quenching experiments (Stern–Volmer studies) of Ir 3. As shown in Scheme 3c, the fluorescence intensity of the photocatalyst Ir 3 decreased with increasing concentration of 1a. A clear linear relationship was observed between I0/I (I0 is the fluorescence intensity of Ir 3 before the addition of 1a and I is the fluorescence intensity after the addition) and the concentration of 1a (Scheme 3d). These fluorescence quenching results are consistent with the DFT calculations and supported a possibility of energy transfer or electron transfer between the photoexcited state Ir 3 and 1a at the initial stage. However, a reductive quenching cycle is also favored, based on the fact that the oxidation potential of 1a (Ered1/2 = +1.30 V vs. SCE, as shown in Scheme 3e) is higher than that of Ir 3 (
vs. SCE).14b Light on/off experiments with 1a under the standard conditions are shown in Scheme 3f. The fact that the reaction proceeded under the irradiation of light and almost stopped without light verified the possibility of a photocatalytic mechanism. Furthermore, the apparent quantum efficiency of the model transformation with 1a was calculated to be 0.86, which indicates a photocatalytic mechanism (details in the ESI†).
According to the mechanistic studies and our previous work,13 we proposed a possible reaction pathway as follows (Scheme 4). Through energy transfer from the excited iridium(III) catalyst, vinyl fluorosulfate 1 underwent homolytic decomposition to generate the enol radical and the fluorosulfonyl radical. Subsequent radical reconstruction of the fluorosulfonyl radical and the enol radical would eventually form the desired product 2 (path A). Alternatively, a redox pathway via the reduction of 1 to a radical anion I by Ir(III)*, followed by radical fragmentation/reconstruction and oxidation, may also be involved to some extent (path B). At this stage, we could not eliminate either of these two pathways.
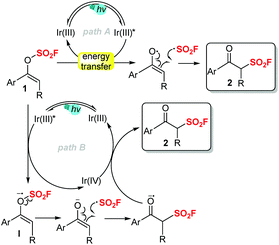 |
| Scheme 4 Proposed mechanism. | |
Conclusions
In conclusion, a photocatalytic procedure to synthesize β-keto sulfonyl fluorides has been developed via radical fragmentation and recombination of vinyl fluorosulfates. 3 W blue LEDs were applied as the light source with 1 mol% of iridium(III) catalyst. This sustainable strategy enables the rapid and efficient transformation of a number of vinyl fluorosulfates into various valuable β-keto sulfonyl fluorides which would be widely utilized in SuFEx chemistry. We anticipate that this sustainable rearrangement reaction will provide an efficient strategy to synthesize various potentially valuable β-keto sulfonyl fluorides and contribute to their advanced studies and applications.
Author contributions
Dr X. S. and Dr Y. L. conceived the project and designed the experiments. J. C., S. W. and W. L. carried out the experiments. J. C., S. K. and J. Z. analysed the experimental data. S. X. performed the DFT calculations. Dr Y. L. and J. C. wrote the manuscript. All the authors discussed the results and commented on the manuscript.
Conflicts of interest
There are no conflicts to declare.
Acknowledgements
This work is financially supported by the National Natural Science Foundation of China (21871049), the Shaanxi Provincial Key Laboratory Project (No. 19JS007), and the Scientific and Technological Innovation Team of Shaanxi Province (2022TD-36).
Notes and references
-
(a) A. M. Gold, Sulfonyl Fluorides as Inhibitors of Esterases. III. Identification of Serine as the Site of Sulfonylation in Phenylmethanesulfonyl α-Chymotrypsin, Biochemistry, 1965, 4, 897–901 CrossRef CAS PubMed;
(b) A. J. Brouwer, T. Ceylan, A. M. Jonker, T. van der Linden and R. M. J. Liskamp, Synthesis and Biological Evaluation of Novel Irreversible Serine Protease Inhibitors Using Amino Acid Based Sulfonyl Fluorides as an Electrophilic Trap, Bioorg. Med. Chem., 2011, 19, 2397–2406 CrossRef CAS PubMed;
(c) A. J. Brouwer, N. H. Álvarez, A. Ciaffoni, H. van de Langemheen and R. M. J. Liskamp, Proteasome Inhibition by New Dual Warhead Containing Peptido Vinyl Sulfonyl Fluorides, Bioorg. Med. Chem., 2016, 24, 3429–3435 CrossRef CAS PubMed;
(d) J. M. Hatcher, G. Wu, C. Zeng, J. Zhu, F. Meng, S. Patel, W. Wang, S. B. Ficarro, A. L. Leggett, C. E. Powell, J. A. Marto, K. Zhang, J. C. K. Ngo, X.-D. Fu, T. Zhang and N. S. Gray, SRPKIN-1: A Covalent SRPK1/2 Inhibitor that Potently Converts VEGF from Pro-Angiogenic to Anti-Angiogenic Isoform, Cell Chem. Biol., 2018, 25, 460–470 CrossRef CAS PubMed.
-
(a) A. J. Brouwer, A. Jonker, P. Werkhoven, E. Kuo, N. Li, N. Gallastegui, J. Kemmink, B. I. Florea, M. Groll, H. S. Overkleeft and R. M. J. Liskamp, Peptido Sulfonyl Fluorides as New Powerful Proteasome Inhibitors, J. Med. Chem., 2012, 55, 10995–11003 CrossRef CAS PubMed;
(b) L. H. Jones, Emerging Utility of Fluorosulfate Chemical Probes, ACS Med. Chem. Lett., 2018, 9, 584–586 CrossRef CAS PubMed;
(c) C. Baggio, P. Udompholkul, L. Gambini, A. F. Salem, J. Jossart, J. J. P. Perry and M. Pellecchia, Aryl-fluorosulfate-Based Lysine Covalent Pan-Inhibitors of Apoptosis Protein (IAP) Antagonists with Cellular Efficacy, J. Med. Chem., 2019, 62, 9188–9200 CrossRef CAS PubMed.
-
(a) S. Tschan, A. J. Brouwer, P. R. Werkhoven, A. M. Jonker, L. Wagner, S. Knittel, M. N. Aminake, G. Pradel, F. Joanny, R. M. Liskamp and B. Mordmuller, Broad-Spectrum Antimalarial Activity of Peptido Sulfonyl Fluorides, a New Class of Proteasome Inhibitors, Antimicrob. Agents Chemother., 2013, 57, 3576–3584 CrossRef CAS PubMed;
(b) C. Yang, J. P. Flynn and J. Niu, Facile Synthesis of Sequence-Regulated Synthetic Polymers Using Orthogonal SuFEx and CuAAC Click Reactions, Angew. Chem., Int. Ed., 2018, 57, 16194–16199 CrossRef CAS PubMed;
(c) Q. Zheng, H. Xu, H. Wang, W.-G. H. Du, N. Wang, H. Xiong, Y. Gu, L. Noodleman, K. B. Sharpless, G. Yang and P. Wu, Sulfur [18F]Fluoride Exchange Click Chemistry Enabled Ultrafast Late-Stage Radiosynthesis, J. Am. Chem. Soc., 2021, 143, 3753–3763 CrossRef CAS PubMed;
(d) N. Suto, S. Kamoshita, S. Hosoya and K. Sakurai, Exploration of the Reactivity of Multivalent Electrophiles for Affinity Labeling: Sulfonyl Fluoride as a Highly Efficient and Selective Label, Angew. Chem., Int. Ed., 2021, 60, 17080–17087 CrossRef CAS PubMed.
- For reviews:
(a) H. C. Kolb, M. G. Finn and K. B. Sharpless, Click Chemistry: Diverse Chemical Function from a Few Good Reactions, Angew. Chem., Int. Ed., 2001, 40, 2004–2021 CrossRef CAS;
(b) P. K. Chinthakindi and P. I. Arvidsson, Sulfonyl Fluorides (SFs): More Than Click Reagents?, Eur. J. Org. Chem., 2018, 3648–3666 CrossRef CAS;
(c) A. S. Barrow, C. J. Smedley, Q. Zheng, S. Li, J. Dong and J. E. Moses, The Growing Applications of SuFEx Click Chemistry, Chem. Soc. Rev., 2019, 48, 4731–4758 RSC;
(d) L. Xu and J. Dong, Click Chemistry: Evolving on the Fringe, Chin. J. Chem., 2020, 38, 414–419 CrossRef CAS. For selected examples:
(e) S. Li, P. Wu, J. E. Moses and K. B. Sharpless, Multidimensional SuFEx Click Chemistry: Sequential Sulfur(VI) Fluoride Exchange Connections of Diverse Modules Launched from an SOF4 Hub, Angew. Chem., Int. Ed., 2017, 56, 2903–2908 CrossRef CAS PubMed;
(f) B. Gao, S. Li, P. Wu, J. E. Moses and K. B. Sharpless, SuFEx Chemistry of Thionyl Tetrafluoride (SOF4) with Organolithium Nucleophiles: Synthesis of Sulfonimidoyl Fluorides, Sulfoximines, Sulfonimidamides and Sulfonimidates, Angew. Chem., Int. Ed., 2018, 57, 1939–1943 CrossRef CAS PubMed;
(g) D. E. Mortenson, G. J. Brighty, L. Plate, G. Bare, W. Chen, S. Li, H. Wang, B. F. Cravatt, S. Forli, E. T. Powers, K. B. Sharpless, I. A. Wilson and J. W. Kelly, “Inverse Drug Discovery” Strategy to Identify Proteins That Are Targeted by Latent Electrophiles as Exemplified by Aryl Fluorosulfates, J. Am. Chem. Soc., 2018, 140, 200–210 CrossRef CAS PubMed;
(h) C. Lee, A. J. Cook, J. E. Elisabeth, N. C. Friede, G. M. Sammis and N. D. Ball, The Emerging Applications of Sulfur(VI) Fluorides in Catalysis, ACS Catal., 2021, 11, 6578–6589 CrossRef CAS PubMed;
(i) C. J. Smedley, J. A. Homer, T. L. Gialelis, A. S. Barrow, R. A. Koelln and J. E. Moses, Accelerated SuFEx Click Chemistry for Modular Synthesis, Angew. Chem., 2022, 61, e202112375 CAS.
-
(a) J. Dong, L. Krasnova, M. G. Finn and K. B. Sharpless, Sulfur(VI) Fluoride Exchange (SuFEx): Another Good Reaction for Click Chemistry, Angew. Chem., Int. Ed., 2014, 53, 9430–9448 CrossRef CAS PubMed;
(b) H.-L. Qin, Q. Zheng, G. A. L. Bare, P. Wu and K. B. Sharpless, A Heck-Matsuda Process for the Synthesis of β-Arylethenesulfonyl Fluorides: Selectively Addressable Bis-electrophiles for SuFEx Click Chemistry, Angew. Chem., Int. Ed., 2016, 55, 14155–14158 CrossRef CAS PubMed;
(c) A. Shavnya, S. B. Coffey, K. D. Hesp, S. C. Ross and A. S. Tsai, Reaction of Alkyl Halides with Rongalite: One-Pot and Telescoped Syntheses of Aliphatic Sulfonamides, Sulfonyl Fluorides and Unsymmetrical Sulfones, Org. Lett., 2016, 18, 5848–5851 CrossRef CAS PubMed;
(d) P. K. Chinthakindi, K. B. Govender, A. S. Kumar, H. G. Kruger, T. Govender, T. Naicker and P. I. Arvidsson, A Synthesis of “Dual Warhead” β-Aryl Ethenesulfonyl Fluorides and One-Pot Reaction to β-Sultams, Org. Lett., 2017, 19, 480–483 CrossRef CAS PubMed;
(e) A. Talko and M. Barbasiewicz, Nucleophilic Fluorination with Aqueous Bifluoride Solution: Effect of the Phase-Transfer Catalyst, ACS Sustainable Chem. Eng., 2018, 6, 6693–6701 CrossRef CAS;
(f) G. Laudadio, A. de A. Bartolomeu, L. M. H. M. Verwijlen, Y. Cao, K. T. de Oliveira and T. Noël, Sulfonyl Fluoride Synthesis through Electrochemical Oxidative Coupling of Thiols and Potassium Fluoride, J. Am. Chem. Soc., 2019, 141, 11832–11836 CrossRef CAS PubMed;
(g) C. J. Smedley, G. Li, A. S. Barrow, T. L. Gialelis, M.-C. Giel, A. Ottonello, Y. Cheng, S. Kitamura, D. W. Wolan, K. B. Sharpless and J. E. Moses, Diversity Oriented Clicking (DOC): Divergent Synthesis of SuFExable Pharmacophores from 2-Substituted-Alkynyl-1-Sulfonyl Fluoride (SASF) Hubs, Angew. Chem., Int. Ed., 2020, 59, 12460–12469 CrossRef CAS PubMed;
(h) X. Nie, T. Xu, J. Song, A. Devaraj, B. Zhang, Y. Chen and S. Liao, Radical Fluorosulfonylation: Accessing Alkenyl Sulfonyl Fluorides from Alkenes, Angew. Chem., Int. Ed., 2021, 60, 3956–3960 CrossRef CAS PubMed;
(i) X. Nie, T. Xu, Y. Hong, H. Zhang, C. Mao and S. Liao, Introducing A New Class of Sulfonyl Fluoride Hubs via Radical Chloro-Fluorosulfonylation of Alkynes, Angew. Chem., Int. Ed., 2021, 60, 22035–22042 CrossRef CAS PubMed;
(j) N. L. Frye, C. G. Daniliuc and A. Studer, Radical 1-Fluorosulfonyl-2-alkynylation of Unactivated Alkenes, Angew. Chem., 2022, 61, e202115593 CAS.
-
(a) L. Tang, Y. Yang, L. Wen, X. Yang and Z. Wang, Catalyst-Free Radical Fluorination of Sulfonyl Hydrazides in Water, Green Chem., 2016, 18, 1224–1228 RSC;
(b) L. Revathi, L. Ravindar, J. Leng, K. P. Rakesh and H.-L. Qin, Synthesis and Chemical Transformations of Fluorosulfates, Asian J. Org. Chem., 2018, 7, 662–682 CrossRef CAS;
(c) C. Lee, N. D. Ball and G. M. Sammis, One-pot Fluorosulfurylation of Grignard Reagents Using Sulfuryl Fluoride, Chem. Commun., 2019, 55, 14753–14756 RSC;
(d) L. Wang and J. Cornella, A Unified Strategy for Arylsulfur(VI) Fluorides from Aryl Halides: Access to Ar-SOF3 Compounds, Angew. Chem., Int. Ed., 2020, 59, 23510–23515 CrossRef CAS PubMed;
(e) Y. Liu, D. Yu, Y. Guo, J. C. Xiao, Q. Y. Chen and C. Liu, Arenesulfonyl Fluoride Synthesis via Copper-Catalyzed Fluorosulfonylation of Arenediazonium Salts, Org. Lett., 2020, 22, 2281–2286 CrossRef CAS PubMed;
(f) T. Zhong, J. Yi, Z. Chen, Q. Zhuang, Y. Li, G. Lu and J. Weng, Photoredox-catalyzed Aminofluorosulfonylation of Unactivated Olefins, Chem. Sci., 2021, 7, 9359–9365 RSC;
(g) M. Magre and J. Cornella, Redox-Neutral Organometallic Elementary Steps at Bismuth: Catalytic Synthesis of Aryl Sulfonyl Fluorides, J. Am. Chem. Soc., 2021, 143, 21497–21502 CrossRef CAS PubMed;
(h) Z. Ma, Y. Liu, X. Ma, X. Hu, Y. Guo, Q.-Y. Chen and C. Liu, Aliphatic Sulfonyl Fluoride Synthesis via Reductive Decarboxylative Fluorosulfonylation of Aliphatic Carboxylic Acid NHPI Esters, Org. Chem. Front., 2022, 9, 1115–1120 RSC.
- T. Henkel, T. Krgerke and K. Seppelt, Isomerization of Benzoylalkylidene Sulfur Tetrafluorides C6H5-CO-CR-SF4 to Dihydrooxathietes, Angew. Chem., Int. Ed. Engl., 1990, 29, 1128–1129 CrossRef.
-
(a)
T. Hirai, D. Jomuta and C. Tamitsuji, Fluorosulfonyl Group-Containing Compound, Fluorosulfonyl Group-Containing Monomer and Their Production Methods, US 2020/0190025 A1, 2020;
(b)
T. Hirai, H. Watabe and S. Saito, Sulfonic Acid Group-Containing Polymer, Fluorosulfonyl Group-Containing Polymer and Liquid Coposotion, US 2021/0380732 A1, 2021.
- D. Chen, X. Nie, Q. Feng, Y. Zhang, Y. Wang, Q. Wang, L. Huang, S. Huang and S. Liao, Electrochemical Oxo-Fluorosulfonylation of Alkynes under Air: Facile Access to β-Keto Sulfonyl Fluorides, Angew. Chem., Int. Ed., 2021, 60, 27271–27276 CrossRef CAS PubMed.
-
(a) P. S. Hanley, M. S. Ober, A. L. Krasovskiy, G. T. Whiteker and W. J. Kruper, Nickel- and Palladium-Catalyzed Coupling of Aryl Fluorosulfonates with Aryl Boronic Acids Enabled by Sulfuryl Fluoride, ACS Catal., 2015, 5, 5041–5046 CrossRef CAS;
(b) Q. Liang, P. Xing, Z. Huang, J. Dong, K. B. Sharpless, X. Li and B. Jiang, Palladium-Catalyzed, Ligand-Free Suzuki Reaction in Water Using Aryl Fluorosulfates, Org. Lett., 2015, 17, 1942–1945 CrossRef CAS PubMed;
(c) A. Markos, S. Voltrová, V. Motornov, D. Tichý, B. Klepetářová and P. Beier, Stereoselective Synthesis of (Z)-β-Enamido Triflates and Fluorosulfonates from N-Fluoroalkylated Triazoles, Chem. – Eur. J., 2019, 25, 7640–7644 CrossRef CAS PubMed;
(d) M. Mendel, I. Kalvet, D. Hupperich, G. Magnin and F. Schoenebeck, Site-Selective, Modular Diversification of Polyhalogenated Aryl Fluorosulfates (ArOSO2F) Enabled by an Air-Stable PdI Dimer, Angew. Chem., Int. Ed., 2020, 59, 2115–2119 CrossRef CAS PubMed.
- F. C. S. Silva, K. Doktor and Q. Michaudel, Modular Synthesis of Alkenyl Sulfamates and β-Ketosulfonamides via Sulfur(VI) Fluoride Exchange (SuFEx) Click Chemistry and Photomediated 1,3-Rearrangement, Org. Lett., 2021, 23, 5271–5276 CrossRef PubMed.
-
(a) N. A. Romero and D. A. Nicewicz, Organic Photoredox Catalysis, Chem. Rev., 2016, 116, 10075–10166 CrossRef CAS PubMed;
(b) C. S. Wang, P. H. Dixneuf and J. F. Soule, Photoredox Catalysis for Building C–C Bonds from C(sp2)–H Bonds, Chem. Rev., 2018, 118, 7532–7585 CrossRef CAS PubMed;
(c) X.-Y. Yu, J.-R. Chen and W.-J. Xiao, Visible Light-Driven Radical-Mediated C–C Bond Cleavage/Functionalization in Organic Synthesis, Chem. Rev., 2021, 121, 506–561 CrossRef CAS PubMed;
(d) N. H. Douglas and D. A. Nicewicz, Photoredox-Catalyzed C–H Functionalization Reactions, Chem. Rev., 2022, 122, 1925–2016 CrossRef PubMed;
(e) A. Y. Chan, I. B. Perry, N. B. Bissonnette, B. F. Buksh, G. A. Edwards, L. I. Frye, O. L. Garry, M. N. Lavagnino, B. X. Li, Y. Liang, E. Mao, A. Millet, J. V. Oakley, N. L. Reed, H. A. Sakai, C. P. Seath and D. W. C. MacMillan, Metallaphotoredox: The Merger of Photoredox and Transition Metal Catalysis, Chem. Rev., 2022, 122, 1485–1542 CrossRef CAS PubMed.
-
(a) X. Su, H. Huang, Y. Yuan and Y. Li, Radical Desulfur-Fragmentation and Reconstruction of Enol Triflates: Facile Access to α-Trifluoromethyl Ketones, Angew. Chem., In. Ed., 2017, 56, 1338–1341 CrossRef CAS PubMed;
(b) L. Xie, X. Zhen, S. Huang, X. Su, M. Lin and Y. Li, Photoinduced Rearrangement of Vinyl Tosylates to β-Ketosulfones, Green Chem., 2017, 19, 3530–3534 RSC.
-
(a) J. I. Day, K. Teegardin, J. Weaver and J. Chan, Advances in Photocatalysis: A Microreview of Visible Light Mediated Ruthenium and Iridium Catalyzed Organic Transformations, Org. Process Res. Dev., 2016, 20, 1156–1163 CrossRef PubMed;
(b) N. A. Romero and D. A. Nicewicz, Organic Photoredox Catalysis, Chem. Rev., 2016, 116, 10075–10166 CrossRef CAS PubMed;
(c) M. R. Becker, E. R. Wearing and C. S. Schindler, Synthesis of Azetidines via Visible-Light-Mediated Intermolecular [2+2] Photocycloaddition, Nat. Chem., 2020, 12, 898–905 CrossRef CAS PubMed.
|
This journal is © the Partner Organisations 2022 |