DOI:
10.1039/D1QO01655E
(Research Article)
Org. Chem. Front., 2022,
9, 1115-1120
Aliphatic sulfonyl fluoride synthesis via reductive decarboxylative fluorosulfonylation of aliphatic carboxylic acid NHPI esters†
Received
3rd November 2021
, Accepted 9th January 2022
First published on 11th January 2022
Abstract
Based on a radical sulfur dioxide insertion and fluorination strategy, we have developed an efficient method for aliphatic sulfonyl fluoride synthesis using abundant carboxylic acids, a reductant, a sulfur dioxide surrogate and the electrophilic fluorination reagent N-fluorobenzenesulfonimide (NFSI) under reduction conditions. This protocol provides a convenient synthetic pathway for various aliphatic sulfonyl fluorides and tolerates a wide range of functional groups.
Introduction
The last two decades have witnessed the rapid development of click chemistry introduced by Sharpless and co-workers in many research fields.1 Since sulfonyl fluorides have a special stability–reactivity pattern, Sulfur(VI) Fluoride Exchange (SuFEx) as the latest reaction identified for click chemistry has attracted enormous attention and has been widely utilized in not only organic synthesis but also chemical biology, medicine, and materials science,2 which has led to a fast-growing demand for chemists to develop reliable methods for the incorporation of this highly valued sulfonyl fluoride group (SO2F) into various organic compounds.
Conventional approaches for the formation of sulfonyl fluorides typically involve the fluorosulfonylation of thiols and their derivatives or ultilization of FSO2-containing synthons.3 These approaches do not involve the formation of the C–SO2F bond. An alternative attractive and straightforward strategy for the introduction of the sulfonyl fluoride group would be the direct formation of a new C–SO2F bond. In recent years, several elegant methods based on transition-metal catalyzed cross-coupling,4 electrophilic fluorosulfonylating reagents,5 fluorosulfonyl radical addition6 or radical sulfur dioxide insertion and fluorination7,8 have emerged for the effective incorporation of SO2F into a variety of organic small molecules. However, the reactions typically lead to the formation of Csp2–SO2F bonds, whereas processes that involve the formation of Csp3–SO2F bonds remain largely unexplored.
Although aliphatic sulfonyl fluorides may have highly selective peptide-type inhibition activity (Fig. 1A) and high potential in SuFEx chemistry,2 their limited availability significantly hampered their study and application. As can be seen in Fig. 1B, the main synthetic approaches without the formation of the C–SO2F bond for aliphatic sulfonyl fluorides rely on F–Cl exchange of the corresponding aliphatic sulfonyl chlorides,2a,9 oxidative fluorination of thiols and their derivatives,10 and various addition reactions of ethenesulfonyl fluoride (ESF).11 Notably, two novel synthetic methods involving the formation of Csp3–SO2F bonds were developed recently on the basis of the nucleophilic substitution process.12 Shavnya and co-workers reported that aliphatic sulfonyl fluorides can be synthesized from nucleophilic substitution of alkyl halides with cheap rongalite as the sulfonyl source followed by fluorination with N-fluorobenzenesulfonimide (NFSI) (Fig. 1Ba).12a,b More recently, Sammis and co-workers demonstrated that aliphatic sulfonyl fluoride synthesis can be realized through the nucleophilic substitution of sulfuryl fluoride (SO2F2) by Grignard reagents (Fig. 1Bb).12c Furthermore, based on the new strategy of radical sulfur dioxide insertion and fluorination,7 we reported several new methods to efficiently synthesize various aliphatic sulfonyl fluorides through perfluoroalkyl radical addition with a variety of alkenes followed by radical sulfur dioxide insertion and oxidative fluorination by NFSI (Fig. 1Bd).7a–c Very recently, the MacMillan group reported the direct conversion of aliphatic C(sp3)–H bonds into the corresponding alkyl sulfinic acids via decatungstate photocatalysis, and that fluorination of sulfinic acids with selectfluor can result in the desired aliphatic sulfonyl fluorides (Fig. 1Bc).13 The Willis group reported that versatile alkyl sulfinates can be prepared from readily available amines, using Katritzky pyridinium salt intermediates by a photoinduced or thermally induced single-electron transfer (SET) process, and fluorination of alkyl sulfinates with NFSI can lead to the desired aliphatic sulfonyl fluorides as well (Fig. 1Bc).14 Despite these invaluable advances, the development of general and efficient methods for aliphatic sulfonyl fluoride synthesis is still in high demand.
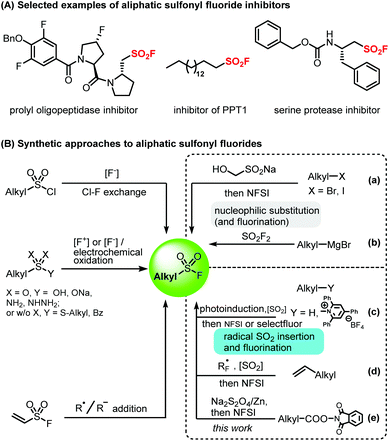 |
| Fig. 1 Aliphatic sulfonyl fluoride inhibitors and the synthetic approaches to aliphatic sulfonyl fluorides. | |
Aliphatic carboxylic acids are abundant and easily available feedstocks, and have been widely utilized as an ideal type of building block in organic synthesis.15 In recent years, their important and valuable utilization is as a convenient radical source via reductive decarboxylation after activation in the form of N-hydroxyphthalimide (NHPI) esters.15d–g Inspired by this progress and in connection with our research interest in sulfonyl fluoride synthesis via a radical sulfur dioxide insertion and fluorination strategy,7 we envisioned that the combination of aliphatic carboxylic acid as a radical source with an appropriate SO2 and fluorine source would forge a general and efficient approach to various aliphatic sulfonyl fluorides (Fig. 1Be). Herein, we report our effort on this approach. The wide availability of carboxylic acids and practical reaction conditions allow for a fast construction of a variety of diverse aliphatic sulfonyl fluorides. Further diversification of the products is also demonstrated and utilization of this method for the preparation of some pharmaceutically important motifs would be expected.
Results and discussion
We commenced our study of the desired decarboxylative fluorosulfonylation of aliphatic carboxylic acids via the oxidative decarboxylation process by using cyclohexane carboxylic acid as the model substrate, the 1,4-diazabicyclo[2.2.2]-octane-bis(sulfur dioxide) adduct (DABSO) as the sulfur dioxide surrogate, NFSI or KF as the fluoride source and K2S2O8 as the oxidant with a catalytic amount of AgNO3 in N,N-dimethylformamide (DMF)/H2O (3
:
1 v/v) at 80 °C for 9 h (Fig. 2a). But no formation of the desired fluorosulfonylation product 2m was observed, which might be due to the complicated and incompatible oxidation and reduction process existing in the reaction system. We then turned our attention toward the reductive decarboxylation process for the desired decarboxylative fluorosulfonylation of aliphatic carboxylic acids by using the corresponding cyclohexane carboxylic acid NHPI ester 1m as the model substrate and Na2S2O4 as both the sulfur dioxide surrogate and reductant16 in DMF/H2O (3
:
1 v/v) at 80 °C for 9 h (Fig. 2b). To our delight, following rapid fluorination with NFSI, the reaction successfully provided the desired fluorosulfonylation product 2m in 30% 19F NMR yield.
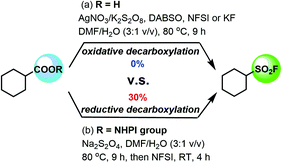 |
| Fig. 2 Initial decarboxylative fluorosulfonylation attempt on aliphatic carboxylic acids. | |
Encouraged by this result, subsequent extensive screening of the reaction conditions revealed that the optimized conditions for the desired decarboxylative fluorosulfonylation of aliphatic carboxylic acids were as follows: aliphatic carboxylic acid NHPI ester (1.0 equiv.), Na2S2O4 (1.5 equiv.), Zn (2.0 equiv.), N,N-dimethylpropionamide (DMPr)/H2O (5
:
1 v/v), Ar atmosphere, 80 °C, 9 h; then NFSI (3.0 equiv.), room temperature, 4 h (Table S8,† entry 1). Replacement of Na2S2O4 with other sulfur dioxide surrogates including DABSO or K2S2O5 resulted in a lower yield of the desired product (Table S8,† entries 2 and 3). Though Na2S2O4 can act as both the sulfur dioxide surrogate and reductant, it was found that zinc powder played an important role in the reaction since the reaction gave a lower yield of 2m by switching zinc with other metals including copper and manganese or in the absence of zinc (Table S8,† entries 4–6). Notably, replacement of DMPr with other common solvents, such as DMF or CH3CN, afforded lower yields of 2m (Table S8,† entries 7 and 8), demonstrating the unique solvent effect of DMPr. Moreover, water was found to be vital for the desired reaction because no formation of 2m was observed in its absence (Table S8,† entry 9). Finally, further examination of reaction temperatures showed that lower or higher reaction temperatures had a deleterious effect on the reaction because 20 °C, 60 °C or 100 °C led to lower yields of the target product 2m (Table S8,† entries 10–12). Detailed screening information on the reaction conditions can be found in the ESI.†
With the optimal reaction conditions successfully established, we next engaged in investigating the substrate scope of the reductive decarboxylative fluorosulfonylation of aliphatic carboxylic acids and the results are presented in Fig. 3. A wide range of aliphatic carboxylic acids including various primary, secondary, and tertiary aliphatic carboxylic acids can be applied to this transformation, providing the target aliphatic sulfonyl fluorides in good yields. For example, a number of primary aliphatic carboxylic acids underwent smooth reductive decarboxylative fluorosulfonylation to give the desired aliphatic sulfonyl fluorides in modest to good yields (2a–l). When secondary aliphatic carboxylic acids were utilized as the substrates, the target products were successfully produced in nice yields (2m–p). Tertiary aliphatic carboxylic acids could also be applied to the reactions to provide the corresponding products in acceptable yields (2q–t). Gratifyingly, the reaction showed good compatibility with a variety of functional groups, including ether (2g, 2i, 2n, 2y), halide (2e, 2k, 2l, 2u), ketone (2d, 2w, 2x, 2bb), ester (2t), alkenyl (2z), and hydroxyl (2bb) groups. Notably, substrates derived from benzyl carboxylic acids are suitable candidates for the protocol and various benzylic sulfonyl fluorides (2h–2l, 2p, 2u–2y) were obtained in good yields. Interestingly, benzylic sulfonyl fluorides 2x, 2y, and 2z are not very stable, and cannot be subjected to column chromatography purification. Consequently, their corresponding derivatives were generated from their reactions with 1-naphthol to unambiguously characterize them. Finally, the superiority of this transformation was further demonstrated in the decarboxylative fluorosulfonylation of various natural or pharmaceutical molecules. The carboxylic groups of drugs such as flurbiprofen, ibuprofen, loxoprofen, ketoprofen, and naproxen could be smoothly transformed into the sulfonyl fluoride group in high yields by this decarboxylative fluorosulfonylation approach (2u–2y). Additionally, the reductive decarboxylative fluorosulfonylation of several natural carboxylic acids (2z, 2aa, 2bb) also permits efficient introduction of a sulfonyl fluoride group in good yields.
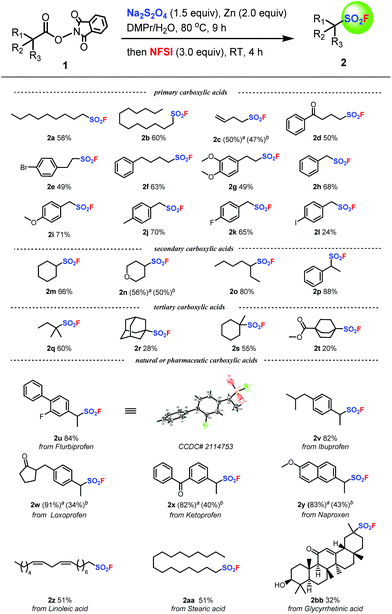 |
| Fig. 3 Reaction conditions: 1 (0.4 mmol), Na2S2O4 (0.6 mmol) and zinc (0.8 mmol) were reacted in a solvent system at 80 °C under the protection of Ar for 6–9 h, and then NFSI (3 equiv.) was added at room temperature for 4 h. Unless noted otherwise, the yields are of the isolated material. a Due to the high volatility or instability of the products, the yields were determined by 19F NMR spectroscopy using 1-methoxy-4-(trifluoromethoxy)benzene as an internal standard. b Derivatization of the desired sulfonyl fluorides with 1-naphthol was carried out in one pot and the isolated yields of the corresponding derivatives are reported. | |
To broaden the scope and utility of this protocol, we decided to investigate the derivatization reactions of the aliphatic sulfonyl fluorides acquired. As shown in Fig. 4, the desired aliphatic sulfonyl fluoride 2v was obtained in 70% isolated yield on a 6.0 mmol scale under the standard conditions via reductive decarboxylative fluorosulfonylation of ibuprofen, demonstrating the good viability of the transformation for scale-up. The aliphatic sulfonyl fluoride 2v obtained was then treated with different N- or O-nucleophiles to give the corresponding products in good yields (3–6), which might be potentially useful molecules for organic synthesis or drug research.
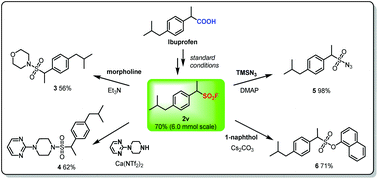 |
| Fig. 4 Scale-up and derivatization reactions of the aliphatic sulfonyl fluoride 2v achieved via reductive decarboxylative fluorosulfonylation of ibuprofen. | |
Next, we conducted several preliminary control experiments to shed light on the mechanism of this transformation (Fig. 5). First, a radical scavenger 2,2,6,6-tetramethyl-1-piperidinyloxy (TEMPO) was added to the reductive decarboxylative fluorosulfonylation reaction of 1a under the standard reaction conditions, resulting in an obvious decrease in the yield and the observation of TEMPO-trapped complex 7 (Fig. 5A). Second, compound 8 as a radical probe was subjected to the standard reaction conditions, and the corresponding ring-opened fluorosulfonylation product 2c was obtained in 43% 19F NMR yield (Fig. 5B). When compound 9 was utilized as a radical probe in the reaction to monitor if a radical intermediate was involved in the reaction, in addition to the desired product 11, the alkyl radical formed in situ by reductive decarboxylation did undergo irreversible intramolecular cyclization to successfully produce the ring-closed product 10 (Fig. 5B). All these observations suggested that the reaction might proceed via a free radical pathway. On the basis of all the experimental results presented above and the literature,16,17 the plausible reaction mechanism is proposed as shown in Fig. 5C. Reductive decarboxylation of aliphatic carboxylic acid NHPI ester 1 by zinc or Na2S2O4 generates the corresponding alkyl radical. This is rapidly trapped by the SO2 radical anion generated from Na2S2O4 to form the corresponding alkyl sulfinate. The desired aliphatic sulfonyl fluoride 2 was finally produced by subsequent rapid fluorination of the resulting alkyl sulfinate by NFSI.
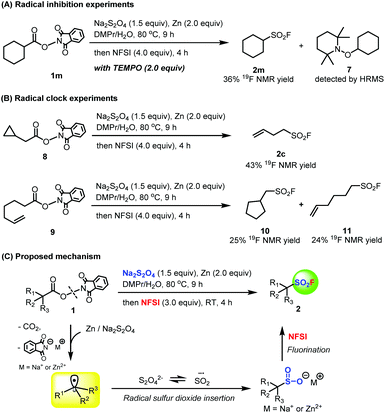 |
| Fig. 5 Control experiments and the proposed mechanism. | |
Conclusions
In conclusion, a reductive decarboxylative fluorosulfonylation reaction of aliphatic carboxylic acid NHPI ester has been developed via a radical sulfur dioxide insertion and fluorination strategy. Cheap and convenient Na2S2O4 was used as the sulfur dioxide surrogate. This method enables rapid and efficient transformation of a number of abundant aliphatic carboxylic acids, including primary, secondary, and tertiary ones, as well as several natural and pharmaceutical carboxylic acids, into various valuable aliphatic sulfonyl fluorides. We anticipate that this reductive decarboxylative fluorosulfonylation reaction will provide a useful method to synthesize various potentially important aliphatic sulfonyl fluorides and promote their further study and application.
Conflicts of interest
The authors declare no competing financial interest.
Acknowledgements
The authors gratefully acknowledge the financial support from the National Natural Science Foundation of China (No. 21871283), the project of Science and Technology Commission of Shanghai Municipality in China (21010503800), the Key Laboratory of Organofluorine Chemistry, Shanghai Institute of Organic Chemistry, Chinese Academy of Sciences, Shanghai Engineering Research Center of Green Fluoropharmaceutical Technology, and the Science Research Foundation of Shanghai Institute of Technology.
Notes and references
- H. C. Kolb, M. G. Finn and K. B. Sharpless, Click chemistry: Diverse Chemical Function from a Few Good Reactions, Angew. Chem., Int. Ed., 2001, 40, 2004 CrossRef CAS PubMed.
-
(a) J. Dong, L. Krasnova, M. G. Finn and K. B. Sharpless, Sulfur(VI) Fluoride Exchange (SuFEx): Another Good Reaction for Click Chemistry, Angew. Chem., Int. Ed., 2014, 53, 9430 CrossRef CAS PubMed;
(b) A. Barrow, C. J. Smedley, Q. Zheng, S. Li, J. Dong and J. E. Moses, The Growing Applications of SuFEx Click Chemistry, Chem. Soc. Rev., 2019, 48, 4731 RSC;
(c) L. Xu and J. Dong, Click Chemistry: Evolving on the Fringe, Chin. J. Chem., 2020, 38, 414 CrossRef CAS;
(d) L. H. Jones, Emerging Utility of Fluorosulfate Chemical Probes, ACS Med. Chem. Lett., 2018, 9, 584 CrossRef CAS PubMed;
(e) Z. Liu, J. Li, S. Li, G. Li, K. B. Sharpless and P. Wu, SuFEx Click Chemistry Enabled Late-stage Drug Functionalization, J. Am. Chem. Soc., 2018, 140, 2919 CrossRef CAS PubMed.
- For recent reviews on the synthesis of sulfonyl fluorides, see:
(a) T. Zhong, Z. Chen, J. Yi, G. Lu and J. Weng, Recent Progress in the Synthesis of Sulfonyl Fluorides for SuFx Click Chemistry, Chin. Chem. Lett., 2021, 32, 2736 CrossRef CAS;
(b) A. Chelagha, D. Louvel, A. Taponard, R. Berthelon and A. Tlili, Synthetic Routes to Arylsulfonyl Fluorides, Catalysts, 2021, 11, 830 CrossRef CAS;
(c) C. Lee, A. Cook, J. E. Elisabeth, N. C. Friede, G. M. Sammis and N. D. Ball, The Emerging Applications of Sulfur(VI) Fluorides in Catalysis, ACS Catal., 2021, 11, 6578 CrossRef CAS PubMed;
(d) Y. Meng, S. Wang, W. Fang, Z. Xie, J. Leng, H. Alsulami and H. Qin, Ethenesulfonyl Fluoride (ESF) and Its Derivatives in SuFEx Click Chemistry and More, Synthesis, 2020, 673 CAS;
(e) R. Lekkala, R. Lekkala, B. Moku, K. P. Rakesh and H. Qin, Applications of Sulfuryl Fluoride (SO2F2) in Chemical Transformations, Org. Chem. Front., 2019, 6, 3490 RSC.
-
(a) A. T. Davies, J. M. Curto, S. W. Bagley and M. C. Willis, One-Pot Palladium-Catalyzed Synthesis of Sulfonyl Fluorides from Aryl Bromides, Chem. Sci., 2017, 8, 1233 RSC;
(b) A. L. Tribby, I. Rodríguez, S. Shariffudin and N. D. Ball, Pd-Catalyzed Conversion of Aryl Iodides to Sulfonyl Fluorides Using SO2 Surrogate DABSO and Selectfluor, J. Org. Chem., 2017, 82, 2294 CrossRef CAS PubMed;
(c) P. K. T. Lo, Y. Chen and M. C. Willis, Nickel(II)-Catalyzed Synthesis of Sulfinates from Aryl and Heteroaryl Boronic Acids and the Sulfur Dioxide Surrogate DABSO, ACS Catal., 2019, 9, 10668 CrossRef CAS;
(d) T. S. Lou, S. W. Bagley and M. C. Willis, Cyclic Alkenylsulfonyl Fluorides: Palladium-Catalyzed Synthesis and Functionalization of Compact Multifunctional Reagents, Angew. Chem., Int. Ed., 2019, 58, 18859 CrossRef CAS PubMed;
(e) L. Wang and J. Cornella, A Unified Strategy for Arylsulfur(VI) Fluorides from Aryl Halides: Access to Ar-SOF3 Compounds, Angew. Chem., Int. Ed., 2020, 59, 23510 CrossRef CAS PubMed.
- For selected reports, see:
(a) T. Guo, G. Meng, X. Zhan, Q. Yang, T. Ma, L. Xu, K. B. Sharpless and J. Dong, A New Portal to SuFEx Click Chemistry: A Stable Fluorosulfuryl Imidazolium Salt Emerging as an “F−SO2+” Donor of Unprecedented Reactivity, Selectivity, and Scope, Angew. Chem., Int. Ed., 2018, 57, 2605 CrossRef CAS PubMed;
(b) H. Zhou, P. Mukherjee, R. Liu, E. Evrard, D. Wang, J. M. Humphrey, T. W. Butler, L. R. Hoth, J. B. Sperry, S. K. Sakata, C. J. Helal and C. W. am Ende, Introduction of a Crystalline, Shelfstable Reagent for the Synthesis of Sulfur(VI) fluorides, Org. Lett., 2018, 20, 812 CrossRef CAS PubMed;
(c) J. Kwon and B. M. Kim, Synthesis of Arenesulfonyl Fluorides via Sulfuryl Fluoride Incorporation from Arynes, Org. Lett., 2019, 21, 428 CrossRef CAS PubMed.
-
(a) X. Nie, T. Xu, J. Song, A. Devaraj, B. Zhang, Y. Chen and S. Liao, Radical Fluorosulfonylation: Accessing Alkenyl Sulfonyl Fluorides from Alkenes, Angew. Chem., Int. Ed., 2021, 60, 3956 CrossRef CAS PubMed;
(b) X. Nie, T. Xu, Y. Hong, H. Zhang, C. Mao and S. Liao, Introducing A New Class of Sulfonyl Fluoride Hubs via Radical Chloro-Fluorosulfonylation of Alkynes, Angew. Chem., Int. Ed., 2021, 60, 22035 CrossRef CAS PubMed.
- For our recent reports on the synthesis of various sulfonyl fluorides via the new strategy of radical sulfur dioxide insertion and fluorination, see:
(a) Y. Liu, H. Wu, Y. Guo, J.-C. Xiao, Q.-Y. Chen and C. Liu, Trifluoromethylfluorosulfonylation of Unactivated Alkenes Using Readily Available Ag(O2CCF2SO2F) and N-Fluorobenzenesulfonimide, Angew. Chem., Int. Ed., 2017, 56, 15432 CrossRef CAS PubMed;
(b) Y. Liu, Q. Lin, Z. Xiao, C. Zheng, Y. Guo, Q.-Y. Chen and C. Liu, Zinc-Mediated Intermolecular Reductive Radical Fluoroalkylsulfination of Unsaturated Carbon-Carbon Bonds with Fluoroalkyl Bromides and Sulfur Dioxide, Chem. – Eur. J., 2019, 25, 1824 CrossRef CAS PubMed;
(c) Q. Lin, Y. Liu, Z. Xiao, L. Zheng, X. Zhou, Y. Guo, Q.-Y. Chen, C. Zheng and C. Liu, Intermolecular Oxidative Radical Fluoroalkylfluorosulfonylation of Unactivated Alkenes with (Fluoroalkyl)trimethylsilane, Silver Fluoride, Sulfur Dioxide and N-fluorobenzenesulfonimide, Org. Chem. Front., 2019, 6, 447 RSC;
(d) Y. Liu, D. Yu, Y. Guo, J.-C. Xiao, Q.-Y. Chen and C. Liu, Arenesulfonyl Fluoride Synthesis via Copper-Catalyzed Fluorosulfonylation of Arenediazonium Salts, Org. Lett., 2020, 22, 2281 CrossRef CAS PubMed;
(e) Q. Lin, Z. Ma, C. Zheng, X.-J. Hu, Y. Guo, Q.-Y. Chen and C. Liu, Arenesulfonyl Fluoride Synthesis via Copper-Free Sandmeyer-Type Fluorosulfonylation of Arenediazonium Salts, Chin. J. Chem., 2020, 38, 1107 CrossRef CAS;
(f) Y. Liu, Q. Pan, X. Hu, Y. Guo, Q.-Y. Chen and C. Liu, Rapid Access to N-Protected Sulfonimidoyl Fluorides: Divergent Synthesis of Sulfonamides and Sulfonimidamides, Org. Lett., 2021, 23, 3975 CrossRef CAS PubMed;
(g) Q. Pan, Y. Liu, W. Pang, J. Wu, X. Ma, X. Hu, Y. Guo, Q.-Y. Chen and C. Liu, Copper-Catalyzed Three-Component Reaction of Arylhydrazine Hydrochloride, DABSO, and NFSI for the Synthesis of Arenesulfonyl Fluorides, Org. Biomol. Chem., 2021, 19, 8999 RSC.
-
(a) T. Zhong, M.-K. Pang, Z.-D. Chen, B. Zhang, J. Weng and G. Lu, Copper-Free Sandmeyer-Type Reaction for the Synthesis of Sulfonyl Fluorides, Org. Lett., 2020, 22, 3072 CrossRef CAS PubMed;
(b) S. Liu, Y. Huang, X.-H. Xu and F.-L. Qing, Fluorosulfonylation of Arenediazonium Tetrafluoroborates with Na2S2O5 and N-fluorobenzenesulfonimide, J. Fluorine Chem., 2020, 240, 109653 CrossRef CAS;
(c) D. Louvel, A. Chelagha, J. Rouillon, P.-A. Payard, L. Khrouz, C. Monnereau and A. Tlili, Metal-Free Visible-Light Synthesis of Arylsulfonyl Fluorides: Scope and Mechanism, Chem. – Eur. J., 2021, 27, 8704 CrossRef CAS PubMed.
-
(a) T. A. Bianchi and L. A. Cate, Phase Transfer Catalysis. Preparation of Aliphatic and Aromatic Sulfonyl Fluorides, J. Org. Chem., 1977, 42, 2031 CrossRef CAS;
(b) A. Talko and M. Barbasiewicz, Nucleophilic Fluorination with Aqueous Bifluoride Solution: Effect of the Phase-Transfer Catalyst, ACS Sustainable Chem. Eng., 2018, 6, 6693 CrossRef CAS.
- For selected examples, see:
(a) M. Kirihara, S. Naito, Y. Nishimura, Y. Ishizuka, T. Iwai, H. Takeuchi, T. Ogata, H. Hanai, Y. Kinoshita, M. Kishida, K. Yamazaki, T. Noguchi and S. Yamashoji, Oxidation of Disulfides with Electrophilic Halogenating Reagents: Concise Methods for Preparation of Thiosulfonates and Sulfonyl Halides, Tetrahedron, 2014, 70, 2464 CrossRef CAS;
(b) L. Tang, Y. Yang, L. Wen, X. Yang and Z. Wang, Catalyst-Free Radical Fluorination of Sulfonyl Hydrazides in Water, Green Chem., 2016, 18, 1224 RSC;
(c) G. Laudadio, A. A. Bartolomeu, L. M. H. M. Verwijlen, Y. Cao, K. T. de Oliveira and T. Noel, Sulfonyl Fluoride Synthesis through Electrochemical Oxidative Coupling of Thiols and Potassium Fluoride, J. Am. Chem. Soc., 2019, 141, 11832 CrossRef CAS PubMed;
(d) Y. Jiang, N. S. Alharbi, B. Sun and H.-L. Qin, Facile One-Pot Synthesis of Sulfonyl Fluorides from Sulfonates or Sulfonic Acids, RSC Adv., 2019, 9, 13863 RSC;
(e) M. Pérez-Palau and J. Cornella, Synthesis of Sulfonyl Fluorides from Sulfonamides, Eur. J. Org. Chem., 2020, 2497 CrossRef.
- For selected examples, see:
(a) J. J. Krutak, R. D. Burpitt, W. H. Moore and J. A. Hyatt, Chemistry of Ethenesulfonyl Fluoride. Fluorosulfonylethylation of Organic Compounds, J. Org. Chem., 1979, 44, 3847 CrossRef CAS;
(b) H. L. Qin, Q. Zheng, G. A. Bare, P. Wu and K. B. Sharpless, A Heck-Matsuda Process for the Synthesis of β-Arylethenesulfonyl Fluorides: Selectively Addressable Bis-electrophiles for SuFEx Click Chemistry, Angew. Chem., Int. Ed., 2016, 55, 14155 CrossRef CAS PubMed;
(c) J. Chen, B. Q. Huang, Z. Q. Wang, X. J. Zhang and M. Yan, Asymmetric Conjugate Addition of Ethylene Sulfonyl Fluorides to 3-Amido-2-oxindoles: Synthesis of Chiral Spirocyclic Oxindole Sultams, Org. Lett., 2019, 21, 9742 CrossRef CAS PubMed;
(d) B. Moku, W. Y. Fang, J. Leng, L. Li, G. F. Zha, K. P. Rakesh and H. L. Qin, Rh-Catalyzed Highly Enantioselective Synthesis of Aliphatic Sulfonyl Fluorides, iScience, 2019, 21, 695 CrossRef CAS PubMed;
(e) B. Moku, W.-Y. Fang, J. Leng, E. A. B. Kantchev and H.-L. Qin, Rh(I)-Diene-Catalyzed Addition of (Hetero)aryl Functionality to 1,3-Dienylsulfonyl Fluorides Achieving Exclusive Regioselectivity and High Enantioselectivity: Generality and Mechanism, ACS Catal., 2019, 9, 10477 CrossRef CAS;
(f) R. Xu, T. Xu, M. Yang, T. Cao and S. Liao, A Rapid Access to Aliphatic Sulfonyl Fluorides, Nat. Commun., 2019, 10, 3752 CrossRef PubMed;
(g) J. Chen, B. Q. Huang, Z. Q. Wang, X. J. Zhang and M. Yan, Asymmetric Conjugate Addition of Ethylene Sulfonyl Fluorides to 3-Amido-2-oxindoles: Synthesis of Chiral Spirocyclic Oxindole Sultams, Org. Lett., 2019, 21, 9742 CrossRef CAS PubMed;
(h) X. Zhang, W. Y. Fang, R. Lekkala, W. Tang and H. L. Qin, An Easy, General and Practical Method for the Construction of Alkyl Sulfonyl Fluorides, Adv. Synth. Catal., 2020, 362, 3358 CrossRef CAS;
(i) J. Chen, D. Y. Zhu, X. J. Zhang and M. Yan, Highly Enantioselective Addition of N-2,2,2-Trifluoroethylisatin Ketimines to Ethylene Sulfonyl Fluoride, J. Org. Chem., 2021, 86, 3041 CrossRef CAS PubMed;
(j) H. R. Chen, Z. Y. Hu, H. L. Qin and H. Tang, A Novel Three-Component Reaction for Constructing Indolizine-containing Aliphatic Sulfonyl Fluorides, Org. Chem. Front., 2021, 8, 1185 RSC.
-
(a) A. Shavnya, S. B. Coffey, K. D. Hesp, S. C. Ross and A. S. Tsai, Reaction of Alkyl Halides with Rongalite: One-Pot and Telescoped Synthesis of Aliphatic Sulfonamides,
Sulfonyl Fluorides and Unsymmetrical Sulfones, Org. Lett., 2016, 18, 5848 CrossRef CAS PubMed;
(b) A. Shavnya, K. D. Hesp and A. S. Tsai, A Versatile Reagent and Method for Direct Aliphatic Sulfonylation, Adv. Synth. Catal., 2018, 360, 1768 CrossRef CAS;
(c) C. Lee, N. D. Ball and G. M. Sammis, One-Pot Fluorosulfurylation of Grignard Reagents Using Sulfuryl Fluoride, Chem. Commun., 2019, 55, 14753 RSC.
- P. J. Sarver, N. B. Bissonnette and D. W. C. MacMillan, Decatungstate-Catalyzed C(sp3)-H Sulfinylation: Rapid Access to Diverse Organosulfur Functionality, J. Am. Chem. Soc., 2021, 143, 9737 CrossRef CAS PubMed.
- J. A. Andrews, L. R. E. Pantaine, C. F. Palmer, D. L. Poole and M. C. Willis, Sulfinates from Amines: A Radical Approach to Alkyl Sulfonyl Derivatives via Donor–Acceptor Activation of Pyridinium Salts, Org. Lett., 2021, 23, 8488 CrossRef CAS PubMed.
- For selected reviews, see:
(a) Y. Wei, P. Hu, M. Zhang and W. Su, Metal-Catalyzed Decarboxylative C-H Functionalization, Chem. Rev., 2017, 117, 8864 CrossRef CAS PubMed;
(b) N. Chen, Z. Ye and F. Zhang, Recent Progress on Electrochemical Synthesis Involving Carboxylic Acids, Org. Biomol. Chem., 2021, 19, 5501 RSC;
(c) A. Varenikov, E. Shapiro and M. Gandelman, Decarboxylative Halogenation of Organic Compounds, Chem. Rev., 2021, 121, 412 CrossRef CAS PubMed;
(d) J. Xuan, Z. G. Zhang and W. J. Xiao, Visible-Light-Induced Decarboxylative Functionalization of Carboxylic Acids and Their Derivatives, Angew. Chem., Int. Ed., 2015, 54, 15632 CrossRef CAS PubMed;
(e) M. Zhou, P. Qin, L. Jing, J. Sun and H. Du, Progress in Photoinduced Decarboxylative Radical Cross-Coupling of Alkyl Carboxylic Acids and Their Derivatives, Chin. J. Org. Chem., 2020, 40, 598 CrossRef CAS;
(f) S. Karmakar, A. Silamkoti, N. A. Meanwell, A. Mathur and A. K. Gupta, Utilization of C(sp3)-Carboxylic Acids and Their Redox-Active Esters in Decarboxylative Carbon-Carbon Bond Formation, Adv. Synth. Catal., 2021, 363, 3693 CrossRef CAS;
(g) S. K. Parida, T. Mandal, S. Das, S. K. Hota, S. De Sarkar and S. Murarka, Single Electron Transfer-Induced Redox Processes Involving N-(Acyloxy)phthalimides, ACS Catal., 2021, 11, 1640 CrossRef CAS.
-
(a) C. Zhang, Q. Chen, Y. Guo, J. Xiao and Y. Gu, Progress in Fluoroalkylation of Organic Compounds via Sulfinatodehalogenation Initiation System, Chem. Soc. Rev., 2012, 41, 4536 RSC;
(b) Y. Li, S. Chen, M. Wang and X. Jiang, Sodium Dithionite-Mediated Decarboxylative Sulfonylation: Facile Access to Tertiary Sulfones, Angew. Chem., Int. Ed., 2020, 59, 8907 CrossRef CAS PubMed;
(c) Y. Li, M. Wang and X. Jiang, Dithionite-Involved Multicomponent Coupling for Alkenyl and Alkyl Tertiary Sulfones, Org. Lett., 2021, 23, 4657 CrossRef CAS PubMed.
-
(a) G. Qiu, K. Zhou, L. Gao and J. Wu, Insertion of Sulfur Dioxide via A Radical Process: An Efficient Route to Sulfonyl Compounds, Org. Chem. Front., 2018, 5, 691 RSC;
(b) G. Qiu, K. Zhou and J. Wu, Recent Advances in the Sulfonylation of C-H Bonds with the Insertion of Sulfur Dioxide, Chem. Commun., 2018, 54, 12561 RSC;
(c) S. Ye, G. Qiu and J. Wu, Inorganic Sulfites as the Sulfur Dioxide Surrogates in Sulfonylation Reactions, Chem. Commun., 2019, 55, 1013 RSC;
(d) S. Ye, M. Yang and J. Wu, Recent Advances in the Sulfonylation Reactions Using Potassium/Sodium Metabisulfite, Chem. Commun., 2020, 56, 4145 RSC;
(e) M. Wang and X. Jiang, The Same Oxidation-State Introduction of Hypervalent Sulfur via Transition-Metal Catalysis, Chem. Rec., 2021, 21, 1 CrossRef.
Footnote |
† Electronic supplementary information (ESI) available. CCDC 2114753. For ESI and crystallographic data in CIF or other electronic format see DOI: 10.1039/d1qo01655e |
|
This journal is © the Partner Organisations 2022 |