Trace elements in peat bog porewaters: indicators of dissolution of atmospheric dusts and aerosols from anthropogenic and natural sources†
Received
5th April 2023
, Accepted 27th July 2023
First published on 2nd August 2023
Abstract
Peat bogs are often used as archives of atmospheric deposition of trace elements (TEs). However, chemical analyses of TEs in peat provide little information about their ecological significance, subsequent to atmospheric deposition. There is potential for the most reactive dusts and aerosols to dissolve in acidic (pH 4), organic-rich, bog water, thereby releasing TEs. Here, we use TEs in bog waters as indicators of the chemical reactivity of atmospheric particles. Porewaters were sampled from bogs i) near open pit bitumen mines of northern Alberta (AB) known to have elevated rates of dust deposition, primarily in the form of coarse particles, and ii) in southern Ontario (ONT), known to have had elevated rates of Pb deposition since industrialization began, supplied mainly as fine aerosols. In the AB bog waters, the elements most often elevated in abundance, relative to the reference site (Utikuma), are Li and Fe, most likely due to proton-promoted and reductive dissolution of their mineral hosts, respectively. In the bog waters collected in ONT, Pb concentrations and enrichments (relative to Y), are far greater than the AB bogs, reflecting the profound differences in cumulative inputs of industrial Pb in the two regions combined with proton-promoted dissolution of Pb-bearing aerosols. Given the affinity of natural organic matter for Pb ions, surface complexation and ligand-promoted dissolution also may have played a role in Pb release. Regardless of the dissolution mechanisms involved, peatland waters provide new insight into the chemical reactivity of dusts and aerosols. These transformations may have broader significance for the chemical composition of peatland drainage waters, and the organic-rich receiving waters of the boreal zone.
Water impact
Peat bogs are 90% water, and represent truly unique aquatic ecosystems at the interface of the atmosphere, biosphere, and hydrosphere. Known for elevated concentrations of iron and dissolved organic carbon, we show that coarse dusts from open pit bitumen mines in northern Alberta, and fine aerosols in industrialized southern Ontario, affect the abundance of trace elements in their interstitial waters.
|
Introduction
Open pit bitumen mining in northern Alberta (AB) generates considerable volumes of dust, not only from the “sand” fraction of the Athabasca bituminous sands (ABS), but also from wind-blown mineral material from dry tailings, gravel roads, and quarries.1,2 Dust deposition rates and their spatial variation have been estimated using the ash content of Sphagnum moss from peat bogs surrounding the mines, combined with the plant growth rates.3 Peat bogs are ombrotrophic which means that Sphagnum and the other plants growing at the surface of the peatland receive inputs exclusively from the atmosphere. In the study by Mullan-Boudreau et al.,3 the ash fraction of the moss was separated into two components. First, the acid-soluble ash (ASA) fraction which consists primarily of oxide, carbonate, phosphate and sulphate minerals. These minerals may occur on the surface of living plants, supplied by windborne dusts, but they may also form in the laboratory during ashing of plant matter, from the oxidation and carbonation of the macronutrients they contain e.g. K, Mg, Ca, P, S, as well as Mn and Fe.4,5 Second, the acid-insoluble ash (AIA) fraction which consists of silicates, aluminosilicates and refractory oxides4,5 which may also occur on plant surfaces and are unaffected by combustion. The AIA fraction is expected to be relatively inert, given that the ABS are dominated by coarse (90% > 100 μm) beach and dune sands consisting of very stable minerals such as quartz, clay minerals such as kaolinite and illite, and heavy minerals such as ilmenite, zircon and rutile which are extremely resistant to chemical weathering.6 One of the main findings of the moss study is that the concentrations of ASA in Sphagnum increased with proximity to industry3 suggesting that industrial emissions contribute increasing amounts of chemically reactive mineral phases with distance toward the mines.
As organic matter accumulates over time in bogs, the surface peat layers become increasingly isolated from surrounding mineral soils, sediments, surface waters and groundwaters.7 With an abundance of decomposing plant matter, the organic acids generated during decay combined with the lack of alkalinity from the surrounding watershed, bog waters become and remain acidic (pH 4). The low pH of these waters combined with the abundance of complex-forming organic ligands, creates a milieu which is ideal for the dissolution of chemically reactive mineral phases such as carbonates, phosphates, and sulphates.8,9 Chemical analyses of the interstitial waters collected at the surface of bogs in the vicinity of open pit bitumen mines, therefore, may provide an indication of the extent of dissolution of industrial dusts.
Sphagnum moss from bogs in the ABS region has also been used to study atmospheric deposition of trace elements (TEs).10,11 In this area, most of the TEs of environmental concern in Sphagnum are strongly correlated with conservative lithophile elements (e.g. Al, Sc, Ti, Y) suggesting that they are mainly associated with mineral matter.10,11 Using age-dated peat cores from bogs in the area, it was found that the enrichments of potentially toxic chalcophile elements such as Ag, Cd, Sb and Tl, as well as elements enriched in bitumen (Mo, Ni and V), have been in decline for decades.12 Regarding Pb, which has generated national concern about the environmental impacts of bitumen mining and upgrading,13 not only has atmospheric deposition been in decline for decades,14 but cumulative inputs of anthropogenic, atmospheric Pb in this region of Canada are very low, ranging from 0.04 to 0.07 g m−2.14 In contrast to northern AB, in the far more industrialized region of southern Ontario (ONT), the cumulative inputs of industrial Pb from atmospheric sources range from 1.0 to 2.4 g m−2.15 Unlike the coarse-grained dust particles generated by mechanical processes such as rock blasting and crushing, Pb-bearing aerosols from high temperature, anthropogenic activities such as smelting and coal combustion, are very small, with a median diameter on the order of 0.5 microns.16 Further, Pb concentrations may be an order of magnitude greater on the surfaces of these particles compared to their interiors,17 with some of this Pb in relatively soluble forms.18 Chemical analyses of porewaters from bogs in southern ONT, therefore, may provide an estimate of the bioaccessibility of anthropogenic Pb derived from the air.
Taken together, the abundance of TEs in the dissolved (i.e., <0.45 μm) fraction of peat bog porewaters may provide an estimate of the bioaccessibility of elements to living organisms. The low pH of bog surface waters combined with the general tendency of mineral dissolution rates to increase with decreasing pH,19–23 together make these unique ecosystems convenient platforms to investigate the chemical reactivity of dust particles and aerosols. To date, however, there has been little effort to use peat bog porewaters with this stated goal.24–28 In respect to other published data on TEs in bog waters, early work was restricted to the most abundant trace metals such as Al, Fe, Mn and perhaps Zn,29–39 with more recent work starting to include the less abundant TEs.40–45
This study has two main objectives: i) to determine whether the elevated rates of dust deposition to peat bogs near open pit bitumen mines and upgraders in northern AB has led to elevated concentrations of any TEs in the dissolved fraction of their interstitial waters, and ii) to determine whether the elevated rates of atmospheric Pb deposition to the bogs of southern ONT has led to elevated concentrations of Pb in their porewaters. To answer these questions, waters were collected and analyzed from four bogs near the bitumen mining industry, and compared with a remote site, far removed from industry. In addition, waters were sampled from three bogs in southern ONT where elevated inputs of atmospheric Pb are well documented46 and cumulative inputs of anthropogenic Pb are known.15 Our hypotheses are that lithophile element concentrations will be elevated in the bog waters near the bitumen mines, but that Pb concentrations will be far more abundant in the bog waters from southern ONT.
Materials and methods
Sampling locations
a) Northern Alberta.
Water samples were collected from four bogs in the vicinity of open pit bitumen mines and upgraders: JPH4, McKay, McMurray, and Anzac (JPH4, McK, McM, and ANZ). In respect to proximity from industry, JPH4 is 12 km from the mid-point between the two central bitumen upgraders, McK 25 km, McM 49 km and ANZ 68 km (Fig. 1). The predominant wind direction is N-S, roughly following the valley of the Athabasca River.3 For comparison and perspective, water also was collected from a bog (UTK) within the Utikuma region study area: this sampling location is 264 km from the centre of the bitumen mining and upgrading industry and serves as the reference site for AB. Brief descriptions of these sites are given elsewhere.11,12 Sampling took place in 2019, twice: first in summer, during late June and early July, when the peat was beginning to thaw, and then again during the autumn, in October. There were no visible pools of surface water, so a shallow pit was excavated within the Sphagnum moss carpet at each site, to obtain interstitial water from surface layers of the bogs i.e. the porewater contained within the poorly decomposed peats. The pits were approximately 30 cm square and 20–30 cm deep, and cut using a stainless steel knife with a serrated edge. The number of sampling sites within each bog, and the number of replicate samples, is provided in the ESI† (SI 1.1). During the summer sampling campaign, frozen peat occurred at each site, approximately 20 to 30 cm below the surface of the bogs, perching water within the poorly decomposed peat layer. All pits were emptied after excavation using a polypropylene bottle, and the pits rapidly refilled with interstitial water. Sampling took place approximately 45 minutes later, to allow most of the large particles to settle. All five AB bogs were sampled again in the autumn of 2021, but only the Pb data are presented here, to allow a comparison with bog waters from ONT that were sampled at the same time, as described below.
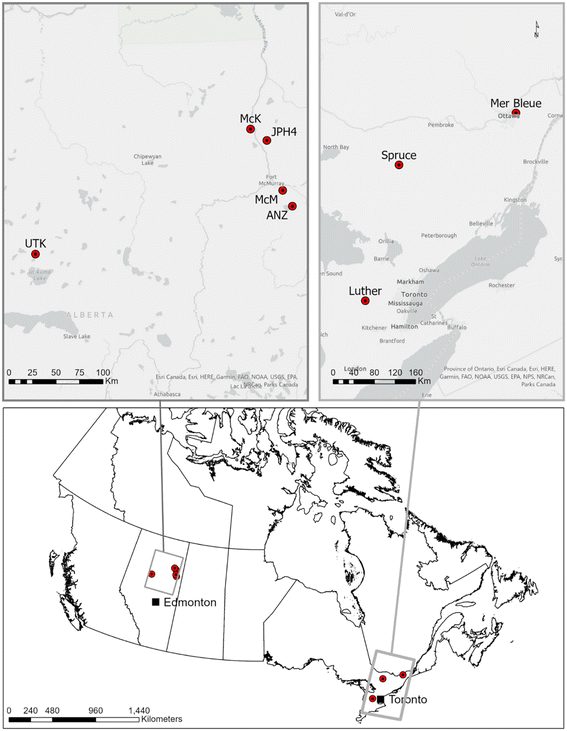 |
| Fig. 1 Map showing the locations of the peat bogs sampled in Alberta (AB), in western Canada, and in Ontario (ONT), in eastern Canada. In AB, UTK serves as the reference location. In ONT, Spruce Bog is the most remote site studied. | |
b) Southern Ontario.
In the autumn of 2021, waters were collected from three peat bogs: Mer Bleue Bog, Luther Bog which is part of the Luther Marsh Conservation Area, and Spruce Bog which is within Algonquin Provincial Park (Fig. 1). Mer Bleue Bog is adjacent to the City of Ottawa, Luther Bog is within an agricultural region, and Spruce Bog represents a remote location. More information about the Luther and Spruce bogs is provided by Givelet et al., (2003),47 and Mer Bleue by Givelet (2004).48 Using the same procedures as noted above, pits were excavated at 3 locations per bog, approximately 10 m apart, and one water sample was collected from each pit.
Sampling methods
Water samples for TE analyses were collected using the protocol described in detail elsewhere.49 Briefly, while wearing hair nets and polyethylene gloves, water was collected by submerging a polypropylene (PP) transfer bottle into the shallow, water-filled pits. For dissolved TEs and major cations, water was removed from the transfer bottles using a PP syringe, and filtered through a 0.45 μm, PTFE filter into bottles containing concentrated nitric acid to bring the pH below 2. These bottles, filters and syringes had all been pre-cleaned in nitric acid which had been purified twice, by sub-boiling distillation in a quartz still (MLS, Leutkirch, Germany) in the metal-free, ultraclean SWAMP Laboratory, using the procedures and protocols described in detail elsewhere.49 Unacidified samples were also collected for chloride analysis. A more detailed description of the field work, including the GPS coordinates of the sampling locations, is provided in ESI† 1 (SI.1. Table S1).
Sample analysis
The pH and electrical conductivity (EC) of the waters were measured in the field, using a handheld combination device (Hanna HI98129, Hanna Instruments, Woonsocket, RI, USA).
In the laboratory, chloride was measured colorimetrically (U.S. EPA method 325.2), or using ion chromatography. Trace element concentrations were determined using quadrupole ICP-MS (ICAP Qc, Thermo Scientific) using the procedures and protocols described in detail elsewhere.49 Major element cations (Na, K, Mg, Ca) were determined using ICP-OES. Information regarding blank values, limits of detection (LOD), limits of quantification (LOQ), and recoveries of the standard reference materials (SRM), are provided in SI.1† (Tables S2–S6).
Presentation of element concentration data
For the AB peatlands, an average composition was calculated for each bog, for both summer and autumn samples. For convenience, the complete set of element concentration data, for both summer and autumn, are summarized in SI.1† as follows: i) alkali metals (Li, Na, K, Rb, Cs) in SI.1† Fig. S1; ii) alkaline earth metals (Be, Mg, Ca, Sr, Ba) in SI.1† Fig. S2; iii) conservative, lithophile elements (Al, Y, La, Th) in SI.1† Fig. S3; iv) metals enriched in bitumen (V, Ni, Mo) in SI.1† Fig. S4; v) Mn and Fe which are sensitive to fluctuations in redox potential, and therefore depth to water table in bogs, in SI.1† Fig. S5; vi) other elements of the first transition series (Cr, Co, Cu, Zn) in SI.1† Fig. S6; and vii) potentially toxic “heavy metals” (Cd, Pb, Sb, Tl) in SI.1† Fig. S7.
For the peat bogs from ONT, porewater Pb concentrations only are presented, and Pb/Y ratios (Fig. 8) along with Pb concentrations in peat (Fig. 9).
Statistical analyses of the data from the Alberta bogs
The data set dimensionality was reduced by identifying the variables that had major explanatory value/correlations with other elements. After reducing the dataset to 14 variables, Principal Component Analysis (PCA) was applied to the overall sample set, using only the data for which the variables were available in both summer and fall of 2019. The complete set of results is provided in the ESI† 2 (SI.2).
Results and discussion
1. Impacts of coarse aerosols on bog waters in Alberta
The cumulative inputs of dust to these bogs, expressed as accumulation rates of ash, AIA and ASA, deposited since AD 1900, was reconstructed using age-dated peat cores,50 and these results are reproduced here (Fig. 2).
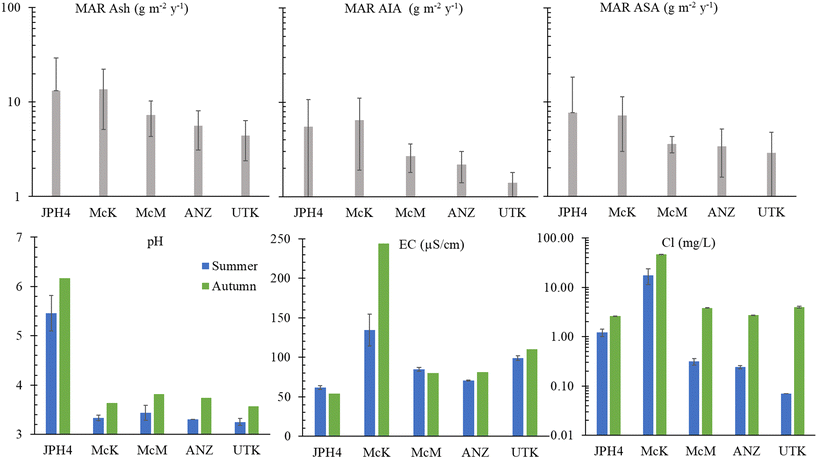 |
| Fig. 2 Upper panel: Mass accumulation rate (MAR) since AD 1900 for ash content, acid-insoluble ash (AIA), and acid-soluble ash (ASA) for JPH4, McK, McM, ANZ and UTK. The data are from Table 1 of Mullan-Boudreau et al.50 and are based on peat cores from each of these bogs that had been age-dated using a combination of 210Pb (CRS model), 241Am, 14C (including the bomb pulse curve) and tephrochronology. Lower panel: pH, EC, and chloride concentrations in peat bog porewaters. | |
pH, electrical conductivity, and chloride.
The pH of the waters are similar, and within the range typically reported for peat bogs7 with the exception of JPH4 (Fig. 2). The pH at JPH4 was significantly greater than the other sites. The elevated pH values at this site may be due to dissolution of dust particles. Alternatively, they may reflect natural differences in pH due to variations in trophic status within the surface of the mire. This peatland is very shallow (<100 cm), and the surface vegetation ranges from ombrotrophic bog plant species to weakly minerotrophic fen species. Given the proximity of JPH4 to industrial activity, this could be a useful site for monitoring atmospheric deposition of contaminants using bog vegetation. In this respect, the anomalous pH value warrants a more detailed study of the spatial variation in pH of the porewaters at the surface of this peatland, to clearly distinguish the truly ombrotrophic zones from the weakly minerotrophic areas.
The EC values at JPH4, McM and ANZ, are slightly or moderately below the value for the reference site (UTK). In contrast, the EC values for the McK bog are clearly anomalous (Fig. 2). The elevated EC values at McK are unlikely due to mineral dissolution, because the pH of the porewaters, a reflection of mineral weathering intensity,51 at McK are similar to McM, ANZ and UTK which all have similar EC values. The chloride concentrations in the autumn were extremely anomalous at McK (Fig. 2) which suggests that this site is either affected by road salt, inputs of saline groundwater, or both. Like JPH4, a more detailed study of the spatial variation in the EC of porewaters at the surface of this peatland is needed to identify ombrotrophic zones which are unaffected by the road.
Na, K, Mg and Ca.
The lowest Na and K concentrations are found in the waters from the reference site (UTK) which also resemble the average values for rainwater in AB (Fig. 3). In contrast, greater concentrations occur at the other sites. In respect to these two metals, the values for McK are clearly anomalous, but consistent with the highly elevated concentrations of chloride at this site. Comparing the Na/K ratios of bog water with the corresponding average value for precipitation in AB, elevated ratios occur at JPH4, McK and McM. While elevated ratios of Na to K at JPH4 may be due to groundwater inputs, and at McK due to road salt or saline groundwaters, at McM where the peat accumulation is substantial (>200 cm), they are most likely due to dissolution of atmospheric dust. Feldspar minerals (e.g. plagioclase and K feldspar) are the most abundant silicates and account for approximately 60% of the volume of the Earth's crust.52 Typically, the dissolution of plagioclase and K feldspars would be the obvious sources of Na and K, respectively to natural waters.19,23,53 In contrast, the ABS are highly weathered, consisting mainly of beach and dune sands,54 and are dominated by quartz: a recent study reported only 3 to 5% potassium feldspar, and no plagioclase.55 Here, however, the presence of clay minerals of the smectite group55 as well as illite55 in the ABS, and presumably the dusts derived from them, render these the most likely sources of Na and K to the bog waters.
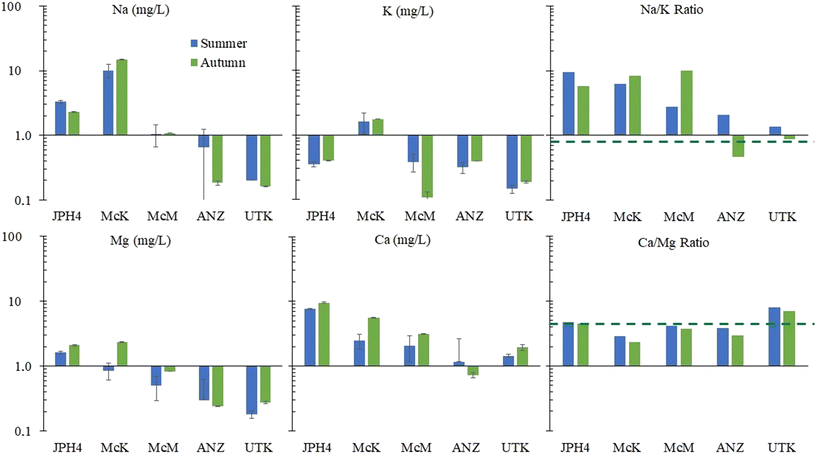 |
| Fig. 3 Upper panel: Na and K concentrations (mg L−1) and Na/K ratio in peat bog porewaters. Lower panel: Mg and Ca concentrations, and Ca/Mg ratio. The horizontal dashed line shows the average values for precipitation in Alberta which are Na/K = 0.90 and Ca/Mg = 5.1. | |
Regarding Mg and Ca, ANZ and UTK show values within a factor of two of rainwater values (Fig. 3). Much greater concentrations of Mg and Ca are found at McM, McK and JPH4, increasing in this order with increasing proximity toward industry. While greater concentrations of Mg and Ca at JPH4 could be due to the influence of groundwater, this cannot explain the elevated values at McK and McM which we know from peat core investigations are strictly ombrotrophic within the top ca. 100 cm.11,12 The Ca/Mg ratios at UTK slightly exceed the average for precipitation, with significantly lower values found in the bogs of the ABS region. The depression in Ca/Mg ratios in the porewaters of bogs sampled near industry suggests that there has been preferential dissolution of Mg-bearing minerals in the atmospheric dusts. Calcite (CaCO3) and dolomite (CaMg[CO3]2) both occur in the ABS,55 and given their chemical reactivities, these are the most likely sources of Ca and Mg in dusts from the mines, as well as the local limestone quarry.
Trace elements: general remarks.
The most abundant TEs, listed in order of decreasing concentrations, with approximate maximum values in brackets, are Fe (1 mg L−1), Al (100 μg L−1), Mn (50 μg L−1), Sr and Zn (10 μg L−1), followed by Li, Rb, V and Ni (1 μg L−1). Most of the other TEs studied are present in concentrations ranging from 100 to 1 ng L−1 or less which illustrates the need for “clean lab” methods, procedures and protocols for their successful determination.56–63 Trace element concentrations are typically an order of magnitude more abundant in the autumn compared to the summer (SI.1,† Fig. S1–S7). In summer, bog waters are more influenced by snowmelt and precipitation. This is due to the dominance of summer precipitation in continental climate regions concurrent with the presence of ice only 20 to 30 cm below the surface which prevents mixing with deeper porewaters in older peat layers. Given the very low concentrations of most TEs, and the fact that the analytical uncertainties associated with the measurements decrease with increasing concentrations, differences between sites are more easily seen using the water samples collected during the autumn. For this reason, results obtained from samples collected in the autumn are emphasized here.
Trace elements in bog waters from impacted sites versus the reference site.
a) Autumn.
To determine whether or not TE concentrations are more abundant in the bogs near industry, the average concentrations of dissolved TEs in the autumn samples at JPH4, McK, McM, and ANZ were each normalized to those of UTK (the reference site). After normalization, the ratios of the elements in bogs near industry, relative to UTK, were summarized in order of decreasing value (Fig. 4). At JPH4, the site nearest the centre of industrial activity, the elements which are more than double their concentration at the reference site are Li, all of the rare earth elements (REE), Y, Ni, Sr, Sb, Mo, Fe, As, Cr, and Be. At McK, the list of elements more than double their concentration at the reference site includes Mn, Li, Rb, Ni, Pb, Cs, Sr, Tl, the REE, V, Y, Be, Co, Fe and Re. At McM, the list of elements that are more than double their concentrations at UTK is restricted to Mn, Pb, Li, Sb, some of the REE, Ni, Fe, and Y. At ANZ, only Mn, Pb and Rb are more than double their concentrations at UTK. In summary, elements enriched in 3 out of the 4 bogs near industry are Li, Fe, Mn, Ni, Y and selected REE (Tm, Dy, Yb, Sm), plus Pb. Of these, Pb has the greatest environmental relevance regarding potential toxicity to living organisms, but the enrichments are modest i.e. McM, 7.8×; McK, 4.9×; ANZ, 3.3×. For most elements that are enriched, i.e. ≥2× the values found at the reference site (UTK), the enrichments are modest: only Li, Mn and Rb show enrichments greater than 10×.
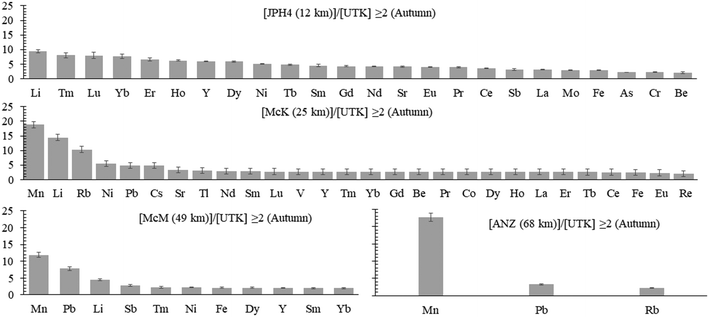 |
| Fig. 4 Bar graphs showing the ratio of concentrations of TEs in the porewaters of peat bogs near industry, to the reference site (UTK), for samples collected in autumn. From top to bottom, listed in order of increasing distance from industry: JPH4, McK, McM and ANZ. | |
b) Summer.
In the summer samples, the extent of enrichment, relative to the reference site, is lower, with none of the enrichments greater than a factor of 10 (Fig. 5). The only elements enriched in 3 out of the 4 bogs are Fe, Li, Mo and V; only Li is enriched in the waters from all 4 bogs. Lead is enriched at only one site (McK) and the enrichment is low (2.1×).
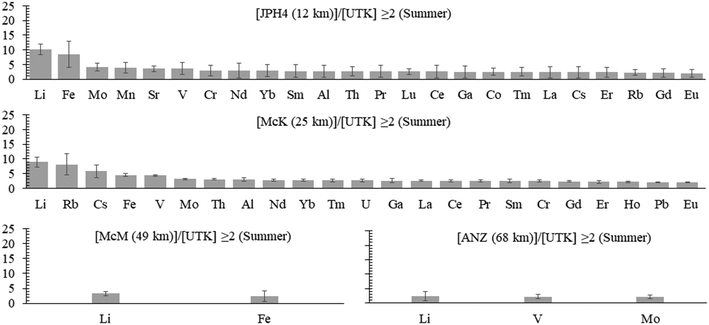 |
| Fig. 5 Bar graphs showing the ratio of concentrations of TEs in the porewaters of peat bogs near industry, to the reference site (UTK), for samples collected in summer. From top to bottom, listed in order of increasing distance from industry: JPH4, McK, McM and ANZ. | |
c) Summary of element enrichments.
When the results from bog waters collected near industry in autumn (n = 4) and summer (n = 4) are combined into a single data set, only a handful of elements are enriched in more than one-half the samples, relative to UTK: Li is elevated in 7 out of 8 cases, Fe in 6 of 8, while Sm, Tm and Yb are elevated in 5 of 8 cases. Thus, the TEs that most often indicate an environmental impact on these bog waters are Li and Fe, plus some of the lanthanides. Regarding the potentially toxic chalcophile elements which generated so much concern about the environmental impacts of bitumen mining and upgrading,64 Pb was enriched in 4 out of 8 cases, but the enrichments are modest (maximum 7.8× at McM). Enrichments of Sb were found in 2 of 8 cases and Tl in 1 of 8; the extent of Sb enrichments were 3.0× (JPH4) and 2.8× (McM), and in the case of Tl, 3.1× (McK). Thus, the enrichments of chalcophile elements are modest, and seen only in autumn when concentrations are elevated, relative to summer values. There were no instances, summer or autumn, in which Cd, Cu or Zn in waters from industrial sites exceeded the reference site by more than 2×. In fact, the greatest concentrations of Cu, both in autumn and summer samples, was found at the reference site (SI.1† Fig. S6).
Trace metal enrichments, relative to the Upper Continental Crust.
To estimate the extent of enrichment of TEs in peat bog waters, relative to their presumed natural abundance, selected elements in the water samples collected in autumn were also used to determine the crustal enrichment factor (EF).65–67 Specifically, using Y as the conservative, reference element, EF values were calculated as the element/Y ratio, normalized to the corresponding ratio in the Earth's Upper Continental Crust (UCC).68 These calculations are presented for V, Ni and Mo which are all enriched in bitumen, as well as Cd, Pb, Sb and Tl which are all potentially toxic “heavy metals” (Fig. 6). Vanadium, Ni and Mo are all enriched at the reference site by factors of 5.2, 5.0 and 42.5, respectively. There is no obvious trend with proximity to industry for V and Ni, with some industrial sites showing lower EFs than the reference site. Molybdenum is exceptional in that it becomes progressively less enriched with distance toward industry (Fig. 6).
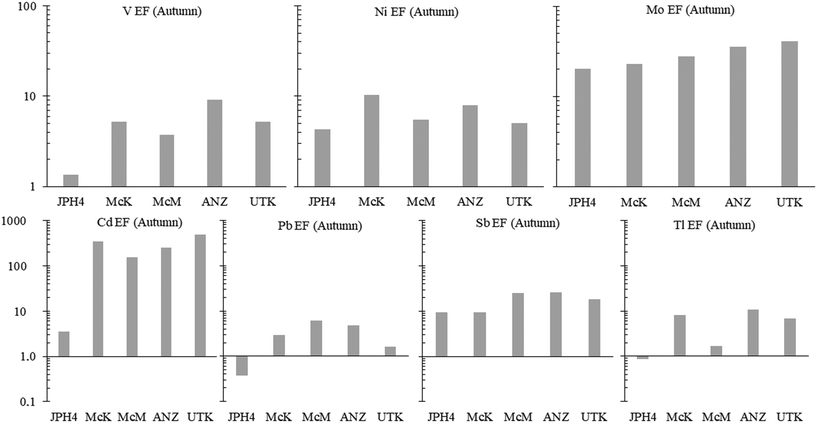 |
| Fig. 6 Enrichment factors (EF) of TEs enriched in bitumen (V, Ni, Mo) and potentially toxic chalcophile trace elements (Cd, Pb, Sb, Tl), for bog water samples collected in autumn. For details of the EF calculations, see text. | |
Except for JPH4 which shows a modest Cd enrichment (3×, relative to the UCC), all of the sites are enriched in Cd, with the greatest enrichment found at the reference site, UTK (Fig. 6). In respect to Pb, the waters at JPH4 (which is closest to industry) are actually depleted in this element whereas the other industrial sites are enriched by up to 3.9×. Regarding Sb, the two sites nearest industry show the lowest enrichments, and the other two industrial sites are enriched, but relative to the reference site, only by 1.4×. In terms of Tl, two industrial sites (JPH4 and McM) show no significant enrichments relative to the UCC; the other two industrial sites (McK and ANZ) do show enrichments. However, relative to the reference site (UTK), the greatest extent of Tl enrichment at these sites is only 1.6×. Given the inherent variability of crustal rocks and the soils derived from them, we consider EF values <2 as being insignificant.69 Thus, none of the enrichments of Sb and Tl are significant, relative to the natural enrichment of these elements in water from the reference site (UTK). In summary, regarding the potentially toxic “heavy metals”, there are no consistent trends in the concentrations of these metals with distance toward industry (SI.1† Fig. S7).
Regarding the EF values, the results presented here illustrate the risk of considering industrial sites in isolation, without also considering natural enrichments of TEs, relative to crustal abundance. The enrichments of V, Ni and Mo provide a helpful illustration of the problem: while they are all enriched in bitumen,70 they are also enriched in the porewaters of the reference site, relative to crustal abundance (Fig. 6), even though the reference site is more than 260 km, and generally upwind, from the bitumen mines and upgraders.
Dissolution of atmospheric dust particles and release of trace elements to bog waters.
The abundances of selected TEs, representing the major geochemical classes noted at the start of the manuscript, are presented in Fig. 7 for the water samples collected in autumn. Of all these elements, Y shows a trend in increasing concentrations toward industry (Fig. 7) which most closely resembles the record of atmospheric dust inputs to the bogs (Fig. 2). Considering the other lithophile elements, Li and Sr are clearly more abundant near industry; so too are Al and Cr, but the range in concentrations between industrial sites and the reference sites is less (Fig. 7). Of the elements enriched in bitumen, Ni shows the clearest differences in concentration (Fig. 7). Of the chalcophile elements, Pb shows no consistent differences, Zn shows little difference whereas Cu is most abundant at the reference site (Fig. 6).
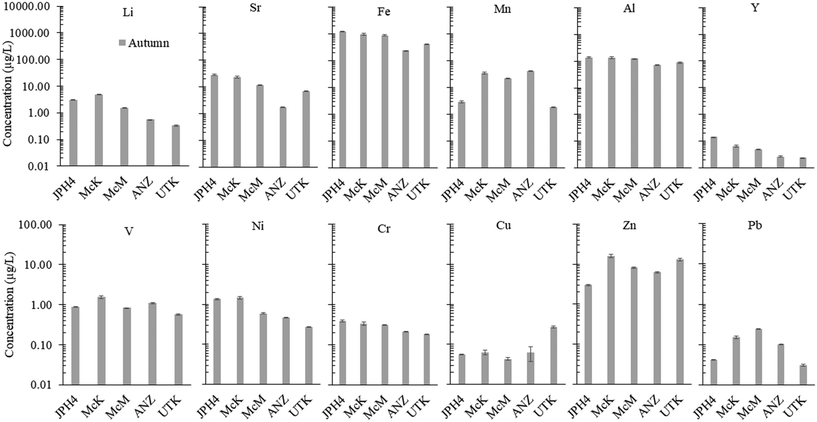 |
| Fig. 7 Concentrations of selected TEs (ng L−1) in the porewaters of peat bogs, collected in autumn. | |
Peat bog waters as monitors of the chemical reactivity of coarse aerosols.
Sodium, K, Mg and Ca are the most obvious indicators of dust dissolution (Fig. 3), and the reasons for this are quite simple. First, following Al and Fe, they are by far the most abundant metals in rocks and soils52 and the atmospheric dusts derived from them. Second, many of the mineral phases which host them are comparatively reactive (e.g. calcite) or are very small, with large surface area (e.g. illite-smectite) and should readily dissolve in peat bog waters.9
In respect to TEs, considering JPH4 which is the site closest to the centre of industry, Fe, Al, Sr, Mn are by far the most abundant elements, followed by Li, Zn, Ni, V, Cr, Cu, Pb and Y (Fig. 7). Given the importance of determining the natural abundance of these same elements in peat bog water from a reference site such as UTK, we have compared the abundance of all the TEs discussed here, with the limits of detection obtained for their determination using ICP-MS in our metal-free, ultraclean lab. This comparison, for summer and autumn samples, is provided in the SI.1† (Fig. S8). In summer when element concentrations are lower, 15 of the 42 elements determined were at least 10× above the LOQ (SI.1† Fig. S8). In the autumn, 39 of the 42 elements measured were at least 10× about the LOQ (SI.1† Fig. S8). Clearly, sampling peat bog waters in autumn when concentrations are greatest is advantageous, as most analytes of interest are at least 10× the LOQ, and the accuracy of measurements is optimal. In general, the elements which are most reliably measured and show the greatest differences among the sites, are comparatively abundant TEs such as Fe, Al, Mn, Sr and Li, along with Ni, V, Y and some of the light REE. For routine monitoring by government agencies, this small list of elements will provide most investigators and their laboratories with an opportunity to document these types of water quality impacts due to industrial development.71
Results of the statistical analyses.
The PCA data shows that PC1 explains 42% of the variation: with high factor loadings for lithophile elements such as Li and Sr, and conservative, lithophile elements such as Al, Ga, Th and the REE (SI.2† Matrix 1, Fig. S2, S3 and S5): these are the elements readily explained by dust inputs. Clearly, inputs of dust represent the dominant factor affecting peat bog water chemistry in the area. PC2, with high factor loadings for pH, Ca and Mg, explains 20% of the variation and distinguishes JPH4 from the other sites (SI.2† Matrix 1, Fig. S2, S4 and S6): PC2 is interpreted as reflecting groundwater inputs to this transitional peatland. PC3, with high factor loadings for K and Cl−, explains 12% of the variation and distinguishes McK from the other bogs (SI.2† Matrix 1, Fig. S3, S4 and S7): PC3 is interpreted as an indicator of inputs of saline water to this site. Finally, PC4 which explains 9% of the variation, has a high factor loading for Cu and distinguishes UTK from other sites (SI.2† Matrix 1, Fig. S5–S7): the bog waters from UTK contain the greatest Cu concentrations (Fig. 7), even though this is the reference site. In summary, the statistical analyses of the data are consistent with the geochemical observations described above.
2. Impacts of fine aerosols on Pb in bog waters from southern Ontario
Lead concentrations in the waters of the bogs in AB sampled in the autumn of 2021 range from 103 to 214 ng L−1 (Fig. 8). In contrast, the bog waters from southern ONT contain 1830 to 6510 ng L−1 (Fig. 8). The greatest concentration found in AB (214 ng L−1 at JPH4) is more than 8 times lower than the lowest concentration found in ONT (1830 ng L−1 in the Spruce Bog). Normalizing the Pb concentrations to those of Y show much greater differences between the two regions (Fig. 8): in the AB bogs, the ratios range from 1.0 to 5.3 (average 2.6) whereas in ONT, they range from 33 to 40 (average 36). On average, therefore, the Pb/Y ratios in the ONT bogs are 14 times the corresponding values in AB. As noted earlier, cumulative inputs of anthropogenic, atmospheric Pb in AB are very low, ranging from 0.04 to 0.07 g m−2.14 In contrast, in southern ONT, the cumulative inputs of industrial Pb from atmospheric sources range from 1.0 g m−2 at the Spruce Bog to 2.4 g m−2 at the Mer Bleue Bog.15 On average, cumulative Pb inputs in ONT (1.5 g m−2) exceed those of AB (0.05 g m−2) by 30 times.
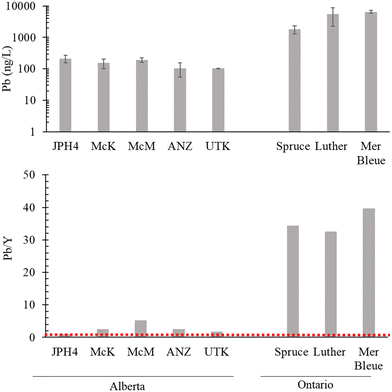 |
| Fig. 8 Lead concentrations (ng L−1) and Pb to Y ratios in the dissolved fraction (i.e. <0.45 μm) of the porewaters of the AB bogs versus ONT bogs. | |
Why are the average differences in Pb/Y ratios in the bog waters (ONT/AB = 14×) less than the differences in cumulative Pb inputs (ONT/AB = 30×)? In general, studies of age-dated peat cores from bogs have shown that Pb is very well preserved over time in ombrotrophic peatlands and effectively immobile (see Shotyk et al., 2016 (ref. 14) for a brief review). At the same time, studies of Sphagnum moss using stable and radioactive Pb isotopes have shown that retention of atmospheric Pb from natural and anthropogenic sources is incomplete, as there are losses of Pb from plant surfaces.72 It is clear from the Pb concentration data for bog waters (Fig. 8) that Pb in the ONT bogs has, to some extent, been mobilized from the solid phase to the aqueous phase. However, post-depositional Pb migration within a peatland depends not only on aqueous phase concentrations, but also the rate of fluid movement. If bog water movement within the surface layers of these peatlands is limited or slow, migration of dissolved species will be too. Thus, caution is needed when interpreting TE concentrations or enrichments in bog waters, in relation to atmospheric deposition, without the considerable benefit of relevant hydrological information. Moreover, southern ONT receives approximately twice the annual amount of precipitation of northern AB, and this would tend to dilute the former compared to the latter. Regardless of these considerations, it seems reasonable to suggest that the elevated Pb concentrations in peat bog waters from ONT, relative to the bog waters in AB, are due to the far greater cumulative inputs of anthropogenic, atmospheric Pb inputs in eastern Canada.
3. Trace elements in peat bog waters: quo vadis?
a) Natural background concentrations of trace elements in bog waters.
Here, UTK is used as the reference location for comparison with bog waters collected in the vicinity of open pit bitumen mines and upgraders. In other words, the bog waters from UTK are taken to represent the natural, “background” concentrations of TEs in bog waters from northern AB. With the exception of Ag which is at or below 1 ng L−1, the bog water samples collected in autumn from UTK were analyzed successfully for every TE of interest (SI.1† Fig. S8). This includes a number of elements that are at or below 10 ng L−1e.g. Be, Cs, Sb, and Tl. There are several lines of evidence to suggest that UTK has provided some of the first reliable data regarding baseline concentrations of TEs in peat bog waters. First, we have shown11 that the average concentrations of Cr, Ni, Pb, Sb, Tl and V in Sphagnum moss samples from UTK are similar to the values found in ancient samples of ombrotrophic peat from the Swiss bog at Etang de la Gruère (EGR). These Swiss samples, dating from the mid-Holocene climate optimum (ca. 6000 to 9000 years ago) yielded some of the lowest TE concentrations ever reported for peat samples collected in the northern hemisphere.73 In other words, the abundance of TEs in Sphagnum moss from UTK today, represents natural “background” concentrations. Second, an age dated peat core collected from UTK shows that atmospheric Pb contamination in the ABS region has been in decline for decades.14 Moreover, Pb concentrations have reached the point where, today, it is difficult to detect any Pb contamination, relative to background levels, in the topmost layers of these ecosystems.14 Third, the Pb contents of wild berries growing in the bog at UTK are not significantly different from the very low Pb concentrations obtained for berries growing in remote areas much further north e.g. Point Brûlé, Birch Mountain Wildlands, and the Caribou Mountain Wildlands.74 Fourth, Pb concentrations and Pb/La ratios in the leaves of Labrador Tea (Rhododendron groenlandicum) from UTK, another bog plant, are among the lowest compared to plants from other regions of Canada.75 Thus, there are good reasons to suggest that i) the bog waters from UTK have generated TE concentration data that predominantly reflect interactions between these organic-rich, acidic waters and the crustal aerosol,66 and ii) provide a reference level against which bog waters from more industrial regions of North America may be compared.
b) Trace elements in bog waters impacted by atmospheric dusts.
Elevated concentrations of conservative, lithophile elements such as Y and REE in bog waters near the open pit mines, are most likely related to the abundance of heavy minerals such as zircon and monazite which occur in the mineral fraction of bitumen-containing ores.76–78 Zircons are rich in Y and monazite is rich in the REE.79 While the elevated concentrations of these elements in the “dissolved” fraction of bog waters seems to imply particle dissolution, these are among the “resistate” minerals of Goldschmidt:80 because of their thermodynamic stability, they are extremely resistant to dissolution, and become residually enriched in soil profiles during chemical weathering.19,53 It seems unlikely that there is active and apparently rapid dissolution of these minerals in peat bog waters today when there is geochemical evidence to suggest that minerals are preserved in acidic peat bog waters for millenia.73,81 An alternative explanation for their enrichment in the “dissolved” (i.e. <0.45 μm) fraction of the waters, is their presence in the colloidal fraction which may result from their emission to the atmosphere as nano-sized dust particles. Size-resolved fractionation of peat bog waters, using AF4-ICP-MS,82 should be able to distinguish between colloids bearing Y and REE that are suspended in peat bog waters and represent inputs of nanodust particles,83versus ionic forms that are derived from mineral dissolution.
While the scope of the current study has been restricted to the environmental impacts of open-pit bitumen mining in northern Canada, there are many obvious opportunities to apply the approach described here to study similar impacts worldwide. The generation of dust from anthropogenic activities such as the extraction, transportation, and use of mineral material is a global phenomenon, resulting from open-pit mining of metallic ores, coal, limestone and aggregate (sand and gravel), as well as from unpaved roads and wind erosion of soils. For perspective and context, mining and mineral extraction worldwide, including overburden related to these, accounts for approximately 27 Gt of material mobilized per year, with another 50 Gt per year from aggregate production.84 Global highway construction has consumed an estimated 200 Gt of sand and gravel to date; the U.S. alone has 2.2 M km of unpaved roads; cement and concrete production is on the order of 31 Gt per year.84 Conversion of forests to cropland, and the subsequent erosion of soils by wind, are also globally significant sources of mineral dusts, with one-half of the soil loss on the continents due to agriculture.84 Peat bog porewaters, therefore, as they are used here, represent an opportunity to study the chemical reactivity of atmospheric dusts from anthropogenic sources worldwide.
c) Trace elements in bog waters impacted by fine aerosols.
The elevated Pb concentrations in the bog waters from southern ONT reflect the much greater cumulative inputs of anthropogenic, atmospheric Pb there, compared to northern AB. While this illustrates the impact of the chemical composition of fine aerosols on aquatic chemistry of these unique ecosystems, the data also raise several questions. Specifically, using peat cores from the Luther and Spruce bogs, dated using a combination of 210Pb and 14C, atmospheric Hg deposition was found to reach its zenith in the late 1950's,47 probably in response to the introduction of the Air Pollution Control Act in the USA in 1955. The Pb concentration profiles of the Spruce, Luther and Mer Bleue bogs (Fig. 9) reach their zenith at the same depths as Hg in these peat cores, and therefore have a similar chronology of deposition from the air. The peak in Pb at Mer Bleue (159 mg kg−1) is 19 cm below the surface of the bog, at Luther Bog (95 mg kg−1) 23 cm and at Spruce Bog (115 mg kg−1) 27 cm below the top of the profile. If atmospheric Pb deposition has been in decline for more than six decades, with peak Pb concentrations well below the surface, why are Pb concentrations still elevated in the porewaters collected from the surface of these bogs? Are there still significant inputs of industrial Pb in southern ONT, or is Pb deposited in the past now being mobilized in acidic bog waters? Elevated Pb concentrations have been reported in peat bog porewaters that have been acidified by smelter emissions85 but such conditions do not apply to the ONT bogs. It seems more likely that the sampling pits excavated in these bogs to collect porewaters may have obtained water from surface layers (relatively recent peat, containing low Pb concentrations) as well as subsurface peat layers (older peat, and containing much higher Pb concentrations). The bog water data presented here (Fig. 8) corresponds to water samples collected in 2021, whereas the peat cores were collected in 2000 (Luther and Spruce bogs) and 2003 (Mer Bleue Bog). Further study is needed of metal concentrations in the porewaters at these sites, with precise sampling, to measure the variations in TEs in the porewaters in relation to the age of the peats: this can be done by expressing porewaters from age-dated peat cores. The peat cores from AB and ONT have already been precisely age-dated by Davies et al.86 and Givelet et al.47 respectively. Fresh peat samples from each slice have been kept frozen in our sample archive, and plans are underway to extract their porewaters and measure TEs.
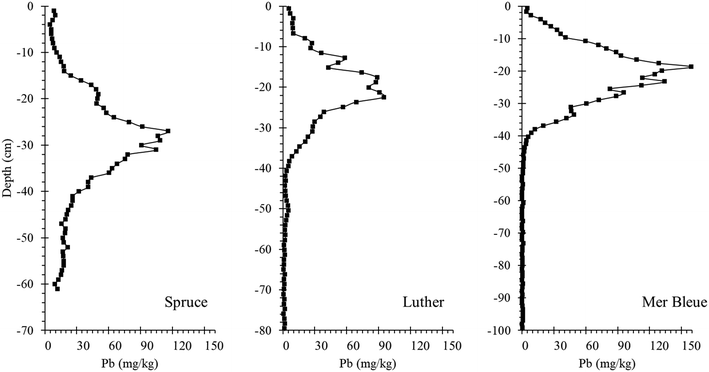 |
| Fig. 9 Lead concentration – depth profiles for peat cores collected from Spruce and Luther Bogs (2000) and Mer Bleue Bog (2003). | |
The scope of this part of the study too, is limited to a handful of bogs from Canada. However, the approach presented here should be of broad interest in Europe where the history of environmental contamination by Pb and related TEs extends back in time for several millennia, and where cumulative inputs of these potentially toxic elements are far greater.14,15
d) Impact of global climate change on peat bog water chemistry.
The bogs of northern AB are far drier than the bogs of southern ONT. While annual potential evapotranspiration (PET) is similar at these latitudes, the bogs of southern ONT receive approximately 1000 mm of precipitation annually, and typically have abundant pools (“hollows”) and often have ponds. In contrast, the bogs of northern AB receive approximately one-half as much precipitation, and, in consequence, ponded “surface water” in the usual sense, occurs much less frequently. Palaeoclimate reconstructions have already been undertaken of the AB peat bogs studied here. Magnan et al.87 provide a long-term record of climate change in the region, based on plant macrofossils of the peat cores, and showed that the bogs have been evolving toward vegetation typical of drier conditions for decades. van Bellen et al.88 used testate amoeba to document the lowering of water tables relative to the peat surface in these bogs during the past few decades. While the AB bogs have always been much drier than the ONT bogs because of the approximately two-fold difference in annual precipitation, in recent decades the AB bogs have become even drier. Drying of the bogs and lowering of the water table will have broad ramifications for the chemistry of peat porewaters. For example, pores will have greater access to air, allowing the introduction of oxygen to surface peat layers, promoting the oxidation of organic matter, and increasing the concentrations of dissolved organic matter (DOM) in the porewaters. Increasing the concentration of DOM will lower the pH of the waters,89 and this, in turn, will promote the dissolution of mineral matter. Enhanced dissolution of mineral matter will increase the concentrations of TEs, while organic matter oxidation will transform the DOM: more aromatic structures will be generated and carboxyl groups created90 which, in turn, will enhance the metal-complexation capacity of DOM and the stability of organic complexes with transition metal ions. The significance of the sum of these processes for the bioaccessibility and bioavailability of TEs in bog waters remains to be seen, provided that there is accessible porewater to investigate as the bogs continue to dry.
Author contributions
William Shotyk (designed the study, wrote the grant application, wrote the paper). Fiorella Barraza (2021 ICP-MS data and summary of QA/QC, discussions, assistance with literature, editing). Sundas Butt (graphs, journal literature). Na Chen (field work, lab work, format of manuscript, including references, for final publication). Chad W. Cuss (statistical analyses of the data). Kevin Devito (climate/weather, hydrology, editing). Lukas Frost (field work, lab work, report of field work). Iain Grant-Weaver (autumn 2019 ICP-MS data, and summary of QA/QC). Muhammad Babar Javed (summer 2019 field work, ICP-MS data, and summary of QA/QC). Tommy Noernberg (summer 2019 field work). Andrii Oleksandrenko (field work, Ontario samples and related peat core data).
Conflicts of interest
The authors declare no competing financial interest.
Acknowledgements
This work was supported jointly by Canada's Oil Sands Innovation Alliance (COSIA) and the Natural Sciences and Engineering Research Council (NSERC) in the form of a CRD grant, and by an NSERC Discovery Grant. Special thanks to John Brogly of COSIA. Tracy Gartner provided administrative assistance and Rick Pelletier created the location map. We are grateful to Emmily Macdonald and Kimberly Tan for their help in the laboratory. Field work in Alberta was assisted by E. M. and K. T. in the summer as well as Taylor Bujaczek, Jacqueline Dennett, and Ron Goyhman in the autumn. Thanks also to R. G. for compiling the precipitation data for the Province of Alberta, Carolynn Forsyth and ArtisInn for accommodation at URSA, and T. B. for help in preparing our formal Field Activity Plans (FAPs). We are grateful to the Friends of Algonquin Park for permission to sample in Spruce Bog, the Grand River Conservation Authority for sampling in the Luther Bog, and the National Capital Commission for sampling at Mer Bleue Bog (with special thanks to Prof. Nigel Roulet of McGill University for access to his research area). Finally, we thank the two anonymous reviewers whose comments helped us to improve this manuscript.
References
- X. Wang, J. C. Chow, S. D. Kohl, L. N. R. Yatavelli, K. E. Percy, A. H. Legge and J. G. Watson, Wind erosion potential for fugitive dust sources in the Athabasca Oil Sands Region, Aeolian Res., 2015, 18, 121–134 CrossRef.
-
J. G. Watson, J. C. Chow, X. Wang, S. D. Kohl and L. N. R. Yatavelli, Windblown fugitive dust characterization in the Athabasca oil sands region, Desert Research Institute, Nevada System of Higher Education, Reno, Neveda, 2014, https://www.researchgate.net/profile/John_Watson13/publication/310327278_Windblown_fugitive_dust_in_the_Athabasca_Oil_Sands_Region/links/582b886c08ae004f74afb968/Windblown-fugitive-dust-in-the-Athabasca-Oil-Sands-Region.pdf Search PubMed.
- G. Mullan-Boudreau, R. Belland, K. Devito, T. Noernberg, R. Pelletier and W. Shotyk, Sphagnum moss as an indicator of contemporary rates of atmospheric dust deposition in the Athabasca Bituminous Sands Region, Environ. Sci. Technol., 2017, 51, 7422–7431 CrossRef CAS PubMed.
- P. Steinmann and W. Shotyk, Geochemistry, mineralogy, and geochemical mass balance on major elements in two peat bog profiles (Jura Mountains, Switzerland), Chem. Geol., 1997, 138, 25–53 CrossRef CAS.
- A. Sapkota, A. K. Cheburkin, G. Bonani and W. Shotyk, Six millennia of atmospheric dust deposition in southern South America (Isla Navarino, Chile), Holocene, 2007, 17, 561–572 CrossRef.
- W. Shotyk, B. Bicalho, C. W. Cuss, M. Donner, I. Grant-Weaver, M. B. Javed and T. Noernberg, Trace elements in the Athabasca Bituminous Sands: A geochemical explanation for the paucity of environmental contamination by chalcophile elements, Chem. Geol., 2021, 581, 120392 CrossRef CAS.
- W. Shotyk, Review of the inorganic geochemistry of peats and peatland waters, Earth-Sci. Rev., 1988, 25, 95–176 CrossRef CAS.
-
W. Chesworth, A. M. Cortizas and E. García-Rodeja, in Developments in Earth Surface Processes: Peatlands, ed. I. P. Martini, A. Martinez-Cortizas and W. Chesworth, Elsevier, 2006, pp. 175–195 Search PubMed.
- G. Le Roux, E. Laverret and W. Shotyk, Fate of calcite, apatite and feldspars in an ombrotrophic peat bog, Black Forest, Germany, J. Geol. Soc., 2006, 163, 641–646 CrossRef CAS.
- W. Shotyk, R. Belland, J. Duke, H. Kempter, M. Krachler, T. Noernberg, R. Pelletier, M. A. Vile, K. Wieder, C. Zaccone and S. Zhang, Sphagnum mosses from 21 ombrotrophic bogs in the Athabasca Bituminous Sands Region show no significant atmospheric contamination of "heavy metals", Environ. Sci. Technol., 2014, 48, 12603–12611 CrossRef CAS PubMed.
- W. Shotyk, B. Bicalho, C. W. Cuss, J. Duke, T. Noernberg, R. Pelletier, E. Steinnes and C. Zaccone, Dust is the dominant source of "heavy metals" to peat moss (Sphagnum fuscum) in the bogs of the Athabasca Bituminous Sands region of northern Alberta, Environ. Int., 2016, 92-93, 494–506 CrossRef CAS PubMed.
- W. Shotyk, P. G. Appleby, B. Bicalho, L. J. Davies, D. G. Froese, I. Grant-Weaver, G. Magnan, G. Mullan-Boudreau, T. Noernberg, R. Pelletier, B. Shannon, S. van Bellen and C. Zaccone, Peat bogs document decades of declining atmospheric contamination by trace metals in the Athabasca Bituminous Sands Region, Environ. Sci. Technol., 2017, 51, 6237–6249 CrossRef CAS PubMed.
- The Globe and Mail, Take Athabasca science seriously, https://www.theglobeandmail.com/opinion/editorials/take-athabasca-science-seriously/article4325489/.
- W. Shotyk, P. G. Appleby, B. Bicalho, L. J. Davies, D. G. Froese, I. Grant-Weaver, M. Krachler, G. Magnan, G. Mullan-Boudreau, T. Noernberg, R. Pelletier, B. Shannon, S. van Bellen and C. Zaccone, Peat bogs in northern Alberta,
Canada reveal decades of declining atmospheric Pb contamination, Geophys. Res. Lett., 2016, 43, 9964–9974 CrossRef CAS.
- W. Shotyk, P. Blaser, A. Grünig and A. K. Cheburkin, A new approach for quantifying cumulative, anthropogenic, atmospheric lead deposition using peat cores from bogs: Pb in eight Swiss peat bog profiles, Sci. Total Environ., 2000, 249, 281–295 CrossRef CAS PubMed.
-
M. L. Corrin and D. F. Natusch, in Lead in the Environment, ed. W. R. Boggess and B. G. Wixson, Castle House Publications, 1979 Search PubMed.
- R. W. Linton, A. Loh, D. F. Natusch, C. A. Evans and P. Williams, Surface predominance of trace elements in airborne particles, Science, 1976, 191, 852–854 CrossRef CAS PubMed.
- D. F. Natusch, Potentially carcinogenic species emitted to the atmosphere by fossil-fueled power plants, Environ. Health Perspect., 1978, 22, 79–90 CrossRef CAS PubMed.
-
M. L. Jackson and G. D. Sherman, in Advances in Agronomy, ed. A. G. Norman, Academic Press, New York, 1953, pp. 219–318 Search PubMed.
-
F. C. Loughnan, Chemical Weathering of the Silicate Minerals, American Elsevier Pub. Co, New York, 1969 Search PubMed.
-
Rates of Chemical Weathering of Rocks and Minerals, ed. S. M. Colman and D. P. Dethier, Academic Press, Orlando, 1986 Search PubMed.
-
W. Chesworth, in Weathering, Soils & Paleosols, ed. I. P. Martini and W. Chesworth, Elsevier, Amsterdam, New York, 1992, pp. 19–40 Search PubMed.
-
H. U. Sverdrup, The Kinetics of Base Cation Release due to Chemical Weathering, Lund University Press, Lund, Sweden, 1990 Search PubMed.
- Y.-M. Cao, X. Chen, Z.-J. Bu and L.-H. Zeng, Spatial variations in the surface water chemistry of subtropical peatlands (central China) linked to anthropogenic pressures, Water, 2017, 9, 505 CrossRef.
- C. Cillero, R. A. Díaz-Varela, M. Rubinos and P. Ramil-Rego, Assessment of anthropogenic pressures on South European Atlantic bogs (NW Spain) based on hydrochemical data, Hydrobiologia, 2016, 774, 137–154 CrossRef CAS.
- B. Smieja-Król and A. Bauerek, Controls on trace-element concentrations in the pore waters of two Sphagnum-dominated mires in southern Poland that are heavily polluted by atmospheric deposition, J. Geochem. Explor., 2015, 151, 57–65 CrossRef.
- G. Xu, Z. Wang, H. Zhao, Z. Bu, M. Peros, S. Liu, H. Li and S. Wang, Characteristics and assessment of trace elements (Hg, As, Sb, Se, and Bi) in mire surface water from the Changbai Mountains, Northeastern China, Water, Air, Soil Pollut., 2021, 232, 1–18 CrossRef.
- L. Bragazza, Heavy metals in bog waters: an alternative way to assess atmospheric precipitation quality?, Glob. Planet. Change, 2006, 53, 290–298 CrossRef.
- E. H. Helmer, N. R. Urban and S. J. Eisenreich, Aluminum geochemistry in peatland waters, Biogeochemistry, 1990, 9, 247–276 CrossRef CAS.
- N. J. Gotelli, P. J. Mouser, S. P. Hudman, S. E. Morales, D. S. Ross and A. M. Ellison, Geographic variation in nutrient availability, stoichiometry, and metal concentrations of plants and pore-water in ombrotrophic bogs in New England, USA, Wetlands, 2008, 28, 827–840 CrossRef.
- Y. A. Kharanzhevskaya, E. S. Voistinova and E. S. Ivanova, Chemical composition and quality of bog waters in the Chaya River basin, Contemp. Probl. Ecol., 2011, 4, 100–105 CrossRef.
- M. Novak and P. Pacherova, Mobility of trace metals in pore waters of two central European peat bogs, Sci. Total Environ., 2008, 394, 331–337 CrossRef CAS PubMed.
- P. J. Blancher and D. K. McNicol, Peatland water chemistry in Central Ontario in relation to acid deposition, Water, Air, Soil Pollut., 1987, 35, 217–232 CrossRef CAS.
- N. R. Urban, S. J. Eisenreich and E. Gorham, Aluminum, iron, zinc, and lead in bog waters of northeastern North America, Can. J. Fish. Aquat. Sci., 1987, 44, 1165–1172 CrossRef CAS.
- M. C. F. Proctor, Seasonal and shorter-term changes in surface-water chemistry on four English ombrogenous bogs, J. Ecol., 1994, 82, 597 CrossRef CAS.
- D. H. Vitt, S. E. Bayley and T.-L. Jin, Seasonal variation in water chemistry over a bog-rich fen gradient in Continental Western Canada, Can. J. Fish. Aquat. Sci., 1995, 52, 587–606 CrossRef CAS.
- L. Bendell-Young, Peatland interstitial water chemistry in relation to that of surface pools along a peatland mineral gradient, Water, Air, Soil Pollut., 2003, 143, 363–375 CrossRef CAS.
- S. F. Mullen, J. A. Janssens and E. Gorham, Acidity of and the concentrations of major and minor metals in the surface waters of bryophyte assemblages from 20 North American bogs and fens, Can. J. Bot., 2000, 78, 718–727 CrossRef CAS.
- F. L. L. Muller and S. P. C. Tankéré-Muller, Seasonal variations in surface water chemistry at disturbed and pristine peatland sites in the Flow Country of northern Scotland, Sci. Total Environ., 2012, 435-436, 351–362 CrossRef CAS PubMed.
- J. Besold, A. Eberle, V. Noël, K. Kujala, N. Kumar, A. C. Scheinost, J. L. Pacheco, S. Fendorf and B. Planer-Friedrich, Antimonite binding to natural organic matter: Spectroscopic evidence from a mine water impacted peatland, Environ. Sci. Technol., 2019, 53, 10792–10802 CrossRef CAS PubMed.
- E. Bozau, S. Lojen and N. Zupančič, The peat bog at Zinnwald-Georgenfeld revisited after 25 years: Geochemical investigation of water, Sphagnum moss and peat cores, Geochemistry, 2022, 82, 125823 CrossRef CAS.
- S. Loiko, T. Raudina, A. Lim, D. Kuzmina, S. Kulizhskiy and O. Pokrovsky, Microtopography controls of carbon and related elements distribution in the west Siberian frozen bogs, Geosciences, 2019, 9, 291 CrossRef CAS.
- S. V. Loiko, O. S. Pokrovsky, T. V. Raudina, A. Lim, L. G. Kolesnichenko, L. S. Shirokova, S. N. Vorobyev and S. N. Kirpotin, Abrupt permafrost collapse enhances organic carbon, CO2 nutrient and metal release into surface waters, Chem. Geol., 2017, 471, 153–165 CrossRef CAS.
- T. V. Raudina, S. V. Loiko, A. Lim, R. M. Manasypov, L. S. Shirokova, G. I. Istigechev, D. M. Kuzmina, S. P. Kulizhsky, S. N. Vorobyev and O. S. Pokrovsky, Permafrost thaw and climate warming may decrease the CO2, carbon, and metal concentration in peat soil waters of the Western Siberia Lowland, Sci. Total Environ., 2018, 634, 1004–1023 CrossRef CAS PubMed.
- T. V. Raudina, S. V. Loiko, A. G. Lim, I. V. Krickov, L. S. Shirokova, G. I. Istigechev, D. M. Kuzmina, S. P. Kulizhsky, S. N. Vorobyev and O. S. Pokrovsky, Dissolved organic carbon and major and trace elements in peat porewater of sporadic, discontinuous, and continuous permafrost zones of western Siberia, Biogeosciences, 2017, 14, 3561–3584 CrossRef CAS.
- W. Shotyk and M. Krachler, The isotopic evolution of atmospheric Pb in central Ontario since AD 1800, and its impacts on the soils, waters, and sediments of a forested watershed, Kawagama Lake, Geochim. Cosmochim. Acta, 2010, 74, 1963–1981 CrossRef CAS.
- N. Givelet, F. Roos-Barraclough and W. Shotyk, Predominant anthropogenic sources and rates of atmospheric mercury accumulation in southern Ontario recorded by peat cores from three bogs: Comparison with natural "background" values (past 8000 years), J. Environ. Monit., 2003, 5, 935–949 RSC.
-
N. Givelet, PhD Thesis, University of Bern, 2004 Search PubMed.
- W. Shotyk, B. Bicalho, C. W. Cuss, M. W. Donner, I. Grant-Weaver, S. Haas-Neill, M. B. Javed, M. Krachler, T. Noernberg, R. Pelletier and C. Zaccone, Trace metals in the dissolved fraction (<0.45μm) of the lower Athabasca River: Analytical challenges and environmental implications, Sci. Total Environ., 2017, 580, 660–669 CrossRef CAS PubMed.
- G. Mullan-Boudreau, L. J. Davies, K. Devito, D. G. Froese, T. Noernberg, R. Pelletier and W. Shotyk, Reconstructing past rates of atmospheric dust deposition in the Athabasca Bituminous Sands Region using peat cores from bogs, Land Degrad. Dev., 2017, 28, 2468–2481 CrossRef.
-
R. Garrels, in Researches in Geochemistry, ed. P. H. Abelson, Wiley, New York, 1959, pp. 405–420 Search PubMed.
-
T. R. Paton, The Formation of Soil Material, G. Allen & Unwin, London, 1978 Search PubMed.
- S. S. Goldich, A study in rock-weathering, J. Geol., 1938, 46, 17–58 CrossRef CAS.
- J. B. F. Champlin and H. N. Dunning, A geochemical investigation of the Athabasca bituminous sands, Econ. Geol., 1960, 55, 797–804 CrossRef.
- M. Osacky, M. Geramian, M. D. Dyar, E. C. Sklute, M. Valter, D. G. Ivey, Q. Liu and T. H. Etsell, Characterisation of petrologic end members of oil sands from the Athabasca region, Alberta, Canada, Can. J. Chem. Eng., 2013, 91, 1402–1415 CrossRef CAS.
- K. W. Bruland, R. P. Franks, G. A. Knauer and J. H. Martin, Sampling and analytical methods for the determination of copper, cadmium, zinc, and nickel at the nanogram per liter level in sea water, Anal. Chim. Acta, 1979, 105, 233–245 CrossRef CAS.
- J. M. Tramontano, J. R. Scudlark and T. M. Church, A method for the collection, handling, and analysis of trace metals in precipitation, Environ. Sci. Technol., 1987, 21, 749–753 CrossRef CAS PubMed.
- C. F. Boutron, A clean laboratory for ultralow concentration heavy metal analysis, Fresenius' J. Anal. Chem., 1990, 337, 482–491 CrossRef CAS.
- J. O. Nriagu, G. Lawson, H. K. Wong and J. M. Azcue, A protocol for minimizing contamination in the analysis of trace metals in Great Lakes waters, J. Great Lakes Res., 1993, 19, 175–182 CrossRef CAS.
- G. Benoit, Clean technique measurement of Pb, Ag, and Cd in freshwater: a redefinition of metal pollution, Environ. Sci. Technol., 1994, 28, 1987–1991 CrossRef CAS PubMed.
- C. L. Creasey and A. R. Flegal, Elemental analyses of goundwater: demonstrated advantage of low-flow sampling and trace-metal clean techniques over standard techniques, Hydrogeol. J., 1999, 7, 161–167 CrossRef.
- M. P. Field and R. M. Sherrell, Direct determination of ultra-trace levels of metals in fresh water using desolvating micronebulization and HR-ICP-MS: application to Lake Superior waters, J. Anal. At. Spectrom., 2003, 18, 254–259 RSC.
- W. Shotyk and M. Krachler, Determination of trace element concentrations in natural freshwaters: how low is “low”, and how low do we need to go?, J. Environ. Monit., 2009, 11, 1747–1753 RSC.
- E. N. Kelly, D. W. Schindler, P. V. Hodson, J. W. Short, R. Radmanovich and C. C. Nielsen, Oil sands development contributes elements toxic at low concentrations to the Athabasca River and its tributaries, Proc. Natl. Acad. Sci. U. S. A., 2010, 107, 16178–16183 CrossRef CAS PubMed.
- D. H. Peirson, P. A. Cawse and R. S. Cambray, Chemical uniformity of airborne particulate material, and a maritime effect, Nature, 1974, 251, 675–679 CrossRef CAS.
-
K. Rahn, The Chemical Composition of the Atmospheric Aerosols, Graduate School of Oceanography, PhD Thesis, University of Rhode Island, Kingston, Rhode Island, USA, 1976 Search PubMed.
-
H. J. M. Bowen, Environmental Chemistry of the Elements, Academic Press, London, 1979 Search PubMed.
-
R. L. Rudnick and S. Gao, in Treatise on Geochemistry, ed. H. D. Holland, Elsevier, Amsterdam, 2014, pp. 1–51 Search PubMed.
- W. Shotyk, D. Weiss, P. G. Appleby, A. K. Cheburkin, M. Gloor, J. D. Kramers, S. Reese and W. O. van der Knaap, History of atmospheric lead deposition since 12,370 14C yr BP from a peat bog, Jura Mountains, Switzerland, Science, 1998, 281, 1635–1640 CrossRef CAS PubMed.
- B. Bicalho, I. Grant-Weaver, C. Sinn, M. W. Donner, S. Woodland, G. Pearson, S. Larter, J. Duke and W. Shotyk, Determination of ultratrace (<0.1 mg/kg) elements in Athabasca Bituminous Sands mineral and bitumen fractions using inductively coupled plasma sector field mass spectrometry (ICP-SFMS), Fuel, 2017, 206, 248–257 CrossRef CAS.
- W. Shotyk, Environmental significance of trace elements in the Athabasca Bituminous Sands: Facts and misconceptions, Environ. Sci.: Processes Impacts, 2022, 24, 1279–1302 RSC.
- W. Shotyk, H. Kempter, M. Krachler and C. Zaccone, Stable (206Pb, 207Pb, 208Pb) and radioactive (210Pb) lead isotopes in 1 year of growth of Sphagnum moss from four ombrotrophic bogs in southern Germany: Geochemical significance and environmental implications, Geochim. Cosmochim. Acta, 2015, 163, 101–125 CrossRef CAS.
- W. Shotyk, M. Krachler, A. Martinez-Cortizas, A. K. Cheburkin and H. Emons, A peat bog record of natural, pre-anthropogenic enrichments of trace elements in atmospheric aerosols since 12 370 14C yr BP, and their variation with Holocene climate change, Earth Planet. Sci. Lett., 2002, 199, 21–37 CrossRef CAS.
- W. Shotyk, Trace elements in wild berries from reclaimed lands: Biomonitors of contamination by atmospheric dust, Ecol. Indic., 2020, 110, 105960 CrossRef CAS.
- W. Shotyk, M. B. Javed and T. Noernberg, Trace elements in labrador tea (Rhododendron groenlandicum): How predominant sources to the plants impact the chemical composition of hot water extracts, Environ. Res., 2020, 183, 109272 CrossRef CAS PubMed.
-
G. Mellon, in Geoglogy of the McMurray Formation, Report No. 72, Edmonton, Alberta, 1956 Search PubMed.
- H. A. Kaminsky, T. H. Etsell, D. G. Ivey and O. Omotoso, Characterization of heavy minerals in the Athabasca oil sands, Miner. Eng., 2008, 21, 264–271 CrossRef CAS.
- E. Roth, T. Bank, B. Howard and E. Granite, Rare earth elements in Alberta Oil Sand process streams, Energy Fuels, 2017, 31, 4714–4720 CrossRef CAS.
- A. K. Cheburkin, R. Frei and W. Shotyk, An energy-dispersive miniprobe multielement analyzer (EMMA) for direct analysis of trace elements and chemical age dating of single mineral grains, Chem. Geol., 1997, 135, 75–87 CrossRef CAS.
- V. M. Goldschmidt, The principles of distribution of chemical elements in minerals and rocks, J. Chem. Soc., 1937, 655–673 RSC.
- W. Shotyk, D. Weiss, J. D. Kramers, R. Frei, A. K. Cheburkin, M. Gloor and S. Reese, Geochemistry of the peat bog at Etang de la Gruère, Jura Mountains, Switzerland, and its record of atmospheric Pb and lithogenic trace metals (Sc, Ti, Y, Zr, and REE) since 12,370 14C yr BP, Geochim. Cosmochim. Acta, 2001, 65, 2337–2360 CrossRef CAS.
- C. W. Cuss, I. Grant-Weaver and W. Shotyk, AF4-ICPMS with the 300 Da membrane to resolve metal-bearing “colloids” < 1 kDa: Optimization, fractogram deconvolution, and advanced quality control, Anal. Chem., 2017, 89, 8027–8035 CrossRef CAS PubMed.
- Y. Yang, M. Vance, F. Tou, A. Tiwari, M. Liu and M. F. Hochella, Nanoparticles in road dust from impervious urban surfaces: distribution, identification, and environmental implications, Environ. Sci.: Nano, 2016, 3, 534–544 RSC.
- J. Syvitski, C. N. Waters, J. Day, J. D. Milliman, C. Summerhayes, W. Steffen, J. Zalasiewicz, A. Cearreta, A. Gałuszka, I. Hajdas, M. J. Head, R. Leinfelder, J. R. McNeill, C. Poirier, N. L. Rose, W. Shotyk, M. Wagreich and M. Williams, Extraordinary human energy consumption and resultant geological impacts beginning around 1950 CE initiated the proposed Anthropocene Epoch, Commun. Earth Environ., 2020, 1, 1–13 CrossRef.
- W. Shotyk, N. Rausch, T. M. Nieminen, L. Ukonmaanaho and M. Krachler, Isotopic composition of Pb in peat and porewaters from three contrasting ombrotrophic bogs in Finland: Evidence of chemical diagenesis in response to acidification, Environ. Sci. Technol., 2016, 50, 9943–9951 CrossRef CAS PubMed.
- L. J. Davies, P. G. Appleby, B. J. Jensen, G. Magnan, G. Mullan-Boudreau, T. Noernberg, B. Shannon, W. Shotyk, S. van Bellen, C. Zaccone and D. G. Froese, High-resolution age modelling of peat bogs from northern Alberta, Canada, using pre- and post-bomb 14C, 210Pb and historical cryptotephra, Quat. Geochronol., 2018, 47, 138–162 CrossRef.
- G. Magnan, S. van Bellen, L. Davies, D. Froese, M. Garneau, G. Mullan-Boudreau, C. Zaccone and W. Shotyk, Impact of the Little Ice Age cooling and 20th century climate change on peatland vegetation dynamics in central and northern Alberta using a multi-proxy approach and high-resolution peat chronologies, Quat. Sci. Rev., 2018, 185, 230–243 CrossRef.
- S. van Bellen, G. Magnan, L. Davies, D. Froese, G. Mullan-Boudreau, C. Zaccone, M. Garneau and W. Shotyk, Testate amoeba records indicate regional 20th-century lowering of water tables in ombrotrophic peatlands in central-northern Alberta, Canada, Global Change Biol., 2018, 24, 2758–2774 CrossRef PubMed.
- T. G. Thompson, J. R. Lorah and G. B. Rigg, The acidity of the waters of some Puget Sound bogs, J. Am. Chem. Soc., 1927, 49, 2981–2988 CrossRef CAS.
-
S. A. Waksman, Humus: Origin, Chemical Composition, and Importance in Nature, Williams & Wilkins, Baltimore, 1936 Search PubMed.
Footnotes |
† Electronic supplementary information (ESI) available. See DOI: https://doi.org/10.1039/d3ew00241a |
‡ University of Alberta, 348B South Academic Building, Edmonton, Alberta, Canada T6G 2H1 E-mail: shotyk@ualberta.ca Fax: +1 780 492 4323 Tel.: +1 780 492 7155. |
§ Current affiliation: H2nanO, 4633 92 Ave NW, Edmonton, AB T6B 2J4, Canada. |
¶ Current affiliation: School of Science and the Environment, Memorial University of Newfoundland, Grenfell Campus, Corner Brook, NL, Canada. |
|| Current affiliation: 360 Energy Liability Management, 202 6 Ave SW Suite 1600, Calgary, AB T2P 2R9, Canada. |
** Current affiliation: Modern West Advisory, Suite 506, 10104 103 Ave NW, Edmonton, AB, T5J 3G1, Canada. |
†† Current affiliation: Hatfield Consultants, Suite A, 300 MacKenzie Boulevard, Fort McMurray, AB T9H 4C4, Canada. |
|
This journal is © The Royal Society of Chemistry 2023 |
Click here to see how this site uses Cookies. View our privacy policy here.