DOI:
10.1039/C5RA04136H
(Communication)
RSC Adv., 2015,
5, 27853-27856
A silver-initiated free-radical intermolecular hydrophosphinylation of unactivated alkenes†
Received
11th March 2015
, Accepted 13th March 2015
First published on 13th March 2015
Abstract
A scalable, operationally easy intermolecular hydrophosphinylation of various unactivated alkenes with H–P(O) compounds via an Ag(I)-initiated free radical process was developed. Mechanistic studies including electron-spin-resonance (ESR) and radical clock experiments suggest that atom transfer processes were involved in this system.
As a large class of important and valuable building blocks, organophosphorus compounds are widely applied in the synthesis of pharmaceuticals, agrochemicals and materials.1 In the past few decades, considerable advances have been made to construct C–P bonds.2 Among them, one of the most atom-economical and attractive strategies is the direct hydrophosphinylation of alkenes.3 The free-radical strategies for the addition of a P–H or (O)P–H bond to alkenes represent one of the most important methods to form a C–P bond.4 Although this radical addition using peroxide,5 AIBN,6 Et3B,7 air/nitrogen,8 and organic dye/photoirradiation9 etc. as the radical initiators has been achieved, more efficient and practical strategies are still highly desirable.
As our continuous investigations on the C–C bond formation via free-radical processes,10 we began to question whether a C–P bond could be formed via a single-electron-transfer (SET) process. As demonstrated in Scheme 1, single-electron oxidation of the secondary phosphine oxide followed by a deprotonation would generate a P-centered free radical. Addition of the phosphinyl radical to an olefin followed by hydrogen abstract from the phosphine oxide would lead to the product by hydrophosphinylation of alkene and regenerate the phosphinyl radical. Fortunately, we successfully accomplished an Ag(I)-initiated intermolecular hydrophosphinylation of a wide range of unactivated alkenes with phosphites (Scheme 1).
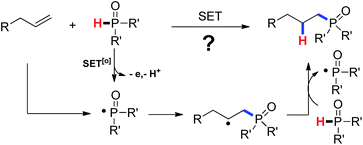 |
| Scheme 1 Free radical hydrophosphinylation of alkene via SET. | |
Initially, a series of experiments were carried out to test the hypothesis for hydrophosphinylation of unactivated alkenes with phosphites through a one-electron transfer process. It can be seen from Table 1 that the desired product was isolated in nearly quantitative yield by using catalytic amount of AgF (20 mol%), which was more efficient than other silver salts such as Ag2CO3, AgNO3, and AgOAc etc. (Table 1, entries 1–7). Further optimization of the typical reaction conditions indicated that the solvent, concentration as well as the temperature also affected the reaction efficiency (entries 8–12). Furthermore, addition of persulfates such as K2S2O8 and (NH4)2S2O8 could slightly raise the yield of the product (entries 13 and 14).
Table 1 Modification of the typical reaction conditionsa

|
Entry |
Radical initiator (mol%) |
Solvent (mL) |
T (°C) |
Yieldb (%) |
Reaction conditions: pent-4-en-1-yl 4-chlorobenzoate (1 equiv., 0.25 mmol), diphenylphosphine oxide (4 equiv., 1.0 mmol), 24 h, unless otherwise noted. Isolated yields. K2S2O8 (3 equiv., 0.75 mmol) was added. (NH4)2S2O8 (3 equiv., 0.75 mmol) was added. |
1 |
— |
DMF (2) |
110 |
— |
2 |
Ag2CO3 (20) |
DMF (2) |
110 |
63 |
3 |
AgNO3 (20) |
DMF (2) |
110 |
26 |
4 |
AgOAc (20) |
DMF (2) |
110 |
50 |
5 |
AgF (20) |
DMF (2) |
110 |
96 |
6 |
AgF (5) |
DMF (2) |
110 |
46 |
7 |
AgF (10) |
DMF (2) |
110 |
70 |
8 |
AgF (20) |
DMSO (2) |
110 |
16 |
9 |
AgF (20) |
CH3CN (2) |
110 |
95 |
10 |
AgF (20) |
DMF (1) |
110 |
60 |
11 |
AgF (20) |
DMF (3) |
110 |
81 |
12 |
AgF (20) |
DMF (2) |
80 |
20 |
13c |
AgF (20) |
DMF (2) |
110 |
97 |
14d |
AgF (20) |
DMF (2) |
110 |
98 |
The substrate scope and functional group tolerance were demonstrated in Scheme 2. A wide range of terminal and internal unactivated alkenes are compatible to this system (entries 1–25). Various functional groups such as ester, halogen, ether, hydroxyl, amide and ketone etc. can all be well-survived. It is noteworthy that the free radical addition didn't happen at the internal C
C double bond but the terminal one when 7-(but-3-en-1-yloxy)-2H-chromen-2-one was used as the substrate (entry 13). (E)-Oct-2-ene afforded a regio-isomers with the ratio of 1.7/1 (entry 19). 2-Vinylpyridine also gave the corresponding product 25 in high yield. However, styrene and its derivatives are not effective in this system. Notably, H-phosphinates and H-phosphonates are proven to be effective substrates (entries 26–28). For example, ethyl phenylphosphinate afforded the desired product in 95% yield (entry 26). Addition of the dimethyl phosphonate and diethyl phosphonate to pent-4-en-1-yl 4-chlorobenzoate gave the corresponding products in 33% and 45% yields, respectively (entries 27 and 28). Obviously, H-phosphonates afford relatively low yields of the desired products, which might be due to the stability of the P-centered radicals. Finally, this reaction can be easily scaled up to gram level, which suggests that it can be potentially applied in chemical industry (eqn (1)).
|
 | (1) |
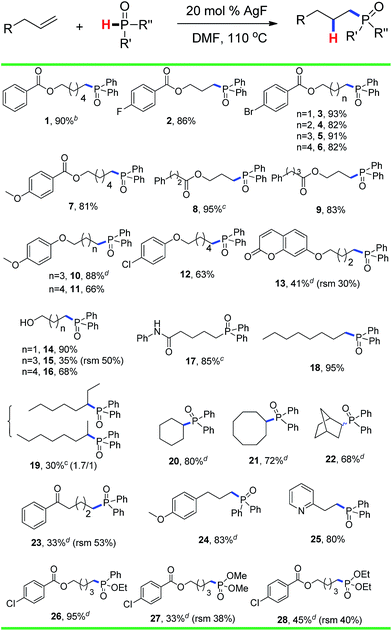 |
| Scheme 2 AgF-promoted hydrophosphinylation of alkenes with H–P(O). aReaction conditions: alkene (1 equiv., 0.25 mmol), organophosphorus compounds (4 equiv., 1.0 mmol), AgF (20 mol%, 0.05 mmol), DMF (2 mL), 110 °C, 24 h, unless otherwise noted. bIsolated yields. cK2S2O8(3 equiv., 0.75 mmol) was added. d(NH4)2S2O8(3 equiv., 0.75 mmol) was added. | |
Mechanistic studies including radical clock and ESR were carried out to confirm the previously proposed free radical process. As depicted in Scheme 3, ((4-methyl-1-tosylpyrrolidin-3-yl)methyl)diphenylphosphine oxide was obtained in 40% yield, which might proceed a radical addition/cyclization cascade process (Scheme 3a). In addition, ethyl 2-cyclopropylacrylate led to a ring opening product 30 in 42% yield (Scheme 3b). Furthermore, a series of experiments were designed to get evidences of key radical intermediates through spin trapping technology and ESR. As a result, the ESR signal of a P-centered radical species (g = 2.0060, aN = 1.411 mT; aH = 1.888 mT; aP = 3.475 mT) was observed by using 5,5-dimethyl-1-pyrroline N-oxide (DMPO) as a radical spin trap (Scheme 4). Overall, the proposed free radical addition mechanism is supported by these studies.
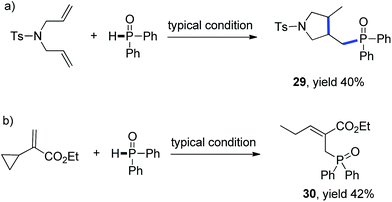 |
| Scheme 3 Radical clock experiments. | |
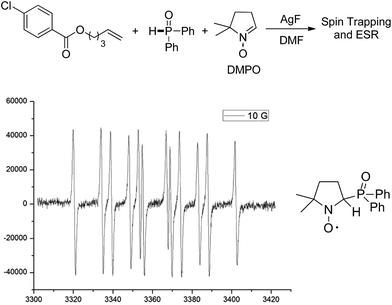 |
| Scheme 4 ESR studies. ESR spectrum of a solution of pent-4-en-1-yl 4-chlorobenzoate (5.0 × 10−2 mol L−1), diphenylphosphine oxide (0.2 mol L−1), AgF (1.0 × 10−2 mol L−1), and DMPO (6.0 × 10−2 mol L−1) in DMF (2 mL), 110 °C for 2.5 h. | |
In summary, a silver(I)-triggered free radical intermolecular C–P bond formation has been developed. A variety of alkyldiphenylphosphine oxides, alkyl phosphinates as well as alkyl phosphonates can be facilely prepared via addition of H–P(O) compounds with unactivated alkenes by using this strategy. The features of wide substrate scope, completely anti-Markovnikov addition and scalability make this methodology attractive to organophosphorus synthetic chemistry. Radical clock and ESR studies support the free-radical addition pathway.
Acknowledgements
This project is supported by the National Science Foundation of China (nos 21272096, 21472080).
Notes and references
- For reviews, see:
(a) Organic Phosphorus Compounds, ed. G. M. Kosolapoff and L. Maier, Wiley-Interscience, New York, 1972 Search PubMed;
(b) A. K. Bhattacharta and G. Thyagarajan, Chem. Rev., 1981, 81, 415 CrossRef;
(c) L. D. Quin, A Guide to Organophosphorus Chemistry, Wiley-Interscience, Hoboken, NJ, 2000 Search PubMed;
(d) C. Giorgio, O. Gianmauro and A. P. Gerard, Tetrahedron, 2003, 59, 9471 CrossRef PubMed;
(e) W. Tang and X. Zhang, Chem. Rev., 2003, 103, 3029 CrossRef CAS PubMed;
(f) D. T. Kolio, Chemistry and Application of H-Phosphonates, Elsevier Science, Oxford, 2006 Search PubMed;
(g) D. E. C. Corbridge, Phosphorus: Chemistry, Biochemistry and Technology, CRC Press, London, 6th edn, 2013 Search PubMed.
- For selected reviews on C–P bond formation, see:
(a) R. Engel and J. I. Cohen, Synthesis of Carbon–Phosphorus Bonds, CRC Press, Boca Raton, 2nd edn, 2003 Search PubMed;
(b) D. K. Wicht and D. S. Glueck, in Catalytic Heterofunctionalization, ed. A. Togni and H. Grützmacher, Wiley-VCH, Weinheim, 2001, ch. 5 Search PubMed and references cited therein;
(c) O. Delacroix and A.-C. Gaumont, Curr. Org. Chem., 2005, 9, 1851 CrossRef CAS;
(d) A. L. Schwan, Chem. Soc. Rev., 2004, 33, 218 RSC;
(e) M. Tanaka, Top. Curr. Chem., 2004, 232, 25 CrossRef CAS;
(f) F. Alonso, I. P. Beletskaya and M. Yus, Chem. Rev., 2004, 104, 3079 CrossRef CAS PubMed;
(g) C. Baillie and J. Xiao, Curr. Org. Chem., 2003, 7, 477 CrossRef CAS.
- For review on hydrophosphinylation, see: L. Coudray and J.-L. Montchamp, Eur. J. Org. Chem., 2008, 3601 CrossRef CAS PubMed.
- For reviews on free radical phosphonylation, see:
(a) D. Leca, L. Fensterbank, E. Lacôte and M. Malacria, Chem. Soc. Rev., 2005, 34, 858 RSC;
(b) S. Marque and P. Tordo, Top. Curr. Chem., 2005, 250, 43 CAS;
(c) A. Baralle, A. Baroudi, M. Daniel, L. Fensterbank, J.-P. Goddard, E. Lacote, M.-H. Larraufie, G. Maestri, M. Malacria and C. Ollivier, in Encyclopedia of Radicals in Chemistry, Biology and Materials, ed. C. Chatgilialoglu and A. Studer, Wiley, Chichester, UK, 2012, pp. 767–816 Search PubMedFor selected examples of radical C–P bond formation, see:;
(d) C. P. Casey, E. L. Paulsen, E. W. Beuttenmueller, B. R. Proft, B. A. Matter and D. R. Powell, J. Am. Chem. Soc., 1999, 121, 63 CrossRef CAS;
(e) L.-B. Han and M. Tanaka, Chem. Commun., 1999, 395 RSC;
(f) L.-B. Han, F. Mirzaei, C.-Q. Zhao and M. Tanaka, J. Am. Chem. Soc., 2000, 122, 5407 CrossRef CAS;
(g) S. Deprèle and J.-L. Montchamp, J. Am. Chem. Soc., 2002, 124, 9386 CrossRef PubMed;
(h) J. R. Moncarz, N. F. Laritcheva and D. S. Glueck, J. Am. Chem. Soc., 2002, 124, 13356 CrossRef CAS PubMed;
(i) M. O. Shulyupin, M. A. Kazakova and I. P. Beletskaya, Org. Lett., 2002, 4, 761 CrossRef CAS PubMed;
(j) L.-B. Han and C.-Q. Zhao, J. Org. Chem., 2005, 70, 10121 CrossRef CAS PubMed;
(k) T. Kagayama, A. Nakano, S. Sakaguchi and Y. Ishii, Org. Lett., 2006, 8, 407 CrossRef CAS PubMed;
(l) T. Hirai and L.-B. Han, J. Am. Chem. Soc., 2006, 128, 7422 CrossRef CAS PubMed;
(m) X.-J. Mu, J.-P. Zou, Q.-F. Qian and W. Zhang, Org. Lett., 2006, 8, 5291 CrossRef CAS PubMed;
(n) X.-Q. Pan, J.-P. Zou, G.-L. Zhang and W. Zhang, Chem. Commun., 2010, 46, 1721 RSC;
(o) W. Wei and J.-X. Ji, Angew. Chem., Int. Ed., 2011, 50, 9097 CrossRef CAS PubMed;
(p) X.-Q. Pan, L. Wang, J.-P. Zou and W. Zhang, Chem. Commun., 2011, 47, 7875 RSC;
(q) Y.-M. Li, M. Sun, H.-L. Wang, Q.-P. Tian and S.-D. Yang, Angew. Chem., Int. Ed., 2013, 52, 3972 CrossRef CAS PubMed;
(r) C. Zhang, Z. Li, L. Zhu, L. Yu, Z. Wang and C. Li, J. Am. Chem. Soc., 2013, 135, 14082 CrossRef CAS PubMed;
(s) B. Zhang, C. Daniliuc and A. Studer, Org. Lett., 2014, 16, 250 CrossRef CAS PubMed;
(t) W. Kong, E. Merino and C. Nevado, Angew. Chem., Int. Ed., 2014, 53, 5078 CAS;
(u) Z. Zhao, W. Xue, Y. Gao, G. Tang and Y. Zhao, Chem.–Asian J., 2013, 8, 713 CrossRef CAS PubMed;
(v) J. Xu, P. Zhang, X. Li, Y. Gao, J. Wu, G. Tang and Y. Zhao, Adv. Synth. Catal., 2014, 356, 3331 CrossRef CAS;
(w) Y.-R. Chen and W.-L. Duan, J. Am. Chem. Soc., 2013, 135, 16754 CrossRef CAS PubMed;
(x) Y. Gao, X. Li, J. Xu, Y. Wu, W. Chen, G. Tang and Y. Zhao, Chem. Commun., 2015, 51, 1605 RSC.
-
(a) O. Dubert, A. Gautier, E. Condamine and S. R. Piettre, Org. Lett., 2002, 4, 359 CrossRef CAS PubMed;
(b) A. Gautier, G. Garipova, C. Salcedo, S. Balieu and S. R. Piettre, Angew. Chem., Int. Ed., 2004, 43, 5963 CrossRef CAS PubMed.
-
(a) E. E. Nifant'ev, R. K. Magdeeva and N. P. Shchepet'eva, J. Gen. Chem. USSR, 1980, 50, 1416 Search PubMed;
(b) D. S. Karanewsky, M. C. Badia, D. W. Cushman, J. M. DeForrest, T. Dejneka, M. J. Loots, M. G. Perri, E. W. Petrillo and J. R. Powell, J. Med. Chem., 1988, 31, 204 CrossRef CAS;
(c) N. G. Anderson, M. L. Coradetti, J. A. Cronin, M. L. Davies, M. B. Gardineer, A. S. Kotnis, D. A. Lust and V. A. Palaniswamy, Org. Process Res. Dev., 1997, 1, 315 CrossRef CAS;
(d) C. M. Jessop, A. F. Parsons, A. Routledge and D. Irvine, Tetrahedron Lett., 2003, 44, 479 CrossRef CAS;
(e) M. I. Antczak and J.-L. Montchamp, Synthesis, 2006, 3080 CAS;
(f) C. M. Jessop, A. F. Parsons, A. Routledge and D. J. Irvine, Eur. J. Org. Chem., 2006, 1547 CrossRef CAS.
-
(a) S. Deprèle and J.-L. Montchamp, J. Org. Chem., 2001, 66, 6745 CrossRef PubMed;
(b) Y. Feng and J. K. Coward, J. Med. Chem., 2006, 49, 770 CrossRef CAS PubMed.
- T. Hirai and L.-B. Han, Org. Lett., 2007, 9, 53 CrossRef CAS PubMed.
-
(a) W.-J. Yoo and S. Kobayashi, Green Chem., 2013, 15, 1844 RSC;
(b) S.-I. Kawaguchi, A. Nomoto, M. Sonoda and A. Ogawa, Tetrahedron Lett., 2009, 50, 624 CrossRef CAS PubMed.
- For our recent contributions on free-radical-initiated C–C bond formation, see:
(a) Z.-Q. Liu, L. Sun, J. Wang, J. Han, Y. Zhao and B. Zhou, Org. Lett., 2009, 11, 1437 CrossRef CAS PubMed;
(b) Z. Cui, X. Shang, X.-F. Shao and Z.-Q. Liu, Chem. Sci., 2012, 3, 2853 RSC;
(c) Z. Li, Z. Cui and Z.-Q. Liu, Org. Lett., 2013, 15, 406 CrossRef CAS PubMed;
(d) Z. Li, Y. Zhang, L. Zhang and Z.-Q. Liu, Org. Lett., 2014, 16, 382 CrossRef CAS PubMed;
(e) Z. Li, F. Fan, J. Yang and Z.-Q. Liu, Org. Lett., 2014, 16, 3396 CrossRef CAS PubMed;
(f) L. Zhang, Z. Li and Z.-Q. Liu, Org. Lett., 2014, 16, 3688 CrossRef CAS PubMed;
(g) Z. Hang, Z. Li and Z.-Q. Liu, Org. Lett., 2014, 16, 3648 CrossRef CAS PubMed;
(h) Z. Xu, C. Yan and Z.-Q. Liu, Org. Lett., 2014, 16, 5670 CrossRef CAS PubMed;
(i) Y. Tian and Z.-Q. Liu, RSC Adv., 2014, 4, 64855 RSC;
(j) X.-J. Shang, Z. Li and Z.-Q. Liu, Tetrahedron Lett., 2015, 56, 233 CrossRef CAS PubMed.
Footnote |
† Electronic supplementary information (ESI) available. See DOI: 10.1039/c5ra04136h |
|
This journal is © The Royal Society of Chemistry 2015 |
Click here to see how this site uses Cookies. View our privacy policy here.