UVR: sun, lamps, pigmentation and vitamin D
Received
28th July 2016
, Accepted 18th October 2016
First published on 25th October 2016
Abstract
Exposure to ultraviolet radiation (UVR) has important and significant consequences on human health. Recently, there has been renewed interest in the beneficial effects of UVR. This perspective gives an introduction to the solar spectrum, UV lamps, UV dosimetry, skin pigment and vitamin D. The health benefits of UVR exposure through vitamin D production or non-vitamin D pathways will be discussed in this themed issue in the following articles.
Solar spectrum
Solar radiation includes ultraviolet radiation (UVR) 100–400 nm, visible radiation (400–780 nm) and infrared radiation (>780 nm) (Fig. 1).1 The wavelengths of UVR defined by the International Commission on Illumination (CIE) are UV-C (100–280 nm), UV-B (280–315 nm) and UV-A (315–400 nm). The UV component of terrestrial radiation from the midday sun comprises about 95% UV-A and 5% UV-B; UV-C and most of UV-B are removed from extraterrestrial radiation by stratospheric ozone.1 The CIE nomenclature is not always followed rigorously and some authors introduce slight variations; for example, distinguishing between UV-B and UV-A at 320 nm rather than 315 nm.1 However, these 5 nm can constitute an important difference when calculating UVR doses. For instance, if the 315 nm border is used, the solar irradiance consists of 2.11% UV-B (measured in Copenhagen in June) and 4.34% if the 320 nm border is used. UV-B has shorter and more energetic wavelengths than UV-A and is more effective in inducing various biological effects. Despite the lower photon energy, UV-A can also induce biological effects and penetrates more deeply into biological tissue than UV-B. The depth of UVR penetration into the skin is dependent on the wavelength. The longer the wavelength, the deeper the penetration.2
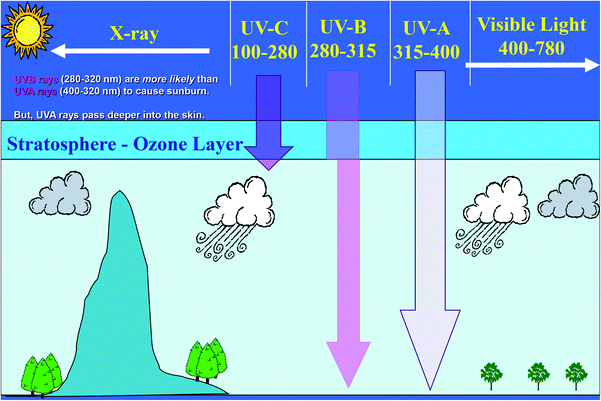 |
| Fig. 1 Ultraviolet radiation (UVR) is part of the electromagnetic spectrum. The CIE bands are: UV-C (100–280 nm), UV-B (280–315 nm) and UV-A (315–400 nm). However, in photobiology a distinction is often made between UV-B and UV-A at 320 rather than 315 nm. Visible light is the region between 400 nm and 780 nm. The atmosphere attenuates these types of UV differently, thus affecting the levels of UV-A, UV-B, and UV-C at ground level on the earth. | |
Ambient exposure
The intensity of solar UVR at the earth's surface is influenced by not only clouds, the ozone layer, air pollution and surface reflections but also the solar height, which is dependent on latitude, elevation, time of day, and season of the year.1 In the following we will describe the factors influencing the intensity of solar UVR in detail.
Weather, ozone layer and air pollution
The quality and quantity of UVR change as the sun's rays pass through the atmosphere. Ozone and pollution particles reduce the UVR reaching the earth's surfaces because they absorb, scatter, and reflect the wavelengths. In the stratosphere (10–50 km above sea level) UVR is absorbed by ozone and scattered by molecules such as N2 and O2.1 In the troposphere (0 to 10 km above sea level) UVR is further absorbed by ozone, NO2, and SO2 and scattered by particulates (e.g., soot) and clouds.3 Clouds are either water or ice droplets, which are very weak absorbers of UVR. Clouds scatter both UV-B and UV-A to the same extent.3 The amount of UVR ultimately reaching the surface of the earth is called ambient UVR.
Elevation, time of day, season and latitude
The angle between the sun and the local vertical is called the solar zenith angle.3 The elevation of the sun above the horizon is called the solar elevation. Both the spectrum (quality) and intensity (quantity) of ambient UVR vary with the solar elevation.3 The solar elevation depends on the time of day, day of year and geographical location (latitude and longitude).3 The UVR irradiance at ground level increases closer to the equator due to a shorter passage through the atmosphere.1 During the day the sun's elevation reaches its maximum around noon (90°) at the equator.1 This also means that approximately 50–60% of the diurnal ambient UVR is irradiated in the 4-hour period around local noon.3 During the year the sun's elevation is the highest around the summer solstice. Fig. 2 shows the number of standard erythema doses (SED) per hour during the day measured in July, April and December in Copenhagen, Denmark, 55.7°N, 12.5°E. An up to 5-fold decrease in erythema-effective UV-B may be seen when moving from the tropics to the most northern parts of Europe.1,4Fig. 3a shows non-erythema-weighted solar spectra in Denmark, Spain and at the equator. However, when the spectra are erythema-weighted (Fig. 3b) the difference in the UV-B area becomes clear. Not only is the overall level of ambient UVR greater at the equator and in Spain than in Denmark, but the spectra also contain a greater proportion of UV-B than in Denmark, which explains why there is a UV index nearly identical to 9 (SED per hour) in Spain and of 6 in Denmark during summer. The UV index is dimensionless and defined as the erythema-weighted UV intensity divided by 25 mW m−2, or more simply the erythema-weighted solar intensity, measured in W m−2, multiplied by 40 (m2 W−1).1 One standard erythema dose (SED) equals to 100 J m−2 erythema-weighted and since there are 3600 seconds in an hour, the solar intensity in number of SED per hour can be calculated as erythema-weighted solar intensity multiplied by 36 (SED per hour × m2 W−1). Even though the UV index is ∼11% higher than SED per hour, for practical purposes we can assume UV index = SED per hour.5
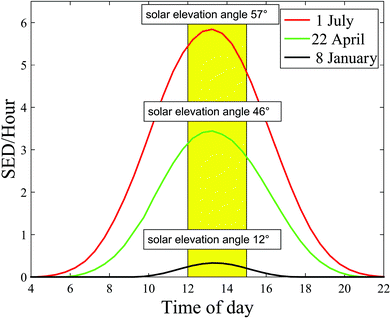 |
| Fig. 2 Number of standard erythema doses (SED) per hour during the day and solar elevation angle measured in July, April and January in Copenhagen, Denmark. | |
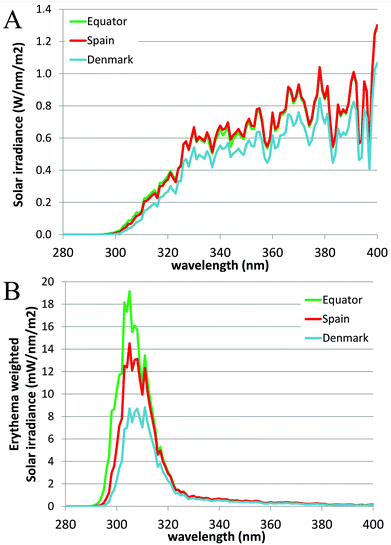 |
| Fig. 3 (a) Solar UVR spectra from the equator, Spain and Denmark. (b) The difference in UV-index (calculated by the area under the curve divided by 25 mW m−2) is visualized when the spectra are erythema-weighted. Not only is the overall level of ambient UVR greater at the equator and in Spain but the spectra also contain a greater proportion of SED than that in Denmark. | |
Surface reflections
UVR is reflected by surfaces such as snow, sand, concrete and water. Reflection of UVR from most ground surfaces is normally less than 10%.1,6 The main exceptions are snow, which can reflect up to 90% although reflectance of about 30–50% is probably more typical and white sand, which reflects about 10–30%.1,3,6 It is often believed that calm water reflects UVR but the figure is actually only about 5%, although up to 20% is reflected from choppy water.1,3,6 UVR goes easily through water, therefore bathing either in the sea or in open-air pools offers little protection against sunburn.3Fig. 4 shows how much UVR one can receive in different outdoor situations. The ambient UVR is 100% when there is no shade and no reflections. Ambient UVR is made up of both UVR directly from the sun and UVR diffused by scattering in the atmosphere. The diffused ambient UVR results in “sun in the shade” meaning that you can still receive about 50% of the ambient UVR if you are situated in the periphery of the shade from a tree compared with less than 15% of the ambient UVR at the centre of the tree's shadow (Fig. 4). Nevertheless, it is also possible to receive more than 100% when reflections from choppy water or sand occur (Fig. 4).
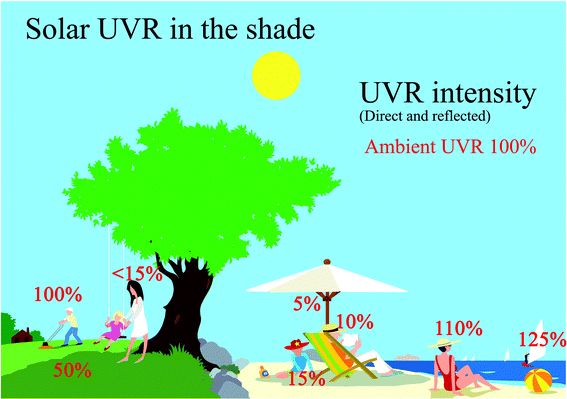 |
| Fig. 4 This figure shows the % of ambient UVR possible to be received in different outdoor situations. 100% ambient UVR consists of direct and diffuse light from the sun without reflections. | |
Personal exposure
The UVR dose an individual receives is also influenced by various parameters: the ambient UVR intensity, the exposure duration, the exposure geometry,6 UVR protection and the individual's behaviour.7
The ambient UVR intensity and exposure duration are self-explanatory terms. Exposure geometry means that the amount of UVR received on different body sites depends on their orientation towards the sun and any obstacles shielding or reflecting the UVR e.g. the urban canyon, mountains, hills and trees. Personal UVR exposure is of course also dependent on the use of sun protection like, sunscreen, shade and clothing. Individual behaviour is also important. UVR exposure received within the same time interval cannot be assumed to be a constant fraction of the ambient UVR exposure because humans react differently to the sun. This is so important that we shall describe sun behaviour in detail in the next section. It is possible to measure personal UVR received by using chemical or electronic UVR dosimeters.3 Chemical dosimeters, for example polysulfone films, have been used in a number of dosimetric studies in the past.3 However, electronic dosimeters can give much more detailed information. One such dosimeter is the SunSaver (Fig. 5) developed by the Department of Dermatology, Bispebjerg Hospital, Copenhagen.8 An example of data obtained from the SunSaver is shown in Fig. 6. This figure also illustrates the large difference in personal UVR exposure depending on an individual's behaviour.
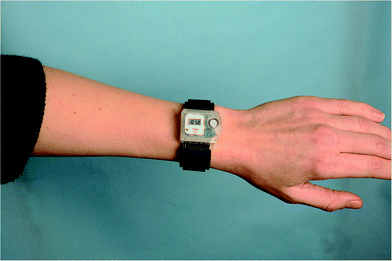 |
| Fig. 5 An example of an electronic dosimeter called the “SunSaver” developed by the Department of Dermatology, Bispebjerg Hospital, Copenhagen.8 An example of the data obtained from the SunSaver is shown in Fig. 6. The sensor can measure every 8th second and store an average of the last 75 measurements every 10 minutes along with the time. The measurement range of the dosimeter is 0.1 SED per hour to 23 SED per hour and it is battery-driven. The dosimeter can run for 145 days without maintenance, and the data can be transferred to a computer. | |
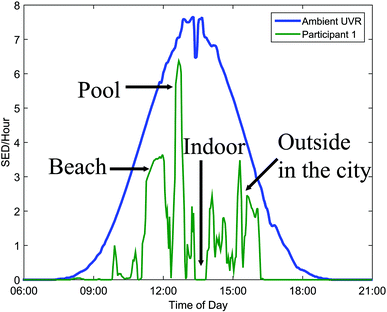 |
| Fig. 6 The mean daily distribution of ambient UVR expressed as standard erythema dose (SED) per hour. The green graph illustrates the large influence of behaviour on personal exposure. | |
Behavior
Studies have shown different behaviour patterns in the sun depending on nationality and culture. In northern latitudes sunshine is sparse and temperatures are low, especially in winter, and this influences people's behaviour when the sun gains power in spring. The difference between nationalities has been shown in a study in which Danish sun-seekers on a short holiday were outdoors significantly longer, received significantly higher percentages of ambient UVR, and received greater accumulated UVR doses than Spanish sun-seekers.9 The Danish sun-seekers received an average of 9.4 SED per day, while the highest daily dose received by one participant was 32 SED and the lowest dose was 2 SED received by another participant on the same day, so substantial differences can be observed on the same day at the same location. Another study investigating the personal UVR exposure of farming families in four European countries indicated a pronounced sun-seeking attitude among farmers, their partners and children at higher latitudes.10 The difference among cultures is shown in a study in which people of South Asian origin resident in the UK are compared with the indigenous UK population. Despite the fact that both groups spent the same time outside, the indigenous UK population received significantly more solar UVR during summer than the UK South Asians due to the latter's sun-avoiding behaviour.11 A skin cancer diagnosis also influences sun behaviour, but only for a short period of time. Newly diagnosed malignant melanoma patients exhibit cautious sun behaviour the first summer after their diagnosis.12 Afterwards, unfortunately, they tend to resume a careless attitude regarding daily UVR dose, days with body exposure, holidays and days abroad, whereas controls maintain a stable UVR exposure dose. Hence it is concluded that patients with CMM do not maintain the initial cautious sun behavior they exhibit the first summer after CMM diagnosis.13 Healthy people also exhibit the same sun exposure habits over the years.14 Sun exposure behaviour is probably as difficult to change as other habits.
Treatment with solar UV and UV lamps
Some thousand years ago solar UVR was already being used to treat different skin conditions. At that time, the importance of UVR was not known, as UV rays were not discovered before the 1800s. Just before the year 1900 Niels Ryberg Finsen introduced light therapy in medicine, first using focused sunlight, later using a carbon arc lamp in treating lupus vulgaris, a skin condition caused by Mycobacterium tuberculosis.15 It was believed that UVR was responsible for the effect of treatment and not until 2005 was it discovered that it was the wavelengths above 340 nm transmitted by Finsen's lens systems that were responsible. Fluorescence measurements on M. tuberculosis showed that the therapeutic effect of Finsen light therapy was probably due to photodynamic therapy with coproporphyrin III produced by Mycobacterium tuberculosis.16 The mercury vapour lamp was invented in 1906.17,18 These lamp types were used for phototherapy until the development of the modern UV lamps in the 1960s. The Goeckerman protocol was introduced in 1925 in the US where psoriasis and atopic eczema were treated with coal tar combined with UV-B.19 In the 1970s PUVA treatment was invented also for conditions like psoriasis.20 PUVA treatment is a combination of the chemical psoralen and UV-A (PUVA).21,22 Full spectrum UV-A (315–400 nm) induces crosslinks efficiently and therefore lamp types used for PUVA are often Cleo (Fig. 7) or a very similar TL09.23 PUVA is generally indicated for chronic plaque psoriasis and atopic eczema if UV-B has not been effective. Failure to respond adequately to UV-B does not predict failure of response to PUVA. PUVA is favoured over UV-B for some indications, such as mycosis fungoides beyond the patch stage, adult pityriasis rubra pilaris, pustular psoriasis, and hand and foot eczema. More than 200 PUVA treatments are linked to increased risk of squamous cell carcinomas.24
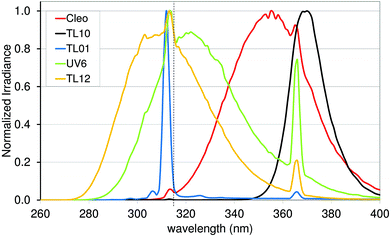 |
| Fig. 7 Emission spectra of light sources used in treatment of skin diseases. The dashed vertical line represents the border (315 nm) between UV-A and UV-B. | |
Today solar UVR is still used in the treatment of various skin disorders, e.g. at the Blue Lagoon, Iceland and also the Dead Sea and other Mediterranean areas, where patients with chronic skin diseases such as psoriasis are exposed to solar UVR.25–27 However, treatment with UVR from UV lamps is preferable; different UVR sources are used for different treatments and guidelines regarding the clinical use of phototherapy have been developed.28 Broadband UV-B is mainly used for radiation between 280 and 350 nm (Philips TL12) or from about 290 to 370 nm (Waldman UV6) (Fig. 6). Both types of lamps have proved effective in treating mild to moderate psoriasis and eczema. The narrow-band UV-B (TL01) lamp, in which just a narrow band around 311 nm is emitted, was developed for use in phototherapy as an alternative to a broad-band UV-B source and to photo chemotherapy. The narrow-band lamp has been proved to be particularly effective at clearing psoriasis but it has also been acknowledged that the TL01 lamp is probably 2–3 times more carcinogenic per minimum erythema dose than broadband UV-B.29 On the other hand, the cumulative dose required in therapy is less than when using broadband UV-B sources.29,30 Narrow-band UV-B is also more erythrogenic (by a factor of 1.6) than expected from the CIE erythema spectrum,31 and therefore adjustment of the UVR dose is needed in therapy if doses are given in SED.
Today phototherapy is still used to treat common skin conditions such as psoriasis, atopic eczema, other forms of dermatitis, generalised itching, pityriasis lichenoides, cutaneous T cell lymphoma, lichen planus, vitiligo and other less common conditions.
Tanning devices
Besides phototherapy artificial UVR sources are also used for cosmetic purposes. Commercial sunbeds were developed in the 1970s and came into widespread use in the 1990s. In 2006 the European Union (EU) Scientific Committee on Consumer Products published an opinion stating that UV tanning equipment should not exceed an erythema-weighted irradiance level of 0.3 W m−2 or 11 SED per hour (see definition in the following section), and that equipment above this level would be considered unsafe in tanning devices used for cosmetic purposes.
A recent review on UVR from indoor tanning devices concludes that in 8 out of 12 studies the erythema-weighted irradiance is above the European limit of 0.3 W m−2.32 In 10 out of 12 studies the erythema-weighted irradiance was higher than that of tropical sunlight (23°S and 23°N) with substantial variations between the devices.32 Also in 12 out of 13 studies UV-A irradiance from tanning devices was higher than that from natural sunlight (Melbourne, Australia, 37°S and Crete, Greece, 35°N).32 However, these studies did not discuss the duration of exposure (UVR doses) received but only the emitted irradiance. Another recent study shows that 52 tanning devices used regularly in Spanish facilities revealed a high variation in erythema-weighted irradiance, not the least in UV type 4 devices (29% of the sample), for which medical advice is required.33
UV dosimetry, erythema, photo skin type and pigment protection factor
Normally the effect of irradiation from UV lamps has been measured as physical irradiance (W m−2) or as a dose (J m−2). However, for the skin it is much better to use a biologically erythema-weighted dose called the standard erythema dose (SED) because erythema is the most common endpoint in UVR treatment of the skin.34 SED is calculated from the measured spectral irradiance of the lamp (W m−2 nm−1) multiplied by the CIE erythema action spectrum and integrated over the whole spectrum35 (black line in Fig. 8). An action spectrum, for a particular biological effect, expresses the effectiveness of radiation at each wavelength as a fraction of the effectiveness at a certain standard wavelength.36 Each biological effect of UVR such as erythema, pigmentation, vitamin D synthesis, etc. has its own action spectrum. An action spectrum can predict the effectiveness of an exposure to a certain spectral output (lamp). The biological effect on the skin will be identical for equal effective doses e.g. erythemal doses. The erythema effect of solar UVR can be seen in Fig. 3b and this figure illustrates why 320 nm is often used as the border between UV-B and UV-A within dermatology and skin photobiology. The thickness of the stratum corneum, cytokines and genetic factors such as pigmentation and responsiveness of the vascular epithelium determines how many SEDs can be tolerated without the skin being burnt.37 One SED is equivalent to an erythemal effective radiant exposure of 100 J m−2.34 The ambient exposure on a summer day with a clear sky in Europe is approximately 30–40 SED and an exposure dose of 4 SED would be expected to produce moderate erythema on unacclimatised white skin, but minimal or no erythema on previously exposed skin.3 An average dose received on a winter holiday on Tenerife is 9.4 SED per day for Danes and only 5.1 SED for Spaniards.9 Both doses are higher than the normally tolerated minimal erythema doses (MED), so without sun protection sunburn will occur. To induce erythema, the erythemal sensitivity of the skin to UVR is very wavelength dependent; at 300 nm a 100 times lower physical dose is needed than that at 320 nm. In Fig. 8 the solar UVR irradiance is depicted for summer and winter in Denmark. Not only is the overall level of ambient UVR greater during summer, but the spectrum also contains a greater proportion of UV-B than that in winter. The erythemal sensitivity of the skin is also dependent on the part of the body exposed.38 The trunk, head and neck are more sensitive than the extremities38 probably because the latter have thicker skin.39
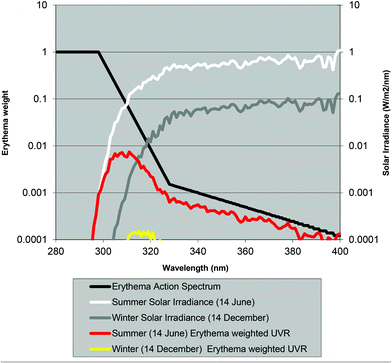 |
| Fig. 8 The CIE erythema action spectrum and solar UVR irradiance measured during summer and winter in Denmark. Not only is the overall level of ambient UVR greater during summer, but the spectrum also contains a greater proportion of UV-B than that in winter. | |
Individual erythema response can be assessed by determining MED, which increases with the degree of pigmentation, and is not predictive of skin phototype because there is considerable variation of MED within different white skin phototypes.40 Earlier the term MED was used widely as a ‘measure’ of erythemal radiation. This is a difficult measure to be used because MED is not a standard measure of anything and it is dependent on the individual's sensitivity to UVR and other variables such as optical and radiometric characteristics of the source; determinants of the exposure such as dose increment and field size; nature of the skin such as pigmentation, previous light exposure and anatomical site; and observational factors such as definition of the endpoint, time of reading after exposure and ambient illumination.3 In 1988, Fitzpatrick published a system for classifying skin phototypes.41 The system is based on self-assessment of the skin's erythema response and the ability to tan after a defined dose in the spring/early summer rather than the degree of pigmentation because pigmentation is difficult to measure. However, the system is strongly dependent on pigmentation. It is based on a simple 6-grade classification system with 4 grades for Caucasians and 2 grades for brown- and black-skinned people. Individuals complete a short questionnaire on their ability to get sunburned and the tendency to tan after the first unprotected solar exposure in the spring/early summer around noon. However, as an alternative to the subjective self-reported Fitzpatrick skin type method it is possible to use a more objective and reliable measure of sun sensitivity expressed as the pigment protection factor (PPF) measured by a skin reflectance meter.37 The PPF score expresses the number of SEDs needed to elicit just perceptible erythema on unexposed buttocks but can also be used on other anatomical sites. The meter has a measuring scale of 1.0–25.0 (1.0 is the PPF of the palest possible person while 25.0 corresponds to the darkest black skin).42
Skin pigment
The skin provides a barrier to harmful environmental effects and consists of three layers: the epidermis (top layer), the dermis (middle layer) and the subcutaneous layer. The epidermis consists mainly of epithelial keratinocytes and melanocytes, and some antigen presenting Langerhans cells and Merkel cells. Melanocytes reside in the lower part of the epidermis and are responsible for the synthesis of melanin within specialised membrane-bound organelles, termed melanosomes, and the subsequent transfer of the melanosomes to the surrounding keratinocytes.43 There are two types of melanin, eumelanin (brown-black) and pheomelanin (red-yellow).44–46 Eumelanin is a brown-black polymer of dihydroxyindole carboxylic acids and their reduced forms.44 Pheomelanin is a red-brown polymer of benzothiazine units largely responsible for red hair and freckles. Besides eu- and pheomelanin pigmentation can also be divided into constitutive pigmentation (the pigmentation you are born with) and facultative pigmentation (the additional pigmentation obtained by UVR).44 It is beyond the scope of the present article to present the details about melanins and melanogenesis but these are described very well in two reviews by d'Ischia et al.43,47
Within a few days of exposure to solar UVR delayed melanogenesis (tanning) occurs. This process is dependent on skin photo type and like erythema is primarily caused by UV-B. UV-A and UV-B induce pigmentation differently. UV-A induces immediate pigment darkening (within minutes after exposure) and delayed tanning (within days after exposure) while UV-B induces only delayed tanning. Immediate pigment darkening is a transitory darkening of the skin. Normally, the greater the constitutive pigmentation, the greater the tendency to develop immediate pigment darkening.48 An in vitro study has shown that the cutaneous melanocyte carries an opsine receptor tuned to a peak of about 360 nm, and that irradiation in the UV-A band activates this receptor which causes immediate pigment darkening.49 Darkening intensity is at its maximum immediately after exposure and decreases rapidly within 1–2 hours.48,50 Delayed tanning is seen about 1–2 days after exposure and may persist for several months.48 It is caused by an augmented activity of the enzyme tyrosinase, which results in a production of new melanin and more melanin granules.48 Different action spectra for delayed tanning (melanogenesis) in human skin have been published.48Fig. 9 shows standard action spectra and proposed standards for pigmentation.48 The standards are based on the data from Parrish et al. determining the action spectrum in Caucasians for wavelengths between 250 and 435 nm (ref. 51) and on Gange et al. who also investigated the action spectrum for melanogenesis but only at 3 different wavelengths and 8 and 24 hours after exposure. The action spectra for melanogenesis and erythema are very similar between 300 and 435 nm for fair untanned skin, which could mean that erythema and melanogenesis are initiated by the same photochemical events, i.e. the same chromophores.52 A UV-B tan is photo-protective against erythema but the level of photo protection is equivalent to a sunscreen with a sun protection factor (SPF) of 2–3.52 However, even though a SPF of 2–3 does not sound like much it actually means a reduction of the UVR dose of 50%. Fig. 10 shows a considerable seasonal variation for skin pigmentation at UVR exposed sites.53
 |
| Fig. 9 Standard action spectra and proposed standards for pigmentation. Adapted from ref. 48 with permission from the European Society for Photobiology, the European Photochemistry Association, and The Royal Society of Chemistry. | |
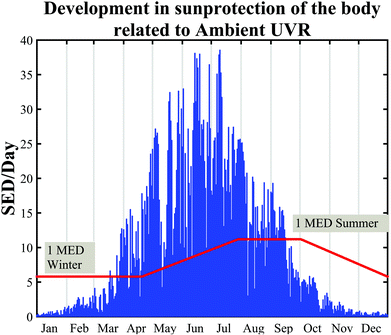 |
| Fig. 10 Considerable seasonal variation for skin pigmentation at UVR-exposed sites from 36 healthy volunteers. The blue “lines” are the daily SED during a year. The red line is the increase and decrease in PPF over the year, adapted from ref. 53. Ambient UVR is measured in Copenhagen, Denmark (55.7°N, 12.5°E). All participants have Danish ancestors. | |
Measurement of melanin levels in the skin has traditionally been via visual assessment performed by clinicians and dermatologists, which is clearly a subjective method.54 Portable instruments that measure reflected light to incidental light non-invasively in vivo are increasingly being used to quantify melanin.55,56 The term pigment protection factor (PPF) is used (described in the previous section) and studies have shown a positive correlation between PPF, phototype and MED.42,57 Our research group and two other research groups have used the quantification of the eumelanin monomer pyrrole-2,3,5-tricarcoxylic acid based on only High Pressure Liquid Chromatography (HPLC) or spectrophotometric analyses but these methods have very low throughput and high detection limits up to 2 μg.58 Recently, Szekely-Klepser et al. (2005) developed a novel and objective method for quantification of eumelanin from skin biopsies by Liquid Chromatography-Mass Spectrometry (LC-MS).59 Immunohistological stainings of melanin are available such as Fontana Masson but they cannot differentiate between eu- and pheomelanin.
In addition to pigmentation, skin thickening especially of the stratum corneum is another UVR-protective mechanism for avoiding further damage.60 UVR-induced skin adaptation/hardening is very dependent on the spectrum of irradiance.61 UV-B enriched sources induced much more epidermal thickening of the skin than UV-A.61
Vitamin D
Exposure to UV-B initiates the synthesis of vitamin D in the skin.62 There is a well-established relationship between the vitamin D status and musculoskeletal health. However, the association between vitamin D levels and other chronic diseases is less clear. High levels of vitamin D have been linked to a reduced risk of internal malignancies.63–69 The marker for vitamin D status is 25-hydroxyvitamin D, a metabolite of vitamin D3. No internationally well-defined values for optimal serum 25-hydroxyvitamin D currently exist (reviewed in ref. 70). However, it is generally believed in Europe that values >50 nmol l−1 (20 ng ml−1) are sufficient. 25-Hydroxyvitamin D levels are often both defined in units of nmol L−1 and ng ml−1
†. A single cutoff value has been debated and a recent study demonstrated a major inter-personal variation in the 25-hydroxyvitamin D response to UV-B as well as the maximal UV-B-induced 25-hydroxyvitamin D level.71 Different action spectra using rats, chickens and humans for the conversion of 7-DHC to previtamin D3 by UVR exist (summarized in ref. 72). All the results, irrespective of the method, assess the optimal wavelength to be in the range 295–303 nm. Furthermore, the production is very low or non-existing above 310 nm. This also includes a recently published action spectrum using ex vivo pig skin and UV light-emitting diodes (LED) to create narrowband UVR.72 The mostly used action spectrum for the conversion of 7-DHC to previtamin D3 by UVR in human skin was determined by MacLaughlin et al. in 1982.73 The same data were further defined and extrapolated by the CIE in 200674 (Fig. 11). The accuracy of the action spectrum has been questioned.75 An updated action spectrum for the conversion of 7-DHC to previtamin D3 by UVR on human skin is under development.76 Studies have shown that serum 25-hydroxyvitamin D concentrations are lower in humans with dark skin than those in fair-skinned humans.77,78 However, the association between skin pigmentation and the change in blood concentrations of 25-hydroxyvitamin D following experimental UV irradiation is not fully elucidated. A recent review found seven studies in which skin pigmentation influenced vitamin D production and five studies in which skin pigmentation did not influence vitamin D production.79 However, when comparisons of studies are performed, possible confounders like time of year, dietary supplementation habits and the 25-hydroxyvitamin D analysis method should be kept in mind.80 Different analytical quantification methods are available, including high-performance liquid chromatography (HPLC), and combined high-performance liquid chromatography and mass spectrometry (LC-MS), radioimmunoassays, enzyme immunoassays, competitive protein-binding assays, automated chemiluminescence protein-binding assays and chemiluminescence immunoassays.81 However, the sensitivity and specificity of these methods vary considerably. The LC-MS method is the most precise and accurate and is the gold standard.80 Snellman et al. analysed blood samples for 25-hydroxyvitamin D using three different methods and found high variability between the different assays.81 Mean 25-hydroxyvitamin D levels were the highest for the LC-MS technique (85 nmol L−1), intermediate for radioimmunoassay (70 nmol L−1) and the lowest for chemiluminescence immunoassays (60 nmol L−1).81 Using the 50 nmol L−1 cutoff, 8% of the subjects were found to be insufficient using by LC-MS, 22% by radioimmunoassay and 43% by chemiluminescence immunoassays.81
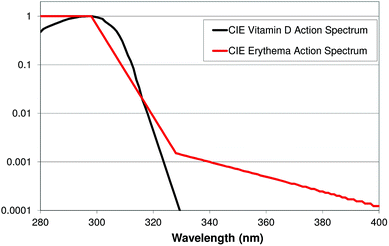 |
| Fig. 11 CIE Vitamin D action spectrum and CIE erythema spectrum. | |
The health benefits of UVR exposure through vitamin D production or non-vitamin D pathways will be discussed in this themed issue in the following articles.
Acknowledgements
The work was funded by The Danish Council of Independent Research (Grant number 0602-01931B) and The Capital Region of Denmark (Grant number R135-A4663).
References
-
WHO, IARC Monographs on the evaluation of carcinogenic risk to humans, Solar and Ultraviolet Radiation, 1992, vol. 55, pp. 1–316 Search PubMed.
- W. A. Bruls, H. Slaper, J. C. van der Leun and L. Berrens, Transmission of human epidermis and stratum corneum as a function of thickness in the ultraviolet and visible wavelengths, Photochem. Photobiol., 1984, 40, 485–494 CrossRef CAS PubMed.
- B. L. Diffey, Sources and measurement of ultraviolet radiation, Methods, 2002, 28, 4–13 CrossRef CAS PubMed.
- G. Seckmeyer, D. Pissulla and M. Glandorf,
et al., Variability of UV irradiance in Europe, Photochem. Photobiol., 2008, 84, 172–179 CAS.
- H. C. Wulf and P. Eriksen, UV index and its implications, Ugeskr. Laeg., 2010, 172, 1277–1279 Search PubMed.
- N. Kromann, H. C. Wulf, P. Eriksen and H. Brodthagen, Relative ultraviolet spectral intensity of direct solar radiation, sky radiation and surface reflections. Relative contribution of natural sources to the outdoor UV irradiation of man, Photodermatology, 1986, 3, 73–82 CAS.
- B. L. Diffey, C. J. Gibson, R. Haylock and A. F. McKinlay, Outdoor ultraviolet exposure of children and adolescents, Br. J. Dermatol., 1996, 134, 1030–1034 CrossRef CAS PubMed.
- J. Heydenreich and H. C. Wulf, Miniature personal electronic UVR dosimeter with erythema response and time-stamped readings in a wristwatch, Photochem. Photobiol., 2005, 81, 1138–1144 CrossRef CAS PubMed.
- B. Petersen, M. Triguero-Mas and B. Maier,
et al., Sun behaviour and personal UVR exposure among Europeans on short term holidays, J. Photochem. Photobiol., B, 2015, 151, 264–269 CrossRef CAS PubMed.
- M. Bodekaer, G. I. Harrison and P. Philipsen,
et al., Personal UVR exposure of farming families in four European countries, J. Photochem. Photobiol., B, 2015, 153, 267–275 CrossRef CAS PubMed.
- R. Kift, J. L. Berry, A. Vail, M. T. Durkin, L. E. Rhodes and A. R. Webb, Lifestyle factors including less cutaneous sun exposure contribute to starkly lower vitamin D levels in U.K. South Asians compared with the white population, Br. J. Dermatol., 2013, 169, 1272–1278 CrossRef CAS PubMed.
- L. W. Idorn, P. Datta, J. Heydenreich, P. A. Philipsen and H. C. Wulf, Sun behaviour after cutaneous malignant melanoma: a study based on ultraviolet radiation measurements and sun diary data, Br. J. Dermatol., 2013, 168, 367–373 CrossRef CAS PubMed.
- L. W. Idorn, P. Datta, J. Heydenreich, P. A. Philipsen and H. C. Wulf, A 3-year follow-up of sun behavior in patients with cutaneous malignant melanoma, JAMA Dermatol., 2014, 150, 163–168 CrossRef PubMed.
- E. Thieden, J. Heydenreich, P. A. Philipsen and H. C. Wulf, People maintain their sun exposure behaviour in a 5-7-year follow-up study using personal electronic UVR dosimeters, Photochem. Photobiol. Sci., 2013, 12, 111–116 CAS.
-
N. R. Finsen, Om anvendelse i medicinen af koncentrerede kemiske lysstråler, Gyldendalske Boghandels Forlag, 1896 Search PubMed.
- K. I. Moller, B. Kongshoj, P. A. Philipsen, V. O. Thomsen and H. C. Wulf, How Finsen's light cured lupus vulgaris, Photodermatol., Photoimmunol. Photomed., 2005, 21, 118–124 CrossRef CAS PubMed.
- E. Kromayer, Quecksilberwassenlampen zurBehandlung von Haut und Schleimhaut, Dtsch. Med. Wochenschr., 1906, 48–51 Search PubMed.
- K. I. Møller and H. C. Wulf, Early lamp developments for phototherapy in dermatology, Forum for Nord Derm. Ven., 2005, 10, 20–24 Search PubMed.
-
L. E. Gibson and O. H. Perry, Goekerman Therapy, in Psoriasis, ed. H. H. Roenigk and H. I. Kaibach, Maarcel Dekker Inc., New York-Basel-Hong Kong, 1998, pp. 467–477 Search PubMed.
- J. W. Melski, L. Tanenbaum, J. A. Parrish, T. B. Fitzpatrick and H. L. Bleich, Oral methoxsalen photochemotherapy for the treatment of psoriasis: a cooperative clinical trial, J. Invest. Dermatol., 1977, 68, 328–335 CrossRef CAS PubMed.
- J. A. Parrish, T. B. Fitzpatrick, L. Tanenbaum and M. A. Pathak, Photochemotherapy of psoriasis with oral methoxsalen and longwave ultraviolet light, N. Engl. J. Med., 1974, 291, 1207–1211 CrossRef CAS PubMed.
- H. C. Wulf, Modified method for demonstrating sister chromatid exchange (SCE), Dan. Med. Bull., 1980, 27, 35–37 CAS.
- D. Bethea, B. Fullmer and S. Syed,
et al., Psoralen photobiology and photochemotherapy: 50 years of science and medicine, J. Dermatol. Sci., 1999, 19, 78–88 CrossRef CAS PubMed.
- R. S. Stern, L. A. Thibodeau, R. A. Kleinerman, J. A. Parrish and T. B. Fitzpatrick, Risk of cutaneous carcinoma in patients treated with oral methoxsalen photochemotherapy for psoriasis, N. Engl. J. Med., 1979, 300, 809–813 CrossRef CAS PubMed.
- D. J. Abels and J. Kattan-Byron, Psoriasis treatment at the Dead Sea: a natural selective ultraviolet phototherapy, J. Am. Acad. Dermatol., 1985, 12, 639–643 CrossRef CAS PubMed.
- J. H. Eysteinsdottir, J. H. Olafsson, B. A. Agnarsson, B. R. Luethviksson and B. Sigurgeirsson, Psoriasis treatment: faster and long-standing results after bathing in geothermal seawater. A randomized trial of three UVB phototherapy regimens, Photodermatol., Photoimmunol. Photomed., 2014, 30, 25–34 CrossRef PubMed.
- H. Matz, E. Orion and R. Wolf, Balneotherapy in dermatology, Dermatol. Ther., 2003, 16, 132–140 CrossRef PubMed.
- H. Moseley, D. Allan and H. Amatiello,
et al., Guidelines on the measurement of ultraviolet radiation levels in ultraviolet phototherapy: report issued by the British Association of Dermatologists and British Photodermatology Group 2015, Br. J. Dermatol., 2015, 173, 333–350 CrossRef CAS PubMed.
- A. A. El-Ghorr and M. Norval, Biological effects of narrow-band (311 nm TL01) UVB irradiation: a review, J. Photochem. Photobiol., B, 1997, 38, 99–106 CrossRef CAS.
- H. C. Wulf, A. B. Hansen and N. Bech-Thomsen, Differences in narrow-band ultraviolet B and broad-spectrum ultraviolet photocarcinogenesis in lightly pigmented hairless mice, Photodermatol., Photoimmunol. Photomed., 1994, 10, 192–197 CAS.
- A. B. Hansen, N. Bech-Thomsen and H. C. Wulf, Erythema after irradiation with ultraviolet B from Philips TL12 and TL01 tubes, Photodermatol., Photoimmunol. Photomed., 1994, 10, 22–25 CAS.
- L. T. Nilsen, M. Hannevik and M. B. Veierod, Ultraviolet exposure from indoor tanning devices: a systematic review, Br. J. Dermatol., 2016, 174, 730–740 CrossRef CAS PubMed.
- Y. Sola, D. Baeza, M. Gomez and J. Lorente, Ultraviolet spectral distribution and erythema-weighted irradiance from indoor tanning devices compared with solar radiation exposures, J. Photochem. Photobiol., B, 2016, 161, 450–455 CrossRef CAS PubMed.
- B. L. Diffey, C. T. Jansen, F. Urbach and H. C. Wulf, The standard erythema dose: a new photobiological concept, Photodermatol., Photoimmunol. Photomed., 1997, 13, 64–66 CrossRef CAS.
-
CIE, Erythema reference action spectrum and standard erythema dose, CIE S 007/E-1998 (ISO 17166:1999), Commission Internationale de l’ É clairage, Vienna, 1998, 1999.
- V. Fioletov, J. B. Kerr and A. Fergusson, The UV index: definition, distribution and factors affecting it, Can. J. Public Health, 2010, 101, I5–I9 Search PubMed.
-
H. C. Wulf, Methods and an apparatus for determining an individual's ability to stand ultraviolet radiation, US Patent4882598, 1986 Search PubMed.
- R. L. Olson, R. M. Sayre and M. A. Everett, Effect of anatomic location and time on ultraviolet erythema, Arch. Dermatol., 1966, 93, 211–215 CrossRef CAS.
- J. Sandby-Moller, T. Poulsen and H. C. Wulf, Epidermal thickness at different body sites: relationship to age, gender, pigmentation, blood content, skin type and smoking habits, Acta Derm.–Venereol., 2003, 83, 410–413 CrossRef PubMed.
- G. I. Harrison and A. R. Young, Ultraviolet radiation-induced erythema in human skin, Methods, 2002, 28, 14–19 CrossRef CAS PubMed.
- T. B. Fitzpatrick, The validity and practicality of sun-reactive skin types I through VI, Arch. Dermatol., 1988, 124, 869–871 CrossRef CAS.
- H. C. Wulf, P. A. Philipsen and M. H. Ravnbak, Minimal erythema dose and minimal melanogenesis dose relate better
to objectively measured skin type than to Fitzpatricks skin type, Photodermatol., Photoimmunol. Photomed., 2010, 26, 280–284 CrossRef PubMed.
- M. d'Ischia, K. Wakamatsu and F. Cicoira,
et al., Melanins and melanogenesis: from pigment cells to human health and technological applications, Pigm. Cell Melanoma Res., 2015, 28, 520–544 CrossRef PubMed.
- S. Ito and K. Jimbow, Quantitative analysis of eumelanin and pheomelanin in hair and melanomas, J. Invest. Dermatol., 1983, 80, 268–272 CrossRef CAS PubMed.
- D. Parsad, K. Wakamatsu, A. J. Kanwar, B. Kumar and S. Ito, Eumelanin and phaeomelanin contents of depigmented and repigmented skin in vitiligo patients, Br. J. Dermatol., 2003, 149, 624–626 CrossRef CAS PubMed.
- K. Wakamatsu and S. Ito, Advanced chemical methods in melanin determination, Pigm. Cell Res, 2002, 15, 174–183 CrossRef CAS.
- M. d'Ischia, K. Wakamatsu and A. Napolitano,
et al., Melanins and melanogenesis: methods, standards, protocols, Pigm. Cell Melanoma Res., 2013, 26, 616–633 CrossRef PubMed.
- A. W. Schmalwieser, S. Wallisch and B. Diffey, A library of action spectra for erythema and pigmentation, Photochem. Photobiol. Sci., 2012, 11, 251–268 CAS.
- N. L. Wicks, J. W. Chan, J. A. Najera, J. M. Ciriello and E. Oancea, UVA phototransduction drives early melanin synthesis in human melanocytes, Curr. Biol., 2011, 21, 1906–1911 CrossRef CAS PubMed.
- C. Routaboul, A. Denis and A. Vinche, Immediate pigment darkening: description, kinetic and biological function, Eur. J. Dermatol., 1999, 9, 95–99 CAS.
- J. A. Parrish, K. F. Jaenicke and R. R. Anderson, Erythema and melanogenesis action spectra of normal human skin, Photochem. Photobiol., 1982, 36, 187–191 CrossRef CAS PubMed.
- N. Agar and A. R. Young, Melanogenesis: a photoprotective response to DNA damage?, Mutat. Res., 2005, 571, 121–132 CrossRef CAS PubMed.
- J. Lock-Andersen and H. C. Wulf, Seasonal variation of skin pigmentation, Acta Derm.–Venereol., 1997, 77, 219–221 CAS.
- K. Miyamoto, H. Takiwaki, G. G. Hillebrand and S. Arase, Utilization of a high-resolution digital imaging system for the objective and quantitative assessment of hyperpigmented spots on the face, Skin Res. Technol., 2002, 8, 73–77 CrossRef PubMed.
- H. Takiwaki, Y. Miyaoka, N. Skrebova, H. Kohno and S. Arase, Skin reflectance-spectra and colour-value dependency on measuring-head aperture area in ordinary reflectance spectrophotometry and tristimulus colourimetry, Skin Res. Technol., 2002, 8, 94–97 CrossRef CAS PubMed.
- G. Zonios, J. Bykowski and N. Kollias, Skin melanin, hemoglobin, and light scattering properties can be quantitatively assessed in vivo using diffuse reflectance spectroscopy, J. Invest. Dermatol., 2001, 117, 1452–1457 CrossRef CAS PubMed.
- M. R. Tylman, J. Narbutt, M. Fracczak, A. Sysa-Jedrzejowska and A. Lesiak, Pigment protection factor as a predictor of skin photosensitivity–a Polish study, Acta Dermatovenerol. Croat., 2015, 23, 23–27 Search PubMed.
- B. Kongshoj, A. Thorleifsson and H. C. Wulf, Pheomelanin and eumelanin in human skin determined by high-performance liquid chromatography and its relation to in vivo reflectance measurements, Photodermatol., Photoimmunol. Photomed., 2006, 22, 141–147 CrossRef CAS PubMed.
- G. Szekely-Klepser, K. Wade, D. Woolson, R. Brown, S. Fountain and E. Kindt, A validated LC/MS/MS method for the quantification of pyrrole-2,3,5-tricarboxylic acid (PTCA), a eumelanin specific biomarker, in human skin punch biopsies, J. Chromatogr. B: Anal. Technol. Biomed. Life Sci., 2005, 826, 31–40 CrossRef CAS PubMed.
- M. Gniadecka, H. C. Wulf, N. N. Mortensen and T. Poulsen, Photoprotection in vitiligo and normal skin. A quantitative assessment of the role of stratum corneum, viable epidermis and pigmentation, Acta Derm.–Venereol., 1996, 76, 429–432 CAS.
- N. Bech-Thomsen and H. C. Wulf, Photoprotection due to pigmentation and epidermal thickness after repeated exposure to ultraviolet light and psoralen plus ultraviolet A therapy, Photodermatol., Photoimmunol.
Photomed., 1996, 11, 213–218 CrossRef CAS.
- R. Vieth, The role of vitamin D in the prevention of osteoporosis, Ann. Med., 2005, 37, 278–285 CrossRef CAS PubMed.
- J. Ahn, U. Peters and D. Albanes,
et al., Serum vitamin D concentration and prostate cancer risk: a nested case-control study, J. Natl. Cancer Inst., 2008, 100, 796–804 CrossRef CAS PubMed.
- D. M. Freedman, A. C. Looker, S. C. Chang and B. I. Graubard, Prospective study of serum vitamin D and cancer mortality in the United States, J. Natl. Cancer Inst., 2007, 99, 1594–1602 CrossRef CAS PubMed.
- E. Giovannucci, The epidemiology of vitamin D and cancer incidence and mortality: a review (United States), Cancer, Causes Control, Pap. Symp., 2005, 16, 83–95 CrossRef PubMed.
- N. Khazai, S. E. Judd and V. Tangpricha, Calcium and vitamin D: skeletal and extraskeletal health, Curr. Rheumatol. Rep., 2008, 10, 110–117 CrossRef CAS PubMed.
- L. Lu, J. Qiu, S. Liu and W. Luo, Vitamin D3 analogue EB1089 inhibits the proliferation of human laryngeal squamous carcinoma cells via p57, Mol. Cancer Ther., 2008, 7, 1268–1274 CrossRef CAS PubMed.
- H. G. Skinner, Vitamin D for the treatment and prevention of pancreatic cancer, Cancer Biol. Ther., 2008, 7, 437–439 CrossRef CAS PubMed.
- R. Z. Stolzenberg-Solomon, Vitamin D and pancreatic cancer, Ann. Epidemiol., 2009, 19, 89–95 CrossRef PubMed.
- E. S. LeBlanc, B. Zakher, M. Daeges, M. Pappas and R. Chou, Screening for vitamin D deficiency: a systematic review for the U.S. Preventive Services Task Force, Ann. Intern. Med., 2015, 162, 109–122 CrossRef PubMed.
- P. Datta, P. A. Philipsen and P. Olsen,
et al., Major inter-personal variation in the increase and maximal level of 25-hydroxy vitamin D induced by UVB, Photochem. Photobiol. Sci., 2016, 15, 536–545 CAS.
- L. L. Barnkob, A. Argyraki, P. M. Petersen and J. Jakobsen, Investigation of the effect of UV-LED exposure conditions on the production of vitamin D in pig skin, Food Chem., 2016, 212, 386–391 CrossRef CAS PubMed.
- J. A. MacLaughlin, R. R. Anderson and M. F. Holick, Spectral character of sunlight modulates photosynthesis of previtamin D3 and its photoisomers in human skin, Science, 1982, 216, 1001–1003 CAS.
-
CIE, Action spectrum for production of previtamin D3 in human skin, CIE Technical Report 174, Commission Internationale de l’ Éclairage, Vienna, 2006, 2006.
- M. Norval, L. O. Bjorn and F. R. de Gruijl, Is the action spectrum for the UV-induced production of previtamin D3 in human skin correct?, Photochem. Photobiol. Sci., 2010, 9, 11–17 CAS.
-
P. A. Philipsen, K. A. Morgan, G. Harrison, B. Petersen, H. C. Wulf and A. R. Young, The CIE action spectrum for pre-vitamin D requires adjustment to be valid in human skin in vivo, European Society for Photobiology, 2015 Congress, 2015 Search PubMed.
- S. S. Harris and B. Dawson-Hughes, Seasonal changes in plasma 25-hydroxyvitamin D concentrations of young American black and white women, Am. J. Clin. Nutr., 1998, 67, 1232–1236 CAS.
- S. Nesby-O'Dell, K. S. Scanlon and M. E. Cogswell,
et al., Hypovitaminosis D prevalence and determinants among African American and white women of reproductive age: third National Health and Nutrition Examination Survey, 1988-1994, Am. J. Clin. Nutr., 2002, 76, 187–192 Search PubMed.
- F. Xiang, R. Lucas, G. F. de and M. Norval, A systematic review of the influence of skin pigmentation on changes in the concentrations of vitamin D and 25-hydroxyvitamin D in plasma/serum following experimental UV irradiation, Photochem. Photobiol. Sci., 2015, 14, 2138–2146 CAS.
- H. C. Wulf, The relation between skin disorders and vitamin D, Br. J. Dermatol., 2012, 166, 471–472 CrossRef CAS PubMed.
- G. Snellman, H. Melhus and R. Gedeborg,
et al., Determining vitamin D status: a comparison between commercially available assays, PLoS One, 2010, 5, e11555 Search PubMed.
Footnote |
† The conversion for 25-hydroxyvitamin D is [nmol L−1] = 2.5 × [ng ml−1]. |
|
This journal is © The Royal Society of Chemistry and Owner Societies 2017 |