Changes of labile, stable and water-soluble fractions of biochar after two years in a vineyard soil†
Received
17th July 2023
, Accepted 3rd October 2023
First published on 5th October 2023
Abstract
The long-term stability of biochar in soil is a key factor for carbon storage and agro-environmental benefits in soil applications. Therefore, to better use biochar as both a sustainable soil organic amendment and a carbon sequestration technique, it is necessary to understand the biochar aging process in soil, as biochar characteristics can be altered by a variety of processes that occur in the environment. Reliable chemical characterization can be obtained through thermoanalytical techniques, which are known to provide a trustworthy estimation of the biochar stability in soil. In the present study, the changes of labile and stable fractions of biochar after two years of application in a vineyard field were quantified before and after the amendment. Aged biochar was manually isolated from the soil-biochar mixture, and its chemical properties were measured by elemental analysis, thermogravimetric analysis (TGA) and analytical pyrolysis (Py-GC-MS). Dissolved organic matter (DOM) was analyzed by elemental and UV-vis spectroscopy analysis. A slight decrease of carbon content (−13 ± 5%) after two years was associated with a significant drop of TGA labile (from 26 to 9%) and water-soluble carbon (from 3.1 to 0.8 mg g−1), concomitantly, the pyrolytic indices of aromaticity (e.g. toluene/naphthalene ratio) decreased. These results contrasted with the increase in the H/C and O/C ratios as stability indexes. The TGA stable fraction remained almost unaltered (49 vs. 52%) confirming the consistency of this index for recalcitrance. The results from Py-GC-MS and TGA techniques are consistent in highlighting significant changes in the biochar labile fraction due to the aging process. Instead, no changes were found in the stable fraction between the aged biochar and the fresh one, with important consequences on carbon cycling and soil sequestration.
Environmental significance
Biochar is proposed as an innovative and sustainable tool to mitigate different environmental issues. Research has demonstrated that biochar could be applied for carbon sequestration, soil amelioration, greenhouse gas emissions reduction and nutrient leaching decrement, as well as waste management. The evaluation of the real environmental benefits of using biochar requires a deep knowledge of how biochar chemistry is affected by the variety of time-dependent processes that occur in the environment, which are termed “aging”. The present study provides new insights related to the effects of aging process on the biochar properties applied to agricultural soils, with regard, particularly, to the quantity and quality characteristics of labile and recalcitrant fractions. It provides an estimation of the biochar stability in soil and on changing the characteristics of biochar applied to soil systems, increasing the knowledge on the potential aging impacts on the physicochemical properties of biochar and on its connected environmental implications.
|
Introduction
Biochar is a carbonaceous solid material obtained from the thermochemical conversion of biomass in an oxygen-limited environment. It has received much scientific interest due to its potential as a sustainable tool to mitigate different environmental issues. Many studies have investigated the possibility of using innovative materials such as compost, zeolites, biochar and engineered nanomaterials, in a green and circular economy loop, to improve soil fertility while providing agro-environmental benefits.1–3 From the economic and environmental point of view, it is interesting to utilize wastes as sources of materials that can be used for several agro-environmental applications. In this regard, biochar is an emerging important carbonaceous material that could be applied for carbon sequestration, soil amelioration, greenhouse gas emissions reduction and nutrient leaching decrement, as well as waste management.4–9 Recent studies suggest that biochar production can be a global economically beneficial endeavor to promote a sustainable valorization of lignocellulosic pruning waste (from orchards, wine, and forest management) towards a new bioeconomy route.10,11 Being conveniently produced and applied at the farm level, reducing unaffordable transportation costs, biochar solves the problem of open field burning of pruning residues, improving soil health and crop yield.12 Regarding the study area, as reported by Greggio et al.,13 in a region highly devoted to agriculture such as Emilia Romagna (Italy), the per capita amount of dry residual biomasses is about 1 tonne per year. Even considering a scarce conversion rate from biomass to biochar (pyrolysis or gasification), around 10%, the theoretical amount of producible biochar is impressive, and its production cost ranges from $571 and $1455 per tonnes.
However, the comprehension of real or possible agro-environmental benefits and drawbacks of using biochar requires a deep knowledge of how biochar chemistry is affected by the variety of time-dependent processes that occur in the environment, which are termed “aging”. Aging processes occurring in soil alter the biochar characteristics influencing its biogeochemical properties. An increasing number of studies highlights that biochars in the environment are exposed to biological, physical and chemical action, such as precipitation events,14 variations in temperature,15 oxidation,16 and biodegradation,17,18 that gradually change their chemical composition.19,20 Aging process can modify the physicochemical properties of biochar, including element composition, aromaticity, specific surface area, surface morphology, pH, ion exchange capacity, etc.8
Currently, many studies have been performed to determine the stability or mineralization of biochar in soils.5,12,19,21,22 Biochar is generally considered a highly stable substance due to its condensed aromatic nature, especially when produced at high temperatures.23,24 However, biochar organic matter (OM) contains at least two pools, namely, labile and stable OM.25–28 Labile and stable OM were referred to as the OM with low and high ability to resist mineralization, respectively. The labile fraction is degradable on annual to decadal timescales, depending on the type of feedstock and pyrolysis condition the biochar was produced from. Results of several studies reported that the decomposition rate of biochar showed a clear decrease pattern with experimental duration, with relatively rapid mineralization of labile organic carbon (OC) (∼2 years) and the remaining stable OC of biochars was not mineralized or was mineralized very slowly.21,27,29
Aromaticity and degree of aromatic condensation are regarded to be key structural factors affecting thermochemical degradation and environmental recalcitrance in biochar.22,30 They can be estimated by carbon–carbon bonds analysis by techniques such as solid-state 13C nuclear magnetic resonance spectroscopy (NMR), elemental analysis and molecular markers by means of pyrolysis-gas chromatography-mass spectrometry (Py-GC-MS)30,31 or the benzene polycarboxylic acid method.32 Moreover, it is often assumed that environmental recalcitrance is intimately connected with thermal stability associated with these structural factors (aromaticity and ring condensation).23,24 Thus thermal/chemical parameters have been proposed to classify the carbon sequestering potential of different biochars by a number of techniques, including thermogravimetry33–35 flash pyrolysis9,30,36 and hydropyrolysis (HyPy).30,37 Py-GC-MS is a powerful analytical technique that permits a direct assessment of biochar stability, providing information on the C structure of biochar through molecular markers. Thermogravimetric analysis (TGA) provides quantitative information on volatile matter (VM), which is a useful index for evaluating biochar stability.34,38
The objective of this work is to assess the aging impacts on the physicochemical properties of biochar and its long-term environmental and agricultural implication. Thus, this study is based on a multi-annual time-scale field experiment conducted in a new vineyard to determine the alterations induced by the environmental conditions, soil properties and native microbiota on the physical and chemical characteristics of biochar. In particular, for the first time, this study reports the combined use of Py-GC-MS and TGA to study aged biochar samples deriving from a 24 month biochar incubation experiment at real farm scale.
Materials and methods
Field experiment
The field experiment was performed in a new vineyard planted in March 2019 (cv Souvignon Kretos, rootstock SO4) in Emilia-Romagna, at Ri. Nova Soc. Coop. farm (721500 m E; 4907810 m N – WGS84/UTM Zone 32; EPSG: 32632) (Fig. 1). The trellis system is Guyot, oriented East-West, with plant row spacing 2.6 m and plants spaced 1 m apart along the row. The vineyard is equipped for emergency irrigations (drop irrigation), even if during these first years of plant growth the water was constantly supplied during dry periods (June–August) with a bi-weekly cadence and about 20 mm of rainfall per event. In March 2019, the biochar was distributed in furrows before shoot transplanting and accurately managed in order to place the amending medium at a depth of 0.15–0.30 m (Fig. SM1†). The treated parcels were randomly distributed in the field and carefully separated by 15 untreated plants in the row and by a complete untreated row between the 50 treated plots (Fig. 1). The shoots were mechanically transplanted in correspondence to the amended furrows locating their roots in tight contact with amending medium.
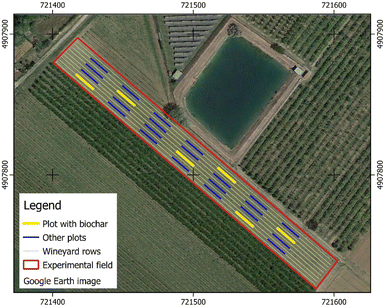 |
| Fig. 1 Experimental field with plot location arranged on a basis of a randomized block design. Treated parcels on the same row are separated by an untreated parcel as a buffer to limit the cross-contamination during agronomical operations. Rows with treated parcels are separated by an untreated row. Each parcel consists of 15 vine plants. The yellow colour indicates the sampled parcels. | |
The aged biochar samples were collected in August 2021 from the five amended parcels (yellow parcels in Fig. 1). In detail, using a manual auger 3 replicas of soil samples containing biochar were collected from each parcel at a depth of 0–0.3 m, mixed and quartered in order to get a representative soil sample. Once at the laboratory, the biochar was manually separated from the soil by the method described in Dong et al.,20 without rinsing the samples with deionized water to remove adhering soil particles. Specifically, aged biochar particles with a diameter >0.5 mm from soil samples were separated by forceps until no visible biochar particles were present in soil samples. Then, as reported in Wang et al.,39 each collected biochar particle was cleaned by removing the apparent soil debris from its surface. Fresh biochar particles with a diameter >0.5 mm were collected from fresh biochar samples to compare the characteristics of fresh and aged biochar. Both fresh and aged biochars were dried at 40 °C, sieved (mesh size: 2 mm), and stored at −18 °C.
Soil properties
Soil at the site is a Eutric Cambisol (Loamic)40 containing 31% sand, 47% silt, and 22% clay with a pH of 7.6 (1
:
2.5 soil
:
water), 1.07% total organic carbon, 0.15% total nitrogen, 25.6 cmol(+) kg−1 cation exchange capacity, slope <2%. Since 2021 soil analysis are yearly performed following the legislative procedure.41 In 2021 the sample collection for the soil analysis coincided with the sample for biochar extraction.
Biochar production and characterization
The applied biochar was one of the few available biochar in the market as of 2019, with an annual industrial production rate exceeding 100 tonnes. It was compliant with Italian legislation for soil improvement, and it was listed as an approved soil amendment since 2015. The methodology employed for biochar production is of an artisanal nature and has been documented in previous studies.5,42,43 In brief, the vineyard pruning residues underwent a conversion process into charcoal through a slow pyrolysis procedure conducted at the “Romagna Carbone s.n.c.” production facility in Italy. The batch pyrolysis process occurred within a transportable cast iron ring kiln with a diameter of 2.2 meters, capable of holding approximately 2 tons of dry feedstock. Following manual ignition of the combustion, the furnace was sealed at the top with a lid, and progressive combustion ensued, consuming all available oxygen, and reaching its maximum temperature in less than 20 minutes. A measured peak temperature of 700 °C was maintained for 3–4 hours, with the average heating rate of 25–30 °C min−1. The pyrolysis process concluded autonomously, and the subsequent day, the biochar underwent sieving and separation based on size.
Biochar samples were oven-dried at 40 °C for 72 h and homogenized using an agate mortar and pistil and stored at −20 °C prior to analysis. Proximate analysis of biomass and biochar was carried out according to ASTM D7582 method with slight modification.34 The method determines relative moisture (M), volatile matter (VM), fixed carbon (FC) content during different analysis steps. For each analysis about 0.5 g of biochar sample was introduced in aluminum oxide crucible (volume of 20 mL) and covered with the specific lid. Then the crucible was inserted in the thermogravimetric analyzer (Eltra Thermostep, Eltra GmbH, Haan, Germany).
Elemental analysis was performed using a Thermo Scientific FLASH 2000 Series CHNS/O Elemental Analyzer (Thermo Fisher Scientific, Waltham, U.S.A.) to determine C, H, and N contents of the biochar samples. The content of carbonate and ash was determined in each biochar as previously reported by Rombolà et al.44 Measurements were performed in triplicate, and oxygen (O) content was determined by difference: O (%) = 100 − C (%) − H (%) − N (%) − Ash (%). Moisture contents were determined (ASTM D-3173) at 105 °C. A mixture of biochar with deionized water at 1
:
10 wt/wt ratio was prepared, thoroughly mixed and pH measured at room temperature with a digital pH meter (VWR pH 100, VWR International).
Characterization of biochar labile and stable fraction
The parameters of the thermally labile fraction were determined by thermogravimetric analysis (recalcitrant OM, T 390–600 °C) and Py-GC-MS (molecular ratios).
Analytical pyrolysis.
Analytical pyrolysis was performed using a multi-shot pyrolizer (EGA/PY-3030D, Frontier Laboratories Ltd, Japan) interfaced to a gas chromatograph (GC) coupled with mass spectrometer (MS) (7890B GC and 5977B MS, Agilent Technologies, USA). A small crucible capsule containing weighed biochar sample (ca. 10 mg) was introduced into the furnace and then pyrolyzed at 700 °C for 100 s using helium as carrier gas (1 mL min−1) and an interface temperature of 280 °C. Pyrolysis products were introduced into the GC fused silica capillary column (HP-5MS, stationary phase 5%-diphenyl, 95%-dimethylpolysiloxane 30 m × 0.25 mm i.d. × 0.25 μm film thickness, Agilent Technologies, USA) with a 20
:
1 ratio. The injector temperature was 280 °C and the thermal program used was: 50 °C to 310 °C at 10 °C min−1, then a hold for 5 min at 310 °C. The MS operated in electron ionisation at 70 eV with scans in the 29–600 m/z range. The temperatures of the ion source and the transfer line were 230 and 250 °C, respectively. The quantity of evolved pyrolysis products (Py-yield) was calculated by dividing the GC peak area by sample amount (area per μg) as reported in Conti et al.34
Thermogravimetric analyses.
Thermogravimetric analysis was conducted by Eltra Thermostep thermogravimetric analyzer (Eltra GmbH, Haan, Germany) under an oxidant atmosphere (air, 90 mL min−1) at 10 °C min−1 to 600 °C for organic material (OM) determination, then they were ramped at 25 °C min−1 to 850 °C for carbonate determination.45 A sample mass of 0.5 ± 0.1 g in a 20 mL alumina crucible was used for each experiment and three replications were performed to test the reproducibility of the results. Specifically, the TGA method allows to determine mass loss relative to labile OM (220–390 °C), recalcitrant OM (390–600 °C) and carbonates (600–800 °C) fraction.44 The mass loss (TG) and derivative curves (DTG) of the samples were represented as a function of temperature.
Biochar-derived DOM extraction and analysis
Dissolved Organic Matter (DOM) was extracted from the fresh and aged biochar according to method reported in Ghidotti et al.,46 and in Rombolà et al.44 Dissolved organic carbon (DOC) and dissolved total nitrogen (DTN) in each DOM extracted from fresh and aged biochar were determined with a total organic carbon analyzer (TOC-L, Shimadzu, Japan) coupled to a TN module (TNM-L, Shimadzu, Japan). DOC and DTN in fresh and aged biochar were analyzed in triplicate, and their standard deviations are presented in Table 5.
The concentration of water-soluble organic carbon (WSOC) (mg g−1 TOC) in fresh and aged biochar was determined as the proportion of biochar OC pool extracted by the water extraction method. In particular, as reported in Rombolà et al.,44 the values were calculated using the following eqn (1):
| 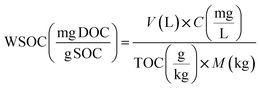 | (1) |
Spectroscopic characterization.
The biochar-derived DOM was characterized using UV-visible spectroscopy. Absorbance was measured using a Cary 300 UV-visible spectrophotometer (Agilent Technologies, USA) within a spectrum of 250–800 nm, at 1 nm increments, using a 1 cm quartz cuvette. Ultrapure water (18.2 MΩ cm) was used as a reference.
The specific UV absorbance at 254 nm (SUVA254, L mg−1 m−1), a proxy for DOM aromaticity, was calculated as follows: SUVA254 = a254/DOC. The a254 indexes is the absorption coefficient at wavelength 254 nm and was calculated using the eqn (2).
| 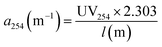 | (2) |
where UV
254 is the absorbance at 254 nm, 2.303 is the transform coefficient, and
l the path length of the cuvette in meters.
47
Statistical analysis
Quantitative data of fresh and aged biochar used to compare results are presented as the mean and standard deviation of three replicates. Data were analyzed using an analysis of variance (ANOVA) procedure for independent samples followed by Tukey's post hoc tests using R Statistical Software (Ver. 4.2.2, 2022, The R Foundation for Statistical Computing). The different properties between fresh and aged biochar were analyzed by t-test (p < 0.05).
Results and discussion
Soil modification due to biochar amendments
After two years of experimentation, the soil properties were slightly modified by the biochar amendment. In Table 1 the main properties of control and biochar-amended soil are resumed.
Table 1 Main soil properties for biochar amended and control soils, mean values and standard deviation in parentheses (n = 3). Data refer to 2021 sample campaign
Sample |
H2O reaction |
EC 1 : 2.5 |
TOC |
TN |
Available P |
Available K |
pH |
dS m−1 |
% |
% |
mg kg−1 |
mg kg−1 |
Biochar amended soil |
7.93 (0.13) |
0.33 (0.09) |
1.51 (0.06) |
0.15 (0.015) |
17.6 (2.7) |
140.4 (48.0) |
Control soil |
7.77 (0.32) |
0.29 (0.10) |
1.07 (0.02) |
0.15 (0.002) |
15.6 (3.2) |
106.3 (43.9) |
The soil pH has been slightly increased by the biochar amendment (from 7.77 to 7.93), even if the high standard deviation in the control parcels highlights the heterogeneity of the soil. The addition of biochar to soil results in a weak increase in EC and a larger increase in TOC and in available P and K, while it does not modify TN content. These modifications are perfectly aligned with outcomes from Schmidt et al.,48 as a result of global meta-analysis on biochar effects on agricultural soils.
Effect of aging on biochar properties
Elemental composition and basic features of biochar with aging at field.
The biochar used in the experiment for soil amendment had a total content of C, N, H and O 80%, 1.7%, 0.5%, 2.5% (dry weight), respectively; 15.2% ash and pH 9.96. The molar H/C and O/C ratios of 0.25 and 0.02, respectively, indicate a high degree of carbonization and aromaticity.24,49,50
The C content decreased from 80 ± 1.9% in the fresh to an average of 67 ± 3.3% with aging (Table 2). Therefore, through the aging process, biochar C content significantly decreased by 13 ± 5% (p < 0.05). Such effect can be due to decomposition of labile C through abiotic and biotic processes51 that take place in the environment, including abiotic and biotic redox reactions, solubilization and interactions with microbes, OM, minerals and solutes in the soil environment.52
Table 2 Proximate and ultimate analysis (oxygen by difference), molar ratios and pH of fresh and aged biochar applied in the field experimental. Mean values ± s.d. (n = 3)
Parameters |
Units |
Fresh biochar |
Aged biochar |
TC |
% |
80 ± 1.9 |
67 ± 3.3 |
TOC |
% |
79 ± 1.9 |
66 ± 3.4 |
TIC |
% |
0.70 ± 0.04 |
0.29 ± 0.08 |
N |
% |
1.66 ± 0.11 |
1.77 ± 0.08 |
H |
% |
0.50 ± 0.01 |
0.94 ± 0.08 |
O |
% |
2.5 ± 1.5 |
10.6 ± 2.1 |
Ash |
% |
15.2 ± 0.4 |
20.1 ± 1.5 |
H/C |
Atomic |
0.25 |
0.32 |
O/C |
Atomic |
0.02 |
0.11 |
VM |
% |
26 ± 0.5 |
20.5 ± 1.4 |
FC |
% |
57 ± 0.9 |
61 ± 1.6 |
pH |
— |
9.96 ± 0.07 |
7.50 ± 0.07 |
Microorganisms likely played an important role in the biochar aging process. As an example, the C lost from abiotic laboratory incubations of biochar was 50–90% less than that of microbially inoculated incubations.16 Moreover, a fraction of biochar-derived C was likely lost through leaching of DOC. Mukherjee and Zimmerman53 measured a significantly high amount of DOC in the soil leachate of biochar amended soils. When in soil environments biochars are exposed to leaching often coupled with mild acid or base hydrolysis.
However, the 13 ± 5.2% reduction of total C concentration in aged biochar after two years soil incubation appears comparable to mean averages reported in literature.19,54,55 In particular, Sorrenti et al.,19 reported losses of total C by 14.5% for aged biochar in a 4 year field experiment, which was partly attributed to mineralization. The impacts of field aging on biochar were evidenced by Spokas et al.,54 who observed that in the pre and post weathered wood-derived biochars (∼3 years after application) there was a ∼16% loss of C, dropping from 78.3 to 62.5% carbon. However, this difference in C content could be related not solely to weathering losses but also to the trapped soil in the pores of post-weathering biochar samples and the formation of an organic coating on aged biochar. The formation of an organic coating on biochar was identified in soil-aged biochars that strengthens biochar–water interactions and thus enhances nutrient retention.56,57 This could imply a reduction of total C in the aged biochar due to increased uptake of mineral and organic compounds into the pore system or via organomineral coating in the aged biochar. Also noteworthy is the observation that all weathered biochar samples possessed higher analytical variability (increase in standard deviations; Table 2). Previous studies reported that one of the causes of this variability can be the presence of entrapped soil into the biochar pores.54,55
The decrease in C content is accompanied by a slight increase of ash, H and N contents, whereas O content of aged biochar (13.7%) was significantly higher than fresh biochar (2.5%). Similar increase of O was found by Campos et al.,58 which detected an increase of aryl C and O-containing functional groups after 2 year of soil application. Increase of O content can be due to abiotic or biotic oxidation, with a number of studies suggesting that abiotic oxidation plays the dominant role.16,59,60 Abiotic biochar oxidation has been observed to occur through various processes. Atmospheric oxygen-induced oxidation can introduce additional oxygen-containing functional groups, such as hydroxyl, carbonyl, and carboxyl, to the biochar surface. This mild oxidation process is slow at ambient temperature.61 Rainfall events can also result in biochar oxidation owing to the dissolved oxygen and nitrogen oxides in rainwater.62,63 Rainfall events can also lead to physical disintegration and acidification, thus causing labile carbon to be released as DOM, minerals to leach out and additional oxygen-containing functional groups, such as hydroxyl, carbonyl, and carboxyl, to be introduced to the biochar surface.
Concerning the N content, numerous studies have reported increases in organic and inorganic N content of aged biochar.14,19,54 However, in a 2 year field experiment, de la Rosa et al.,55 reported a decrease in N content for not wood-derived aged biochar. The impact of aging has been hypothesized as a critical factor for the interaction of biochar with the plant and soil systems, particularly for the sorption of nitrogen containing compounds.55,64 A relative enrichment could be due to contributions of dead vegetation, which were incorporated into the pores or adsorbed to the surface of the biochar.55 Similarly, previous studies reported that H content of aged biochar was significantly higher than fresh biochar.20,54
The molar H/C and O/C ratios of 0.25 and 0.02, respectively, indicate a lower degree of carbonization and aromaticity of aged biochar.24,50 As a result of weathering, the aged biochar had a significant alteration in the H/C and O/C ratios, which increased from 0.25 to 0.32 and from 0.02 to 0.11, respectively. After incubation in soil for 240 days, Huang et al.,65 reported that biochar of rice husk increased H/C and O/C ratios from 0.46 to 0.50 and from 0.26 to 0.33, respectively. Joseph et al.,14 observed a decrease in the total C of biochar samples (poultry waste and green waste biochar) and an increase in the oxygen content leading to an alteration in the O/C ratio from <0.2 to ∼0.75 after 2 years of field weathering. In the present study, the increase in the O/C ratio for aged biochar was not as large as the alteration documented in Joseph et al.,14 but it is the same as the increase (from 0.02 to 0.11) reported by Spokas.54 Both ratios reflect physical–chemical properties of biochar related to stability, as the proportion of elemental compounds (H and O) relative to C present in biochar, and the molar ratio of H/C and of both ratios have been adopted by IBI and EBC, respectively. The present study showed that after the aging period in soil results of biochar molar H/C and O/C ratios are inconsistent with those obtained by thermoanalytical techniques as discussed in the next section.
The pH value of fresh biochar through the aging process decreased from 9.96 to 7.50 and it is in accordance with the results of several studies.14,19,54 These studies have reported that the pH values of the biochars decreased with an aging period in the field as a consequence of the field weathering. Weathering typically results in the increased abundance of carbonyl, carboxylic, and phenolic functional groups on the biochar surface,58,66,67 which leads to a pH decrease in aged biochar.67 This suggests that biochar liming potential is limited to a few years after its application. Hence, biochar-induced benefits in nutrient availability in acid soils may be more pronounced in the first seasons following application.
Characterization of fresh and aged biochar by analytical pyrolysis.
Examples of TIC pyrograms related to the fresh and aged biochars obtained at 600 °C are depicted in Fig. 2. The results of Py-GC-MS analysis were used to estimate of thermal stability of fresh and aged biochars.68 In particular, the degree of thermal stability was estimated using specific molecular ratios (toluene/benzene (T/B), toluene/naphthalene (T/NAP), methylnaphthalene/naphthalene (MeNAP/NAP) and methylphenanthrene/phenanthrene (MePHE/PHE)). The degree of alkylation detected by Py-GC-MS is widely regarded as a molecular index of biochar thermal stability.30,34,36,38,69,70 Kaal et al.,36 proposed that the decrease in T/B and MeNAP/NAP ratios can be representative of dealkylation associated with polycondensation and aromatization that increase with charring. The validity of T/NAP and MePHE/PHE molecular ratio as proxies of charring extent was confirmed in previous research.34 These molecular indices were applied to characterize fresh and aged biochars. Results reported in Table 3 showed that the ratios were lower for the aged biochar in accordance with a decrease in the relative abundance of O-alkyl C and alkyl C.55,68,71 As reported in Table 3, Py-yields (area per μg) decreased from 390
000 ± 20
000 to 170
000 ± 48
000 showing effects of aging in soil on the thermally labile fraction of aged biochar. The changes in biochar composition with age can be summarized as a reduced diversity of products, a decrease in markers of non/weakly-charred biomass and a relative increase in the products of relatively condensed black carbon. These results suggest that, unsurprisingly, the more condensed aromatic compounds of biochar are selectively preserved during long-term storage in soil.
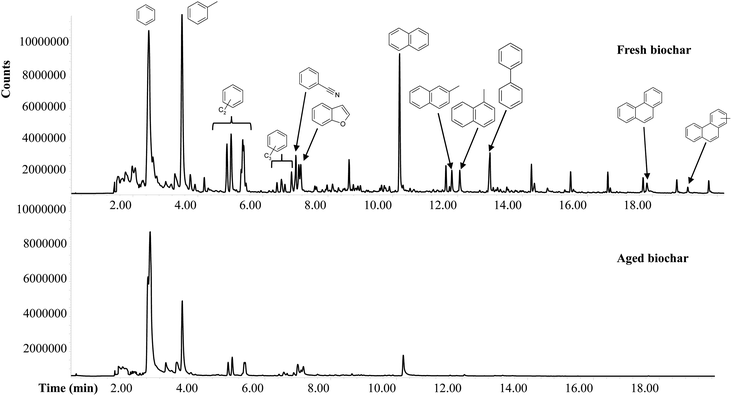 |
| Fig. 2 Total ion chromatograms from Py-GC-MS at 600 °C of fresh and aged biochar. | |
Table 3 Yields of pyrolysis products (peak area per μg) and molecular ratios MePHE/PHE (methylphenanthrene/phenanthrene), methylnaphthalene/naphthalene (MeNAP/NAP) toluene/naphthalene (T/NAP) and toluene/benzene (T/B) from Py-GC-MS at 600 °C of fresh and aged biochar. Mean values ± s.d. (n = 3)
Samples |
MePHE/PHE |
MeNAP/NAP |
T/NAP |
T/B |
Py-yield |
Fresh biochar |
0.256 ± 0.002 |
0.26 ± 0.04 |
1.8 ± 0.2 |
0.5 ± 0.1 |
390 000 ± 20 000 |
Aged biochar |
0.14 ± 0.01 |
0.16 ± 0.03 |
1.1 ± 0.3 |
0.26 ± 0.03 |
170 000 ± 48 000 |
Table 3 shows that the variability of molecular ratios between fresh and aged biochar was much greater (45% MePHE/PHE, 38% MeNAP/NAP, 39% T/NAP, 48% T/B) than that of TOC (13%), VM (6%) and labile OM (17%). Therefore, the molecular ratios may explain not only the mass loss or C loss alone during field aging but also the aging process that induces primary changes in the aromatic structure of biochar.
Good correlations between Py-GC-MS results and ratio of H/C were found in several research.24,34 In the present study, however, the depicted Py-GC-MS derived stability molecular ratios are not aligned with molar H/C and O/C ratios for fresh and aged biochars. The molar H/C and O/C ratios of 0.25 and 0.02 for fresh biochar and of 0.32 and 0.11 for aged biochar, respectively, indicate a greater degree of aromaticity and stability of fresh biochar than aged. Nevertheless, the degree of thermal stability estimated by Py-GC-MS using specific molecular ratios suggested that in aged biochar, through the aging process, the labile fraction significantly decreases. As discussed above, the data reported in this study suggest that molar H/C and O/C ratios do not seem to efficiently distinguish between the biochar with a higher level of labile C fraction such as that fresh from the aged biochar with a lower level of labile C fraction.
The data obtained by Py-GC-MS are coupled with the quantitative information on the weight loss with temperature obtained by TGA.
Thermal characterisation by TGA.
TG and DTG curves obtained from fresh and aged biochar are shown in Fig. SM2 and SM3.† As reported in previous works, the OM decomposition takes place into two main temperature intervals associated to labile (220–390 °C) and recalcitrant (390–600 °C) components.44,45,72 A third mass loss in the range 500–650 °C, associated to carbonate fraction was also proposed.45 Results of thermal analysis by TGA showed that labile OM of fresh biochar was 26.1 ± 0.6%, and it decreased to 9.1 ± 0.3% with aging (Table 4). Moreover, as reported in Table 4, recalcitrant OM slightly increased not showing significant impacts of aging in soil on the recalcitrant fraction of aged biochar. Therefore, these data show that, as expected, the field aging of biochar did not affect the mass loss in the thermal region associated to recalcitrant OM (390–600 °C), while it caused a significant decrease in the thermal region of labile OM (220–390 °C). Total labile OM concentration was reduced by 17% by aging, not consistent with the decomposed amount of the labile fraction estimated at roughly 6–7% after 2 years by the double first-order exponential decay model.29 Similar to other organic materials added into soils, biochar decomposition can be approximated well by biphasic patterns. However, the decomposition rates for the labile pool (∼0.0093% day−1) and recalcitrant pools (∼0.0018% year−1) estimated by the double first-order exponential decay model vary considerably for biochars from different feedstocks and pyrolysis conditions.29
Table 4 Weight loss of fresh and aged biochar samples determined by the TGA method. Labile OM from 220 to 390 °C, recalcitrant OM from 390 to 600 °C and carbonates from 600 to 800 °C. Mean values ± s.d. (n = 3)
Samples |
105–220 °C weight loss (%) |
220–390 °C weight loss (%) |
390–600 °C weight loss (%) |
600–800 °C weight loss (%) |
Residue (%) |
Fresh biochar |
6.9 ± 0.1 |
26.1 ± 0.6 |
48.7 ± 0.7 |
2.76 ± 0.05 |
15.5 ± 0.1 |
Aged biochar |
7.0 ± 0.1 |
9.1 ± 0.3 |
51.8 ± 0.3 |
3.26 ± 0.07 |
28.0 ± 0.7 |
Results from several studies reported that the decomposition rate of biochar showed a clear decrease pattern with experimental duration, with intensive initial decomposition which disappeared after 2 years and was maintained at a very low level for prolonged periods.18,21,73 In the field, therefore, optimal incubation conditions in the short-term period often involves the uppermost decomposition rates of the biochar labile fraction with respect to those estimated by the models.23,27,29,74 For instance, this might be caused by the physical breakdown of biochar structure during aging in the environment that would accelerate the degradation process.15
The trend resulting from TGA was confirmed by elemental analysis of fresh and aged biochar, with a decrease during the field aging in the C content and a slight increase in the stable structures. The results of Py-GC-MS analysis, as for TGA results, showed that the field aging process clearly influenced levels and molecular composition of thermally labile fraction. However, a variable fraction of the carbon in biochar is labile (degradable on annual to decadal timescales) and hence only a proportion of total carbon in biochar allows long-term storage of carbon in soils. Therefore, understanding the real or possible benefits and drawbacks of using biochar as tool for carbon sequestration requires knowledge of recalcitrance of carbon in biochar. According to results shown in this study, decreased molar ratio MePHE/PHE, MeNAP/NAP, T/NAP and T/B, as well as decreased TGA losses at low temperature, confirmed to be correlated with the stabile (2 year aged) fraction of the biochar. Therefore, TGA and Py-GC-MS confirmed to be useful tools for the estimation of biochar stability.
DOM analysis
Elemental analysis of fresh and aged biochar DOM.
The changes in DOC contents during the aging period in the field are shown in Table 5. Significant differences (ANOVA, p < 0.05) were observed in DOC and in WSOC due to aging in the field of biochar (Table 5), indicating that the biochar aging gave rise to a sharp decrease in DOC. Specifically, after 29 months of field aging, the biochar-derived DOC concentrations significantly decreased from 500 ± 34 mg L−1 in fresh biochar to 114 ± 14 mg L−1 in aged biochar, representing a loss of 77%.
Table 5 Total nitrogen (TN) in water-soluble matter, dissolved organic carbon (DOC) and water-soluble organic carbon (WSOC) concentration of fresh and aged biochar from field experiment. Mean values ± s.d. (n = 3)
Samples |
TN (mg L−1) |
DOC (mg L−1) |
WSOC (mg g−1 TOC) |
Fresh biochar |
0.5 ± 0.1 |
500 ± 34 |
3.1 ± 0.2 |
Aged biochar |
34.7 ± 0.4 |
114 ± 14 |
0.8 ± 0.1 |
In biochar-derivate DOM, moreover, more marked differences are observed between fresh and aged biochar in the dissolved fraction of total OC (WSOC = mg DOC g−1 TOC). The values of WSOC decreased after 24 months of field aging from 3.1 ± 0.2 mg g−1 TOC to 0.8 ± 0.1 mg g−1 TOC (Table 5).
In contrast, TN content of aged biochar DOM was higher than fresh biochar, with a significant increase from 0.5 ± 0.1 mg L−1 to 34.7 ± 0.4 mg L−1, respectively. This result is consistent with the increase of N content in aged biochar previously indicated. Moreover, this increase is in accordance with the findings of Yang et al.,75 who described a consistent decrease in the TN levels of the WSOM in biochar-amended soil due to the high abundance of micropores with a large specific surface area in higher-temperature biochar.
Total C concentration of the biochar was reduced by 13 ± 5.2% (Table 2) by aging after two years soil incubation. This decrease is partly due to biochar C-fraction lost through the leaching of DOC. Many recent studies focus solely on the content, composition and characteristics of biochar DOM,47,76,77 while few studies have reported how the degree of DOM release changes due to aging. In fact, aging leads to changes in the surface functionality and reactivity of biochar, leading to changes in the release of DOM.78 Furthermore, it was indicated that biochar might release indigenous DOM into soil and adsorb the intrinsic soil DOM at the same time.45,75,79 Rainfall events can also lead to the release of labile carbon as DOM but, during the aging process, it can be established a balance between release and adsorption. In this study the DOC of fresh biochar probably represents the amount of biochar-derived DOM that is readily released during the biochar application (especially when heavy storms happen), while the lower DOC concentrations in the aged biochars reflect the balance between release and adsorption.
UV-vis spectral characterization of fresh and aged biochar DOM.
The specific UV absorbance (SUVA254) is an index of the DOM aromaticity, where the absorbance coefficient measured at 254 nm is normalized by the DOC value of DOM sample. Higher UVA254 values indicated DOM composition with a larger molecular weight and more humic acid-like content,80 whereas lower UVA254 values (e.g., lower than 3) denoted abundant hydrophilic DOM substances.81Table 6 presents the change of SUVA254 values of biochar-derived DOM with the aging period in the field.
Table 6 Biochar derived DOM characteristics: specific absorbance (SUVA254), UV-vis absorption, spectral slope (SR) and absorption coefficient (A254). Mean values ± s.d. (n = 3)
Samples |
A
254 (m−1) |
SUVA254 (L mg−1 m−1) |
S
R
|
Fresh biochar |
2290 ± 220 |
4.7 ± 0.4 |
0.67 ± 0.02 |
Aged biochar |
1170 ± 90 |
10.7 ± 0.1 |
1.07 ± 0.01 |
The high initial SUVA254 value of about 4 L mg−1 m−1 reflects that the DOM in fresh biochar included high molecular weight and aromatic compounds, which were strongly unsaturated and mainly hydrophobic.82,83 The SUVA254 value of biochar through the aging process increased from 4.7 ± 0.4 to 10.7 ± 0.1 L mg−1 m−1, indicating that highly hydrophobic substances were likely contained in the DOM from aged biochar and that aging induces increases in the hydrophobic and aromatic components and a decrease in the smaller molecules (low molecular weight) after two years in soil.84 Therefore, after the incubation time biochar showed a rising trend in the levels of SUVA value as reported by Huang et al.,77 possibly implying the preferential utilization of non-aromatic fractions by microorganisms and/or the microbial transformation of simple labile substrates into aromatic carbon structures.85 Furthermore, due to high molecular and aromatic substances in DOM samples, the biochar-derived DOM would generally remain stable, with high resistance, after the aging period in the field. A consistent conclusion can be made from the SR value which varied from 0.67 ± 0.02 in fresh biochar to 1.07 ± 0.01 in aged biochar. Moreover, these results are in accordance with the findings of previous studies that examined the biochar impact on soil DOM.76,86,87 These studies showed that the values of SUVA254 significantly increased in the extracted DOM of biochar-amended soils relative to the control soils by high molecular and aromatic substances released from biochar as well as from the impact of biochar on the carbon dynamics and microbial communities in soil.47,80
Biochar stability: environmental implications
A fundamental condition for the use of biochar as a carbon storage is that the carbon in the biochar is stable, meaning that most of the carbon stored in biochar persists in soils for hundreds or even thousands of years.5,18 However, a variable C-rich component in many biochars is degradable on annual to decadal timescales and hence, only a fraction of total carbon in biochar provides long-term carbon storage.5,88,89
Our findings suggest that the effects of biochar on the environment may change with time, raising a number of questions. Aging of biochar changes its physiochemical properties such as composition, pH, element ratio and functional surface groups. Therefore, the aging process has significant consequences for the bioavailability and transport of nutrients and contaminants. Regarding the biochar stability or biochar's resistance to biotic and abiotic degradations in soil, results suggest that biochar used in field experiment contained two pools of OM, namely, labile and stable OM as in previous studies.18,27,30,90
The C loss from biochar during two years in an agricultural field was 13 ± 5.2% (Table 2), with a decrease in labile OM of aged biochar of 17 ± 0.3% (Table 4). The rate of C loss for biochar in the present field experiment is higher than those estimated by the models.23,27,29,74 In fact, according to the commonly used model to estimate biochar persistence in soil, the decomposed amount of biochar has been estimated to be roughly 6–7% after 2 years, although the decomposition rates depend on biochar characteristics.29 However, various factors lacking in laboratory incubation may accelerate the decomposition of biochar under field conditions.58,91 These factors include physical crushing and natural physical damage, freeze–thaw cycles, alternate drying and wetness, microbial activity and mixing of soil, destruction of soil aggregates, etc.
Furthermore, the physiochemical properties of biochar, as well as soil properties (e.g., soil organic carbon, minerals, microorganisms, pH, temperature, and moisture) and the crops and plants growing on soil, can have a decisive influence on biochar environmental behavior and its degree of aging.21,88,91,92 Therefore, the behavior of weathering is not universal across all biochar types and the effects of aging on the biochar properties need to be extensively explored, possibly through long term field experiments.93 Previous studies reported microbial decomposition and leaching of biochar DOC as the two predominant mechanisms that likely explain biochar C loss, with a C loss from biochar of the 0.5–1% year−1 by microbial degradation94,95 and of the 0.5% year−1 by leaching.94
Noteworthy is that no significant differences were found in the levels of stable fraction between the aged biochar and the fresh one, indicating that the biochar used in this study was relatively recalcitrant even under field conditions. Therefore, it seems reasonable to assume that after the relatively rapid mineralization of labile OC, the remaining ∼50% recalcitrant fraction of biochar (TGA data; Table 4) is not degradable on annual to decadal timescales and hence, only this proportion of total carbon in biochar provides long-term carbon storage. The persistence of OC biochar demonstrated by TGA and Py-GC-MS analysis supports the role played by biochar as a sustainable soil management and carbon sequestration technique, but potential losses due to SOC dynamics should be carefully evaluated especially in long-term field experiments where abiotic and biotic components drive the carbon stability rather than the inherent biochar recalcitrance.
In addition to the potential accumulation of the stable C in soil, the present study confirms that biochar also increases labile forms of soil C. When biochar is applied to soil, the labile C fractions evolved from biochar and can impact microbial activity, including the balance of indigenous labile pools, and therefore on the overall soil ecosystem.26,96 As summarized in Table 4, the amount of biochar labile fraction in fresh biochar was 26.1 ± 0.6%, and it decreased to an average of 9.1 ± 0.3% with aging. Therefore soil fertility can be improved thanks to the labile carbon loss from biochar.
Conclusions
The knowledge of the recalcitrance of carbon in biochar and the behavior of the labile C fraction are crucial issues for evaluating the agro-environmental impact of biochar. This work reported the first comprehensive and quantitative study exploring the multi-annual environmental behavior of biochar by Py-GC-MS and TG analysis of aged samples. It provides insights related to the effects of aging on the biochar properties applied to agricultural soils, particularly regarding the quantity and quality characteristics of labile and recalcitrant fractions after two years of field experiment. Biochar used in the field experiment contained two pools of OM, namely, labile and recalcitrant OM. Results highlighted the effects of aging on these two pools of OM. Specifically, comparing Py-GC-MS and TGA data, similar trends were observed for the % labile and recalcitrant fraction (TGA), and molecular ratios (Py-GC-MS) values. Results showed lower labile fraction (TGA) and increased aromaticity (Py-GC-MS) in aged biochar. This finding may explain the lower proportion of DOM released by aged biochar, but with a higher degree of aromaticity (UV-vis) in comparison to fresh biochar. In the case of aged biochar, results showed that the use of H/C and O/C ratios as stability indexes will not offer an estimation of the aged biochar real stability. Instead, the qualification of aged biochar C structures such as aromaticity or degree of aromatic condensation by Py-GC-MS analysis and the thermal quantification of the labile and recalcitrant C by TGA provide comprehensive detailed information on biochar behavior in soil. In summary, with regard to the objectives of this study, it is concluded that: (1) no changes in the stable fraction of biochar between the aged and fresh biochar; (2) significant decrease of the biochar labile fraction and of total C concentration in biochar from 26% to 9.1% and from 80% to 67%, respectively, during the aging process in soil; (3) usefulness of Py-GC-MS and TGA to shed light on the characteristics of biochar applied to soil systems, providing consistent information concerning the stability of biochar under real environmental conditions. Therefore, the present work increases the knowledge on the potential aging impacts on the physicochemical properties of biochar and on its connected environmental implications. However, more efforts are needed to better understand the causes of these biochar changes as well as their effects on biochar performance over time.
Author contributions
The manuscript was written through the contributions of all authors. All authors have approved the final version of the manuscript. Conception and design of study: A. G. R., N. G.; acquisition of data: A. G. R., N. G., A. B.; analysis and/or interpretation of data: A. G. R., N. G., D. F.; drafting the manuscript: A. G. R.; revising the manuscript critically for important intellectual content: A. G. R., N. G., D. F., A. B., C. T.; approval of the version of the manuscript to be published: A. G. R., N. G., D. F., A. F., C. T., R. P., C. C., E. B., D. M., D. Z., A. B.
Conflicts of interest
There are no conflicts to declare.
Acknowledgements
This research was funded by EIT Food program (Black to the Future Project, EIT-21217). This EIT Food activity has received funding from the European Institute of Innovation and Technology (EIT), a body of the European Union, under Horizon Europe, the EU Framework Programme for Research and Innovation.
References
- S. Donn, R. E. Wheatley, B. M. McKenzie, K. W. Loades and P. D. Hallett, Improved soil fertility from compost amendment increases root growth and reinforcement of surface soil on slopes, Ecol. Eng., 2014, 71, 458–465 CrossRef.
- S. A. A. Nakhli, M. Delkash, B. E. Bakhshayesh and H. Kazemian, Application of zeolites for sustainable agriculture: a review on water and nutrient retention, Water, Air, Soil Pollut., 2017, 228, 464 CrossRef.
- L. Alessandrino, N. Colombani, V. Aschonitis, A. L. Eusebi and M. Mastrocicco, Performance of graphene and traditional soil improvers in limiting nutrients and heavy metals leaching from a sandy Calcisol, Sci. Total Environ., 2023, 858, 159806 CrossRef CAS PubMed.
- D. Woolf, J. E. Amonette, F. A. Street-Perrott, J. Lehmann and S. Joseph, Sustainable biochar to mitigate global climate change, Nat. Commun., 2010, 1, 56 CrossRef PubMed.
- A. G. Rombolà, W. Meredith, C. E. Snape, S. Baronti, L. Genesio, F. P. Vaccari, F. Miglietta and D. Fabbri, Fate of Soil Organic Carbon and Polycyclic Aromatic Hydrocarbons in a Vineyard Soil Treated with Biochar, Environ. Sci. Technol., 2015, 49(18), 11037–11044 CrossRef PubMed.
-
J. Lehmann and S. Joseph, Biochar for Environmental Management: Science, Technology and Implementation, ed. J. Lehmann and S. Joseph, Taylor & Francis, London, United Kingdom, 2015, 1, pp. 1–13 Search PubMed.
- D. Woolf, J. Lehmann and D. R. Lee, Optimal bioenergy power generation for climate change mitigation with or without carbon sequestration, Nat. Commun., 2016, 7, 13160 CrossRef CAS PubMed.
- L. Wang, Y. S. Ok, D. C. W. Tsang, D. S. Alessi, J. Rinklebe, H. Wang, O. Mašek, R. Hou, D. O'Connor and D. Hou, New Trends in Biochar Pyrolysis and Modification Strategies: Feedstock, Pyrolysis Conditions, Sustainability Concerns and Implications for Soil Amendment, Soil Use Manage., 2020, 36, 1–29 CrossRef CAS.
- D. Lefebvre, A. Williams, J. Meersmans, G. J. Kirk, S. Sohi, P. Goglio and P. Smith, Modelling the potential for soil carbon sequestration using biochar from sugarcane residues in Brazil, Sci. Rep., 2020, 10(1), 1–11 CrossRef PubMed.
- K. N. Palansooriya, Y. S. Ok, Y. M. Awad, S. S. Lee, J. K. Sung, A. Koutsospyros and D. H. Moon, Impacts of biochar application on upland agriculture: a review, J. Environ. Manage., 2019, 234, 52–64 CrossRef CAS PubMed.
- M. Nematian, C. Keske and J. N. Ng’ombe, A techno-economic analysis of biochar production and the bioeconomy for orchard biomass, Waste Manage., 2021, 135, 467–477 CrossRef PubMed.
- M. H. Raza, M. Abid, T. Yan, S. Naqvi, S. Akhtar and M. Faisal, Understanding farmers' intentions to adopt sustainable crop residue management practices: a structural equation modeling approach, J. Cleaner Prod., 2019, 227, 613–623 CrossRef.
- N. Greggio, E. Balugani, C. Carlini, A. Contin, N. Labartino, R. Porcelli, M. Quaranta, S. Righi, L. Vogli and D. Marazza, Theoretical and unused potential for residual biomasses in the Emilia Romagna Region (Italy) through a revised and portable framework for their categorization, Renewable Sustainable Energy Rev., 2019, 112, 590–606 CrossRef.
- S. D. Joseph, M. Camps-Arbestain, Y. Lin, P. Munroe, C. H. Chia, J. Hook, L. van Zwieten, S. Kimber, A. Cowie, B. P. Singh, J. Lehmann, N. Foidl, R. J. Smernik and J. E. Amonette, An investigation into the reactions of biochar in soil, Soil Res., 2010, 48(7), 501–515 CrossRef CAS.
- K. Spokas, J. Novak, C. A. Masiello, M. G. Johnson, E. C. Colosky, J. A. Ippolito and C. Trigo, Physical disintegration of biochar: an overlooked process, Environ. Sci. Technol. Lett., 2014, 1, 326–332 CrossRef CAS.
- A. R. Zimmerman, Environ. Abiotic and microbial oxidation of laboratory-produced black carbon (biochar), Environ. Sci. Technol., 2010, 44, 1295–1301 CrossRef CAS PubMed.
- M. Farrell, T. K. Kuhn, L. M. Macdonald, T. M. Maddern, D. V. Murphy, P. A. Hall, B. P. Singh, E. S. Krull and J. A. Baldock, Microbial utilisation of biochar derived carbon, Sci. Total Environ., 2013, 465, 288–297 CrossRef CAS PubMed.
- Y. Kuzyakov, Y. Bogomolova and B. Glaser, Biochar stability in soil: decomposition during eight years and transformation as assessed by compound-specific 14C analysis, Soil Biol. Biochem., 2014, 70, 229–236 CrossRef CAS.
- G. Sorrenti, C. A. Masiello, B. Dugan and M. Toselli, Biochar physical-chemical properties as affected by environmental exposure, Sci. Total Environ., 2016, 563, 237–246 CrossRef PubMed.
- X. Dong, G. Li, Q. Lin and X. Zhao, Quantity and quality changes of biochar aged for 5 years in soil under field conditions, Catena, 2017, 159, 136–143 CrossRef CAS.
- Y. Fang, B. Singh, B. P. Singh and E. Krull, Biochar carbon stability in four contrasting soils, Eur. J. Soil Sci., 2014, 65, 60–71 CrossRef CAS.
- H. Zheng, D. Liu, X. Liao, Y. Miao, Y. Li, J. Li, J. J. Li, J. J. Yuan, Z. M. Chen and W. Ding, Field-aged biochar enhances soil organic carbon by increasing recalcitrant organic carbon fractions and making microbial communities more conducive to carbon sequestration, Agric., Ecosyst. Environ., 2022, 340, 108177 CrossRef CAS.
- B. P. Singh, A. L. Cowie and R. J. Smernik, Biochar carbon stability in a clayey soil as a function of feedstock and pyrolysis temperature, Environ. Sci. Technol., 2012, 46, 11770–11778 CrossRef CAS PubMed.
- R. Conti, A. G. Rombolà, A. Modelli, C. Torri and D. Fabbri, Evaluation of the thermal and environmental stability of switchgrass biochars by Py-GC-MS, J. Anal. Appl. Pyrolysis, 2014, 110(1), 239–247 CrossRef CAS.
- F. Braadbaart, I. Poole and A. A. Van Brussel, Preservation potential of charcoal in alkaline environments: an experimental approach and implications for the archaeological record, J. Archaeol. Sci., 2009, 36, 1672–1679 CrossRef.
- A. Cross and S. P. Sohi, The priming potential of biochar products in relation to labile carbon contents and soil organic matter status, Soil Biol. Biochem., 2011, 43, 2127–2134 CrossRef CAS.
- L. Leng, H. Huang, H. Li, J. Li and W. Zhou, Biochar stability assessment methods: a review, Sci. Total Environ., 2019, 647, 210–222 CrossRef CAS PubMed.
- L. Han, K. Sun, Y. Yang, X. Xia, F. Li, Z. Yang and B. Xing, Biochar's stability and effect on the content, composition and turnover of soil organic carbon, Geoderma, 2020, 364, 114184 CrossRef CAS.
- J. Wang, Z. Xiong and Y. Kuzyakov, Biochar stability in soil: meta-analysis of decomposition and priming effects, Glob. Change Biol. Bioenergy, 2016, 8, 512–523 CrossRef CAS.
- A. G. Rombolà, D. Fabbri, W. Meredith, C. E. Snape and A. Dieguez-Alonso, Molecular characterization of the thermally labile fraction of biochar by hydropyrolysis and pyrolysis-GC/MS, J. Anal. Appl. Pyrolysis, 2016, 121, 230–239 CrossRef.
- D. B. Wiedemeier, S. Abiven, W. C. Hockaday, M. Keiluweit, M. Kleber, C. A. Masiello, A. V. McBeath, P. S. Nico, L. A. Pyle, M. P. W. Schneider, R. J. Smernik, G. L. B. Wiesenberg and M. W. Schmidt, Aromaticity and degree of aromatic condensation of char, Org. Geochem., 2015, 78, 135–143 CrossRef CAS.
- A. McBeath, R. Smernik and E. Plant, Determination of the aromaticity and the degree of aromatic condensation of a thermosequence of wood charcoal using NMR, Org. Geochem., 2011, 42, 1194–1202 CrossRef CAS.
- O. Mašek, P. Brownsort, A. Cross and S. Sohi, Influence of production conditions on the yield and environmental stability of biochar, Fuel, 2013, 103, 151–155 CrossRef.
- R. Conti, D. Fabbri, I. Vassura and L. Ferroni, Comparison of chemical and physical indices of thermal stability of biochars from different biomass by analytical pyrolysis and thermogravimetry, J. Anal. Appl. Pyrolysis, 2016, 122, 160–168 CrossRef CAS.
- R. Zornoza, F. Moreno-Barriga, J. A. Acosta, M. A. Muñoz and A. Faz, Stability, nutrient availability and hydrophobicity of biochars derived from manure, crop residues, and municipal solid waste for their use as soil amendments, Chemosphere, 2016, 144, 122–130 CrossRef CAS PubMed.
- J. Kaal, M. P. W. Schneider and M. W. I. Schmidt, Rapid molecular screening of black carbon (biochar) thermosequences obtained from chestnut wood and rice straw: a pyrolysis-GC/MS study, Biomass Bioenergy, 2012, 45, 115–129 CrossRef CAS.
- A. V. McBeath, C. M. Wurster and M. I. Bird, Influence of feedstock properties and pyrolysis conditions on biochar carbon stability as determined by hydrogen pyrolysis, Biomass Bioenergy, 2015, 73, 155–173 CrossRef CAS.
- R. C. Pereira, J. Kaal, M. C. Arbestain, R. P. Lorenzo, W. Aitkenhead, M. Hedley and J. A. Maciá-Agulló, Contribution to characterisation of biochar to estimate the labile fraction of carbon, Org. Geochem., 2011, 42, 1331–1342 CrossRef.
- L. Wang, C. Gao, K. Yang, Y. Sheng, J. Xu, Y. Zhao, J. Lou, R. Sun and L. Zhu, Effects of biochar aging in the soil on its mechanical property and performance for soil CO2 and N2O emissions, Sci. Total Environ., 2021, 782, 146824 CrossRef CAS PubMed.
-
IUSS Working Group WRB, World Reference Base for Soil Resources. International Soil Classification System for Naming Soils and Creating Legends for Soil Maps, 4th Edition, International Union of Soil Sciences (IUSS), Vienna, Austria, 2022 Search PubMed.
-
D.M. 13/09/99. Gazzetta Ufficiale – DECRETO MINISTERIALE 13 settembre 1999, https://www.gazzettaufficiale.it/eli/id/1999/10/21/099A8497/sg Accessed March 2023.
- S. Baronti, F. P. Vaccaria, F. Miglietta, C. Calzolari, E. Lugato, S. Orlandini, R. Pini, C. Zulian and L. Genesio, Impact of biochar application on plant water relations in Vitis vinifera (L.), Eur. J. Agron., 2014, 53, 38–44 CrossRef CAS.
- A. Maienza, S. Baronti, A. Cincinelli, T. Martellini, A. Grisolia, F. Miglietta, G. Renella, S. R. Stazi, F. P. Vaccari and L. Genesio, Biochar improves the fertility of a Mediterranean vineyard without toxic impact on the microbial community, Agron. Sustainable Dev., 2017, 37, 47 CrossRef.
- A. G. Rombolà, C. Torri, I. Vassura, E. Venturini, R. Reggiani and D. Fabbri, Effect of biochar amendment on organic matter and dissolved organic matter composition of agricultural soils from a two-year field experiment, Sci. Total Environ., 2022, 812, 151422 CrossRef PubMed.
- G. N. Kasozi, P. Nkedi-Kizza and W. G. Harris, Varied Carbon Content of Organic Matter in Histosols, Spodosols, and Carbonatic Soils, Soil Sci. Soc. Am. J., 2009, 73, 1313–1318 CrossRef CAS.
- M. Ghidotti, D. Fabbri, O. Mašek, C. L. Mackay, M. Montalti and A. Hornung, Source and Biological Response of Biochar Organic Compounds Released into Water; Relationships with Bio-Oil Composition and Carbonization Degree, Environ. Sci. Technol., 2017, 51(11), 6580–6589 CrossRef CAS PubMed.
- G. Li, S. Khan, M. Ibrahim, T. R. Sun, J. F. Tang, J. B. Cotner and Y. Y. Xu, Biochars induced modification of dissolved organic matter (DOM) in soil and its impact on mobility and bioaccumulation of arsenic and cadmium, J. Hazard. Mater., 2018, 348, 100–108 CrossRef CAS PubMed.
- H. P. Schmidt, C. Kammann, N. Hagemann, J. Leifeld, T. D. Bucheli, A. Sánchez Monedero and M. L. Cayuela, Biochar in agriculture – A systematic review of 26 global meta-analyses, GCB Bioenergy, 2021, 13(11), 1708–1730 CrossRef CAS.
- K. Crombie, O. Masek, S. P. Sohi, P. Brownsort and A. Cross, The effect of pyrolysis conditions on biochar stability as determined by three methods, Glob. Change Biol. Bioenergy, 2013, 5, 122–131 CrossRef CAS.
- L. Leng and H. Huang, An overview of the effect of pyrolysis process parameters on biochar stability, Bioresour. Technol., 2018, 270, 627–642 CrossRef CAS PubMed.
- C. H. Cheng, J. Lehmann, J. E. Thies, S. D. Burton and M. H. Engelhard, Oxidation of black carbon through biotic and abiotic processes, Org. Geochem., 2006, 37, 1477–1488 CrossRef CAS.
- M. J. Norwood, P. Louchouarn, L. J. Kuo and O. R. Harvey, Characterization and biodegradation of water-soluble biomarkers and organic carbon extracted from low temperature chars, Org. Geochem., 2013, 56, 111–119 CrossRef CAS.
- A. Mukherjee and A. R. Zimmerman, Organic carbon and nutrient release from a range of laboratory-produced biochars and biochar–soil mixtures, Geoderma, 2013, 193, 122–130 CrossRef.
- K. A. Spokas, Impact of biochar field aging on laboratory greenhouse gas production potentials, GCB Bioenergy, 2013, 5(2), 165–176 CrossRef CAS.
- J. M. de la Rosa, M. Rosado, M. Paneque, A. Z. Miller and H. Knicker, Effects of ageing under field conditions on biochar structure and composition: implications for biochar stability in soils, Sci. Total Environ., 2018, 613, 969–976 CrossRef PubMed.
- N. Hagemann, S. Joseph, H.-P. Schmidt, C. I. Kammann, J. Harter, T. Borch, R. B. Young, K. Varga, S. Taherymoosavi, K. W. Elliott, A. McKenna, M. Albu, C. Mayrhofer, M. Obst, P. Conte, A. Dieguez-Alonso, S. Orsetti, E. Subdiaga, S. Behrens and A. Kappler, Organic coating on biochar explains its nutrient retention and stimulation of soil fertility, Nat. Commun., 2017, 8(1), 1089 CrossRef PubMed.
- S. Joseph, C. I. Kammann, J. G. Shepherd, P. Conte, H.-P. Schmidt, N. Hagemann, A. M. Rich, C. E. Marjo, J. Allen, P. Munroe, D. R. G. Mitchell, S. Donne, K. Spokas and E. R. Graber, Microstructural and associated chemical changes during the composting of a high temperature biochar: mechanisms for nitrate, phosphate and other nutrient retention and release, Sci. Total Environ., 2018, 618, 1210–1223 CrossRef CAS PubMed.
- P. Campos, H. Knicker, A. Z. Miller, M. Velasco-Molina and J. M. de la Rosa, Biochar ageing in polluted soils and trace elements immobilisation in a 2-year field experiment, Environ. Pollut., 2021, 290, 118025 CrossRef CAS PubMed.
- C. H. Cheng, J. Lehmann and M. H. Engelhard, Natural oxidation of black carbon in soils: changes in molecular form and surface charge along a climosequence, Geochim. Cosmochim. Acta, 2008, 72, 1598–1610 CrossRef CAS.
- Q. Fan, L. Cui, G. Quan, S. Wang, J. Sun, X. Han, J. Wang and J. Yan, Effects of wet oxidation process on biochar surface in acid and alkaline soil environments, Materials, 2018, 11, 2362 CrossRef CAS PubMed.
- H. Wang, M. Feng, F. Zhou, X. Huang, D. C. W. Tsang and W. Zhang, Effects of atmospheric
ageing under different temperatures on surface properties of sludge-derived biochar and metal/metalloid stabilization, Chemosphere, 2017, 184, 176–184 CrossRef CAS PubMed.
- M. Feng, W. Zhang, X. Wu, Y. Jia, C. Jiang, H. Wei, R. Qiu and D. C. W. Tsang, Continuous leaching modifies the surface properties and metal(loid) sorption of sludge-derived biochar, Sci. Total Environ., 2018, 625, 731–737 CrossRef CAS PubMed.
- W.-Y. Xia, Y.-J. Du, F.-S. Li, G.-L. Guo, X.-L. Yan, C.-P. Li, A. Arulrajah, F. Wang and S. Wang, Field evaluation of a new hydroxyapatite based binder for ex-situ solidification/stabilization of a heavy metal contaminated site soil around a Pb-Zn smelter, Constr. Build. Mater., 2019, 210, 278–288 CrossRef CAS.
- J. Wang, X. Pan, Y. Liu, X. Zhang and Z. Xiong, Effects of biochar amendment in two soils on greenhouse gas emissions and crop production, Plant Soil, 2012, 360, 287–298 CrossRef CAS.
- Z. Huang, L. Hu and J. Dai, Effects of ageing on the surface characteristics and cu (II) adsorption behaviour of rice husk BC in soil, Open Chem., 2020, 18, 1421–1432 CAS.
- C. H. Cheng and J. Lehmann, Ageing of black carbon along a temperature gradient, Chemosphere, 2009, 75, 1021–1027 CrossRef CAS PubMed.
- F. X. Yao, M. C. Arbestain, S. Virgel, F. Blanco, J. Arostegui, J. A. Maciá-Agulló and F. Macías, Simulated geochemical weathering of a mineral ash-rich biochar in a modified Soxhlet reactor, Chemosphere, 2010, 80(7), 724–732 CrossRef CAS PubMed.
- S. Ghysels, D. Rathnayake, P. Maziarka, O. Mašek, S. Sohi and F. Ronsse, Biochar stability scores from analytical pyrolysis (Py-GC-MS), J. Anal. Appl. Pyrolysis, 2022, 161, 105412 CrossRef CAS.
- D. Fabbri, C. Torri and K. A. Spokas, Analytical pyrolysis of synthetic chars derived from biomass with potential agronomic application (biochar). Relationship with impacts on microbial carbon dioxide production, J. Anal. Appl. Pyrolysis, 2012, 93, 77–84 CrossRef CAS.
- J. Kaal, A. M. Cortizas, O. Reyes and M. Solino, Molecular characterization of Ulex europaeus biochar obtained from laboratory heat treatment experiment – a pyrolysis-GC/MS study, J. Anal. Appl. Pyrolysis, 2012, 95, 205–212 CrossRef CAS.
- J. Kaal and C. Rumpel, Can pyrolysis-GC/MS be used to estimate the degree of thermal alteration of black carbon?, Org. Geochem., 2009, 40, 1179–1187 CrossRef CAS.
- R. Pallasser, B. Minasny and A. B. McBratney, Soil carbon determination by thermogravimetrics, Peerj, 2013, 1, e6 CrossRef PubMed.
- Y. Kuzyakov, I. Subbotina, H. Chen, I. Bogomolova and X. Xu, Black carbon decomposition and incorporation into soil microbial biomass estimated by 14C labeling, Soil Biol. Biochem., 2009, 41, 210–219 CrossRef CAS.
- N. P. Gurwick, L. A. Moore, C. Kelly and P. Elias, A systematic review of biochar research: with a focus on its stability in situ and its promise as a climate mitigation strategy, PLoS One, 2013, 8(9), e75932 CrossRef CAS PubMed.
- X. Y. Yang, K. H. Chang, Y. J. Kim, J. Zhang and G. Yoo, Effects of different biochar amendments on carbon loss and leachate characterization from an agricultural soil, Chemosphere, 2019, 226, 625–635 CrossRef CAS PubMed.
- C. Liu, H. Wang, P. Li, Q. Xian and X. Tang, Biochar's impact on dissolved organic matter (DOM) export from a cropland soil during natural rainfalls, Sci. Total Environ., 2019, 650, 1988–1995 CrossRef CAS PubMed.
- M. Huang, Z. Li, N. Luo, R. Yang, J. Wen, B. Huang and G. Zeng, Application potential of biochar in environment: insight from degradation of biochar-derived DOM and complexation of DOM with heavy metals, Sci. Total Environ., 2019, 646, 220–228 CrossRef CAS PubMed.
- H.-B. Kim, J.-G. Kim, T. Kim, D. S. Alessi and K. Baek, Interaction of biochar stability and abiotic aging: influences of pyrolysis reaction medium and temperature, Chem. Eng. J., 2021, 411, 128441 CrossRef CAS.
- T. Jamieson, E. Sager and C. Guéguen, Characterization of biochar-derived dissolved organic matter using UV-visible absorption and excitation–emission fluorescence spectroscopies, Chemosphere, 2014, 103, 197–204 CrossRef CAS PubMed.
- P. Zhang, P. Huang, X. Xu, H. Sun, B. Jiang and Y. Liao, Spectroscopic and molecular characterization of biochar-derived dissolved organic matter and the associations with soil microbial responses, Sci. Total Environ., 2020, 708, 134619 CrossRef CAS PubMed.
- J. Weishaar, G. Aiken, B. Bergamaschi, M. Fram, R. Fugii and K. Mopper, Evaluation of specific ultraviolet absorbance as an indicator of the chemical composition and reactivity of dissolved organic carbon, Environ. Sci. Technol., 2003, 37, 4702–4708 CrossRef CAS PubMed.
- T. Karanfil, M. A. Schlautman and I. Erdogan, Survey of DOC and UV measurement practices with implications for SUVA determination, J. - Am. Water Works Assoc., 2002, 94, 68–80 CrossRef CAS.
- A. Selberg, M. Viik, K. Ehapalu and T. Tenno, Content and composition of natural organic matter in water of Lake Pitkjärv and mire feeding Kuke River (Estonia), J. Hydrol., 2011, 400, 274–280 CrossRef.
- C. He, X. He, J. Li, Y. Luo, J. Li, Y. Pei and J. Jiang, The spectral characteristics of biochar-derived dissolved organic matter at different pyrolysis temperatures, J. Environ. Chem. Eng., 2021, 9, 106075 CrossRef CAS.
- J. Hur, B.-M. Lee and H.-S. Shin, Microbial degradation of dissolved organic matter (DOM) and its influence on phenanthrene–DOM interactions, Chemosphere, 2011, 85, 1360–1367 CrossRef CAS PubMed.
- A. Smebye, V. Alling, R. D. Vogt, T. C. Gadmar, J. Mulder, G. Cornelissen and S. E. Hale, Biochar amendment to soil changes dissolved organic matter content and composition, Chemosphere, 2016, 142, 100–105 CrossRef CAS PubMed.
- Z. Feng, Z. Fan, H. Song, K. Li, H. Lu, Y. Liu and F. Cheng, Biochar induced changes of soil dissolved organic matter: the release and adsorption of dissolved organic matter by biochar and soil, Sci. Total Environ., 2021, 783, 147091 CrossRef CAS PubMed.
-
A. R. Zimmerman and B. Gao, in Biochar and Soil Biota, ed. N. Ladygina and F. Rineau, CRC Press, Taylor & Francis Group, USA, 2013, 1, pp. 1–40 Search PubMed.
- J. Yin, L. Zhao, X. Xu, D. Li, H. Qiu and X. Cao, Evaluation of long-term carbon sequestration of biochar in soil with biogeochemical field model, Sci. Total Environ., 2022, 822, 153576 CrossRef CAS PubMed.
- C. J. Ennis, A. G. Evans, M. Islam, T. K. Ralebitso-Senior and E. Senior, Biochar: carbon sequestration, land remediation, and impacts on soil microbiology, Crit. Rev. Environ. Sci. Technol., 2012, 42, 2311–2364 CrossRef CAS.
- Y. Liu and J. Chen, Effect of ageing on biochar properties and pollutant management, Chemosphere, 2022, 292, 133427 CrossRef CAS PubMed.
- J. Lehmann and M. Kleber, The contentious nature of soil organic matter, Nature, 2015, 528, 60–68 CrossRef CAS PubMed.
- D. Marazza, S. Pesce, N. Greggio, F. P. Vaccari, E. Balugani and A. Buscaroli, The Long-Term Experiment Platform for the Study of Agronomical and Environmental Effects of the Biochar: Methodological Framework, Agriculture, 2022, 12(8), 1244 CrossRef CAS.
- J. Major, J. Lehmann, M. Rondon and C. Goodale, Fate of soil-applied black carbon: downward migration, leaching and soil respiration, Global Change Biol., 2010, 16, 1366–1379 CrossRef.
- B. Maestrini, S. Abiven, N. Singh, J. Bird, M. S. Torn and M. W. Schmidt, Carbon losses from pyrolysed and original wood in a forest soil under natural and increased N deposition, Biogeosciences, 2014, 11, 5199–5213 CrossRef.
- E. W. Bruun, H. Hauggaard-Nielsen, N. Ibrahim, H. Egsgaard, P. Ambus, P. A. Jensen and K. Dam-Johansen, Influence of fast pyrolysis temperature on biochar labile fraction and short-term carbon loss in a loamy soil, Biomass Bioenergy, 2011, 35, 1182–1189 CrossRef CAS.
|
This journal is © The Royal Society of Chemistry 2023 |