DOI:
10.1039/D2BM01595A
(Review Article)
Biomater. Sci., 2023,
11, 2620-2638
Multivalent sialic acid materials for biomedical applications
Received
1st October 2022
, Accepted 29th November 2022
First published on 1st December 2022
Abstract
Sialic acid is a kind of monosaccharide expressed on the non-reducing end of glycoproteins or glycolipids. It acts as a signal molecule combining with its natural receptors such as selectins and siglecs (sialic acid-binding immunoglobulin-like lectins) in intercellular interactions like immunological surveillance and leukocyte infiltration. The last few decades have witnessed the exploration of the roles that sialic acid plays in different physiological and pathological processes and the use of sialic acid-modified materials as therapeutics for related diseases like immune dysregulation and virus infection. In this review, we will briefly introduce the biomedical function of sialic acids in organisms and the utilization of multivalent sialic acid materials for targeted drug delivery as well as therapeutic applications including anti-inflammation and anti-virus.
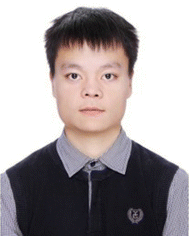 Yusong Cao | Yusong Cao is studying for a Ph.D. degree under the guidance of Prof. Xuesi Chen, at the Changchun Institute of Applied Chemistry (CIAC), Chinese Academy of Sciences (CAS). He obtained his B.S. degree from Zhejiang University in polymer science and engineering in 2016. His current research focuses on the design and synthesis of biomaterials for immune regulation. |
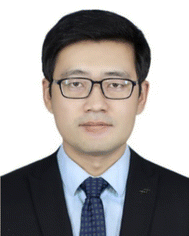 Wantong Song | Dr Wantong Song is a full professor at the Changchun Institute of Applied Chemistry (CIAC), Chinese Academy of Sciences (CAS). He obtained his B.S. degree from Nanjing University in 2008, and Ph.D. degree from the University of Chinese Academy of Sciences in 2013, and he then started his research career at CIAC. He undertook postdoctoral training by working with Prof. Leaf Huang at the University of North Carolina at Chapel Hill from 2016 to 2018. Dr Song's research focuses on developing innovative polymeric carriers for drug, gene and vaccine delivery. He has published more than 70 papers in Nat. Nanotechnol., Nat. Commun., Adv. Mater., etc., and applied for more than 20 patents. |
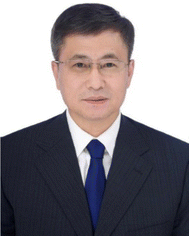 Xuesi Chen | Prof. Xuesi Chen was born in 1959 in Changchun, China. He got his bachelor's degree from the Department of Chemistry at Jilin University in 1982, master's degree from Changchun Institute of Applied Chemistry (CIAC), Chinese Academy of Sciences (CAS) in 1988, and doctor's degree from Waseda University in 1997 (supervised by Hiroyuki Nishide). After postdoctoral study at the University of Pennsylvania, he joined the faculty at CIAC as a professor in 1999. Prof. Chen's research interests include the synthesis of Schiff base catalysts for ring-opening polymerization of lactides, preparation of biodegradable polymers for biomedical applications such as bone fracture repair, drug and gene carriers, and hydrogels, and industrialization of polylactide (PLA) as a green plastic. |
Introduction
Apart from nucleic acids, proteins, and lipids, almost half of the ‘building blocks of life’ are glycans – sugar chains composed of various monosaccharides.1 Glycans cover the membrane of every living cell and possess a staggering structural variety (the glycome), greatly exceeding the diversity of the genome and proteome. Carbohydrates or glycans are indispensable components of the cell surface. They play crucial biological and structural roles including establishing protective physical barriers against the outside environment, participating in cell–cell and cell–extracellular matrix interactions and modulating intracellular signaling.2
One family of monosaccharides that stands out from other glycan components are the sialic acids.3 Since it was first defined by Landsteiner4 and Walz5 simultaneously, more than 50 natural derivates have been identified, mainly containing N-acetylneuraminic acid (Neu5Ac, also called sialic acid without specific demonstration), N-glycolylneuraminic acid (Neu5Gc) and deaminoneuraminic acid (KDN). This nine-carbon α-keto aldonic acid monosaccharide family is usually found at the non-reducing end of cell-surface glycoproteins or glycosphingolipids.6 The structural diversity of sialic acids and their derivatives and the ways of linking them with other monosaccharides determine the diversity of their roles in physiological and pathological processes.7 Sialic acids often act as a signal molecule for self-recognition to protect the cell surface and glycoproteins from proteases,8 regulate the serum half-life of glycoproteins and erythrocytes that are cleared in the liver upon desialylation,9–11 and promote the formation of the blood vessel lumen.12 In the immune system, sialoglycans such as sialyl Lewis x contribute to immune cell trafficking via binding to selectins on the endothelium,13 and they also act as ligands of the immunomodulatory Siglec (Sialic acid-binding Immunoglobulin-like lectins) receptors that set the threshold for immune activation.14,15 However, the Siglecs could also be utilized by pathogens as binding sites to enter into cells through sialoglycan–Siglec interactions.16,17 Furthermore, aberrant sialoglycan expressed on tumor cells could help tumor cell immune evasion and metastasis.18,19
Tremendous efforts have been made to obtain insight into what is happening during these processes, and the multivalent interactions between sialoglycans and the protein receptors have been proven to be the culprit.20,21 Studies in these fields have enabled scientists to develop materials containing multivalent sialic acids for modulating these physiological and pathological processes that could potentially be used as novel treatments for autoimmune disease, infectious disease and cancer. However, few reviews have been published in the past few decades that systematically introduce the roles of sialic acid in physiological and pathological processes, and especially describe using sialic acid materials in treatments for relevant diseases. Hence, in this review, we aim to give a brief introduction of sialic acid and its physiological and pathological roles in immune regulation, tumor metastasis and infectious disease, and then focus on reporting some excellent pioneer studies on materials containing multivalent sialic acids for regulating these processes and diseases.
The role of sialic acid and its receptors in biological processes
Sialic acids play important roles in many physiological processes including cell–cell interactions, immune surveillance and nerve development as well as pathological processes such as pathogen infection, autoimmunity and cancer.22 Sialic acids exert their functions in biology through interaction with multiple sialic acid-binding receptors such as selectins, Siglecs, and proteins of pathogens like viruses. In this part, we will give a brief introduction of the complicated roles and functions of sialic acid via interaction with different receptors including selectins, siglecs and virus proteins.
Siglecs and their roles in immune regulation
It was in 1998 when the name “siglecs”, short for “sialic-acid-binding immunoglobulin-like lectins”, was used to describe a subset of I-type lectins within the immunoglobulin gene superfamily that specifically bind sialylated glycans and have highly similar structural motifs within their N-terminal and C-2 set domains for the first time.23 Since sialic acids are expressed by all mammalian cells, siglecs can help the immune system in distinguishing between self and non-self signals. Recognition of their sialylated ligands by the N-terminal variable (V)-Ig like domain triggers cell signalling through their regulatory motifs in their cytoplasmic domains. In this immunoglobulin-like lectin family, 15 members in humans and 9 members in mice have been found up to now. According to their structural similarity and evolutionary conservation, they are classified into two subsets. Conserved Siglecs include Siglec-1 (also known as sialoadhesin and CD169), Siglec-2 (also known as CD22), Siglec-4 (also known as myelin-associated glycoprotein, MAG) and the recently discovered Siglec-15 (which has a different structure from the other siglecs, with ∼25–30% sequence identity). These four members and their orthologues exist in almost all mammalian species examined. Another group of siglecs containing Siglec-3, Siglec-5 to Siglec-12, Siglec-14 and Siglec-16 are named CD33-related Siglecs since they share ∼50–99% identity.24 However, this group of siglecs seem to be evolving rapidly by multiple processes, including gene duplication, exon shuffling, and exon loss, resulting in important diversity in the sequence structures and functions among mammalian species.
Although Siglec-4 expresses on oligodendrocytes and Schwann cells, and Siglec-6 expresses on placental trophoblasts, most of the siglecs are expressed on the surface of immune cells and act as cell function regulators in the innate and adaptive immune systems through glycan recognition.25 Some siglecs only express on specific species of immune cells. For instance, Siglec-1 is highly restrictedly expressed on subsets of resident tissue and inflammatory macrophages and activated monocytes.26 Siglec-2 only expresses on immature B cells and its expression decays during B cell maturation and differentiation into plasma cells.27 Siglec-8 expresses on eosinophils in a highly cell-type-restricted manner, and also expresses on basophils with relatively weak expression. Although there is no ortholog of Siglec-8 in mice, it was found that Siglec-F could act as a functional paralog due to its restricted expression on eosinophils.28 Besides, Siglec-9 is primarily expressed on monocytes, neutrophils and dendritic cells; Siglec-15 is mainly expressed on osteoclasts and as a potential therapeutic target for osteoporosis.29Fig. 1 shows a schematic diagram of the structures and expression of different kinds of siglecs that have been detected.
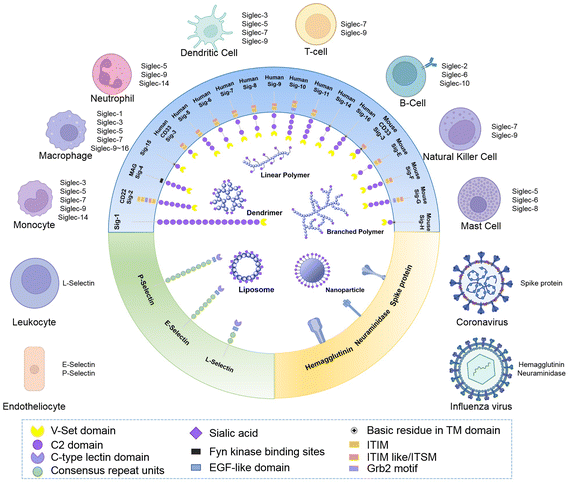 |
| Fig. 1 Receptors of sialic acid include siglecs, selectins and virus proteins. A variety of materials containing sialic acids have been developed to combine with these receptors to achieve the goal of immune modulation, targeted drug delivery and anti-virus treatments. The extracellular domain of siglecs often contains of a V-set domain for combining with sialic acid (except for Siglec-12 whose V-set domain mutated and lost this function) and several C2 domains. For most siglecs, these regulatory motifs are composed of immunoreceptor tyrosine-base inhibitory motifs (ITIMs), which serve to recruit phosphatases to phosphorylate downstream signaling molecules and inhibit cell activation. Some have other intracellular regulatory motifs like the immunoreceptor tyrosine switch motif (ITSM); growth factor receptor-bound 2 motif (Grb 2); Fyn kinase binding site. Some have positively charged amino acids in the transmembrane (TM) domain that associate with activating adaptors. The selectins often contain a sialic acid combining C-type lectin domain and EGF-like domain, which is an evolutionary conserved protein domain and derives its name from the epidermal growth factor. Several coronavirus adhere to host cells via the sialic acid binding sites on their spike proteins, and the hemagglutinin on the influenza virus has as a similar role during the infection process. The schematic image was created with BioRender.com. | |
Siglecs expressed on immune cells are immune regulatory molecules. Different siglecs have different preferences in terms of the structure of sialylated glycans. With the development of molecular biology and analytical methods, more and more structures of sialic acid and its derivatives as ligands of siglecs have been identified. However, no matter how the DNA encoding of siglecs changes, there is one consensus that sialic acid is bound to a shallow pocket in the N-terminal immunoglobulin domain of the Siglec, mainly via a salt bridge formed by the negatively charged carboxyl group of sialic acid and a conserved essential arginine of the siglecs. Siglec-2 to 12 carry immunoreceptor tyrosine-based inhibitory motifs (ITIMs). The prototype ITIM contains a 6-amino acid sequence of (Ile/Val/Leu/Ser)-X-Tyr-X-X-(Leu/Val), where X denotes any amino acid. Ligand induced clustering of these inhibitory receptors results in tyrosine phosphorylation, often by an Src family kinase, which provides a docking site for the recruitment of cytoplasmic phosphatases having an Src homology 2(SH2) domain. Two classes of SH2-containing inhibitory signaling effector molecules have been identified: the tyrosine phosphatase SHP-1 and the inositol phosphatase SHIP (SH2-containing inositol polyphosphate 5-phosphatase).30 These phosphatases could phosphorylate the signal molecules generated by toll-like receptors (TLRs) and the B cell receptor and other receptors and block the activating cascades.31 However, Siglec-12 lost its sialic acid-binding capacity and Siglec-13 and Siglec-17 have been inactivated during human evolution and so are not discussed in this review.15,32 In contrast, Siglec-14, -15, and 16 have a positive charge in their transmembrane domains that mediates association with DAP12 to generate active immune responses. Siglec-1 has no tyrosine-based signalling motifs in its intracellular domain so it is thought to play a role in cell adhesion and endocytosis instead of cell signalling.
Normally, siglecs on an immune cell surface bind to a glycoprotein or glycolipid ending with sialic acid on the same cell in a “cis” fashion. This kind of interaction aims to establish immune tolerance and increase the activation threshold of immune cells. Some autoimmune diseases arise due to the disruption of “cis” binding. For example, when Siglec-8 on mast cells and eosinophils loses its binding to sialic acid, systemic inflammation and allergic reactions may happen.33 Correspondingly, “trans” binding refers to siglecs binding to sialic acids on other cells and regulating immune response during the inflammation stage to prevent excessive activation of immune cells and sepsis.34
Since these siglecs on immune cells can downregulate the activity of immune cells, tumor cells have developed a strategy to escape from immune surveillance by upregulating the expression of sialic acid residues. It has been proven that these sialic acids over-expressed by tumor cells could bind specifically to Siglec-7 and Siglec-9 on natural killer (NK) cells to suppress their cytotoxicity,35 and at the same time inhibit neutrophil activation.36 The interaction between sialic acids expressed by tumor cells and Siglec-7 and Siglec-9 on monocycles contributes to the differentiation of monocytes into macrophages with an immune-suppressive phenotype.37 Sialic acid containing CD24 on tumor cells interacts with siglec-10 on tumor-associated macrophages to help tumor cells to escape from immunological surveillance.38 T cells expressing Siglec-9 and Siglec-15 could also be suppressed by interaction with sialic acids on tumor cells.39–41 Focusing on the immune inhibitory function of sialic acids and siglecs offers potential for developing novel therapeutic means for cancer immunotherapy and anti-tumor drug delivery strategies. Ding and coworkers have been working on anti-tumor nanodrug delivery systems for many years42–44 and have made pioneering progress targeting the overexpressed sialic acid on the surface of tumor cells for drug delivery.45
Selectin, cell–cell interaction and cell adhesion
Selectins are another category of sialic acid binding proteins. Selectins are a family of calcium-dependent (C-type) lectins that play important roles in mediating immune cell adhesion to the endothelium and promoting entry to secondary lymphoid organs and sites of inflammation.46 Early in 1978, Görög and his coworkers found that removing sialic acids on the cell surface resulted in activation of aggravated neutrophil adhesion and inflammation.47 However, at that time, when characterization methods were scarce, they knew little about the existence of the selectin protein, nor that the underlying mechanism of this phenomenon was the interaction between sialic acids and selectins leading to the adhesion of neutrophils.
There are three members in the selectin family, which are named after their expression patterns: P-selectin refers to those expressed on platelets, E-selectin represents those expressed on endothelial cells and L-selectin is used to name those expressed on leukocytes (Fig. 1). These three types of selectins also have different preferences in biological activities and ligands. P-selectin was originally found to be expressed by activated platelets. Monocytes and neutrophils also express P-selectin on their surface. The function of P-selectin is to mediate the rolling of granulocytes and monocytes on the surface of endothelial cells and the adhesion of granulocytes and monocytes to platelets.48 L-selectin is fundamentally expressed on all circulating leukocytes and may shed the cell surface while circulating. E-selectin is mainly found on endothelial cells in postcapillary venules of the bone marrow and skin. It is also expressed on the endothelium in other organs, which is exposed to pro-inflammatory stimuli such as endogenic tumor necrosis factor (TNF), interleukin 1β (IL-1β) and ectogenic lipopolysaccharide (LPS).
Among the best described ligands for the selectins are tetrasaccharides and their derivatives, which include fucose and sialic acids, such as sialyl Lewis x (sLeX, Neu5Acα-2–3-Galβ-1–4(Fucα-1–3) GlcNAc) and sialyl Lewis a (sLea, Neu5Acα-2–3-Galβ-1–3(Fucα1–4) GlcNAc).49 The conditions in which these glycans are expressed determine their affinity with different selectins. For example, the primary ligand for P-selectin is a sLeX
50,51 decorated homodimeric mucin called P-selectin glycoprotein ligand 1 (PSGL1), however P-selectin could also bind with sulfatides, non-sialylated glycosphingolipids52 and CD2453 with high-affinity. L-selectin prefers to bind to glycoproteins, such as CD34, glycosylation-dependent cell adhesion molecule 1 (GlyCAM1), mucosal addressing cell adhesion molecule 1 (MAdCAM1) and PSGL1, which contain sulfated sLeX incorporated into N-linked and O-linked glycans.54,55
Under normal physiological conditions, selectins play critical roles in mediating leukocyte circulation through secondary lymphoid tissues and recruitment to inflammatory sites. In the earliest stage of leukocyte extravasation,56 cells flowing through the vascular compartment are captured by selectin–ligand interactions and begin rolling on endothelial cells. At secondary lymphoid tissues, L-selectin expressed by leukocytes binds to glycoproteins displayed by endothelial cells in the high endothelial venule to promote extravasation. The recruitment of leukocytes to inflammatory sites also depends on the upregulated expression of E-selectin on endothelial cells there and P-selectin on platelets. The L-selectin expressed by the gathering immune cells themselves amplifies the recruitment and adhesion of more leukocytes. Therefore, upregulated expression of selectins or their ligands in specific sites directs leukocytes to particular locations: through the combinatorial expression of particular selectins and their ligands, leukocyte subsets can be specifically recruited to different tissues and organs in the body.46
Spike protein of coronavirus, hemagglutinin on the influenza virus and their roles in infection processes
As well as playing an important role in regulating immune interaction, in the field of infectious diseases, sialic acid may also play a role in helping viruses to enter into cells. In the field of virology, sialic acids were the first identified virus receptors that play an essential role in post-attachment events in the entry processes.57 In the process of viruses infecting host cells, sialic acid on the cell surface plays the role of a double-edged sword. Since sialic acid is widely expressed on the cytomembrane, a variety of viruses specifically bind to host sialic acids as primary receptors for cell infection. Meanwhile, the host, normally vertebrates, has developed a trap via equipping mucus with abundant sialic acids to protect cells from being infected.58,59 However, the viruses have evolved to be selective towards specific interactions with particular sialic acid forms and linkages on different hosts and tissues.
The preference of a ligand depends on the structure of the virus’ protein receptors, whose expression depends on the species of virus. The influenza virus binds to the cell surface mainly via hemagglutinin (HA), and it is HA that determines the preference of differently structural ligands.60,61 As for coronavirus, for example OC43, HKU1,62 Middle East respiratory syndrome coronavirus (MERS-CoV)63 and severe acute respiratory syndrome coronavirus 2 (SARS-CoV-2),64 the sialic acid binding sets are often contained in the receptor-binding domain (RBD) of the spike (S) protein domain A. Other viruses such as mumps virus, also binds α-2,3-linked sialic acid as the leading factor of infection. For some viruses like Zika virus, however, recognition of sialic acid facilitates internalization instead of binding, however the mechanism is still unknown.65 Due to the diversity of viruses and their viral capsid protein, details will be discussed in the following part together with anti-virus materials.
The use of sialic acid by viruses to infect host cells is not alone among pathogens. Many bacteria and protozoan also use sialic acid on the surface of host cells as the target of infection and camouflage themselves by ingesting the sialic acid to evade the immune surveillance of the host.66 The ability of V. cholerae to take up sialic acids from the host promotes its intestinal colonization.67 Other bacteria, for example, enterohemorrhagic Escherichia coli, Haemophilus influenzae, Haemophilus ducreyi, Neisseria gonorrhoeae and Neisseria meningitidis, coat their surfaces with sialic acid to protect their antigens from the host immune system.68 Besides its roles in viral and bacterial infection, sialic acid also determines the infection of protozoan diseases. The etiological agent of malaria, Merozoites of Plasmodium falciparum, invades erythrocytes via both sialic acid independent and sialic acid dependent mechanisms69,70 relying on the binding of Plasmodium proteins to glycophorins, the major sialylated proteins on the erythrocyte.71 Other protozoan parasites, such as Toxoplasma gondii, the pathogen leading to toxoplasmosis, also recognize and attach to sialic acid on the host cells during the process of invasion. In fact, the higher the abundance of sialic acid on the host cell surface, the easier it is to be infected by protozoan.72 Recent proteomic analysis found sialic-acid-high-affinity binding proteins on T. gondii and named them SABP1 (sialic acid binding protein).72 Parasites whose SABP1 have been knocked out showed lower adhesion and infection in vitro and lower virulence in vivo, indicating the reliance of parasites on SABP1-sialic acid interaction for survival and disease establishment.73 An elegant review written by Jáskiewicz and coworkers enumerated these pathogens and their utilization of sialic acid as an infection target in detail,74 which is worth reading if interested.
The necessity of multivalent interaction in sialic acid-protein interactions
Normally, sialic acid residues bind to protein receptors mainly through weak interactions including electrostatic interaction via salt bridges and hydrogen bonds, which are so weak that they dissociate easily. Hence, a multivalent interaction is necessary whether binding siglecs or other proteins. This theory has been discussed by Whitesides and his coworkers in their review published in 1998.75 They examined the theoretical framework for polyvalency, described a consistent nomenclature for polyvalent interactions, and discussed the central role of entropy in polyvalency in detail. The comprehension of the multivalent interactions guided their research on designing multivalent sialic acid decorated materials for antivirus applications.75 Toone,76 Stoddart,21 Pieters,77 Haag78et al. also concentrated on this field and gradually enriched this theory. Receptor clustering is activated when monovalent lectins are anchored and exposed to cell membranes in which receptors diffuse through the dynamic lipid bilayer to form a cluster that is tightened by the binding to the multivalent ligand. This mechanism is responsible for the clustering of the intracellular domains of transmembrane proteins triggering signal transduction events.79–82
As the mechanisms and effects of the interaction between sialic acid and its protein receptors are more and more clearly elucidated, organic chemists have made great efforts to use modified sialic acid and sialomimetics as artificial ligands for their respective protein receptors.83–85 These monovalent derivates can efficiently bind to siglecs as blocking or targeting ligands. However, without clustering, the function of siglecs in modulating immune activity could hardly be activated.
As discussed above, it is necessary to form multivalent clusters in order to ensure high affinity with protein receptors for biomedical application. A primary idea is to covalently bind sialic acid on polymer, dendrimer, micelle and nanoparticles to form multivalent sialic acid clusters. As Whitesides demonstrated in his review, there may be a balance between the valence and steric stabilization in interactions between multivalent sialic acid materials and protein receptors. More and more different kinds of sialic acid decorated materials for biomedical application are emerging under the unremitting efforts of material scientists, biochemists and organic chemists. In the following part, we discuss some pioneer works and recent progress in this field.
Multivalent sialic acid materials for biomedical application
Multivalent sialic acids as targeting ligands for drug delivery
In the early period of identifying siglecs, scientists took them as markers of immune cells without defining the structure of siglecs and their functions in immune modulation. Therefore, multivalent sialic acid functionalized materials and monovalent chemically decorated sialylated glycans were synthesized for tracing immune cells in vivo, targeting cancerous leukocytes or immune cells in the tumor microenvironment for drug delivery. The research on siglecs mainly focused on Siglec-1, Siglec-2 and Siglec-4 since they are restrictedly expressed on specific immune cells.
Siglec-1, also known as sialoadhesins, is the only siglec without an intracellular function domain, meanwhile it has the longest extracellular C2 domain. The main duty of Siglec-1 is thought to be mediating cell–cell adhesion and endocytosis.86 Hence, targeting Siglec-1 mainly enables tracing macrophages in vivo or blocking its sialic acid binding domain to inhibit cell–cell adhesion and endocytosis. High binding affinity could be achieved either by modifying sialic acid structures87 or forming multivalent combinations.88 As is well known, there is an inflammatory nature to the tumor microenvironment. Macrophages have been proven to be the crucial inflammatory cells within the tumor microenvironment.89 These macrophages are called tumor-associated macrophages (TAMs), and are derived from monocytes in the blood and their polarized phenotype beyond M1 or M2. Although the polarized phenotype is different from normal macrophages, the expression of Siglec-1 on TAMs is abundant enough to be the target of drug delivery. Deng et al. made unremitting efforts to modify sialic acids with hydrophobic groups and thus cause them to assemble into liposomes carrying drugs to kill TAMs for tumor therapy.90–95 Paulson and coworkers developed sialic acid decorated nanoparticles that target Siglec-1 to deliver antigens to macrophages for improving the innate immune response.96
Siglec-2 is highly restrictedly expressed on B cells. This siglec mainly participates in setting a threshold for antigen-induced activation of B cells.97 This function is performed via activation of as many as six tyrosine-based motifs in the cytoplasmic domain of Siglec-2, including three immunoreceptor tyrosine-based inhibitory motifs (ITIMs). As well as regulating the activity of B cells, targeting Siglec-2 on B cells is a potential treatment method for B-cell lymphoma. Kiessling's group developed a series of polymers using a ruthenium initiator to initiate the ring-opening metathesis polymerization (ROMP) of norbornene carboxylic acid decorated with trisaccharide, Neu5Ac-α-2,6-Gal-β-1,4-Glc, and used it as an inhibitor of B cell activation via multivalent binding to Siglec-2 in trans.98,99 Paulson, Finn and coworkers performed studies on targeting Siglec-2 on B cells as a diagnosis, tracing and treatment method for B-cell lymphoma. Their works utilized a sialic acid decorated Qβ capsid packaging fluorescent protein to trace B-cell lymphoma in vivo,100 sialic acid bearing virus-like nanoparticles for photodynamic therapy101 and liposomes as drug delivery cargo.102 In another work they published in 2003, they employed Siglec-2 as a target to deliver antigen to B cells for stimulation of humoral immunity.103,104 Zhang and coworkers developed biocompatible sialic acid-modified chitosan–PLGA hybrid nanoparticles as a drug carrier for the targeted treatment of Siglec-2-positive non-Hodgkin's lymphoma.105 As knowledge on Siglec-2 has developed, scientists have discovered its trace existence on microglia. Wyss-coray and coworkers recently found that it could suppress phagocytosis that is upregulated on aged microglia – the decreased ability of microglia endocytose protein aggregates and cellular debris may be the leading cause of neurodegenerative disease. Aminooxy glycan-conjugated polymers with a poly(methyl vinyl ketone) backbone coupled to a dipalmitoylphosphatidylethanolamine lipid anchor were used to decorate the cell surface and confirmed this significant discovery.106
Siglec-4 is also a member of the immunoglobulin (Ig) super-family whose main function is inhibiting axonal growth. The expression of Siglec-4 on neuronal and microglial cells indicated that it mainly played a role in mediating the regulation and development of the nervous system.107 In the central nervous system (CNS), Siglec-4 participates in the initiation of myelination, formation of myelin sheaths, and long-term maintenance of oligodendrocyte structure and myelin integrity. In the peripheral nervous system (PNS), Siglec-4 seems to be involved only in the formation of intact myelin and long-term maintenance of myelin structure but not in the initiation of myelination.108 However, due to the complexity of the nervous system and the existence of the blood–brain barrier (BBB), it is not easy to target Siglec-4 to achieve the goal of targeted drug delivery, and few works have been reported on targeting Siglec-4 with multivalent sialic acids.109
Selectins are another category of sialic acid receptors expressed on the surface of mammal cells. So, targeting selectins that are upregulated at inflammatory sites and tumor sites using drug carriers decorated with sialic acid is a promising strategy for the treatment of inflammation and tumor. Yongzhong Du's group reported a series of works on sialic acid decorated materials targeting selectins for drug delivery. Their works are mainly focused on targeting E-Selectin at inflammation sites or in the tumor environment. The backbone of the materials they used ranged from modified natural polymers such as dextran to synthesized copolymers with complex three-dimensional structures. Sialic acid and hydrophobic group, such as octadecanoic acid (OA), decorated dextran (Dex) is one of the drug delivery platforms they successfully applied. They used these materials to deliver the curcumin polymeric prodrug for targeted therapy of acute kidney injury;110 methotrexate for rheumatoid arthritis;111 and doxorubicin for tumor treatment.112,113 They proved that this platform has a significant binding selectivity to E-selectin and could improve the accumulation of the drugs at the inflammation sites. In more recent works, they also developed other micelles for drug delivery112,114 and combined sialic acid modified polymers such as PEG with inorganic nanoparticles to form particles with more complex three-dimensional structures.115–118
Mediating inflammation by using multivalent sialic acid materials
Innate immune cells, such as monocytes, macrophages and neutrophils, dominate early inflammation.119,120 Activated inflammatory cells not only phagocytose the pathogen and clear non-apoptotic cells, but also release plenty of acute mediators of inflammation,121 such as the pro-inflammatory cytokines TNF-α, IL-1β and IL-6 to recruit and activate more leukocytes, which affects tissue regeneration, response to infection or pain, and neuronal activity significantly,122 and ceaselessly amplify this cascade. When the process of production of proinflammatory neutrophil extracellular traps (NETs) and release of pro-inflammation cytokines is too acute to be under control, these pro-inflammatory cytokines can cause diseases like systemic inflammatory response syndrome, atherosclerosis, rheumatoid arthritis, multiple sclerosis and septic shock.123 Human Siglec-7 and Siglec-9 and their murine orthologs Siglec-E are key immunomodulatory receptors found predominantly on hematopoietic cells and regulate immune balance in inflammatory diseases.
Siglec-7 is an inhibitory receptor mainly expressed on natural killer (NK) cells and monocytes. NK cells are important in tumor immunological surveillance and killing tumor cells. Hiroshi Tanaka and coworkers developed an allene monomer decorated by an α(2,8) disialic acid and initiated polymerization by a p-allyl nickel complex with an azido group to produce end-functionalized glycopolymers as a competitive ligand against natural ligand GD3, aiming to modulate the activation of NK cells.124 Soon after, Chihiro Sato collaborated with them and designed a new Siglec-7 ligand based on Dextran. This DiSia-Dex showed high binding avidity toward Siglec-7, with a Kd value of 5.87 × 10−10 M, and a high inhibitory activity for the interaction between Siglec-7 and GD3, with an IC50 value of 1.0 nM.125
The epidemic of SARS-CoV-2 caused a serious threat to global health. The main factors leading to the death of COVID-19 patients are the overreaction of the systemic immune system caused by coronavirus infection and acute respiratory distress syndrome caused by a large number of immune cells infiltrating into the lungs. Therefore, the regulation of immune cells became of great concern to scientists and clinicians. Although the glucocorticoid drugs and antibody drugs also have a certain effect, the long-term use of glucocorticoid hormone drugs can cause serious sequelae, and the antibody drugs could only neutralize the already produced inflammatory cytokines. Therefore, there is still a pressing need for developing more effective and safe drugs that can control the over activation of immune cells.
Considering that macrophages and neutrophils act as the leading cause of inflammation cascade, Sigec-9 on their plasmalemma has become an ideal regulator of over-activated immune cells. Bertozzi and her group have been working in this field for years. By ring-opening polymerization of glycosylated NCA monomers, they synthesized a series of materials based on polyaminoacids to target Siglec-9 on the surface of macrophages and neutrophils to inhibit the expression of macrophage pro-inflammatory cytokines such as IL-6 and TNF-α and the release of NETs. They employed a hydrophobic tail at the end of the polymer and realized cis binding between Siglec-9 and the materials using a novel method. The result showed that compared with soluble polymers, the hydrophobic tail could anchor in the cell membrane and promote the interaction between Siglec-9 and sialic acid on the materials in a cis pattern, and the glycosylated peptides anchored on the cell surface form mimic glycoproteins and exhibit excellent inhibiting effects on the expression of pro-inflammation cytokines and the release of NETs.126,127 Bertozzi also did lots of outstanding research on biological orthogonal reaction to modify saccharide on the cell surface on-site with the aim of regulating immune activation and just won the Nobel Prize in 2022.128
Many studies have also been reported by other groups to modulate systemic inflammation overresponses through siglecs. Scott and coworkers decorated α-2,8-linked sialic acid on PLGA nanoparticles, and proved their therapeutic effect in a variety of systemic inflammation on mouse models via interaction with Siglec-E on macrophages.129 Paulson and his coworkers developed an antibody connected siglec ligand that targeted immune cells via anti-IgD/E antibody and inhibited unwanted immune cell activation.130 This work defined a new idea of antibody–drug conjugates. Ohmae coated poly(sarcosine)-block-poly(L-lactic acid) (lactosome) with sialic acid monosaccharides and oligosaccharides to prevent accelerated blood clearance (ABC) via interaction with Siglec-E. They proved in this study that sialic acid interacting with Siglec-E could not only inhibit the expression of pro-inflammation cytokines but also suppress phagocytosis of the opsonized nanocarriers.131
Multivalent sialic acid materials against viruses
The Spanish flu pandemic of 1918 killed 50 million people, even more than those killed in World War I, and flu still kills hundreds of thousands of people around the world every year. So, humans have been struggling with pandemics caused by the viruses since then. After realizing that multivalent interactions in adhesion are the very first step in the infection process of pathogens like virus,132,133 bacteria,80,134 or fungi135,136 to host cells, multivalent sialic acid inhibitors have been seen as a promising tool and focus of research. Since the SARS-CoV-2 pandemic began, investigation of multivalent sialic acid inhibitors as antiviral drugs has been brought back in trend. In this part, we will review a few works of interest mainly on the application of multivalent sialic acid materials as anti-viral agents.
Current anti-virus therapies usually require high doses of small molecule drugs that have potential side effects and can lead to drug resistance in patients. Multivalent sialic acid materials as inhibitors do not kill pathogens but can block them from binding to cells, which can reduce the chances of developing drug-resistant strains.116 The efficiency of the virus binding materials highly depends on the valence, steric structure and size of materials. An elegant review written by Haag and his coworkers systemically demonstrated the relationship between inhibition efficacy of materials and these factors.137
Metallic nanoparticles absorb and scatter with great efficiency when interacting with light. The chemical properties of gold and silver indicated that different ligands can be linked to metal particles easily via reaction between these noble metals and sulfydryl. Thus, a few works have reported that multivalent sialic acid decorated metal nanoparticles could be used to detect virus. Niikura and coworkers linked monovalent sialic acid trisaccharides via a sulfate sulfhydryl functional amphiphilic linker. They proved that due to the regular steric structure of the virus capsids, their nanoparticles could arrange on mock virions neatly and cause a large red shift (∼200 nm) of the plasmon band, which could be used to detect virus.138 Russell and coworkers developed a novel system that specifically binds and detects human virus with a rapid reaction period of 30 min by decorating trivalent α-2,6-linked sialic acid on gold nanoparticles.139 Although these kinds of materials have been widely used in virus detection using other precise instruments,140–142 facing a virus like SARS-CoV-2 causing a pandemic, the simpler the test, the more efficient it is. Several scientists have reported a simple, visual and rapid detection of SARS-CoV-2.143–145
As a killer that has threatened human beings several times, the influenza virus has been investigated widely during the past century. Since the structure of the sialic acid binding protein HA and the mechanism of binding have been clearly characterized,146 plenty of multivalent sialic acid materials have been developed as inhibitors of influenza virus infection.147 Early in the 1990s, Whitesides and Roy led the trend of application of multivalent sialic acid materials as antivirus agents. While Roy focused on diverse dendritic structures,148–150 Whitesides was more interested in studying the mechanism and kinetics of multivalent interactions between this kind of material and the natural receptors.75,151–154 Based on these early findings, numerous research studies have been conducted in this field in the past few decades. Nishimura et al. ameliorated methods by employing trivalent α-2,3-sialyl trisaccharide and α-2,6-sialyl trisaccharide with poly(acrylic acid) (PAA) instead of monovalent sialic acid, and their materials exhibited much higher biological activities than the effects achieved by glycopolymers derived from the simple monovalent-type glycomonomers.155 They also employed cyclic glycopeptides as the backbone and linked three oligosaccharides containing sialic acid to the trimeric structure of HA.156 In another work, they replaced the oxygen of the glycoside bond of sialoside with sulfur to prevent hydrolytic digestion of the N-acetylneuraminic acid residue by viral neuraminidase and linked several sialic acids to gold and silver nanoparticles.157 Different from Whitesides's study preferring polyacrylamides as the backbone, Haag employed flexible and biocompatible polyglycerols as their novel backbone of multivalent sialic acid materials. Both linear and dendritic polyglycerols were employed to estimate the influence of several factors such as size, flexibility, steric structure and valence on the efficacy of inhibition. They precisely controlled the diameter of dendritic polyglycerol sialoside particles and found that the inhibitory activity of the tested polymeric nanoparticles drastically increased with size and particles with similar dimensions to the virus (50–100 nm) are exceedingly effective. They also observed a saturation point in degree of surface functionalization (i.e. ligand density), above which inhibition was not significantly improved.158 They proved that compared with rigid nanoparticles like gold nanoparticles, adaptive flexible sialylated nanogels based on polyglycerol achieved improved IAV inhibition by 400 times.159,160 The more flexible linear polyglycerol sialosides (PGSAs) exhibited higher inhibition potential than the dendritic polyglycerol sialosides to prevent virus attachment to the host cells.161 More recently, Haag cooperated with Kai Ludwig and Mohsen Adeli to decorate multivalent sialic acids on a flexible 2D graphene platform to achieve the trapping, blocking, and deactivation of respiratory viruses.162 Electron microscopy techniques such as cryo-TEM and single-molecule force spectroscopy were used to characterize their materials in a more direct way and promoted their investigation further and in a more quantitative manner.162,163
Since sialic acid is naturally expressed as the terminal unit of glycoproteins and glycolipids, sialoglycopolypeptide and sialic acid decorated lipid micelles were employed as natural ligand mimics to inhibit virus infection.164–173 Natural polysaccharides like chitosan could also be employed as a backbone due to their low immunogenicity, biocompatibility and degradability.174,175 As another biomacromolecule, nucleic acids could also be used as flexible backbones of sialic acid decorated materials.176 Since Haag and coworkers observed that nanoparticles with a size similar to influenza virus possess a higher inhibition efficacy, Hackenberger and coworkers decorated sialic acids on phage capsid to obtain a defined ligand arrangement block inhibitor for influenza virus entry.177 The high efficacy of inhibition also benefits from the host cell HA ligands on bacteriophage capsids. These host cell HA ligands are in an arrangement matching the geometry of binding sites of the spike protein, and can bind to viruses in a defined multivalent mode. Sialyllactose-conjugated filamentous bacteriophages were also used as antivirus agents via blocking HA with sialic acids decorated on them.178
Besides these works utilizing natural multivalent sialic acids to inhibit virus infection,179 more and more synthetic materials with novel structures have been used as backbones with the development of organic chemistry, materials chemistry and polymer science. A variety of molecules,180 dendrimers,181–183 polymers184–186 and nanoparticles123,187–189 have been developed as sialic acid carriers. Calixarene and dendrimers have the advantage of a defined molecular structure and thus have a strong future in clinical medical application.
There are many other viruses as well as influenza virus that use sialic acids on the surface of host cells as a binding site during the infection process.62–65,190–193 Acquired immunodeficiency syndrome (AIDS) caused by infection of human immunodeficiency virus (HIV) is still an incurable disease at present. It has been reported that CD4 is a major receptor for HIV-1194,195 and CD26 acts as a co-factor for entry of HIV-1 into CD4-positive cells.196 An α-2,8 ketosidic linked polysialic acid called colominic acid and its derivate obtained by modifying a sulfonic group at C9 showed significant inhibiting effects on HIV.197 Many other works have also been published,198 among which McReynolds and coworkers continually reported different anti-HIV materials based on dendrimers.199,200 Polyomaviruses are small, non-enveloped DNA viruses that cause persistent asymptomatic infections in various species, and can, upon activation to productive infection, lead to serious diseases in immunocompromised individuals.201 Divalent sialylated precision glycooligomers synthesized by Hartmann and coworkers via solid phase synthesis exhibit inhibiting effects on polyomaviruses.202 Some types of adenovirus and coronavirus could also be inhibited by synthesized sialic acid materials such as different multivalent dendrimers and sialic acid linked polypeptides (Table 1).203–205
Table 1 Materials and their reported applications
Target |
Materials |
Targeted protein subset |
Function |
Ref. |
Siglecs |
Benzene |
Siglec-1 |
Not mentioned |
88
|
Sialic acideoctadecylamine conjugate liposome |
Siglec-1 |
Antitumor drug delivery |
90–95
|
Sialic acid–modified polynorbornene |
Siglec-2 |
Inhibiting activation of B cells |
98 and 99 |
Qβ capsid |
Siglec-2 |
Tracing B-cell lymphoma |
100
|
Qβ virus-like particles |
Siglec-2 |
Photodynamic therapy |
101
|
Sialic conjugate liposome |
Siglec-2 |
Antitumor drug delivery |
102
|
Sialic conjugate liposome |
Siglec-2 |
Inhibiting activation of B cells |
103 and 104 |
Sialic acid-modified chitosan–PLGA hybrid nanoparticles |
Siglec-2 |
Antitumor drug delivery |
106
|
Polymers with a poly(methyl vinyl ketone) backbone coupled to a dipalmitoylphosphatidylethanolamine lipid anchor |
Siglec-2 |
Inhibiting microglia phagocytosis |
108
|
Sialic acid modified chitosan-poly(lactic-co-glycolic acid) (PLGA) nanoparticles |
Siglec-4 |
Drug delivery to the central nervous system |
119
|
Poly(allene monomer containing an a(2,8) disialic acid) |
Siglec-7 |
Modulating the activation of NK cells |
125
|
Sialic conjugate liposome |
Siglec-7 |
Antigen delivery to DCs |
126
|
Siglecs |
Sialic acid decorated polyamino acids |
Siglec-9 |
Suppressing the activation of immune cells |
127
|
α-2,8-Linked sialic acid decorated PLGA nanoparticles |
Siglec-E |
Suppressing the activation of immune cells |
129
|
Poly(sarcosine)-block-poly(L-lactic acid) (Lactosome) with sialic acid monosaccharides |
Siglec-E |
Suppressing phagocytosis of the opsonized nanocarriers |
130
|
Sialic acid decorated dextran |
E-Selectin |
Drug delivery |
109–111
|
Sialic acid-dextran-octadecanoic acid (SDO) micelles |
E-Selectin |
Drug delivery |
112
|
Sialic acid-functionalized PEG−PLGA microspheres |
E-Selectin |
Drug delivery |
113
|
Sialic acid (SA)-modified chitosan oligosaccharide-based biphasic calcium phosphate |
E-Selectin |
Drug delivery |
114
|
Sialic acid poly(ethylene glycol)-poly(acrylamide-co-acrylonitrile) |
E-Selectin |
Drug delivery |
115
|
Sialic acid modified and Fe3+ chelated Superparamagnetic iron oxide-loaded MPDA NPs |
E-Selectin |
Drug delivery |
116
|
Sialic acid-PEG-modified mesoporous polydopamine nanoparticles |
E-Selectin |
Drug delivery |
117
|
Virus proteins |
Sialic acid decorated gold nanoparticles |
Virus proteins |
Detecting virus |
137–140
|
Sialic acid decorated gold nanoparticles |
Influenza Hemagglutinins |
Investigate binding affinity |
141
|
Sialic acid decorated gold nanoparticles |
SARS-CoV-2 spike protein |
Detecting virus |
142–145
|
Virus proteins |
Dendritic sialoside |
Influenza virus haemagglutinin |
Antivirus therapy |
147, 149 and 181 |
|
Sialic acid decorated poly(acrylic acid) |
Influenza virus haemagglutinin |
Antivirus therapy |
151
|
|
Sialic acid decorated polyacrylamide |
Influenza virus haemagglutinin |
Antivirus therapy |
152–154 and 184 |
|
Sialic acid decorated cyclic glycopeptides |
Influenza virus haemagglutinin |
Antivirus therapy |
155
|
|
Thiosialosides conjugated metal nanoparticles |
Influenza virus haemagglutinin |
Antivirus therapy |
156
|
|
Sialic acid-conjugated polyglycerol-based nanoparticles |
Influenza virus haemagglutinin |
Antivirus therapy |
157
|
|
Sialic-acid-functionalized gold nanoparticles |
Influenza virus haemagglutinin |
Antivirus therapy |
158
|
|
Sialic acid-conjugated polyglycerol-based nanogels |
Influenza virus haemagglutinin |
Antivirus therapy |
159
|
|
Linear and dendritic polyglycerol sialosides |
Influenza virus haemagglutinin |
Antivirus therapy |
160
|
|
Sialic-acid-functionalized graphene |
Influenza virus haemagglutinin |
Antivirus therapy |
161
|
|
Sialoglycopolypeptide |
Influenza virus haemagglutinin |
Antivirus therapy |
163 and 164 |
|
Ganglioside ligand |
Influenza virus haemagglutinin |
Antivirus therapy |
165
|
|
Sialic acid bearing liposomes |
Influenza virus haemagglutinin |
Antivirus therapy |
166–168
|
|
Multivalent sialoside protein conjugates |
Influenza hemagglutinin and neuraminidase |
Antivirus therapy |
167 and 169–172 |
Virus proteins |
Sialyloligosaccharide decorated chitosan |
Influenza virus haemagglutinin |
Antivirus therapy |
173 and 174 |
|
Sialic acid decorated nucleic acids |
Influenza virus haemagglutinin |
Antivirus therapy |
175
|
|
Sialic acids decorated phage capsid |
Influenza virus haemagglutinin |
Antivirus therapy |
176
|
|
Sialyllactose-conjugated filamentous bacteriophages |
Influenza virus haemagglutinin |
Antivirus therapy |
177
|
|
Trivalent glycopeptide mimetic and analogues |
Influenza virus haemagglutinin |
Antivirus therapy |
179
|
|
Multivalent sialic acid molecules |
Influenza virus haemagglutinin |
Antivirus therapy |
180
|
|
Multivalent sialyl oligosaccharides decorated brush polymers |
Influenza virus haemagglutinin |
Antivirus therapy |
184
|
|
Sialic acid decorated poly(3,4-ethylenedioxythiophene (EDOT)) |
Influenza virus haemagglutinin |
Antivirus therapy |
185
|
|
Sialic acid modified poly(L-lactic acid)-b-poly(ethylene glycol) (PLLA-b-PEG) |
Influenza virus haemagglutinin |
Antivirus therapy |
187
|
|
Sialic acid decorated poly(ethylene oxide)–polycaprolactone polymersomes |
Influenza virus haemagglutinin |
Antivirus therapy |
188
|
|
Sialic acid conjugated highly-branched α-glucuronic acid-linked cyclic dextrins |
Influenza virus haemagglutinin |
Antivirus therapy |
189
|
|
Sulfated colominic acid |
HIV |
Antivirus therapy |
197
|
|
Sialic acid conjugated glycopeptide |
HIV |
Antivirus therapy |
198
|
|
Sialic acid-PAMAM glycodendrimers |
HIV |
Antivirus therapy |
199
|
|
Hexavalent sialic acid sulfoglycodendrimers |
HIV |
Antivirus therapy |
200
|
Virus proteins |
Divalent sialylated glycooligomers conjugated to resins |
Polyomaviruses protein 1 |
Antivirus therapy |
202
|
Triazole linker-based trivalent sialic acid |
Adenovirus type 37 |
Antivirus therapy |
203
|
Multimeric sialic acid glycoclusters |
SARS-CoV-2 spike protein |
Antivirus therapy |
204
|
Mucins |
Human coronavirus OC43 spike protein |
Antivirus therapy |
205
|
Conclusions and perspective
Sialic acids and polysialic acids play complex roles in different processes and stages of living activities, which also determines the variety of applications of multivalent sialic acid materials in biomedicine. In this review, we summarized the role of sialic acid and the use of multivalent sialic acid materials for biomedical applications. Since the function of sialic acid in life activities has been defined more and more clearly, the development of multivalent sialic acid materials for biomedical applications has bloomed. Based on the utilization of sialic acid combined with its receptors such as siglecs, selectins and virus proteins, various materials have been synthesized for the treatment of inflammation, tumor, virus and infectious disease. Multiple parameters such as the valence of sialic acid, the size and hardness of the material, and steric hindrance between multiple ligands collaboratively determine the affinity of the materials to the protein receptors, which, in other words, determines the efficiency of the materials.
The design of multivalent sialic acid materials based on polymers and other materials is expected to adapt to the natural characteristics of multivalent recognition in natural processes and generate unique new macromolecular therapeutic drug forms.206 At present, many pioneering biomedical studies on multivalent sialic acid modified materials have verified their efficacy and safety in cells or animal models, but this field is still yet to be fully developed. In the future, there will be some key issues that need to be solved for this kind of therapeutic material: First, it is known that the biological effects of the majority of sialic acids are immune-suppressive, however, in light of the wide expression of sialic acid receptors across the body, how to realize specific binding is a difficult task. There has been many studies focusing on the structural modification of sialic acid to achieve specific binding, but the macromolecular architectural design of multivalent sialic acid materials may constitute another dimension for optimizing the specificity. Second, a charming point of the sialic acid–siglec interaction lies in the fact that siglecs are mostly expressed on the immune cells, and this provides a clear target for utilizing multivalent sialic acid materials for immune modulation. Since immune-related diseases constitute a large part of human diseases and generally lack effective therapeutic means, study on multivalent sialic acid materials may provide new treatment opportunities for these immune-related diseases. Third, the concept of multivalent/polyvalent materials as therapeutic agents has not been recognized by the pharmaceutical industry, and the corresponding standardized research and evaluation methods still need to be studied and established. These factors may constitute a key hurdle to finally putting a therapeutic material into the clinic. However, considering the unique characteristics of multivalent interactions in biological processes, especially the sugar system, we believe that multivalent sialic acid materials can become a very meaningful class of therapeutic materials and play a more and more important role in biomedical and biointerface regulation within body systems.
Conflicts of interest
There are no conflicts to declare.
Acknowledgements
This work was supported by the National Natural Science Foundation of China (22222509, 51973215 and 51829302), Bureau of International Cooperation Chinese Academy of Sciences (121522KYSB20200029), Jilin Provincial International Cooperation Key Laboratory of Biomedical Polymers (20210504001GH), and the Youth Innovation Promotion Association of Chinese Academy of Sciences (2020232).
References
- J. D. Marth, A unified vision of the building blocks of life, Nat. Cell Biol., 2008, 10, 1015–1016 CrossRef CAS PubMed.
- S. Weinbaum, J. M. Tarbell and E. R. Damiano, The structure and function of the endothelial glycocalyx layer, Annu. Rev. Biomed. Eng., 2007, 9, 121–167 CrossRef CAS PubMed.
- C. Bull, T. Heise, G. J. Adema and T. J. Boltje, Sialic Acid Mimetics to Target the Sialic Acid-Siglec Axis, Trends Biochem. Sci., 2016, 41, 519–531 CrossRef PubMed.
- P. A. Levene and K. Landsteiner, On some new lipoids, J. Biol. Chem., 1927, 75, 607–612 CrossRef CAS.
- E. Walz, Über das Vorkommen von Kerasin in der normalen Rindermilz, Biol. Chem., 1927, 166(4–6), 210–222 CrossRef CAS.
-
R. Schauer and J. P. Kamerling, Chapter One – Exploration of the Sialic Acid World, in Advances in Carbohydrate Chemistry and Biochemistry, ed. D. C. Baker, Academic Press, 2018, vol. 75, pp. 1–213 Search PubMed.
- H. Yang, L. Lu and X. Chen, An overview and future prospects of sialic acids, Biotechnol. Adv., 2021, 46, 107678 CrossRef CAS PubMed.
- P. Gorog and J. D. Pearson, Sialic acid moieties on surface glycoproteins protect endothelial cells from proteolytic damage, J. Pathol., 1985, 146, 205–212 CrossRef CAS PubMed.
- A. L. Sorensen, V. Rumjantseva, S. Nayeb-Hashemi, H. Clausen, J. H. Hartwig, H. H. Wandall and K. M. Hoffmeister, Role of sialic acid for platelet life span: exposure of beta-galactose results in the rapid clearance of platelets from the circulation by asialoglycoprotein receptor-expressing liver macrophages and hepatocytes, Blood, 2009, 114, 1645–1654 CrossRef PubMed.
- A. G. Morell, G. Gregoriadis, I. H. Scheinberg, J. Hickman and G. Ashwell, The Role of Sialic Acid in Determining the Survival of Glycoproteins in the Circulation, J. Biol. Chem., 1971, 246, 1461–1467 CrossRef CAS PubMed.
- W. H. Yang, P. V. Aziz, D. M. Heithoff, M. J. Mahan, J. W. Smith and J. D. Marth, An intrinsic mechanism of secreted protein aging and turnover, Proc. Natl. Acad. Sci. U. S. A., 2015, 112, 13657–13662 CrossRef CAS PubMed.
- B. Strilić, J. Eglinger, M. Krieg, M. Zeeb, J. Axnick, P. Babál, D. J. Müller and E. Lammert, Electrostatic Cell-Surface Repulsion Initiates Lumen Formation in Developing Blood Vessels, Curr. Biol., 2010, 20, 2003–2009 CrossRef PubMed.
- R. P. McEver, K. L. Moore and R. D. Cummings, Leukocyte trafficking mediated by selectin-carbohydrate interactions, J. Biol. Chem., 1995, 270, 11025–11028 CrossRef CAS PubMed.
- M. S. Macauley, P. R. Crocker and J. C. Paulson, Siglec-mediated regulation of immune cell function in disease, Nat. Rev. Immunol., 2014, 14, 653–666 CrossRef CAS PubMed.
- C. Büll, T. Heise, G. J. Adema and T. J. Boltje, Sialic Acid Mimetics to Target the Sialic Acid–Siglec Axis, Trends Biochem. Sci., 2016, 41, 519–531 CrossRef PubMed.
- T. Heise, J. D. Langereis, E. Rossing, M. I. de Jonge, G. J. Adema, C. Büll and T. J. Boltje, Selective Inhibition of Sialic Acid-Based Molecular Mimicry in Haemophilus influenzae Abrogates Serum Resistance, Cell Chem. Biol., 2018, 25, 1279–1285 CrossRef CAS PubMed.
- J. E. Stencel-Baerenwald, K. Reiss, D. M. Reiter, T. Stehle and T. S. Dermody, The sweet spot: defining virus–sialic acid interactions, Nat. Rev. Microbiol., 2014, 12, 739–749 CrossRef CAS PubMed.
- E. A. Visser, S. J. Moons, S. Timmermans, H. de Jong, T. J. Boltje and C. Bull, Sialic acid O-acetylation: From biosynthesis to roles in health and disease, J. Biol. Chem., 2021, 297, 100906 CrossRef CAS PubMed.
- R. Schauer and J. P. Kamerling, Exploration of the Sialic Acid World, Adv. Carbohydr. Chem. Biochem., 2018, 75, 1–213 CrossRef PubMed.
- N. J. Overeem, E. van der Vries and J. Huskens, A Dynamic, Supramolecular View on the Multivalent Interaction between Influenza Virus and Host Cell, Small, 2021, 17(13), e2007214 CrossRef PubMed.
- S. A. Kalovidouris, O. Blixt, A. Nelson, S. Vidal, W. Bruce Turnbull, J. C. Paulson and J. Fraser Stoddart, Chemically Defined Sialoside Scaffolds for Investigation of Multivalent Interactions with Sialic Acid Binding Proteins, J. Org. Chem., 2003, 68, 8485–8493 CrossRef CAS PubMed.
- H. Yang, L. Lu and X. Chen, An overview and future prospects of sialic acids, Biotechnol. Adv., 2021, 46, 107678 CrossRef CAS PubMed.
- P. Crocker, E. A. Clark, Y. Jones and J. H. Kehrl, Siglecs: a family of sialic-acid binding lectins, Glycobiology, 1998, 8, 5–6 Search PubMed.
- I. Ibarlucea-Benitez, P. Weitzenfeld, P. Smith and J. V. Ravetch, Siglecs-7/9 function as inhibitory immune checkpoints in vivo and can be targeted to enhance therapeutic antitumor immunity, Proc. Natl. Acad. Sci. U. S. A., 2021, 118(26), e2107424118 CrossRef CAS PubMed.
- M. Pia Lenza, U. Atxabal and I. Oyenarte, Jesús Jiménez-Barbero and June Ereño-Orbea, Current Status on Therapeutic Molecules Targeting Siglec Receptors, Cells, 2020, 9 Search PubMed.
- P. R. Crocker and S. Gordon, Properties and distribution of a lectin-like hemagglutinin differentially expressed by murine stromal tissue macrophages, J. Exp. Med., 1986, 164, 1862–1875 CrossRef CAS PubMed.
- D. R. Boué and T. W. Lebien, Structural characterization of the human B lymphocyte- restricted differentiation antigen CD22. Comparison with CD21 (complement receptor type 2/Epstein–Barr virus receptor), J. Immunol., 1988, 140, 192–199 CrossRef.
- T. Kiwamoto, N. Kawasaki, J. C. Paulson and B. S. Bochner, Siglec-8 as a drugable target to treat eosinophil and mast cell-associated conditions, Pharmacol. Ther., 2012, 135, 327–336 CrossRef CAS PubMed.
- T. Angata, Siglec-15: A potential regulator of osteoporosis, cancer, and infectious diseases, J. Biomed. Sci., 2020, 27, 10 CrossRef PubMed.
- J. V. Ravetch and L. L. Lanier, Immune Inhibitory Receptors, Science, 2000, 290, 84–89 CrossRef CAS PubMed.
- Y. Wu, D. Ren and G.-Y. Chen, Siglec-E Negatively Regulates the Activation of TLR4 by Controlling Its Endocytosis, J. Immunol., 2016, 197, 3336–3347 CrossRef CAS PubMed.
- X. Wang, N. Mitra, I. Secundino, K. Banda, P. Cruz, V. Padler-Karavani, A. Verhagen, C. Reid, M. Lari, E. Rizzi, C. Balsamo, G. Corti, G. De Bellis, L. Longo, N. C. S. Program, W. Beggs, D. Caramelli, S. A. Tishkoff, T. Hayakawa, E. D. Green, J. C. Mullikin, V. Nizet, J. Bui and A. Varki, Specific inactivation of two immunomodulatory SIGLEC genes during human evolution, Proc. Natl. Acad. Sci. U. S. A., 2012, 109, 9935–9940 CrossRef CAS PubMed.
- B. A. Youngblood, J. Leung, R. Falahati, J. Williams, J. Schanin, E. C. Brock, B. Singh, A. T. Chang, J. A. O'Sullivan, R. P. Schleimer, N. Tomasevic, C. R. Bebbington and B. S. Bochner, Discovery, Function, and Therapeutic Targeting of Siglec-8, Cells, 2020, 10, 19 CrossRef PubMed.
- P. R. Crocker, J. C. Paulson and A. Varki, Siglecs and their roles in the immune system, Nat. Rev. Immunol., 2007, 7, 255–266 CrossRef CAS PubMed.
- H. Xiao, E. C. Woods, P. Vukojicic and C. R. Bertozzi, Precision glycocalyx editing as a strategy for cancer immunotherapy, Proc. Natl. Acad. Sci. U. S. A., 2016, 113, 10304–10309 CrossRef CAS PubMed.
- H. Laubli, O. M. Pearce, F. Schwarz, S. S. Siddiqui, L. Deng, M. A. Stanczak, L. Deng, A. Verhagen, P. Secrest, C. Lusk, A. G. Schwartz, N. M. Varki, J. D. Bui and A. Varki, Engagement of myelomonocytic Siglecs by tumor-associated ligands modulates the innate immune response to cancer, Proc. Natl. Acad. Sci. U. S. A., 2014, 111, 14211–14216 CrossRef CAS PubMed.
- E. Rodriguez, K. Boelaars, K. Brown, R. J. Eveline Li, L. Kruijssen, S. C. M. Bruijns, T. van Ee, S. T. T. Schetters, M. H. W. Crommentuijn, J. C. van der Horst, N. C. T. van Grieken, S. J. van Vliet, G. Kazemier, E. Giovannetti, J. J. Garcia-Vallejo and Y. van Kooyk, Sialic acids in pancreatic cancer cells drive tumour-associated macrophage differentiation via the Siglec receptors Siglec-7 and Siglec-9, Nat. Commun., 2021, 12, 1270 CrossRef CAS PubMed.
- A. A. Barkal, R. E. Brewer, M. Markovic, M. Kowarsky, S. A. Barkal, B. W. Zaro, V. Krishnan, J. Hatakeyama, O. Dorigo, L. J. Barkal and I. L. Weissman, CD24 signalling through macrophage Siglec-10 is a target for cancer immunotherapy, Nature, 2019, 572, 392–396 CrossRef CAS PubMed.
- J. Wang, J. Sun, L. N. Liu, D. B. Flies, X. Nie, M. Toki, J. Zhang, C. Song, M. Zarr, X. Zhou, X. Han, K. A. Archer, T. O'Neill, R. S. Herbst, A. N. Boto, M. F. Sanmamed, S. Langermann, D. L. Rimm and L. Chen, Siglec-15 as an immune suppressor and potential target for normalization cancer immunotherapy, Nat. Med., 2019, 25, 656–666 CrossRef CAS PubMed.
- L. A. M. Cornelissen, A. Blanas, J. C. van der Horst, L. Kruijssen, A. Zaal, T. O'Toole, L. Wiercx, Y. van Kooyk and S. J. van Vliet, Disruption of sialic acid metabolism drives tumor growth by augmenting CD8(+) T cell apoptosis, Int. J. Cancer, 2019, 144, 2290–2302 CrossRef CAS PubMed.
- C. Bull, T. J. Boltje, N. Balneger, S. M. Weischer, M. Wassink, J. J. van Gemst, V. R. Bloemendal, L. Boon, J. van der Vlag, T. Heise, M. H. den Brok and G. J. Adema, Sialic Acid Blockade Suppresses Tumor Growth by Enhancing T-cell-Mediated Tumor Immunity, Cancer Res., 2018, 78, 3574–3588 CrossRef CAS PubMed.
- X. Feng, J. Liu, W. Xu, G. Li and J. Ding, Tackling autoimmunity with nanomedicines, Nanomedicine, 2020, 15, 1585–1597 CrossRef CAS PubMed.
- J. Chen, J. Ding, W. Xu, T. Sun, H. Xiao, X. Zhuang and X. Chen, Receptor and Microenvironment Dual-Recognizable Nanogel for Targeted Chemotherapy of Highly Metastatic Malignancy, Nano Lett., 2017, 17, 4526–4533 CrossRef CAS PubMed.
- X. Feng, W. Xu, Z. Li, W. Song, J. Ding and X. Chen, Immunomodulatory Nanosystems, Adv. Sci., 2019, 6, 1900101 CrossRef PubMed.
- J. Zhang, Y. Lin, Z. Lin, Q. Wei, J. Qian, R. Ruan, X. Jiang, L. Hou, J. Song, J. Ding and H. Yang, Stimuli-Responsive Nanoparticles for Controlled Drug Delivery in Synergistic Cancer Immunotherapy, Adv. Sci., 2022, 9, e2103444 CrossRef PubMed.
- B. A. H. Smith and C. R. Bertozzi, The clinical impact of glycobiology: targeting selectins, Siglecs and mammalian glycans, Nat. Rev. Drug Discovery, 2021, 20, 217–243 CrossRef CAS PubMed.
- P. Görög and I. B. Kovács, Anti-inflammatory
effect of sialic acid, Agents Actions, 1978, 8, 543–545 CrossRef PubMed.
- R. P. McEver, Selectins: initiators of leucocyte adhesion and signalling at the vascular wall, Cardiovasc. Res., 2015, 107, 331–339 CrossRef CAS PubMed.
- C. Foxall, S. Watson, D. Dowbenko, C. Fennie, L. Lasky, M. Kiso, A. Hasegawa, D. Asa and B. Brandley, The three members of the selectin receptor family recognize a common carbohydrate epitope, the sialyl Lewis(x) oligosaccharide, J. Cell Biol., 1992, 117(4), 895–902 CrossRef CAS PubMed.
- D. Sako, X.-J. Chang, K. M. Barone, G. Vachino, H. M. White, G. Shaw, G. M. Veldman, K. M. Bean, T. J. Ahern, B. Furie, D. A. Cumming and G. R. Larsen, Expression cloning of a functional glycoprotein ligand for P-selectin, Cell, 1993, 75, 1179–1186 CrossRef CAS PubMed.
- K. E. Norgard, K. L. Moore, S. Diaz, N. L. Stults, S. Ushiyama, R. P. McEver, R. D. Cummings and A. Varki, Characterization of a specific ligand for P-selectin on myeloid cells. A minor glycoprotein with sialylated O-linked oligosaccharides, J. Biol. Chem., 1993, 268, 12764–12774 CrossRef CAS PubMed.
- A. Aruffo, W. Kolanus, G. Walz, P. Fredman and B. Seed, CD62/P-selectin recognition of myeloid and tumor cell sulfatides, Cell, 1991, 67, 35–44 CrossRef CAS PubMed.
- S. Aigner, Z. M. Sthoeger, M. Fogel, E. Weber, J. R. Zarn, M. Ruppert, Y. Zeller, D. Vestweber, R. Stahel, M. Sammar and P. Altevogt, CD24, a Mucin-Type Glycoprotein, Is a Ligand for P-Selectin on Human Tumor Cells, Blood, 1997, 89, 3385–3395 CrossRef CAS PubMed.
- Y. Lmai, L. A. Lasky and S. D. Rosen, Sulphation requirement for GlyCAM-1, an endothelial ligand for L-selectin, Nature, 1993, 361, 555–557 CrossRef PubMed.
- R. P. McEver and C. Zhu, Rolling cell adhesion, Annu. Rev. Cell Dev. Biol., 2010, 26, 363–396 CrossRef CAS PubMed.
- R. P. McEver, Selectins: initiators of leucocyte adhesion and signalling at the vascular wall, Cardiovasc. Res., 2015, 107, 331–339 CrossRef CAS PubMed.
-
M. Matrosovich, G. Herrler and H. D. Klenk, Sialic Acid Receptors of Viruses, in SialoGlyco Chemistry and Biology II: Tools and Techniques to Identify and Capture Sialoglycans, ed. R. Gerardy-Schahn, P. Delannoy and M. von Itzstein, Springer International Publishing, Cham, 2015, pp. 1–28 Search PubMed.
- M. R. Knowles and R. C. Boucher, Mucus clearance as a primary innate defense mechanism for mammalian airways, J. Clin. Invest., 2002, 109, 571–577 CrossRef CAS PubMed.
- Q. Xu, Y. Shan, N. Wang, Y. Liu, M. Zhang and M. Ma, Sialic acid involves in the interaction between ovomucin and hemagglutinin and influences the antiviral activity of ovomucin, Int. J. Biol. Macromol., 2018, 119, 533–539 CrossRef CAS PubMed.
- I. Bakai-Bereczki, M. Herczeg, B. György, L. Naesens and P. Herczegh, Synthesis of a sialic acid derivative of ristocetin aglycone as an inhibitor of influenza virus, Chem. Pap., 2015, 69, 1136–1140 CAS.
- S. Yamada, Y. Suzuki, T. Suzuki, M. Q. Le, C. A. Nidom, Y. Sakai-Tagawa, Y. Muramoto, M. Ito, M. Kiso, T. Horimoto, K. Shinya, T. Sawada, M. Kiso, T. Usui, T. Murata, Y. Lin, A. Hay, L. F. Haire, D. J. Stevens, R. J. Russell, S. J. Gamblin, J. J. Skehel and Y. Kawaoka, Haemagglutinin mutations responsible for the binding of H5N1 influenza A viruses to human-type receptors, Nature, 2006, 444, 378–382 CrossRef CAS PubMed.
- R. J. G. Hulswit, Y. Lang, M. J. G. Bakkers, W. Li, Z. Li, A. Schouten, B. Ophorst, F. J. M. van Kuppeveld, G. J. Boons, B. J. Bosch, E. G. Huizinga and R. J. de Groot, Human coronaviruses OC43 and HKU1 bind to 9-O-acetylated sialic acids via a conserved receptor-binding site in spike protein domain A, Proc. Natl. Acad. Sci. U. S. A., 2019, 116, 2681–2690 CrossRef CAS PubMed.
- W. Widagdo, N. M. A. Okba, W. Li, A. de Jong, R. L. de Swart, L. Begeman, J. M. A. van den Brand, B. J. Bosch and B. L. Haagmans, Species-Specific Colocalization of Middle East Respiratory Syndrome Coronavirus Attachment and Entry Receptors, J. Virol., 2019, 93, e00107–19 CrossRef CAS PubMed.
- L. Nguyen, K. A. McCord, D. T. Bui, K. M. Bouwman, E. N. Kitova, M. Elaish, D. Kumawat, G. C. Daskhan, I. Tomris, L. Han, P. Chopra, T. J. Yang, S. D. Willows, A. L. Mason, L. K. Mahal, T. L. Lowary, L. J. West, S. D. Hsu, T. Hobman, S. M. Tompkins, G. J. Boons, R. P. de Vries, M. S. Macauley and J. S. Klassen, Sialic acid-containing glycolipids mediate binding and viral entry of SARS-CoV-2, Nat. Chem. Biol., 2022, 18, 81–90 CrossRef CAS PubMed.
- C. W. Tan, C. H. Huan Hor, S. S. Kwek, H. K. Tee, I. C. Sam, E. L. K. Goh, E. E. Ooi, Y. F. Chan and L. F. Wang, Cell surface alpha2,3-linked sialic acid facilitates Zika virus internalization, Emerging Microbes Infect., 2019, 8, 426–437 CrossRef CAS PubMed.
- E. Severi, D. W. Hood and G. H. Thomas, Sialic acid utilization by bacterial pathogens, Microbiology, 2007, 153(9), 2817–2822 CrossRef CAS PubMed.
- N. D. McDonald, J.-B. Lubin, N. Chowdhury and E. F. Boyd, Host-Derived Sialic Acids Are an Important Nutrient Source Required for Optimal Bacterial Fitness In Vivo, MBio, 2016, 7, e02237–e02215 CrossRef CAS PubMed.
- S. Almagro-Moreno and E. F. Boyd, Sialic Acid Catabolism Confers a Competitive Advantage to Pathogenic Vibrio cholerae in the Mouse Intestine, Infect. Immun., 2009, 77, 3807–3816 CrossRef CAS PubMed.
- A. F. Cowman and B. S. Crabb, Invasion of Red Blood Cells by Malaria Parasites, Cell, 2006, 124, 755–766 CrossRef CAS PubMed.
- R. L. Ord, M. Rodriguez, T. Yamasaki, S. Takeo, T. Tsuboi and C. A. Lobo, Targeting sialic acid dependent and independent pathways of invasion in Plasmodium falciparum, PLoS One, 2012, 7, e30251 CrossRef CAS PubMed.
- M. Jungery, G. Pasvol, C. I. Newbold and D. J. Weatherall, A lectin-like receptor is involved in invasion of erythrocytes by Plasmodium falciparum, Proc. Natl. Acad. Sci. U. S. A., 1983, 80, 1018–1022 CrossRef CAS PubMed.
- V. G. Monteiro, C. P. Soares and W. de Souza, Host cell surface sialic acid residues are involved on the process of penetration of Toxoplasma gondii into mammalian cells, FEMS Microbiol. Lett., 1998, 164, 323–327 CrossRef CAS PubMed.
- M. Xing, N. Yang, N. Jiang, D. Wang, X. Sang, Y. Feng, R. Chen, X. Wang and Q. Chen, A Sialic Acid-Binding Protein SABP1 of Toxoplasma gondii Mediates Host Cell Attachment and Invasion, J. Infect. Dis., 2020, 222, 126–135 CrossRef PubMed.
- P. Burzynska, L. F. Sobala, K. Mikolajczyk, M. Jodlowska and E. Jaskiewicz, Sialic Acids as Receptors for Pathogens, Biomolecules, 2021, 11, 831–852 CrossRef CAS PubMed.
- M. Mammen, S.-K. Choi and G. M. Whitesides, Polyvalent Interactions in Biological Systems: Implications for Design and Use of Multivalent Ligands and Inhibitors, Angew. Chem., Int. Ed., 1998, 37, 2754–2794 CrossRef PubMed.
- J. J. Lundquist and E. J. Toone, The Cluster Glycoside Effect, Chem. Rev., 2002, 102, 555–578 CrossRef CAS PubMed.
- R. J. Pieters, Maximising multivalency effects in protein-carbohydrate interactions, Org. Biomol. Chem., 2009, 7, 2013–2025 RSC.
- M. Weber, A. Bujotzek and R. Haag, Quantifying the rebinding effect in multivalent chemical ligand-receptor systems, J. Chem. Phys., 2012, 137, 054111 CrossRef PubMed.
- C. R. Bertozzi and L. Kiessling Laura, Chemical Glycobiology, Science, 2001, 291, 2357–2364 CrossRef CAS PubMed.
- C.-H. Heldin, Dimerization of cell surface receptors in signal transduction, Cell, 1995, 80, 213–223 CrossRef CAS PubMed.
- J. E. Gestwicki and L. L. Kiessling, Inter-receptor communication through arrays of bacterial chemoreceptors, Nature, 2002, 415, 81–84 CrossRef CAS PubMed.
- L. L. Kiessling, J. E. Gestwicki and L. E. Strong, Synthetic multivalent ligands as probes of signal transduction, Angew. Chem., Int. Ed., 2006, 45, 2348–2368 CrossRef CAS PubMed.
- A. Gonzalez-Gil and R. L. Schnaar, Siglec Ligands, Cells, 2021, 10, 1260–1281 CrossRef CAS PubMed.
- L. D. Movsisyan and M. S. Macauley, Structural advances of Siglecs: insight into synthetic glycan ligands for immunomodulation, Org. Biomol. Chem., 2020, 18, 5784–5797 RSC.
- N. Komura, K. Kato, T. Udagawa, S. Asano, H.-N. Tanaka, A. Imamura, H. Ishida, M. Kiso and H. Ando, Constrained sialic acid donors enable selective synthesis of α-glycosides, Science, 2019, 364, 677–680 CrossRef CAS PubMed.
- P. R. Crocker, J. C. Paulson and A. Varki, Siglecs and their roles in the immune system, Nat. Rev. Immunol., 2007, 7, 255–266 CrossRef CAS PubMed.
- C. M. Nycholat, C. Rademacher, N. Awasaki and J. C. Paulson, In silico-aided design of a glycan ligand of sialoadhesin for in vivo targeting of macrophages, J. Am. Chem. Soc., 2012, 134, 15696–15699 CrossRef CAS PubMed.
- Z. Gan and R. Roy, Sialoside clusters as potential ligands for siglecs (sialoadhesins), Can. J. Chem., 2002, 80(8), 908–916 CrossRef CAS.
- L. M. Coussens and Z. Werb, Inflammation and cancer, Nature, 2002, 420, 860–867 CrossRef CAS PubMed.
- Z. She, T. Zhang, X. Wang, X. Li, Y. Song, X. Cheng, Z. Huang and Y. Deng, The anticancer efficacy of pixantrone-loaded liposomes decorated with sialic acid-octadecylamine conjugate, Biomaterials, 2014, 35, 5216–5225 CrossRef CAS PubMed.
- J. Sun, Y. Song, M. Lu, X. Lin, Y. Liu, S. Zhou, Y. Su and Y. Deng, Evaluation of the antitumor effect of dexamethasone palmitate and doxorubicin co-loaded liposomes modified with a sialic acid-octadecylamine conjugate, Eur. J. Pharm. Sci., 2016, 93, 177–183 CrossRef CAS PubMed.
- S. Zhou, T. Zhang, B. Peng, X. Luo, X. Liu, L. Hu, Y. Liu, D. Di, Y. Song and Y. Deng, Targeted delivery of epirubicin to tumor-associated macrophages by sialic acid-cholesterol conjugate modified liposomes with improved antitumor activity, Int. J. Pharm., 2017, 523, 203–216 CrossRef CAS PubMed.
- Q. Qiu, C. Li, Y. Song, T. Shi, X. Luo, H. Zhang, L. Hu, X. Yan, H. Zheng, M. Liu, M. Liu, M. Liu, S. Yang, X. Liu, G. Chen and Y. Deng, Targeted delivery of ibrutinib to tumor-associated macrophages by sialic acid-stearic acid conjugate modified nanocomplexes for cancer immunotherapy, Acta Biomater., 2019, 92, 184–195 CrossRef CAS PubMed.
- X. Tang, D. Sui, M. Liu, H. Zhang, M. Liu, S. Wang, D. Zhao, W. Sun, M. Liu, X. Luo, X. Lai, X. Liu, Y. Deng and Y. Song, Targeted delivery of zoledronic acid through the sialic acid - Siglec axis for killing and reversal of M2 phenotypic tumor-associated macrophages - A promising cancer immunotherapy, Int. J. Pharm., 2020, 590, 119929 CrossRef CAS PubMed.
- C. Li, Q. Qiu, X. Gao, X. Yan, C. Fan, X. Luo, X. Liu, S. Wang, X. Lai, Y. Song and Y. Deng, Sialic acid conjugate-modified liposomal platform modulates immunosuppressive tumor microenvironment in multiple ways for improved immune checkpoint blockade therapy, J. Controlled Release, 2021, 337, 393–406 CrossRef CAS PubMed.
- W. C. Chen, N. Kawasaki, C. M. Nycholat, S. Han, J. Pilotte, P. R. Crocker and J. C. Paulson, Antigen delivery to macrophages using liposomal nanoparticles targeting sialoadhesin/CD169, PLoS One, 2012, 7, e39039 CrossRef CAS PubMed.
- G. M. Doody, L. B. Justement, C. C. Delibrias, R. J. Matthews, J. Lin, M. L. Thomas and D. T. Fearon, A Role in B Cell Activation for CD22 and the Protein Tyrosine Phosphatase SHP, Science, 1995, 269, 242–244 CrossRef CAS PubMed.
- A. H. Courtneya, E. B. Puffer, J. K. Pontrellob, Z.-Q. Yangb and L. L. Kiessling, Sialylated multivalent antigens engage CD22 in trans and inhibit B cell activation, Proc. Natl. Acad. Sci. U. S. A., 2009, 106, 2500–2505 CrossRef PubMed.
- Z.-Q. Yang, E. B. Puffer, J. K. Pontrello and L. L. Kiessling, Synthesis of a multivalent display of a CD22-binding trisaccharide, Carbohydr. Res., 2002, 337, 1605–1613 CrossRef CAS PubMed.
- J. K. Rhee, M. Hovlid, J. D. Fiedler, S. D. Brown, F. Manzenrieder, H. Kitagishi, C. Nycholat, J. C. Paulson and M. G. Finn, Colorful virus-like particles: fluorescent protein packaging by the Qbeta capsid, Biomacromolecules, 2011, 12, 3977–3981 CrossRef CAS PubMed.
- J. K. Rhee, M. Baksh, C. Nycholat, J. C. Paulson, H. Kitagishi and M. G. Finn, Glycan-targeted virus-like nanoparticles for photodynamic therapy, Biomacromolecules, 2012, 13, 2333–2338 CrossRef CAS PubMed.
- W. C. Chen, G. C. Completo, D. S. Sigal, P. R. Crocker, A. Saven and J. C. Paulson, In vivo targeting of B-cell lymphoma with glycan ligands of CD22, Blood, 2010, 115, 4778–4786 CrossRef CAS PubMed.
- M. S. Macauley, F. Pfrengle, C. Rademacher, C. M. Nycholat, A. J. Gale, A. von Drygalski and J. C. Paulson, Antigenic liposomes displaying CD22 ligands induce antigen-specific B cell apoptosis, J. Clin. Invest., 2013, 123, 3074–3083 CrossRef CAS PubMed.
- F. Pfrengle, M. S. Macauley, N. Kawasaki and J. C. Paulson, Copresentation of antigen and ligands of Siglec-G induces B cell tolerance independent of CD22, J. Immunol., 2013, 191, 1724–1731 CrossRef CAS PubMed.
- H. Zhang, J. Zhao, X. Gu and Y. Wen, Targeted treatment of CD22-positive non-Hodgkin's lymphoma with sialic acid–modified chitosan-PLGA hybrid nanoparticles, J. Nanopart. Res., 2019, 21, 154 CrossRef.
- J. V. Pluvinage, M. S. Haney, B. A. H. Smith, J. Sun, T. Iram, L. Bonanno, L. Li, D. P. Lee, D. W. Morgens, A. C. Yang, S. R. Shuken, D. Gate, M. Scott, P. Khatri, J. Luo, C. R. Bertozzi, M. C. Bassik and T. Wyss-Coray, CD22 blockade restores homeostatic microglial phagocytosis in ageing brains, Nature, 2019, 568, 187–192 CrossRef CAS PubMed.
- L. J. Yang, C. B. Zeller, N. L. Shaper, M. Kiso, A. Hasegawa, R. E. Shapiro and R. L. Schnaar, Gangliosides are neuronal ligands for myelin-associated glycoprotein, Proc. Natl. Acad. Sci. U. S. A., 1996, 93, 814–818 CrossRef CAS PubMed.
- N. Al-Bashir, W. Mellado and M. T. Filbin, Sialic Acid Is Required for Neuronal Inhibition by Soluble MAG but not for Membrane Bound MAG, Front. Mol. Neurosci., 2016, 9, 21 Search PubMed.
- Y. C. Kuo, L. J. Wang and R. Rajesh, Targeting human brain cancer stem cells by curcumin-loaded nanoparticles grafted with anti-aldehyde dehydrogenase and sialic acid: Colocalization of ALDH and CD44, Mater. Sci. Eng., C, 2019, 102, 362–372 CrossRef CAS PubMed.
- J. B. Hu, D. Liu, J. Qi, K. J. Lu, F. Y. Jin, X. Y. Ying, J. You and Y. Z. Du, An E-selectin targeting and MMP-2-responsive dextran-curcumin polymeric prodrug for targeted therapy of acute kidney injury, Biomater. Sci., 2018, 6, 3397–3409 RSC.
- X. L. Xu, W. S. Li, X. J. Wang, Y. L. Du, X. Q. Kang, J. B. Hu, S. J. Li, X. Y. Ying, J. You and Y. Z. Du, Endogenous sialic acid-engineered micelles: a multifunctional platform for on-demand methotrexate delivery and bone repair of rheumatoid arthritis, Nanoscale, 2018, 10, 2923–2935 RSC.
- M. L. Zhu, X. L. Xu, X. J. Wang, N. N. Zhang, K. J. Lu, J. Qi, F. Y. Jin, D. Liu and Y. Z. Du, Sialic-Acid-Anchored Micelles: A Hierarchical Targeting Device for Enhanced Tumor Tissue Accumulation and Cellular Internalization, Mol. Pharm., 2018, 15, 4235–4246 CrossRef CAS PubMed.
- X. L. Xu, M. L. Zhu, D. Liu, G. F. Shu, J. Qi, Y. Lu, F. Wang, X. Y. Ying, J. Chen and Y. Z. Du, Highly Integrated Nanoplatform Based on an E-Selectin-Targeting Strategy for Metastatic Breast Cancer Treatment, Mol. Pharm., 2019, 16, 3694–3702 CrossRef CAS PubMed.
- F. Jin, D. Liu, H. Yu, J. Qi, Y. You, X. Xu, X. Kang, X. Wang, K. Lu, X. Ying, J. You, Y. Du and J. Ji, Sialic Acid-Functionalized PEG-PLGA Microspheres Loading Mitochondrial-Targeting-Modified Curcumin for Acute Lung Injury Therapy, Mol. Pharm., 2019, 16, 71–85 CrossRef CAS PubMed.
- X. L. Xu, G. F. Shu, X. J. Wang, J. Qi, F. Y. Jin, Q. Y. Shen, X. Y. Ying, J. S. Ji and Y. Z. Du, Sialic acid-modified chitosan oligosaccharide-based biphasic calcium phosphate promote synergetic bone formation in rheumatoid arthritis therapy, J. Controlled Release, 2020, 323, 578–590 CrossRef CAS PubMed.
- Y. Du, D. Liu, M. Sun, G. Shu, J. Qi, Y. You, Y. Xu, K. Fan, X. Xu, F. Jin, J. Wang, Q. Shen, L. Zhu, X. Ying, J. Ji, L. Wu, D. Liu and Y. Du, Multifunctional Gd-CuS loaded UCST polymeric micelles for MR/PA imaging-guided chemo-photothermal tumor treatment, Nano Res., 2021, 15, 2288–2299 CrossRef.
- G. Shu, M. Chen, J. Song, X. Xu, C. Lu, Y. Du, M. Xu, Z. Zhao, M. Zhu, K. Fan, X. Fan, S. Fang, B. Tang, Y. Dai, Y. Du and J. Ji, Sialic acid-engineered mesoporous polydopamine nanoparticles loaded with SPIO and Fe(3+) as a novel theranostic agent for T1/T2 dual-mode MRI-guided combined chemo-photothermal treatment of hepatic cancer, Bioact. Mater., 2021, 6, 1423–1435 CrossRef CAS PubMed.
- X.-L. Xu, M.-X. Chen, X.-F. Lou, Y.-Y. Du, G.-F. Shu, J. Qi, M.-L. Zhu, X.-Y. Ying, L. Yu, J.-S. Ji and Y.-Z. Du, Sialic acid-modified mesoporous polydopamine induces tumor vessel normalization to enhance photodynamic therapy by inhibiting VE-cadherin internalization, Chem. Eng. J., 2021, 414, 128743 CrossRef CAS.
- J. A. Kellum and D. Y. Fuhrman, The handwriting is on the wall: there will soon be a drug for AKI, Nat. Rev. Nephrol., 2019, 15, 65–66 CrossRef CAS PubMed.
- M. F. Neurath, Targeting immune cell circuits and trafficking in inflammatory bowel disease, Nat. Immunol., 2019, 20, 970–979 CrossRef CAS PubMed.
- M. Back, A. Yurdagul Jr., I. Tabas, K. Oorni and P. T. Kovanen, Inflammation and its resolution in atherosclerosis: mediators and therapeutic opportunities, Nat. Rev. Cardiol., 2019, 16, 389–406 Search PubMed.
- A. Mantovani, C. A. Dinarello, M. Molgora and C. Garlanda, Interleukin-1 and Related Cytokines in the Regulation of Inflammation and Immunity, Immunity, 2019, 50, 778–795 CrossRef CAS PubMed.
- I. B. McInnes, C. D. Buckley and J. D. Isaacs, Cytokines in rheumatoid arthritis—shaping the immunological landscape, Nat. Rev. Rheumatol., 2016, 12, 63–68 CrossRef CAS PubMed.
- S. Ohira, Y. Asuda, I. Tomita, K. Kitajima, T. Takahashi, C. Sato and H. Tanaka, Synthesis of end-functionalized glycopolymers containing alpha(2,8) disialic acids via pi-allyl nickel catalyzed coordinating polymerization and their interaction with Siglec-7, Chem. Commun., 2017, 53, 553–556 RSC.
- S. Yamaguchi, A. Yoshimura, Y. Yasuda, A. Mori, H. Tanaka, T. Takahashi, K. Kitajima and C. Sato, Chemical Synthesis and Evaluation of a Disialic Acid-Containing Dextran Polymer as an Inhibitor for the Interaction between Siglec 7 and Its Ligand, ChemBioChem, 2017, 18, 1194–1203 CrossRef CAS PubMed.
- C. S. Delaveris, S. H. Chiu, N. M. Riley and C. R. Bertozzi, Modulation of immune cell reactivity with cis-binding Siglec agonists, Proc. Natl. Acad. Sci. U. S. A., 2021, 118, e2012408118 CrossRef CAS PubMed.
- C. S. Delaveris, A. J. Wilk, N. M. Riley, J. C. Stark, S. S. Yang, A. J. Rogers, T. Ranganath, K. C. Nadeau, C. A. Blish and C. R. Bertozzi, Synthetic Siglec-9 Agonists Inhibit Neutrophil Activation Associated with COVID-19, ACS Cent. Sci., 2021, 7, 650–657 CrossRef CAS PubMed.
- D. H. Dube and C. R. Bertozzi, Metabolic oligosaccharide engineering as a tool for glycobiology, Curr. Opin. Chem. Biol., 2003, 7, 616–625 CrossRef CAS PubMed.
- S. Spence, M. K. Greene, F. Fay, E. Hams, S. P. Saunders, U. Hamid, M. Fitzgerald, J. Beck, B. K. Bains, P. Smyth, E. Themistou, D. M. Small, D. Schmid, C. M. O’Kane, D. C. Fitzgerald, S. M. Abdelghany, J. A. Johnston, P. G. Fallon, J. F. Burrows, D. F. McAuley, A. Kissenpfennig and C. J. Scott, Targeting Siglecs with a sialic acid–decorated nanoparticle abrogates inflammation, Sci. Transl. Med., 2015, 7, 303–140 Search PubMed.
- M. Islam, B. M. Arlian, F. Pfrengle, S. Duan, S. A. Smith and J. C. Paulson, Suppressing Immune Responses Using Siglec Ligand-Decorated Anti-receptor Antibodies, J. Am. Chem. Soc., 2022, 144, 9302–9311 CrossRef CAS PubMed.
- M. Ohmae, M. Kojima, K. Mihara, Y. Yamazaki, I. Hara, E. Hara and S. Kimura, Reduced immune response to polymeric micelles coating sialic acids, Bioorg. Med. Chem. Lett., 2016, 26, 4976–4982 CrossRef CAS PubMed.
- D. Bhella, The role of cellular adhesion molecules in virus attachment and entry, Philos. Trans. R. Soc., B, 2015, 370, 20140035 CrossRef PubMed.
- J. Grove and M. Marsh, The cell biology of receptor-mediated virus entry, J. Cell Biol., 2011, 195, 1071–1082 CrossRef CAS PubMed.
- A. M. Krachler, H. Ham and K. Orth, Outer membrane adhesion factor multivalent adhesion molecule 7 initiates host cell binding during infection by Gram-negative pathogens, Proc. Natl. Acad. Sci. U. S. A., 2011, 108, 11614–11619 CrossRef CAS PubMed.
- D. Serrano-Gómez, A. Domínguez-Soto, J. Ancochea, J. A. Jimenez-Heffernan, J. A. Leal and A. L. Corbí, Dendritic Cell-Specific Intercellular Adhesion Molecule 3-Grabbing Nonintegrin Mediates Binding and Internalization of Aspergillus fumigatus Conidia by Dendritic Cells and Macrophages, J. Immunol., 2004, 173, 5635 CrossRef PubMed.
- M. J. S. Mendes-Giannini, C. P. Soares, J. L. M. da Silva and P. F. Andreotti, Interaction of pathogenic fungi with host cells: Molecular and cellular approaches, FEMS Immunol. Med. Microbiol., 2005, 45, 383–394 CrossRef CAS PubMed.
- S. Bhatia, L. C. Camacho and R. Haag, Pathogen Inhibition by Multivalent Ligand Architectures, J. Am. Chem. Soc., 2016, 138, 8654–8666 CrossRef CAS PubMed.
- K. Niikura, K. Nagakawa, N. Ohtake, T. Suzuki, Y. Matsuo, H. Sawa and K. Ijiro, Gold Nanoparticle Arrangement on Viral Particles through Carbohydrate Recognition: A Non-Cross-Linking Approach to Optical Virus Detection, Bioconjugate Chem., 2009, 20, 1848–1852 CrossRef CAS PubMed.
- M. J. Marin, A. Rashid, M. Rejzek, S. A. Fairhurst, S. A. Wharton, S. R. Martin, J. W. McCauley, T. Wileman, R. A. Field and D. A. Russell, Glyconanoparticles for the plasmonic detection and discrimination between human and avian influenza virus, Org. Biomol. Chem., 2013, 11, 7101–7107 RSC.
- Y. Horiguchi, T. Goda, A. Matsumoto, H. Takeuchi, S. Yamaoka and Y. Miyahara, Gold Nanoparticles with Ligand/Zwitterion Hybrid Layer for Individual Counting of Influenza A H1N1 Subtype Using Resistive Pulse Sensing, Langmuir, 2019, 35, 1798–1806 CrossRef CAS PubMed.
- C. Lee, P. Wang, M. A. Gaston, A. A. Weiss and P. Zhang, Plasmonics-Based Detection of Virus Using Sialic Acid Functionalized Gold Nanoparticles, Methods Mol. Biol., 2017, 1571, 109–116 CrossRef CAS PubMed.
- S. J. Richards, A. N. Baker, M. Walker and M. I. Gibson, Polymer-Stabilized Sialylated Nanoparticles: Synthesis, Optimization, and Differential Binding to Influenza Hemagglutinins, Biomacromolecules, 2020, 21, 1604–1612 CrossRef CAS PubMed.
- A. N. Baker, S. J. Richards, C. S. Guy, T. R. Congdon, M. Hasan, A. J. Zwetsloot, A. Gallo, J. R. Lewandowski, P. J. Stansfeld, A. Straube, M. Walker, S. Chessa, G. Pergolizzi, S. Dedola, R. A. Field and M. I. Gibson, The SARS-COV-2 Spike Protein Binds Sialic Acids and Enables Rapid Detection in a Lateral Flow Point of Care Diagnostic Device, ACS Cent. Sci., 2020, 6, 2046–2052 CrossRef CAS PubMed.
- H. A. Alfassam, M. S. Nassar, M. M. Almusaynid, B. A. Khalifah, A. S. Alshahrani, F. A. Almughem, A. A. Alshehri, M. O. Alawad, S. Massadeh, M. Alaamery, I. M. Aldeailej, A. A. Alamri, A. Z. Binjomah and E. A. Tawfik, Development of a Colorimetric Tool for SARS-CoV-2 and Other Respiratory Viruses Detection Using Sialic Acid Fabricated Gold Nanoparticles, Pharmaceutics, 2021, 13 CrossRef CAS PubMed.
- P. G. Georgiou, C. S. Guy, M. Hasan, A. Ahmad, S. J. Richards, A. N. Baker, N. V. Thakkar, M. Walker, S. Pandey, N. R. Anderson, D. Grammatopoulos and M. I. Gibson, Plasmonic Detection of SARS-CoV-2 Spike Protein with Polymer-Stabilized Glycosylated Gold Nanorods, ACS Macro Lett., 2022, 11, 317–322 CrossRef CAS PubMed.
- W. Weis, J. H. Brown, S. Cusack, J. C. Paulson, J. J. Skehel and D. C. Wiley, Structure of the influenza virus haemagglutinin complexed with its receptor, sialic acid, Nature, 1988, 333, 426–431 CrossRef CAS PubMed.
- G. D. Glick and J. R. Knowles, Molecular recognition of bivalent sialosides by influenza virus, J. Am. Chem. Soc., 1991, 113, 4701–4703 CrossRef CAS.
- R. Roy, D. Zanini, S. J. Meunier and A. Romanowska, Solid-phase synthesis of dendritic sialoside inhibitors of influenza A virus haemagglutinin, J. Chem. Soc., Chem. Commun., 1993, 1869–1872 RSC.
- D. Z. A. R. Roy, Synthesis of New α-Thiosialodendrimers and Their Binding Properties to the Sialic Acid Specific Lectin from Limax flavus, J. Am. Chem. Soc., 1997, 119, 2088–2095 CrossRef.
- R. Roy, F. O. Andersson, G. Harms, S. Kelm and R. Schauer, Synthesis of Esterase-resistant 9-O-Acetylated Polysialoside as Inhibitor of Influenza C Virus Hemagglutinin, Angew. Chem., Int. Ed. Engl., 1992, 31, 1478–1481 CrossRef.
- J. Rao, J. Lahiri, R. M. Weis and G. M. Whitesides, Design, Synthesis, and Characterization of a High-Affinity Trivalent System Derived from Vancomycin and l-Lys-d-Ala-d-Ala, J. Am. Chem. Soc., 2000, 122, 2698–2710 CrossRef CAS.
- C. Seok-Ki, M. Mammen and G. M. Whitesides, Poly(acrylic acid) Presenting Sialosides as Side Chains as Polyvalent Inhibitors of Influenza, J. Am. Chem. Soc., 1997, 119, 4103–4111 CrossRef.
- W. J. Lees, A. Spaltenstein, J. E. Kingery-Wood and G. M. Whitesides, Polyacrylamides Bearing Pendant -Sialoside Groups Strongly Inhibit Agglutination of Erythrocytes by Influenza A Virus: Multivalency and Steric Stabilization of Particulate Biological Systems, J. Med. Chem., 1994, 37, 3419–3433 CrossRef CAS PubMed.
- A. Spaltenstein and G. M. Whitesides, Polyacrylamides bearing pendant alpha.-sialoside groups strongly inhibit agglutination of erythrocytes by influenza virus, J. Am. Chem. Soc., 1991, 113, 686–687 CrossRef CAS.
- T. Furuike, S. Aiba, T. Suzuki, T. Takahashi, Y. Suzuki, K. Yamada and S.-I. Nishimura, Synthesis and anti-influenza virus activity of novel glycopolymers having triantennary oligosaccharide branches, J. Chem. Soc., Perkin Trans. 1, 2000, 1, 3000–3005 RSC.
- T. Ohta, N. Miura, N. Fujitani, F. Nakajima, K. Niikura, R. Sadamoto, C.-T. Guo, T. Suzuki, Y. Suzuki, K. Monde and S.-I. Nishimura, Glycotentacles: Synthesis of Cyclic Glycopeptides, Toward a Tailored Blocker of Influenza Virus Hemagglutinin, Angew. Chem., 2003, 115, 5344–5347 CrossRef.
- F. Feng, Y. Sakoda, T. Ohyanagi, N. Nagahori, H. Shibuya, M. Okamastu, N. Miura, H. Kida and S. Nishimura, Novel thiosialosides tethered to metal nanoparticles as potent influenza A virus haemagglutinin blockers, Antiviral Chem. Chemother., 2013, 23, 59–65 CrossRef CAS PubMed.
- I. Papp, C. Sieben, A. L. Sisson, J. Kostka, C. Bottcher, K. Ludwig, A. Herrmann and R. Haag, Inhibition of influenza virus activity by multivalent glycoarchitectures with matched sizes, ChemBioChem, 2011, 12, 887–895 CrossRef CAS PubMed.
- I. Papp, C. Sieben, K. Ludwig, M. Roskamp, C. Bottcher, S. Schlecht, A. Herrmann and R. Haag, Inhibition of influenza virus infection by multivalent sialic-acid-functionalized gold nanoparticles, Small, 2010, 6, 2900–2906 CrossRef CAS PubMed.
- S. Bhatia, M. Hilsch, J. L. Cuellar-Camacho, K. Ludwig, C. Nie, B. Parshad, M. Wallert, S. Block, D. Lauster, C. Bottcher, A. Herrmann and R. Haag, Adaptive Flexible Sialylated Nanogels as Highly Potent Influenza A Virus Inhibitors, Angew. Chem., Int. Ed., 2020, 59, 12417–12422 CrossRef CAS PubMed.
- S. Bhatia, D. Lauster, M. Bardua, K. Ludwig, S. Angioletti-Uberti, N. Popp, U. Hoffmann, F. Paulus, M. Budt, M. Stadtmuller, T. Wolff, A. Hamann, C. Bottcher, A. Herrmann and R. Haag, Linear polysialoside outperforms dendritic analogs for inhibition of influenza virus infection in vitro and in vivo, Biomaterials, 2017, 138, 22–34 CrossRef CAS PubMed.
- S. Bhatia, I. S. Donskyi, S. Block, C. Nie, A. Burdinski, D. Lauster, J. Radnik, A. Herrmann, R. Haag, K. Ludwig and M. Adeli, Wrapping and Blocking of Influenza A Viruses by Sialylated 2D Nanoplatforms, Adv. Mater. Interfaces, 2021, 8, 2100285 CrossRef CAS.
- J. L. Cuellar-Camacho, S. Bhatia, V. Reiter-Scherer, D. Lauster, S. Liese, J. P. Rabe, A. Herrmann and R. Haag, Quantification of Multivalent Interactions between Sialic Acid and Influenza A Virus Spike Proteins by Single-Molecule Force Spectroscopy, J. Am. Chem. Soc., 2020, 142, 12181–12192 CrossRef CAS PubMed.
- M. Ogata, K. I. P. J. Hidari, W. Kozaki, E. Y. Park, T. Suzuki, T. Murata and T. Usui, Molecular Design of Spacer-N-Linked Sialoglycopolypeptide as Polymeric Inhibitors Against Influenza Virus Infection, Biomacromolecules, 2009, 10, 1894–1903 CrossRef CAS PubMed.
- T. H. A. T. Masuda, Synthesis of 4-Guanidino-7-Modified-Neu5Ac2en Derivatives and Their Biological Activities as Influenza Sialidase Inhibitors, J. Synth. Org. Chem., Jpn., 2009, 67, 1005–1114 Search PubMed.
- T. Nohara, A. Imamura, M. Yamaguchi, K. I. Hidari, T. Suzuki, T. Komori, H. Ando, H. Ishida and M. Kiso, Design and synthesis of a novel ganglioside ligand for influenza A viruses, Molecules, 2012, 17, 9590–9620 CrossRef CAS PubMed.
- H. W. Yeh, T. S. Lin, H. W. Wang, H. W. Cheng, D. Z. Liu and P. H. Liang, S-Linked, sialyloligosaccharides bearing liposomes and micelles as influenza virus inhibitors, Org. Biomol. Chem., 2015, 13, 11518–11528 RSC.
- Y. Yang, H. P. Liu, Q. Yu, M. B. Yang, D. M. Wang, T. W. Jia, H. J. He, Y. He, H. X. Xiao, S. S. Iyer, Z. C. Fan, X. Meng and P. Yu, Multivalent S-sialoside protein conjugates block influenza hemagglutinin and neuraminidase, Carbohydr. Res., 2016, 435, 68–75 CrossRef CAS PubMed.
- H. W. Cheng, H. W. Wang, T. Y. Wong, H. W. Yeh, Y. C. Chen, D. Z. Liu and P. H. Liang, Synthesis of S-linked NeuAc-alpha(2-6)-di-LacNAc bearing liposomes for H1N1 influenza virus inhibition assays, Bioorg. Med. Chem., 2018, 26, 2262–2270 CrossRef CAS PubMed.
- T. Tanaka, K. Nakashima, S. Tsuji, X. Han, J. Zhao, Y. Honda, K. Sakakibara, Y. Kurebayashi, T. Takahashi and T. Suzuki, Controlled synthesis of glycopolymers with pendant complex-type sialylglycopeptides and their binding affinity with a lectin and an influenza virus, Polym. Chem., 2019, 10, 5124–5130 RSC.
- C. S. Delaveris, E. R. Webster, S. M. Banik, S. G. Boxer and C. R. Bertozzi, Membrane-tethered mucin-like polypeptides sterically inhibit binding and slow fusion kinetics of influenza A virus, Proc. Natl. Acad. Sci. U. S. A., 2020, 117, 12643–12650 CrossRef CAS PubMed.
- Y. Yu, J. P. Zhou, Y. H. Jin, X. Wang, X. X. Shi, P. Yu, M. Zhong and Y. Yang, Guanidinothiosialoside-Human Serum Albumin Conjugate Mimics mucin Barrier to Restrict Influenza Infection, Int. J. Biol. Macromol., 2020, 162, 84–91 CrossRef CAS PubMed.
- M. Zhong, Y. Yu, J. Q. Song, T. W. Jia, A. Y. Liu, T. F. Zhao, H. J. He, M. B. Yang, W. X. Zhang and Y. Yang, Amide-sialoside protein conjugates as neomucin bioshields prevent influenza virus infection, Carbohydr. Res., 2020, 495, 108088 CrossRef CAS PubMed.
- M. Umemura, Y. Makimura, M. Itoh, T. Yamamoto, T. Mine, S. Mitani, I. Simizu, H. Ashida and K. Yamamoto, One-step synthesis of efficient binding-inhibitor for influenza virus through multiple addition of sialyloligosaccharides on chitosan, Carbohydr. Polym., 2010, 81, 330–334 CrossRef CAS.
- X. Li, P. Wu, G. F. Gao and S. Cheng, Carbohydrate-functionalized chitosan fiber for influenza virus capture, Biomacromolecules, 2011, 12, 3962–3969 CrossRef CAS PubMed.
- M. Yamabe, A. Fujita, K. Kaihatsu and Y. Ebara, Synthesis of neuraminidase-resistant sialoside-modified three-way junction DNA and its binding ability to various influenza viruses, Carbohydr. Res., 2019, 474, 43–50 CrossRef CAS PubMed.
- D. Lauster, S. Klenk, K. Ludwig, S. Nojoumi, S. Behren, L. Adam, M. Stadtmuller, S. Saenger, S. Zimmler, K. Honzke, L. Yao, U. Hoffmann, M. Bardua, A. Hamann, M. Witzenrath, L. E. Sander, T. Wolff, A. C. Hocke, S. Hippenstiel, S. De Carlo, J. Neudecker, K. Osterrieder, N. Budisa, R. R. Netz, C. Bottcher, S. Liese, A. Herrmann and C. P. R. Hackenberger, Phage capsid nanoparticles with defined ligand arrangement block influenza virus entry, Nat. Nanotechnol., 2020, 15, 373–379 CrossRef CAS PubMed.
- J. Chung, Y. Jung, C. Hong, S. Kim, S. Moon, E. A. Kwak, B. J. Hwang, S. H. Park, B. L. Seong, D. H. Kweon and W. J. Chung, Filamentous anti-influenza agents wrapping around viruses, J. Colloid Interface Sci., 2021, 583, 267–278 CrossRef CAS PubMed.
- T. Terabayashi, M. Morita, M. Ueno, T. Nakamura and T. Urashima, Inhibition of influenza-virus-induced cytopathy by sialylglycoconjugates, Carbohydr. Res., 2006, 341, 2246–2253 CrossRef CAS PubMed.
- M. Waldmann, R. Jirmann, K. Hoelscher, M. Wienke, F. C. Niemeyer, D. Rehders and B. Meyer, A nanomolar multivalent ligand as entry inhibitor of the hemagglutinin of avian influenza, J. Am. Chem. Soc., 2014, 136, 783–788 CrossRef CAS PubMed.
- Z.-L. Yang, X.-F. Zeng, H.-P. Liu, Q. Yu, X. Meng, Z.-L. Yan, Z.-C. Fan, H.-X. Xiao, S. S. Iyer, Y. Yang and P. Yu, Synthesis of multivalent difluorinated zanamivir analogs as potent antiviral inhibitors, Tetrahedron Lett., 2016, 57, 2579–2582 CrossRef CAS.
- S. J. Kwon, D. H. Na, J. H. Kwak, M. Douaisi, F. Zhang, E. J. Park, J. H. Park, H. Youn, C. S. Song, R. S. Kane, J. S. Dordick, K. B. Lee and R. J. Linhardt, Nanostructured glycan architecture is important in the inhibition of influenza A virus infection, Nat. Nanotechnol., 2017, 12, 48–54 CrossRef CAS PubMed.
- K. Farabi, Y. Manabe, H. Ichikawa, S. Miyake, M. Tsutsui, K. Kabayama, T. Yamaji, K. Tanaka, S. C. Hung and K. Fukase, Concise and Reliable Syntheses of Glycodendrimers via Self-Activating Click Chemistry: A Robust Strategy for Mimicking Multivalent Glycan-Pathogen Interactions, J. Org. Chem., 2020, 85, 16014–16023 CrossRef CAS PubMed.
- S. Tang, W. B. Puryear, B. M. Seifried, X. Dong, J. A. Runstadler, K. Ribbeck and B. D. Olsen, Antiviral Agents from Multivalent Presentation of Sialyl Oligosaccharides on Brush Polymers, ACS Macro Lett., 2016, 5, 413–418 CrossRef CAS PubMed.
- W. Hai, T. Goda, H. Takeuchi, S. Yamaoka, Y. Horiguchi, A. Matsumoto and Y. Miyahara, Specific Recognition of Human Influenza Virus with PEDOT Bearing Sialic Acid-Terminated Trisaccharides, ACS Appl. Mater. Interfaces, 2017, 9, 14162–14170 CrossRef CAS PubMed.
- M. Nagao, T. Matsubara, Y. Hoshino, T. Sato and Y. Miura, Synthesis of Various Glycopolymers Bearing Sialyllactose and the Effect of Their Molecular Mobility on Interaction with the Influenza Virus, Biomacromolecules, 2019, 20, 2763–2769 CrossRef CAS PubMed.
- Y. S. Ahn, H. J. Baik, B. R. Lee, E. S. Lee, K. T. Oh, D. H. Lee and Y. S. Youn, Preparation of multifunctional polymeric micelles for antiviral treatment, Macromol. Res., 2010, 18, 747–752 CrossRef CAS.
- A. Nazemi, S. M. Haeryfar and E. R. Gillies, Multifunctional dendritic sialopolymersomes as potential antiviral agents: their lectin binding and drug release properties, Langmuir, 2013, 29, 6420–6428 CrossRef CAS PubMed.
- M. Ogata, S. Umemura, N. Sugiyama, N. Kuwano, A. Koizumi, T. Sawada, M. Yanase, T. Takaha, J. I. Kadokawa and T. Usui, Synthesis of multivalent sialyllactosamine-carrying glyco-nanoparticles with high affinity to the human influenza virus hemagglutinin, Carbohydr. Polym., 2016, 153, 96–104 CrossRef CAS PubMed.
- M. Kubota, K. Takeuchi, S. Watanabe, S. Ohno, R. Matsuoka, D. Kohda, S. I. Nakakita, H. Hiramatsu, Y. Suzuki, T. Nakayama, T. Terada, K. Shimizu, N. Shimizu, M. Shiroishi, Y. Yanagi and T. Hashiguchi, Trisaccharide containing alpha2,3-linked sialic acid is a receptor for mumps virus, Proc. Natl. Acad. Sci. U. S. A., 2016, 113, 11579–11584 CrossRef CAS PubMed.
- B. R. Wasik, K. N. Barnard and C. R. Parrish, Effects of Sialic Acid Modifications on Virus Binding and Infection, Trends Microbiol., 2016, 24, 991–1001 CrossRef CAS PubMed.
- M. A. Tortorici, A. C. Walls, Y. Lang, C. Wang, Z. Li, D. Koerhuis, G. J. Boons, B. J. Bosch, F. A. Rey, R. J. de Groot and D. Veesler, Structural basis for human coronavirus attachment to sialic acid receptors, Nat. Struct. Mol. Biol., 2019, 26, 481–489 CrossRef PubMed.
- P. Wielgat, K. Rogowski, K. Godlewska and H. Car, Coronaviruses: Is Sialic Acid a Gate to the Eye of Cytokine Storm? From the Entry to the Effects, Cells, 2020, 9 CrossRef CAS PubMed.
- A. G. Dalgleish, P. C. L. Beverley, P. R. Clapham, D. H. Crawford, M. F. Greaves and R. A. Weiss, The CD4 (T4) antigen is an essential component of the receptor for the AIDS retrovirus, Nature, 1984, 312, 763–767 CrossRef CAS PubMed.
- D. Klatzmann, E. Champagne, S. Chamaret, J. Gruest, D. Guetard, T. Hercend, J.-C. Gluckman and L. Montagnier, T-lymphocyte T4 molecule behaves as the receptor for human retrovirus LAV, Nature, 1984, 312, 767–768 CrossRef CAS PubMed.
- C. Callebaut, B. Krust, E. Jacotot and A. G. Hovanessian, T Cell Activation Antigen, CD26, as a Cofactor for Entry of HIV in CD4+ Cells, Science, 1993, 262, 2045–2050 CrossRef CAS PubMed.
- D.-W. Yang, Y. Ohta, S. Yamaguchi, Y. Tsukada, Y. HaraguchP, H. Hoshino, H. Amagai and I. Kobayashi, Sulfated colominic acid: an antiviral agent that inhibits the human immunodeficiency virus type 1 in vitro, Antiviral Res., 1996, 31, 95–104 CrossRef CAS PubMed.
- M. Elofsson, L. A. Salvador and J. Kihlherg, Preparation of Tn and sialyl Tn building blocks used in Fmoc solid-phase synthesis of glycopeptide fragments from HIV gp120, Terrahedron, 1997, 53, 369–390 CrossRef CAS.
- R. Clayton, J. Hardman, C. C. LaBranche and K. D. McReynolds, Evaluation of the synthesis of sialic acid-PAMAM glycodendrimers without the use of sugar protecting groups, and the anti-HIV-1 properties of these compounds, Bioconjugate Chem., 2011, 22, 2186–2197 CrossRef CAS PubMed.
- C. Vierra, D. K. Eggers, C. C. LaBranche and K. D. McReynolds, An Efficient Microwave-Mediated Synthesis of Hexavalent Sialic Acid Sulfoglycodendrimers as Potential Anti-HIV Agents, ACS Appl. Polym. Mater., 2020, 2, 4345–4351 CrossRef CAS PubMed.
- T. Dalianis and H. H. Hirsch, Human polyomaviruses in disease and cancer, Virology, 2013, 437, 63–72 CrossRef CAS PubMed.
- M. Baier, N. H. Rustmeier, J. Harr, N. Cyrus, G. J. Reiss, A. Grafmuller, B. S. Blaum, T. Stehle and L. Hartmann, Divalent Sialylated Precision Glycooligomers Binding to Polyomaviruses and the Effect of Different Linkers, Macromol. Biosci., 2019, 19, e1800426 CrossRef PubMed.
- R. Caraballo, M. Saleeb, J. Bauer, A. M. Liaci, N. Chandra, R. J. Storm, L. Frangsmyr, W. Qian, T. Stehle, N. Arnberg and M. Elofsson, Triazole linker-based trivalent sialic acid inhibitors of adenovirus type 37 infection of human corneal epithelial cells, Org. Biomol. Chem., 2015, 13, 9194–9205 RSC.
- S. J. L. Petitjean, W. Chen, M. Koehler, R. Jimmidi, J. Yang, D. Mohammed, B. Juniku, M. L. Stanifer, S. Boulant, S. P. Vincent and D. Alsteens, Multivalent 9-O-Acetylated-sialic acid glycoclusters as potent inhibitors for SARS-CoV-2 infection, Nat. Commun., 2022, 13, 2564 CrossRef CAS PubMed.
- C. L. Wardzala, A. M. Wood, D. M. Belnap and J. R. Kramer, Mucins Inhibit Coronavirus Infection in a Glycan-Dependent Manner, ACS Cent. Sci., 2022, 8, 351–360 CrossRef CAS PubMed.
- J. Y. Zhao, W. T. Song, Z. H. Tang and X. S. Chen, Macromolecular Effects in Medicinal Chemistry, Acta Chim. Sin., 2022, 80, 563–569 CrossRef.
|
This journal is © The Royal Society of Chemistry 2023 |