DOI:
10.1039/C9SC05668H
(Edge Article)
Chem. Sci., 2020,
11, 2001-2005
Sulfoxide-mediated oxidative cross-coupling of phenols†
Received
8th November 2019
, Accepted 9th January 2020
First published on 15th January 2020
Abstract
A metal-free, oxidative coupling of phenols with various nucleophiles, including arenes, 1,3-diketones and other phenols, is reported. Cross-coupling is mediated by a sulfoxide which inverts the reactivity of the phenol partner. Crucially, the process shows high selectivity for cross-versus homo-coupling and allows efficient access to a variety of aromatic scaffolds including biaryls, benzofurans and, through an iterative procedure, aromatic oligomers.
Introduction
Metal-catalyzed cross-coupling, involving an aryl halide and an organometallic partner, is a powerful tool for biaryl synthesis (Scheme 1A).1 However, oxidative, C–H/C–H couplings, involving non-prefunctionalized partners, have recently come to the fore as an attractive alternative (Scheme 1B).2 Their development remains a challenge, as the reactivity of one partner must be inverted, and known processes are compromised by the requirement for expensive, supply risk, metal oxidants or metal catalysts.2 The development of selective, metal-free C–H/C–H coupling reactions is, therefore, an important goal.3
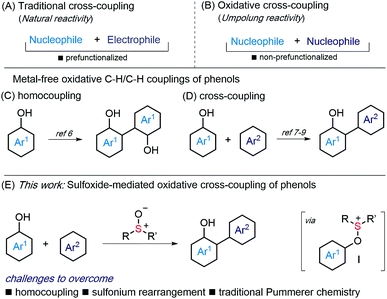 |
| Scheme 1 (A and B) Types of cross-coupling. (C and D) Metal-free, oxidative coupling of phenols. (E) Sulfoxide-mediated, oxidative coupling of phenols. | |
Phenols, in particular unsymmetrical phenol-derived biaryls, are ubiquitous in nature, biomaterials and ligand collections for catalysis.4 Approaches to these compounds generally require multiple steps – prefunctionalization of partners or manipulation of protecting groups – and/or the use of metals.5 Metal-free oxidative coupling of unprotected phenols is therefore of interest, however, avoiding homocoupling is a challenge (Scheme 1C).6 Nevertheless, metal-free cross-coupling of phenols has been described, most notably using electroorganic synthesis7 or hypervalent iodine reagents,8 amongst other approaches9 (Scheme 1D).
We proposed that sulfoxides10,11 could be used to invert the reactivity of a phenol partner, thus providing an alternative approach to their oxidative coupling (Scheme 1E). Capture of phenols by sulfoxides will deliver aryloxysulfonium intermediates I that are electrophilic and capable of coupling with various nucleophiles (e.g. Ar2).12–14 The major challenge in such an approach is the avoidance of homocoupling.13 Furthermore, alternative Pummerer chemistry of the sulfoxide15 and rearrangement of sulfonium intermediates I9a,10,16 must be by-passed.
Here we describe the metal-free, oxidative cross-coupling of phenols with various carbon nucleophilic partners, including other phenols, arenes, and 1,3-diketones (Scheme 1E). Couplings deliver biaryls, 2-aryl 1,3-dicarbonyl compounds and benzofurans. An iterative procedure allows selective double functionalization of phenols and the preparation of aryl oligomers.
Results and discussion
Oxidative cross-coupling of phenols with phenols, phenol derivatives and arenes
Guided by our previous studies, phenol 1a in CH2Cl2 was treated with sulfoxide 4a, activated using trifluoroacetic anhydride (TFAA), before subsequent addition of 2a (1.5 equivalents), to give the product of cross-coupling 3a in 91% isolated yield (see the ESI† for optimisation).
2-Naphthols bearing bromo (3c, 3e, 3h), methoxy (3b), phenyl (3d), cyano (3f) and ester (3g, 3i) groups at the 3-, 6- and 7-positions were found to be compatible with the coupling (Scheme 2). The process also embraced 1-naphthol (3j), phenols (3k–3m) and their methyl ether derivatives (3n–3q). Of particular note, pyrene (3r) underwent coupling with 1a to give 3r. The structure of 3r was confirmed by X-ray crystallographic analysis.17
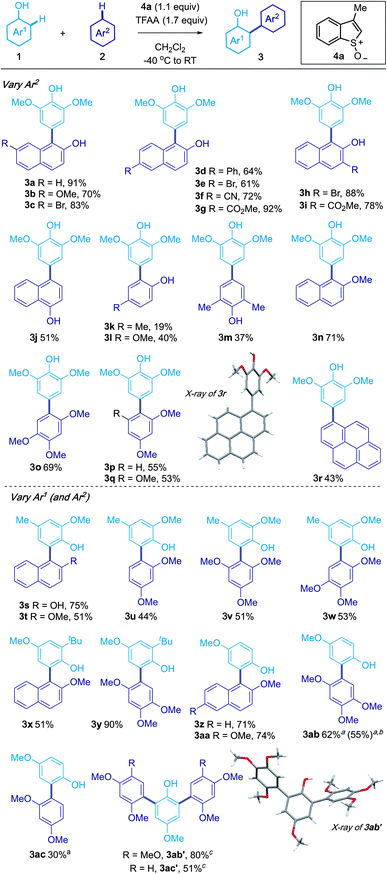 |
| Scheme 2 Oxidative cross-coupling of phenols with phenols, phenol derivatives and arenes. Reaction conditions: to sulfoxide 4a (0.11 mmol) in CH2Cl2 (1 mL, 0.1 M) in an oven-dried tube flushed with N2 at −40 °C was added TFAA (0.17 mmol, 1.7 equiv.). After 5 min, phenol 1 (0.1 mmol in 0.5 mL CH2Cl2) was added in one portion. Arene 2 (0.15 mmol in 0.5 mL CH2Cl2) was then added immediately. After 15 min at −40 °C, the mixture was warmed to room temperature and stirred for 2 h. a CH2Cl2/TFA (1 : 1) as solvent. b Larger scale: (1.2 g of 1 was used). c 2 equiv. of 2 and 2.2 equiv. of 4a. | |
The phenol coupling partner (Ar1) could also be varied and products of ortho-coupling with a range of nucleophilic partners gave products 3s–3ac (30–90% yield). Interestingly, treatment of 4-methoxyphenol with 1,2,4-trimethoxybenzene, under our standard conditions, gave the product of double arylation 3ab′ in 46% yield. The yield of 3ab′ could be increased by using 2.2 equivalent of the sulfoxide 4a and 2.0 equivalents of 1,2,4-trimethoxybenzene (80%). Diarylated compound 3ac′ could also be obtained. Interestingly, the couplings could be tuned to favour products of mono- or bis-coupling; using CH2Cl2/TFA (1
:
1) as solvent favoured formation of the mono-arylated products 3ab and 3ac. Finally, the oxidative coupling could be carried out on a gram scale; the use of 1.2 g of 4-methoxyphenol produced 1.6 g of 3ab (55% isolated yield). In all cross-couplings, 3-methyl benzothiophene was recovered in high yield by chromatography and could be reused.
Oxidative coupling of phenols with 1,3-diketones
1,3-Dicarbonyl compounds could be used as the second nucleophilic partner (Scheme 3). For example, treatment of 1a with 1,3-diphenylpropane-1,3-dione afforded 6a in 85% yield. The products of ortho coupling underwent cyclization to give benzofuran products; for example, the use of 4-methoxyphenol gave aroyl[b]benzofuran186e in 55% isolated yield.
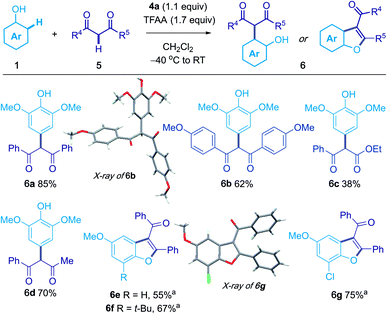 |
| Scheme 3 Oxidative coupling of phenols with 1,3-diketones. Reaction conditions: to sulfoxide 4a (0.11 mmol) in CH2Cl2 (1 mL, 0.1 M) in an oven dried tube flushed with N2 at −40 °C was added TFAA (0.17 mmol, 1.7 equiv.). After 5 min, phenol 1 (0.1 mmol in 0.5 mL CH2Cl2) was added in one portion. 1,3-Dicarbonyl 5 (0.15 mmol in 0.5 mL CH2Cl2) was then added immediately. After 15 min at −40 °C, the mixture was warmed to room temperature and stirred for 2 h. a CH2Cl2/TFA (1 : 1) as solvent. | |
Iterative coupling of three nucleophiles
Intrigued by the formation of the triaryl products 3ab′ and 3ac′ (Scheme 2), we considered an iterative process that would allow the sequential, metal-free, oxidative coupling of phenols with two different nucleophilic partners (Scheme 4). For example, 4-methoxy phenol was first coupled with 1,2,4-trimethoxybenzene to afford 3ab. Subsequent treatment of 3ab with 1,3-dimethoxybenzene gave the unsymmetrical, diarylated phenol 7a in 68% yield. 1,3-Diphenylpropane-1,3-dione could also be used as the third nucleophilic partner and gave C7-arylated benzofurans 7c and 7h.19
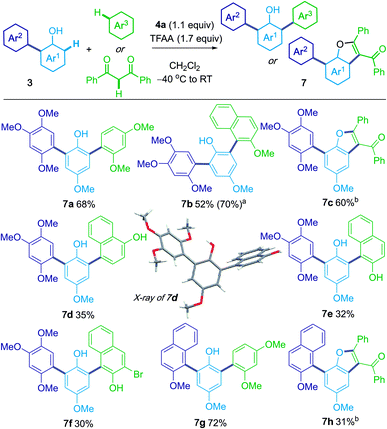 |
| Scheme 4 Iterative coupling of three nucleophiles. Reaction conditions: to sulfoxide 4a (0.11 mmol) in CH2Cl2 (1 mL, 0.1 M) in an oven dried tube flushed with N2 at −40 °C was added TFAA (0.17 mmol, 1.7 equiv.). After 5 min, 3 (0.1 mmol in 0.5 mL CH2Cl2) was added in one portion. A third nucleophile (0.15 mmol in 0.5 mL CH2Cl2) was then added immediately. After 15 min at −40 °C, the mixture was warmed to room temperature and stirred for 2 h. a Compound 3z was used as the substrate. b CH2Cl2/TFA (1 : 1) as solvent. | |
Mechanistic studies
Based on the above results, and our previous studies,10,13 a possible mechanism for the oxidative cross-coupling is shown in Scheme 5A.13 Activation of sulfoxide 4a with TFAA gives acyloxysulfonium salt II and interrupted Pummerer reaction with a phenol coupling partner gives aryloxysulfonium salt I. Subsequent attack of the second partner, at the ortho or para position of the first, results in C–C bond formation and expulsion of 3-methylbenzothiophene. The control experiments in Scheme 5B highlight the important role of the hydroxy group in the first partner and suggest that activation of the phenol occurs via intermediate I. However, we were unable to detect or isolate this intermediate and further studies are needed to confirm the exact mechanism for phenol activation. Scheme 5C shows that the order in which the two nucleophilic partners are combined can be critical, suggesting that rapid and irreversible, aryloxysulfonium salt formation takes place between the activated sulfoxide I and the first phenol partner, and that aryloxysulfonium salt intermediates have very different reactivities.20
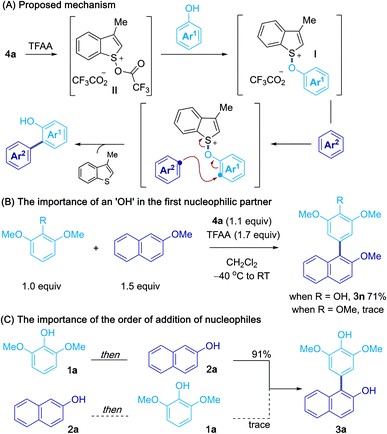 |
| Scheme 5 Proposed mechanism and support for the intermediacy of an aryloxysulfonium salt. | |
Conclusions
In summary, a metal-free, sulfoxide-mediated, oxidative cross-coupling unites phenols and various nucleophilic partners, including phenols, 1,3-diketones and arenes. The capture and inversion of reactivity of the first nucleophilic partner, using an interrupted Pummerer reaction, prior to coupling with the second nucleophile, is key to the cross-coupling. Homocoupling is not observed and alternative Pummerer and rearrangement processes are avoided. Iterative sulfoxide-mediated couplings allow the construction of polyaryl compounds.
Conflicts of interest
There are no conflicts to declare.
Acknowledgements
We thank EPSRC (EP/M005062/1; Postdoctoral Fellowship to Z. H.; Established Career Fellowship to D. J. P.) and The University of Manchester (Lectureship to G. J. P.·P.) for their generous support.
Notes and references
-
(a) C. C. C. Johansson Seechurn, M. O. Kitching, T. J. Colacot and V. Snieckus, Angew. Chem., Int. Ed., 2012, 51, 5062–5085 CrossRef CAS PubMed;
(b) G. Bringmann, R. Walter and R. Weirich, Angew. Chem., Int. Ed., 1990, 29, 977–991 CrossRef;
(c) I. Hussain and T. Singh, Adv. Synth. Catal., 2014, 356, 1661–1696 CrossRef CAS.
-
(a) C. S. Yeung and V. M. Dong, Chem. Rev., 2011, 111, 1215–1292 CrossRef CAS PubMed;
(b) S. H. Cho, J. Y. Kim, J. Kwak and S. Chang, Chem. Soc. Rev., 2011, 40, 5068–5083 RSC;
(c) C. Liu, H. Zhang, W. Shi and A. Lei, Chem. Rev., 2011, 111, 1780–1824 CrossRef CAS PubMed;
(d) C. Liu, J. Yuan, M. Gao, S. Tang, W. Li, R. Shi and A. Lei, Chem. Rev., 2015, 115, 12138–12204 CrossRef CAS PubMed;
(e) Y. Yang, J. Lan and J. You, Chem. Rev., 2017, 117, 8787–8863 CrossRef CAS PubMed;
(f) H. Wang, X. Gao, Z. Lv, T. Abdelilah and A. Lei, Chem. Rev., 2019, 119, 6769–6787 CrossRef CAS PubMed.
-
(a) C.-L. Sun and Z.-J. Shi, Chem. Rev., 2014, 114, 9219–9280 CrossRef CAS PubMed;
(b) R. Narayan, K. Matcha and A. P. Antonchick, Chem.–Eur. J., 2015, 21, 14678–14693 CrossRef CAS PubMed.
-
(a) S. Quideau, D. Deffieux, C. Douat-Casassus and L. Pouységu, Angew. Chem., Int. Ed., 2011, 50, 586–621 CrossRef CAS PubMed;
(b) G. Bringmann, T. Gulder, T. A. M. Gulder and M. Breuning, Chem. Rev., 2011, 111, 563–639 CrossRef CAS PubMed;
(c) D. Parmar, E. Sugiono, S. Raja and M. Rueping, Chem. Rev., 2014, 114, 9047–9153 CrossRef CAS PubMed;
(d) R. Maji, S. C. Mallojjala and S. E. Wheeler, Chem. Soc. Rev., 2018, 47, 1142–1158 RSC;
(e) M. A. Rahim, S. L. Kristufek, S. Pan, J. J. Richardson and F. Caruso, Angew. Chem., Int. Ed., 2019, 58, 1904–1927 CrossRef CAS PubMed;
(f) J. Guo, T. Suma, J. J. Richardson and H. Ejima, ACS Biomater. Sci. Eng., 2019, 5, 5578–5596 CrossRef CAS.
- Z. Huang and J.-P. Lumb, ACS Catal., 2019, 9, 521–555 CrossRef CAS.
-
(a) H. Eickhoff, G. Jung and A. Rieker, Tetrahedron, 2001, 57, 353–364 CrossRef CAS;
(b) G. Bringmann and S. Tasler, Tetrahedron, 2001, 57, 331–343 CrossRef CAS;
(c) C. Boldron, G. Aromí, G. Challa, P. Gamez and J. Reedijk, Chem. Commun., 2005, 5808–5810 RSC;
(d) A. Kirste, M. Nieger, I. M. Malkowsky, F. Stecker, A. Fischer and S. R. Waldvogel, Chem.–Eur. J., 2009, 15, 2273–2277 CrossRef CAS PubMed;
(e) A. Kirste, G. Schnakenburg and S. R. Waldvogel, Org. Lett., 2011, 13, 3126–3129 CrossRef CAS PubMed;
(f) A. Kirste, S. Hayashi, G. Schnakenburg, I. M. Malkowsky, F. Stecker, A. Fischer, T. Fuchigami and S. R. Waldvogel, Chem.–Eur. J., 2011, 17, 14164–14169 CrossRef CAS PubMed.
-
(a) A. Kirste, G. Schnakenburg, F. Stecker, A. Fischer and S. R. Waldvogel, Angew. Chem., Int. Ed., 2010, 49, 971–975 CrossRef CAS PubMed;
(b) A. Kirste, B. Elsler, G. Schnakenburg and S. R. Waldvogel, J. Am. Chem. Soc., 2012, 134, 3571–3576 CrossRef CAS PubMed;
(c) B. Elsler, D. Schollmeyer, K. M. Dyballa, R. Franke and S. R. Waldvogel, Angew. Chem., Int. Ed., 2014, 53, 5210–5213 CrossRef CAS;
(d) A. Wiebe, D. Schollmeyer, K. M. Dyballa, R. Franke and S. R. Waldvogel, Angew. Chem., Int. Ed., 2016, 55, 11801–11805 CrossRef CAS PubMed;
(e) S. Lips, A. Wiebe, B. Elsler, D. Schollmeyer, K. M. Dyballa, R. Franke and S. R. Waldvogel, Angew. Chem., Int. Ed., 2016, 55, 10872–10876 CrossRef CAS PubMed;
(f) A. Wiebe, S. Lips, D. Schollmeyer, R. Franke and S. R. Waldvogel, Angew. Chem., Int. Ed., 2017, 56, 14727–14731 CrossRef CAS PubMed;
(g) S. Lips, D. Schollmeyer, R. Franke and S. R. Waldvogel, Angew. Chem., Int. Ed., 2018, 57, 13325–13329 CrossRef CAS PubMed.
- K. Morimoto, K. Sakamoto, T. Ohshika, T. Dohi and Y. Kita, Angew. Chem., Int. Ed., 2016, 55, 3652–3656 CrossRef CAS PubMed.
- For other selected metal-free C–H/C–H couplings of phenols, see:
(a) S. Sharma, S. K. R. Parumala and R. K. Peddinti, J. Org. Chem., 2017, 82, 9367–9383 CrossRef CAS PubMed;
(b) K. Liu, S. Tang, P. Huang and A. Lei, Nat. Commun., 2017, 8, 775 CrossRef PubMed;
(c) L. Bering, M. Vogt, F. M. Paulussen and A. P. Antonchick, Org. Lett., 2018, 20, 4077–4080 CrossRef CAS PubMed.
- For the reactions of benzothiophene S-oxides with phenols, see:
(a) H. J. Shrives, J. A. Fernández-Salas, C. Hedtke, A. P. Pulis and D. J. Procter, Nat. Commun., 2017, 8, 14801 CrossRef PubMed;
(b) Z. He, H. J. Shrives, J. A. Fernández-Salas, A. Abengózar, J. Neufeld, K. Yang, A. P. Pulis and D. J. Procter, Angew. Chem., Int. Ed., 2018, 57, 5759–5764 CrossRef CAS PubMed;
(c) K. Yang, A. P. Pulis, G. J. P. Perry and D. J. Procter, Org. Lett., 2018, 20, 7498–7503 CrossRef CAS PubMed.
- For examples of interrupted Pummerer and related reactions, see:
(a) T. Kobatake, D. Fujino, S. Yoshida, H. Yorimitsu and K. Oshima, J. Am. Chem. Soc., 2010, 132, 11838–11840 CrossRef CAS PubMed;
(b) K. Murakami, H. Yorimitsu and A. Osuka, Angew. Chem., Int. Ed., 2014, 53, 7510–7513 CrossRef CAS PubMed;
(c) T. Yanagi, S. Otsuka, Y. Kasuga, K. Fujimoto, K. Murakami, K. Nogi, H. Yorimitsu and A. Osuka, J. Am. Chem. Soc., 2016, 138, 14582–14585 CrossRef CAS PubMed;
(d) X. Huang and N. Maulide, J. Am. Chem. Soc., 2011, 133, 8510–8513 CrossRef CAS PubMed;
(e) X. Huang, M. Patil, C. Farès, W. Thiel and N. Maulide, J. Am. Chem. Soc., 2013, 135, 7312–7323 CrossRef CAS PubMed;
(f) B. Peng, D. Geerdink, C. Farès and N. Maulide, Angew. Chem., Int. Ed., 2014, 53, 5462–5466 CrossRef CAS PubMed;
(g) B. Peng, X. Huang, L.-G. Xie and N. Maulide, Angew. Chem., Int. Ed., 2014, 53, 8718–8721 CrossRef CAS PubMed;
(h) D. Kaiser, L. F. Veiros and N. Maulide, Chem.–Eur. J., 2016, 22, 4727–4732 CrossRef CAS PubMed;
(i) D. Kaiser, L. F. Veiros and N. Maulide, Adv. Synth. Catal., 2017, 359, 64–77 CrossRef CAS;
(j) D. Kaldre, I. Klose and N. Maulide, Science, 2018, 361, 664–667 CrossRef CAS PubMed;
(k) A. J. Eberhart and D. J. Procter, Angew. Chem., Int. Ed., 2013, 52, 4008–4011 CrossRef CAS PubMed;
(l) J. A. Fernández-Salas, A. J. Eberhart and D. J. Procter, J. Am. Chem. Soc., 2016, 138, 790–793 CrossRef PubMed;
(m) M. Šiaučiulis, S. Sapmaz, A. P. Pulis and D. J. Procter, Chem. Sci., 2018, 9, 754–759 RSC;
(n) M. H. Aukland, F. J. T. Talbot, J. A. Fernández-Salas, M. Ball, A. P. Pulis and D. J. Procter, Angew. Chem., Int. Ed., 2018, 57, 9785–9789 CrossRef CAS PubMed;
(o) D. Chen, Q. Feng, Y. Yang, X.-M. Cai, F. Wang and S. Huang, Chem. Sci., 2017, 8, 1601–1606 RSC;
(p) M. Šiaučiulis, N. Ahlsten, A. P. Pulis and D. J. Procter, Angew. Chem., Int. Ed., 2019, 58, 8779–8783 CrossRef PubMed;
(q) L. Zhang, J.-N. He, Y. Liang, M. Hu, L. Shang, X. Huang, L. Kong, Z.-X. Wang and B. Peng, Angew. Chem., Int. Ed., 2019, 58, 5316–5320 CrossRef CAS PubMed;
(r) X. Meng, D. Chen, X. Cao, J. Luo, F. Wang and S. Huang, Chem. Commun., 2019, 55, 12495–12498 RSC;
(s) X. Huang, S. Klimczyk and N. Maulide, Synthesis, 2012, 44, 175–183 CAS;
(t) D. Kaiser, I. Klose, R. Oost, J. Neuhaus and N. Maulide, Chem. Rev., 2019, 119, 8701–8780 CrossRef CAS PubMed.
- For examples of interrupted Pummerer reactions involving alkene and alkyne nucleophiles, see;
(a) B. Waldecker, F. Kraft, C. Golz and M. Alcarazo, Angew. Chem., Int. Ed., 2018, 57, 12538–12542 CrossRef CAS PubMed;
(b) Z. Zhang, Y. Luo, H. Du, J. Xu and P. Li, Chem. Sci., 2019, 10, 5156–5161 RSC . See also ref. 11l, 11n and 11p.
- For the homocoupling of 2-naphthols using a benzothiophene catalyst, see: Z. He, A. P. Pulis and D. J. Procter, Angew. Chem., Int. Ed., 2019, 58, 7813–7817 CrossRef CAS PubMed.
- A cross-coupling procedure was low yielding and not general, see: K. Higuchi, T. Tago, Y. Kokubo, M. Ito, M. Tayu, S. Sugiyama and T. Kawasaki, Org. Chem. Front., 2018, 5, 3219–3225 RSC.
- L. H. S. Smith, S. C. Coote, H. F. Sneddon and D. J. Procter, Angew. Chem., Int. Ed., 2010, 49, 5832–5844 CrossRef CAS PubMed.
- T. Yanagi, K. Nogi and H. Yorimitsu, Tetrahedron Lett., 2018, 59, 2951–2959 CrossRef CAS.
- X-ray crystallographic data for 3r, 3ab′, 6b, 6g, and 7d can be found at the Cambridge Crystallographic Data Centre (CCDC); 1944706–1944710.†.
- 3-Aroyl[b]benzofurans are found in bioactive molecules.
(a) T. Kálai, G. Várbiró, Z. Bognár, A. Pálfi, K. Hantó, B. Bognár, E. Ősz, B. Sümegi and K. Hideg, Bioorg. Med. Chem., 2005, 13, 2629–2636 CrossRef PubMed;
(b) C. C. Felder, K. E. Joyce, E. M. Briley, M. Glass, K. P. Mackie, K. J. Fahey, G. J. Cullinan, D. C. Hunden, D. W. Johnson, M. O. Chaney, G. A. Koppel and M. Brownstein, J. Pharmacol. Exp. Ther., 1998, 284, 291–297 CAS;
(c) R. C. Heel, R. N. Brogden, T. M. Speight and G. S. Avery, Drugs, 1977, 14, 349–366 CrossRef CAS PubMed.
- In some cases, when lower yields were obtained (e.g. formation of 7d, 7e, 7f, 7h), unreacted starting material was recovered (10–15% of the phenol 3 and 20–35% of the other coupling partner – naphthol or diketone) and some decomposition took place (side products could not be isolated).
- When reversing the order of addition of the coupling partners (Scheme 5C, bottom), the phenol could be recovered (85% recovery), however, only a trace of the naphthol component was observed. We have been unable to detect any other side products and are currently investigating possible decomposition pathways.
Footnote |
† Electronic supplementary information (ESI) available: Full experimental details, NMR spectra, CCDC numbers for X-ray structures. CCDC 1944706–1944710. For ESI and crystallographic data in CIF or other electronic format see DOI: 10.1039/c9sc05668h |
|
This journal is © The Royal Society of Chemistry 2020 |
Click here to see how this site uses Cookies. View our privacy policy here.