DOI:
10.1039/C9SC02323B
(Edge Article)
Chem. Sci., 2019,
10, 7690-7694
Reactive oxygen species-triggered off-on fluorescence donor for imaging hydrogen sulfide delivery in living cells†
Received
13th May 2019
, Accepted 8th July 2019
First published on 10th July 2019
Abstract
Hydrogen sulfide (H2S), an important gasotransmitter, can mediate a variety of pathophysiological processes, and H2S-based donors have been intensively explored for the therapy of cardiovascular injury, nerve damage and intestinal disorders. However, most of the H2S donors are not capable of simultaneously real-time tracking intracellular H2S delivery, which limits their biological application for elucidating the specific function of H2S. Herein we develop the first reactive oxygen species (ROS)-triggered off-on fluorescence H2S donor (NAB) by incorporating ROS-responsive arylboronate into a fluorophore through thiocarbamate. The donor NAB can release carbonyl sulfide (COS) and the fluorophore with a fluorescence off-on response via a ROS-triggered self-immolative reaction, and then COS is quickly converted to H2S by the ubiquitous carbonic anhydrase. This dual function makes NAB suitable for not only in situ and real-time monitoring of the intracellular H2S release but also rescuing RAW264.7 cells from the hazardous oxidative environment under the stimulation of phorbol-12-myristate-13-acetate, revealing the possible potential of NAB as a therapeutic prodrug with the fluorescence imaging capacity.
Introduction
Hydrogen Sulfide (H2S) is an important gasotransmitter, which can mediate a variety of physiological and pathological processes. Recent studies have demonstrated that H2S is associated with iron homeostasis,1 vasodilation2 and neurotransmission.3 Modulation of H2S levels is suggested to have potential therapeutic value. However, further understanding the biological function of H2S remains an open challenge due to the lack of effective tools to modulate and monitor cellular H2S levels. Some inorganic sulfide salts (NaSH, Na2S, etc) and commercially available GYY4137 can be used for H2S delivery, but they suffer from uncontrollable releasing efficiency and imaging inability, which limits their application in cells. So far, some synthetic donors have been reported,4 which can be activated by controllable manners, such as pH modulation,5 cellular amines,6 thiols,7 enzymes,8 light,9 bio-orthogonal reaction,10 and reactive oxygen species (ROS).11 Despite the great progresses in researching the biological roles of H2S, these donors always need additional analytical methods to verify H2S release. Fluorescence spectroscopy has attracted much attention because of its great temporal and spatial sampling capability as well as high sensitivity.12 We envision that it should be highly favorable for in situ and real-time monitoring of H2S release in complex biological systems if a controllable H2S donor can release H2S accompanied by fluorescence change. However, such H2S donors are still rare.
Herein, we design the first ROS-triggered fluorescence H2S donor (NAB; Fig. 1A), which is constructed by linking ROS-responsive arylboronate to the fluorophore of 3-amino-N-butyl-1,8-naphthalimide (NAH) with thiocarbamate (Scheme S1; Fig. S1–S6†). NAB itself emits rather weak fluorescence; upon reaction with ROS, the donor NAB releases carbonyl sulfide (COS) and the strongly fluorescent NAH via a self-immolative reaction. Then COS is quickly transformed to H2S by the ubiquitous enzyme of carbonic anhydrase (CA). As a result, H2S delivery is achieved by NAB, concomitant with an off-on fluorescence, which can be used for in situ and real time monitoring of H2S release in living biosystems such as cells.
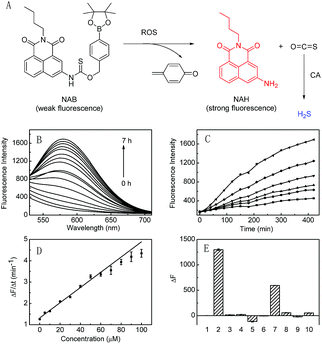 |
| Fig. 1 (A) Response mechanism of H2S donation from NAB with ROS. (B) Fluorescence spectra of NAB (10 μM) with H2O2 (100 μM) at varied time in phosphate buffer (20 mM, pH 7.4). (C) Fluorescence kinetic curves of NAB (10 μM) with varied H2O2 (from bottom to top): 0 (control), 5, 10, 20, 50, 100 μM. (D) Linear fitting curve of the initial rate of fluorescence intensity changes toward the concentration of H2O2 in the range of 0–100 μM. (E) Fluorescence changes of NAB (10 μM) in the presence of different ROS species: (1) NAB only; (2) H2O2 (100 μM); (3) NO (100 μM); (4) OCl− (100 μM); (5) ˙OH (100 μM); (6) 1O2 (100 μM); (7) O2˙− (100 μM); (8) TBHP (100 μM); (9) TBO˙ (100 μM); (10) ONOO− (10 μM). λex/em = 405/577 nm. | |
Results and discussion
Spectroscopic response of NAB to ROS
The reaction of NAB with H2O2 was investigated in the phosphate buffer (20 mM, pH 7.4). NAB displayed a maximum absorption at 300 nm, and the corresponding fluorophore NAH displayed two absorption peaks at 346 nm and 405 nm (Fig. S7A†). However, addition of H2O2 to NAB caused a remarkable decrease of the absorption peak at 300 nm, accompanying the formation of a spectrum similar to that of NAH (Fig. S7A†). Moreover, NAB itself produced faint fluorescence signal under the excitation wavelength of 405 nm, and its reaction solution with H2O2 showed a fluorescence emission peak at 577 nm, which is in agreement with the fluorescence spectrum of NAH (Fig. S7B†). The reaction products of NAB with H2O2 were further analyzed by mass spectrum. As depicted in Fig. S8,† the ESI-MS spectrum of the reaction solution shows a mass peak at m/z = 267.2 ([M–H]−), which is the same as that of NAH. The above results clearly indicate that the reaction of NAB with H2O2 yields the strongly fluorescent NAH.
It was noted that the conversion of NAB to NAH was incomplete even after 7 h according to the spectra of NAH (Fig. S7†). Furthermore, the time-dependent fluorescence responses of NAB to varied H2O2 also revealed that NAB could be used as a controllable H2S donor by H2O2 (Fig. 1). The initial rate of fluorescence increase at varied concentrations of H2O2 displayed good linearity with an equation of ΔF/Δt = 0.0357 × [H2O2] (μM) + 1.32 (R = 0.990) in the range of 0–100 μM H2O2 (Fig. 1D). The lowest triggering concentration of H2O2 that NAB needed was 2.21 μM (k = 3), which was higher than the reported physiological concentration (usually ≤0.7 μM) of intracellular H2O2,13 implying that NAB was probably unable to deliver H2S at the normal level of intracellular H2O2 with the exception of some situations over 2.21 μM H2O2 caused by other stimulations.
The effects of pH and temperature were also studied. As shown in Fig. S9,† the reaction between NAB and H2O2 remains inert under the acidic environment, but the fluorescence intensity is dramatically increased along with the rise in pH value. It might be due to the fact that alkaline media could accelerate the hydrolysis of the aryl-boronic esters to the corresponding phenols.14 Although higher temperature was favorable for the reaction, NAB also worked effectively under the physiological conditions (37 °C, pH 7.4).
Then we tested the fluorescent response of NAB to various ROS. As depicted in Fig. 1E, NAB exhibits the strongest response to H2O2 over the other ROS, among which O2˙− showed some response because it is the precusor of H2O2.15 This indicates that ROS, in particular their representative H2O2 (the most abundant one in cells),16 can effectively trigger the H2S release of NAB. The selectivity of NAB was examined over other potential interfering species in living cells, such as inorganic salts (KCl, MgCl2, CaCl2, ZnCl2), glucose, vitamin C, glutathione and amino acids (glycine, cysteine, lysine, glutamic acid and alanine). As shown in Fig. S10,† NAB displays high selectivity for H2O2 over the other tested species.
Methylene blue colorimetric method for H2S detection
To verify if the decomposed product of NAB could be converted to H2S in the presence of CA, the well-known methylene blue method11 was used to measure the H2S generation, by which the generation of H2S can be proved by the formation of methylene blue that has a characteristic absorption peak at 670 nm. As shown in Fig. S11,† the mixture of the MB solution and the NAB solution with H2O2 indeed produces an apparent absorption peak at 670 nm, clearly indicating the H2S release by NAB. However, when acetazolamide, a CA inhibitor, was added to the reaction system, the absorbance at 670 nm was significantly suppressed, revealing that the H2S release of NAB was dependent on CA in the COS-to-H2S conversion.
Based on the H2S calibration curve (Fig. S11B†), 10, 20 and 30 μM of NAB could generate 2.3, 3.6 and 5.0 μM of H2S, respectively, within 7 h, revealing a non-quantitative H2S release of NAB. The reason for this phenomenon may be complicated, but H2O2 may be one of the influence factors. As shown in Fig. S12,† more H2O2 (>100 μM) can cause a significant decrease of absorbance at 670 nm. Thus, a further study on H2O2 effect was made. The influence of H2O2 on MB itself was excluded first (Fig. S13†). Then, 10 μM Na2S solution was mixed with varied H2O2 (100–300 μM) for 0.5 h and the mixture was transferred to the MB solution for measurement. As depicted in Fig. S14,† the MB formation is inhibited significantly, and this inhibition is H2O2- and time-dependent. For instance, prolonging the reaction time between Na2S and 300 μM H2O2 to 7 h resulted in the decrease of absorbance at 670 nm nearly to zero (Fig. S14†), which suggested that H2O2 may deplete H2S in this case, probably via an oxidizing action. Conversely, such an action means that the released H2S from NAB may scavenge H2O2, thereby relieving the oxidative stress.
Cytotoxicity of NAB
The toxicity of NAB was evaluated with HeLa and RAW264.7 cells (these two kinds of cell lines were obtained from Nanjing KeyGEN BioTECH Co., LTD, China) by standard MTT assay. As shown in Fig. S15†,† cell viability of both cell lines is not significantly affected by the incubation with NAB for 24 h, suggesting the good biocompatibility of the donor.
Fluorescence imaging of H2S release in living cells
As mentioned above, reaction of NAB with H2O2 can produce fluorescence off-on response, thus this behavior was investigated to image the H2S release from the donor in living cells. As shown in Fig. S16,† the NAB-loaded HeLa cells scarcely exhibit fluorescence, but display a gradually increased fluorescence with time upon addition of H2O2. Moreover, the fluorescence became much brighter when the concentration of H2O2 was elevated from 50 μM to 100 μM (Fig. 2). Nevertheless, after pretreated with NAC (an antioxidant to scavenge H2O2), the cells exhibited significantly decreased fluorescence (Fig. 2B). These results demonstrate the triggering ability of ROS in the fluorescence signal of NAB in cells.
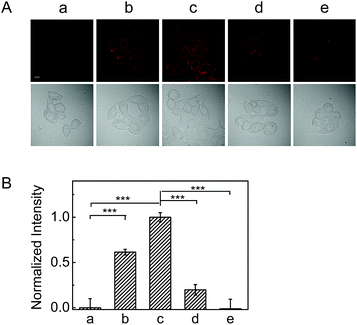 |
| Fig. 2 (A) Confocal fluorescence images of HeLa cells. Cells incubated with NAB (10 μM) for 4 h in the presence of (a) 0, (b) 50, and (c) 100 μM H2O2; cells pretreated with H2O2 (100 μM) in the presence of (d) 100 μM and (e) 1 mM NAC for 10 min, and then incubated with NAB (10 μM) for 4 h. Scale bar: 10 μm. The second row is the corresponding differential interference contrast (DIC) images. (B) The normalized intensity of the corresponding images in panel A [the pixel intensity is obtained by subtracting that (control) from image a; the fluorescence intensity from image c is defined as 1.0]. The results are the mean ± standard deviation of five cells. ***p < 0.001, two-sided Student's t-test. | |
To verify the intracellular ROS-triggered H2S release, a commercially available probe (WSP-1), whose fluorescence can be selectively turned on by H2S,17 was used for the detection of H2S in HeLa cells. As shown in Fig. S17 (images c–e),† the fluorescence of WSP-1 in the green channel rises with increasing the dose of NAB in the presence of H2O2, suggesting the generation of the gradually increased H2S. However, the fluorescence signal was largely attenuated when the cells were incubated with much more H2O2 (Fig. S18†), which is consistent with the aforementioned MB test results, that is, the released H2S from NAB may be consumed by excess H2O2. These results validated NAB as the fluorescence donor for H2S delivery, and suggested that our dual-functional donor could be used as a fluorescent probe for real time monitoring of the H2S release in cells.
H2S release by endogenous ROS in living cells
To evaluate the ability of NAB to release H2S via endogenous ROS in living cells, RAW264.7 cells were chosen as a model because they are believed to generate a considerable amount of ROS in inflammatory pathology induced by PMA.18 As shown in Fig. 3, RAW264.7 cells pretreated with PMA for 1 h and then with NAB for 4 h display a remarkable fluorescence increase when compared to the control group without PMA, and the fluorescence further increases with the treatment of more PMA. This indicates that NAB can release H2S via the endogenous ROS. Similarly, such a fluorescence increase can be efficiently inhibited by NAC, which is consistent with the above results in HeLa cells. Taking together, NAB may be capable of releasing H2S under pathological ROS levels and in situ imaging this event in cells.
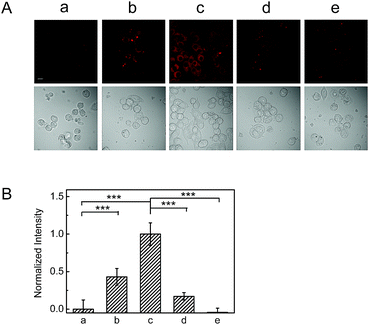 |
| Fig. 3 (A) Confocal fluorescence images of RAW264.7 cells under different conditions. (a) Cells incubated with NAB (10 μM) for 4 h; cells pre-treated with (b) 0.5 and (c) 1 μg mL−1 PMA for 1 h and then incubated with NAB (10 μM) for 4 h; cells pre-treated with PMA (1 μg mL−1) in the presence of (d) 100 μM and (e) 1 mM NAC for 1 h and then incubated with NAB (10 μM) for 4 h. Scale bar: 10 μm. (B) Normalized intensity of the fluorescence images in panel A [the pixel intensity is obtained by subtracting that (control) from image a; the fluorescence intensity from image c is defined as 1.0]. The results are presented as mean ± standard deviation of five cells. ***p < 0.001, two-sided Student's t-test. | |
Protective effect of NAB against cellular inflammation
Since PMA induced inflammation can promote apoptosis through excess oxidative stress in cells, and H2S has been discovered to alleviate inflammatory response effectively, as a proof of concept, we investigated the protective effect of NAB against inflammation on RAW264.7 cells. As shown in Fig. 4A, after cells are exposed to varied concentrations of PMA for 1 h, the MTT assay manifests that 1 μg mL−1 PMA could lead to an about 25% decrease in the cell viability as compared to the control. However, the cells exposed to PMA can significantly recover their viability after they are incubated with NAB, and this viability recovery is increased as the dose of NAB rises. A negative control experiment was also conducted by treating cells with NAH under the same PMA conditions (Fig. S19†), which demonstrates that NAH cannot protect RAW264.7 cells from the stimulation of PMA. Moreover, the ROS scavenging capacity (an anti-inflammatory behavior) of NAB was observed via monitoring the intracellular ROS change with a commercial dye CRDR (Fig. S20†). All the above findings disclose that NAB could rescue RAW264.7 cells from PMA induced cellular inflammation possibly via scavenging H2O2, and might serve as a potential therapeutic H2S donor.
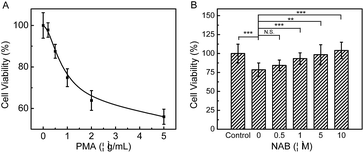 |
| Fig. 4 Cell viability of RAW264.7 cells. (A) Cells treated with PMA at varied concentrations for 1 h; (B) cells treated with PMA (1 μg mL−1) for 1 h and then incubated with NAB at varied concentrations for 4 h. Control is the untreated cells. The results are the mean ± standard deviation of five separate measurements. ***p < 0.001, **p < 0.01, N.S.: no significance, p > 0.01, two-sided Student's t-test. | |
Conclusions
In summary, we have reported NAB as the first ROS-triggered off-on fluorescent H2S donor. H2S release from NAB was detected in both aqueous buffer and live cells. Unlike the previous reported H2S donors, NAB can achieve H2S release with a concomitant off-on fluorescence after activated by ROS. More importantly, using NAB, we demonstrated the real-time imaging of H2S delivery and the cytoprotection against ROS in RAW264.7 cells. The developed fluorescent donor may find a wide use in the delivery and real-time monitoring studies of H2S in some biosystems.
Conflicts of interest
There are no conflicts to declare.
Acknowledgements
This work is supported by grants from the NSF of China (No. 21820102007, 21675159, 21535009, 91732104, 21775152, 21435007 and 21621062), the Chinese Academy of Sciences (Grant XDB14030102), and Youth Innovation Promotion Association of CAS (2016027).
Notes and references
- M. W. Zhang, G. Yang, Y. F. Zhou, C. Qian, M. D. Mu, Y. Ke and Z. M. Qian, J. Cell. Physiol., 2019, 234, 3158–3169 CrossRef CAS.
-
(a) L. Li, M. Whiteman, Y. Y. Guan, K. L. Neo, Y. Cheng, S. W. Lee, Y. Zhao, R. Baskar, C. H. Tan and P. K. Moore, Circulation, 2008, 117, 2351–2360 CrossRef CAS;
(b) M. Kuksis, P. M. Smith and A. V. Ferguson, PLoS One, 2014, 9, e105772 CrossRef.
- A. P. Lan, X. X. Liao, L. Q. Mo, C. T. Yang, Z. L. Yang, X. Y. Wang, F. Hu, P. X. Chen, J. Q. Feng, D. D. Zheng and L. C. Xiao, PLoS One, 2011, 6, e25921 CrossRef CAS.
-
(a) C. Szabo and A. Papapetropoulos, Pharmacol. Rev., 2017, 69, 497–564 CrossRef CAS;
(b) Y. Zhao, T. D. Biggs and M. Xian, Chem. Commun., 2014, 50, 11788–11805 RSC.
-
(a) J. M. Kang, Z. Li, C. L. Organ, C. M. Park, C. T. Yang, A. Pacheco, D. F. Wang, D. J. Lefer and M. Xian, J. Am. Chem. Soc., 2016, 138, 6336–6339 CrossRef CAS;
(b) Y. Zhao, A. K. Steiger and M. D. Pluth, Angew. Chem., Int. Ed., 2018, 57, 13101–13105 CrossRef CAS.
- C. R. Powell, J. C. Foster, B. Okyere, M. H. Theus and J. B. Matson, J. Am. Chem. Soc., 2016, 138, 13477–13480 CrossRef CAS.
-
(a) Y. Zhao, A. K. Steiger and M. D. Pluth, Chem. Commun., 2018, 54, 4951–4954 RSC;
(b) Y. Zhao, H. Wang and M. Xian, J. Am. Chem. Soc., 2011, 133, 15–17 CrossRef CAS;
(c) Y. Zhao, M. M. Cerda and M. D. Pluth, Chem. Sci., 2019, 10, 1873–1878 RSC;
(d) Y. Zhao, S. Bhushan, C. T. Yang, H. Otsuka, J. D. Stein, A. Pacheco, B. Peng, N. O. Devarie-Baez, H. C. Aguilar, D. J. Lefer and M. Xian, ACS Chem. Biol., 2013, 8, 1283–1290 CrossRef CAS PubMed;
(e) Y. Zhao, C. T. Yang, C. Organ, Z. Li, S. Bhushan, H. Otsuka, A. Pacheco, J. M. Kang, H. C. Aguilar, D. J. Lefer and M. Xian, J. Med. Chem., 2015, 58, 7501–7511 CrossRef CAS.
-
(a) Y. Q. Zheng, B. C. Yu, Z. Li, Z. N. Yuan, C. L. Organ, R. K. Trivedi, S. M. Wang, D. J. Lefer and B. H. Wang, Angew. Chem., Int. Ed., 2017, 56, 11749–11753 CrossRef CAS;
(b) A. K. Steiger, M. Marcatti, C. Szabo, B. Szczesny and M. D. Pluth, ACS Chem. Biol., 2017, 12, 2117–2123 CrossRef CAS PubMed.
-
(a) N. O. Devarie-Baez, P. E. Bagdon, B. Peng, Y. Zhao, C. M. Park and M. Xian, Org. Lett., 2013, 15, 2786–2789 CrossRef CAS;
(b) J. J. Woods, J. Cao, A. R. Lippert and J. J. Wilson, J. Am. Chem. Soc., 2018, 140, 12383–12387 CrossRef CAS;
(c) Y. Zhao, S. G. Bolton and M. D. Pluth, Org. Lett., 2017, 19, 2278–2281 CrossRef CAS.
- A. K. Steiger, Y. Yang, M. Royzen and M. D. Pluth, Chem. Commun., 2017, 53, 1378–1380 RSC.
-
(a) P. Chauhan, S. Jos and H. Chakrapani, Org. Lett., 2018, 20, 3766–3770 CrossRef CAS;
(b) Y. Zhao, H. A. Henthorn and M. D. Pluth, J. Am. Chem. Soc., 2017, 139, 16365–16376 CrossRef CAS;
(c) Y. Zhao and M. D. Pluth, Angew. Chem., Int. Ed., 2016, 55, 14638–14642 CrossRef CAS;
(d) M. D. Hartle and M. D. Pluth, Chem. Soc. Rev., 2016, 45, 6108–6117 RSC.
-
(a) Y. C. Chen, C. C. Zhu, J. J. Cen, Y. Bai, W. J. He and Z. J. Guo, Chem. Sci., 2015, 6, 3187–3194 RSC;
(b) L. W. He, X. L. Yang, K. X. Xu, X. Q. Kong and W. Y. Lin, Chem. Sci., 2017, 8, 6257–6265 RSC;
(c) S. Xu, H. W. Liu, X. Yin, L. Yuan, S. Y. Huan and X. B. Zhang, Chem. Sci., 2019, 10, 320–325 RSC;
(d) X. H. Li, X. H. Gao, W. Shi and H. M. Ma, Chem. Rev., 2014, 114, 590–659 CrossRef CAS PubMed;
(e) Z. Q. Mao, H. Jiang, Z. Li, C. Zhong, W. Zhang and Z. H. Liu, Chem. Sci., 2017, 8, 4533–4538 RSC;
(f) L. Y. Wu, Y. D. Sun, K. Sugimoto, Z. L. Luo, Y. Ishigaki, K. Y. Pu, T. Suzuki, H. Y. Chen and D. J. Ye, J. Am. Chem. Soc., 2018, 140, 16340–16352 CrossRef CAS PubMed;
(g) B. Shi, Q. L. Yan, J. Tang, K. Xin, J. C. Zhang, Y. Zhu, G. Xu, R. C. Wang, J. Chen, W. Gao, T. L. Zhu, J. Y. Shi, C. H. Fan, C. C. Zhao and H. Tian, Nano Lett., 2018, 18, 6411–6416 CrossRef CAS.
- D. J. R. Stone and S. Yang, Antioxid. Redox Signaling, 2006, 8, 243–270 CrossRef PubMed.
- L. C. Lo and C. Y. Chu, Chem. Commun., 2003, 2728–2729 RSC.
-
(a) R. H. Burdon, Free Radical Biol. Med., 1995, 18, 775–794 CrossRef CAS;
(b) M. Abo, Y. Urano, K. Hanaoka, T. Terai, T. Komatsu and T. Nagano, J. Am. Chem. Soc., 2011, 133, 10629–10637 CrossRef CAS PubMed.
-
(a) M. C. Y. Chang, A. Pralle, E. Y. Isacoff and C. J. Chang, J. Am. Chem. Soc., 2011, 133, 15–17 CrossRef;
(b) M. Giorgio, M. Trinei, E. Migliaccio and P. G. Pelicci, Nat. Rev. Mol. Cell Biol., 2007, 8, 722–728 CrossRef CAS;
(c) J. Liu, J. Ren, X. J. Bao, W. Gao, C. L. Wu and Y. B. Zhao, Anal. Chem., 2016, 88, 5865–5870 CrossRef CAS.
- C. R. Liu, J. Pan, S. Li, Y. Zhao, L. Y. Wu, C. E. Berkman, A. R. Whorton and M. Xian, Angew.
Chem., Int. Ed., 2011, 50, 10327–10329 CrossRef CAS.
- J. Xu, Y. Zhang, H. Yu, X. D. Gao and S. J. Shao, Anal. Chem., 2016, 88, 1455–1461 CrossRef CAS.
Footnote |
† Electronic supplementary information (ESI) available. See DOI: 10.1039/c9sc02323b |
|
This journal is © The Royal Society of Chemistry 2019 |
Click here to see how this site uses Cookies. View our privacy policy here.