DOI:
10.1039/C4QO00161C
(Review Article)
Org. Chem. Front., 2014,
1, 1225-1252
Recent studies on the chemical constituents of Trigonostemon plants
Received
30th May 2014
, Accepted 5th October 2014
First published on 6th October 2014
Abstract
The plants of the Trigonostemon genus (Euphorbiaceae family) comprising about 50 species are mainly distributed in tropical and subtropical Asia. Chemical studies on this plant genus have led to the discovery of about 200 structurally diverse compounds in the last two decades, some of which have shown promising biological activities. Diterpenoids and alkaloids are the major and most important components of Trigonostemon plants, and are also the hot topics in synthetic chemistry. This review was attempted to provide the timely and comprehensive coverage of the chemical and biological studies on Trigonostemon plants, and especially to focus on the recent research progress.
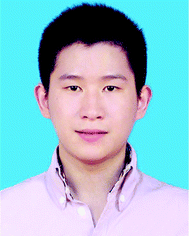 Jin-Biao Xu | Jin-Biao Xu was born in Fujian, China, in 1985. He received his B.S. degree in Pharmaceutical Engineering from Jiangsu University in 2008. In 2009, he joined the research group of Professor Jian-Min Yue as a Ph.D. candidate at Shanghai Institute of Materia Medica (SIMM), Chinese Academy of Sciences (CAS), where he worked for his Ph.D. dissertation in natural products chemistry, and published 5 papers in peer-reviewed journals. |
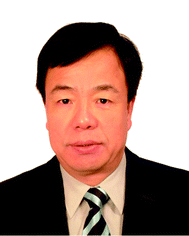 Jian-Min Yue | Jian-Min Yue is a full-time Professor in Medicinal Chemistry at Shanghai Institute of Materia Medica (SIMM), CAS. He received his B.Sc. degree in Chemistry in 1984 from Lanzhou University, where he received his Ph.D. degree in Organic Chemistry in 1990. After his post-doctoral research at Kunming Institute of Botany (KIB), CAS (1991–1993), and University of Bristol (1993–1994), he worked at KIB as an Associate Professor (1994–1996). He joined the staff of the Joint Laboratory of Unilever Research and the Shanghai Institute of Organic Chemistry, CAS, as a Senior Research Scientist (1996–1999) in Natural Products Chemistry. He then moved to SIMM where he has been working up to now. During his research career, he has published over 250 scientific papers, book chapters, and patents. He currently serves as an associate editor of Natural Products and Bioprospecting, and is an editorial member for Journal of Asian Natural Products Research, and Journal of Chinese Pharmaceutical Sciences. His group is actively involved in the isolation, structure determination, and synthetic optimization of natural products, and is currently focusing on natural products with significant bioactivities against infectious diseases, cancer, and neurodegenerative disorders. |
1. Introduction
The Trigonostemon genus belongs to the Euphorbiaceae family, and comprises about 50 species globally. The plants of this genus are trees or shrubs, and are mainly distributed in tropical and subtropical Asia.1 The plants of the Trigonostemon genus have long been used as folk medicine to treat asthma, diarrhea, and skin diseases in China and Thailand. These plants metabolize diverse compound classes including diterpenoids, triterpenoids, alkaloids, phenolics, and steroids. In particular, the structurally highly modified and biologically active daphnane diterpenoids and/or daphnane diterpenoid orthoesters (DDOs), and some alkaloids are the most important components, which have attracted great interest from the scientific communities in both natural products and organic synthesis fields. Since the first chemical investigation on the plant T. reidioides in 1990,2 over 10 plant species of the Trigonostemon genus have been extensively investigated chemically in the last two decades, which resulted in the isolation of about 200 structurally diversified compounds including terpenoids (triterpenoids, diterpenoids, and sesquiterpenoids), alkaloids, phenolics (flavonoids, lignans, coumarins, and phenyl propanoids), and steroids.3
Recently, a minireview was provided by Chen's group,3 which included a total of 66 compounds isolated from Trigonostemon plants before early 2010. From that time on, a rapid research progress has been witnessed, e.g. over 117 new compounds with diverse structures along with a large array of known analogues were isolated and characterized from eight species of Trigonostemon plants. Biological evaluation of these isolates revealed that some of the compounds, e.g. daphnane diterpenoids and alkaloids, show a wide spectrum of significant biological activities. A number of diterpenoids and alkaloids isolated from this plant genus with interesting frameworks and/or important bioactivities have also been successfully synthesized. The present report is aimed to provide a timely update and a comprehensive summary of the chemical and biological studies of this plant genus, and to serve as a pointer to their diverse applications. The main aspects covered in this review are the chemical components of Trigonostemon plants, the biosynthetic considerations of the major metabolites, their biological activities, and the synthetic work on some important diterpenoids and alkaloids isolated. For the compounds described in the previous review, this report only tabulated their chemical names, structures, occurrences, and distributions in the plants, as well as their bioactivities, but no detailed discussions are further made.
2. Chemical constituents
2.1. Diterpenoids
Diterpenoids are the major and most important ingredients of Trigonostemon plants, which included the compound types of daphnane, norditerpenoid dimer, phenanthrenone, abietane, 3,4-seco cleistanthane, and tigliane.
2.1.1. Daphnane diterpenoids.
A large number of daphnane diterpenoids were isolated from the genus Trigonostemon in the last two decades. This review included 90 daphnane diterpenoids (Table 1) obtained from the eight species of Trigonostemon plants, T. reidioides, T. thyrsoideum, T. chinensis, T. cherrieri, T. xyphophylloides, T. howii, T. lii, and T. heterophyllus. According to the modification and esterification patterns, they were categorized into five groups in this review, i.e. highly modified macrocyclic daphnane diterpenoid 9,12,14-orthoesters (Fig. 1), daphnane diterpenoid 9,12,14-orthoesters (Fig. 2), daphnane diterpenoid 9,13,14-orthoesters (Fig. 3), daphnane diterpenoid 12,13,14-orthoesters (Fig. 4), and simple daphnane (Fig. 5).
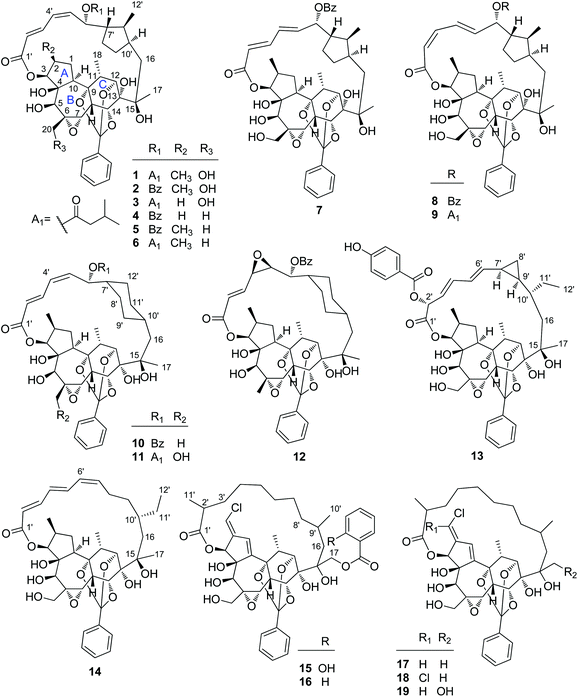 |
| Fig. 1 The structures of highly modified macrocyclic daphnane diterpenoid 9,12,14-orthoesters. | |
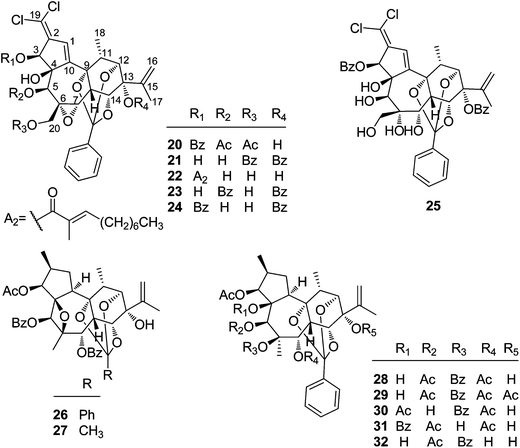 |
| Fig. 2 The structures of daphnane diterpenoid 9,12,14-orthoesters. | |
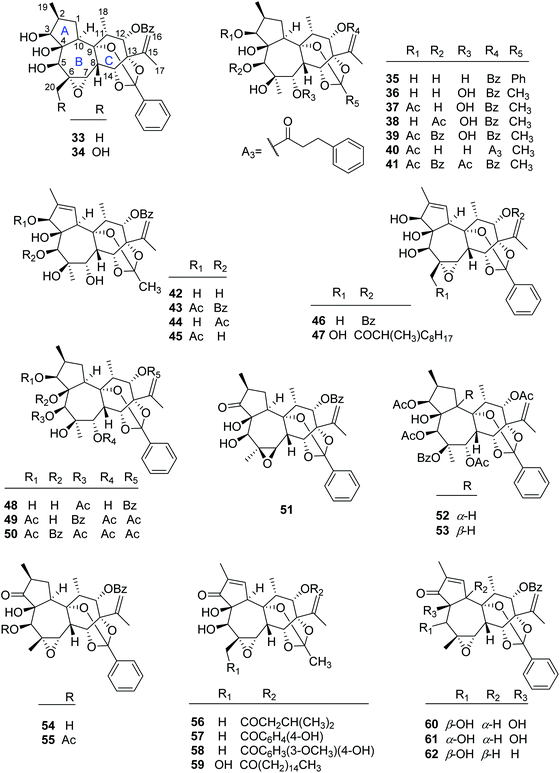 |
| Fig. 3 The structures of daphnane diterpenoid 9,13,14-orthoesters. | |
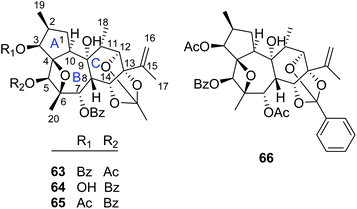 |
| Fig. 4 The structures of daphnane diterpenoid 12,13,14-orthoesters. | |
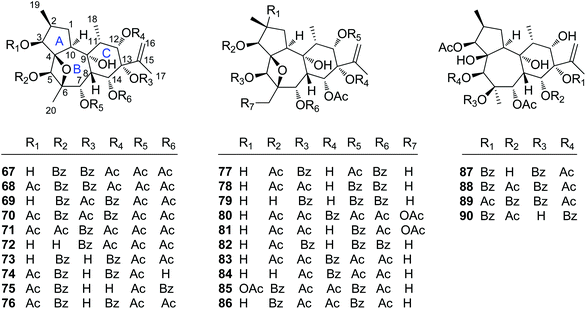 |
| Fig. 5 The structures of simple daphnane diterpenoids. | |
Table 1 Daphnane diterpenoids in the genus Trigonostemon
No. |
Compound |
Source |
Part |
Ref. |
|
Highly modified macrocyclic daphnane diterpenoid 9,12,14-orthoesters
|
1
|
Rediocide A |
T. reidioides
|
Stems, leaves and wood |
4
|
2
|
Rediocide C |
T. reidioides
|
Roots |
5
|
3
|
Rediocide E |
T. reidioides
|
Roots |
5
|
4
|
Rediocide F |
T. reidioides
|
Roots |
6
|
5
|
Trigothysoid O |
T. thyrsoideum
|
Twigs and leaves |
10
|
6
|
Trigothysoid P |
T. thyrsoideum
|
Twigs and leaves |
10
|
7
|
Trigonosin E |
T. thyrsoideum
|
Roots |
9
|
8
|
Trigonosin F |
T. thyrsoideum
|
Roots |
9
|
9
|
Trigothysoid N |
T. thyrsoideum
|
Twigs and leaves |
10
|
10
|
Rediocide G |
T. reidioides
|
Roots |
7
|
11
|
Rediocide B |
T. reidioides
|
Roots |
5
|
12
|
Rediocide D |
T. reidioides
|
Roots |
5
|
13
|
Trigochilide A |
T. chinensis
|
Leaves and twigs |
8
|
14
|
Trigochilide B |
T. chinensis
|
Leaves and twigs |
8
|
15
|
Trigocherriolide A |
T. cherrieri
|
Bark and wood |
11
|
16
|
Trigocherriolide C |
T. cherrieri
|
Bark and wood |
11
|
17
|
Trigocherriolide B |
T. cherrieri
|
Bark and wood |
11
|
18
|
Trigocherriolide D |
T. cherrieri
|
Bark and wood |
11
|
19
|
Trigocherriolide E |
T. cherrieri
|
Leaves |
12
|
|
|
Daphnane diterpenoid 9,12,14-orthoesters
|
20
|
Trigocherrin A |
T. cherrieri
|
Bark |
13
|
21
|
Trigocherrin B |
T. cherrieri
|
Bark and wood |
11
|
22
|
Trigocherrin C |
T. cherrieri
|
Bark and wood |
11
|
23
|
Trigocherrin D |
T. cherrieri
|
Bark and wood |
11
|
24
|
Trigocherrin F |
T. cherrieri
|
Bark and wood |
11
|
25
|
Trigocherrin E |
T. cherrieri
|
Bark and wood |
11
|
26
|
Trigochinin C |
T. chinensis
|
Twigs and leaves |
14
|
27
|
Trigothysoid K |
T. thyrsoideum
|
Twigs and leaves |
10
|
28
|
Trigoxyphin D |
T. xyphophylloides
|
Twigs |
15
|
29
|
Trigoxyphin E |
T. xyphophylloides
|
Twigs |
15
|
30
|
Trigohownin A |
T. howii
|
Twigs |
16
|
31
|
Trigohownin B |
T. howii
|
Twigs |
16
|
32
|
Trigohownin C |
T. howii
|
Twigs |
16
|
|
|
Daphnane diterpenoid 9,13,14-orthoesters
|
33
|
Trigonosin A |
T. thyrsoideum
|
Roots |
9
|
34
|
Trigonosin B |
T. thyrsoideum
|
Roots |
9
|
35
|
Trigonosin D |
T. thyrsoideum
|
Roots |
9
|
36
|
Trigothysoid D |
T. thyrsoideum
|
Twigs and leaves |
10
|
37
|
Trigothysoid E |
T. thyrsoideum
|
Twigs and leaves |
10
|
38
|
Trigothysoid F |
T. thyrsoideum
|
Twigs and leaves |
10
|
39
|
Trigothysoid G |
T. thyrsoideum
|
Twigs and leaves |
10
|
40
|
Trigoheterin D |
T. heterophyllus
|
Twigs |
18
|
41
|
Trigohownin E |
T. howii
|
Twigs |
16
|
42
|
Trigothysoid A |
T. thyrsoideum
|
Twigs and leaves |
10
|
43
|
Trigothysoid B |
T. thyrsoideum
|
Twigs and leaves |
10
|
44
|
Trigothysoid C |
T. thyrsoideum
|
Twigs and leaves |
10
|
45
|
Trigonothyrin G |
T. thyrsoideum
|
Stems |
17
|
46
|
Trigothysoid M |
T. thyrsoideum
|
Twigs and leaves |
10
|
47
|
Trigocherrierin A |
T. cherrieri
|
Leaves |
12
|
48
|
Trigonothyrin F |
T. thyrsoideum
|
Stems |
17
|
49
|
Trigoheterin C |
T. heterophyllus
|
Twigs |
18
|
50
|
Trigohownin D |
T. howii
|
Twigs |
16
|
51
|
Trigoheterin E |
T. heterophyllus
|
Twigs |
18
|
52
|
Trigoxyphin F |
T. xyphophylloides
|
Twigs |
15
|
53
|
Trigoxyphin I |
T. xyphophylloides
|
Twigs |
19
|
54
|
Trigoxyphin B |
T. xyphophylloides
|
Twigs |
15
|
55
|
Trigoxyphin C |
T. xyphophylloides
|
Twigs |
15
|
56
|
Trigochinin G |
T. chinensis
|
Twigs and leaves |
22
|
57
|
Trigochinin H |
T. chinensis
|
Twigs and leaves |
22
|
58
|
Trigochinin I |
T. chinensis
|
Twigs and leaves |
22
|
59
|
Trigoxyphin J |
T. xyphophylloides
|
Stems |
20
|
60
|
Trigoxyphin A |
T. xyphophylloides
|
Twigs |
15
|
61
|
Trigoxyphin K |
T. xyphophylloides
|
Stems |
20
|
62
|
Trigoxyphin H |
T. xyphophylloides
|
Twigs |
19
|
|
Daphnane diterpenoid 12,13,14-orthoesters
|
63
|
Trigonothyrin A |
T. thyrsoideum
|
Stems |
21
|
64
|
Trigonothyrin B |
T. thyrsoideum
|
Stems |
21
|
65
|
Trigonothyrin C |
T. thyrsoideum
|
Stems |
21
|
66
|
Trigothysoid L |
T. thyrsoideum
|
Twigs and leaves |
10
|
|
|
Simple daphnane diterpenoids
|
67
|
Trigochinin A |
T. chinensis
|
Twigs and leaves |
14
|
68
|
Trigochinin B |
T. chinensis
|
Twigs and leaves |
14
|
69
|
Trigochinin D |
T. chinensis
|
Twigs and leaves |
22
|
70
|
Trigochinin E |
T. chinensis
|
Twigs and leaves |
22
|
71
|
Trigochinin F |
T. chinensis
|
Twigs and leaves |
22
|
72
|
Trigonosin E |
T. thyrsoideum
|
Roots |
9
|
73
|
Trigolin A |
T. lii
|
Stems |
23
|
74
|
Trigolin B |
T. lii
|
Stems |
23
|
75
|
Trigolin C |
T. lii
|
Stems |
23
|
76
|
Trigolin D |
T. lii
|
Stems |
23
|
77
|
Trigolin E |
T. lii
|
Stems |
23
|
78
|
Trigolin F |
T. lii
|
Stems |
23
|
79
|
Trigolin G |
T. lii
|
Stems |
23
|
80
|
Trigothysoid H |
T. thyrsoideum
|
Twigs and leaves |
10
|
81
|
Trigothysoid I |
T. thyrsoideum
|
Twigs and leaves |
10
|
82
|
Trigothysoid J |
T. thyrsoideum
|
Twigs and leaves |
10
|
83
|
Trigonothyrin D |
T. thyrsoideum
|
Stems |
17
|
84
|
Trigonothyrin E |
T. thyrsoideum
|
Stems |
17
|
85
|
Trigoheterin A |
T. heterophyllus
|
Twigs |
18
|
86
|
Trigoheterin B |
T. heterophyllus
|
Twigs |
18
|
87
|
Trigohownin F |
T. howii
|
Twigs |
16
|
88
|
Trigohownin G |
T. howii
|
Twigs |
16
|
89
|
Trigohownin H |
T. howii
|
Twigs |
16
|
90
|
Trigohownin I |
T. howii
|
Twigs |
16
|
2.1.1.1. Highly modified macrocyclic daphnane diterpenoid 9,12,14-orthoesters.
Rediocide A (1), the first highly modified daphnane diterpenoid, was obtained from the leaves and stems of T. reidioides collected from Thailand by Singh's group in 2000 via a bioassay guided identification that was designed to kill mosquito larvae (Aedes aegypti). Rediocide A possessed a 9,12,14-orthoester group and an unusual 12-carbon polyketide appendage at C-16 furnishing a macro-lactone ring with C-3 (Fig. 1).4 Four additional minor analogues, rediocides B–E (11, 2, 12, and 3, resp.), were reported by the same research group from its roots four years later.5 Soon afterwards, Wongsinkongman's and Ruchirawat's groups identified two more congeners, rediocides F (4)6 and G (10),7 from the roots of the same Thai plant, respectively. In 2009, from the leaves of a Chinese species T. chinensis native to Yunnan Province of China, our research group reported the isolation of two unique macrocyclic daphnane diterpenoid 9,12,14-orthoesters, trigochilides A (13) and B (14), for the first time.8 Trigochilide A featured a unique 12-carbon polyketide appendage with a cyclopropane ring. Several years later, Hao's group reported five analogues, trigonosins E (7) and F (8) in 2011,9 and trigothysoids N–P (9, 5, and 6, resp.) in 2013
10 from a Yunnan species T. thyrsoideum. Litaudon's group also identified five additional analogues trigocherriolides A–E (15–19) from T. cherrieri in 2012 and 2014, sequentially.11,12 The diagnostic features of this class of compounds are daphnane diterpenoids with diverse 12-carbon-containing polyketide appendages and a common 9,12,14-orthoester motif.
2.1.1.2. Daphnane diterpenoid 9,12,14-orthoesters.
Trigocherrin A (20) (Fig. 2) was the first example of natural chlorinated daphnane diterpenoid 9,12,14-orthoester isolated by Litaudon et al. from the bark of T. cherrieri collected from the Poya region in the west of New Caledonia.13 The absolute configuration of 20 was determined by a combined strategy of comparing the experimental electronic circular dichroism (ECD) data with that of calculated ECD. Four more chlorinated congeners, trigocherrins B–F (21–25), were also reported from the bark and wood of T. cherrieri in the same period by the same research group.11 Two novel daphnane diterpenoid 9,12,14-orthoesters trigochinin C (26)14 and trigothysoid K (27)10 with a unique 4β,6β-oxetanyl group were isolated from twigs and leaves of T. chinensis and T. thyrsoideum, respectively. The absolute structure of trigochinin C was confirmed by X-ray crystallography analysis. Five highly oxygenated daphnane diterpenoid 9,12,14-orthoesters, trigoxyphins D (28) and E (29)15 from the twigs of T. xyphophylloides, and trigohownins A–C (30–32)16 from the twigs of T. howii, were further reported by Yue's research group, and both of the plant samples were collected from the Sanya area in Hainan Island of China.
2.1.1.3. Daphnane diterpenoid 9,13,14-orthoesters.
To date, 30 daphnane diterpenoid 9,13,14-orthoesters (Fig. 3) have been isolated from the plants of T. thyrsoideum, T. heterophyllus, T. howii, T. chinensis, T. cherrieri, and T. xyphophylloides. In this class, 16 compounds including trigonosins A (33), B (34) and D (35),9 trigothysoid M (46),10 trigocherrierin A (47),12 trigonothyrin F (48),17 trigoheterins C (49) and E (51),18 trigohownin D (50),16 trigoxyphins A–C (60, 54, and 55, resp.),15 F (52),15 H (62),19 I (53),19 and K (61)20 possessed the same 9,13,14-orthobenzoate moiety at the six-membered C-ring, while the rest of the 14 analogues, namely trigothysoids A–E (42–44, 36–39, resp.),10 trigoheterin D (40),18 trigohownin E (41),16 trigonothyrin G (45),17 trigochinins G–I (56–58),22 and trigoxyphin J (59),20 furnished a common 9,13,14-orthoacetate at the C-ring. For the most of these daphnane diterpenoids, the H-10 was α-configured, but trigoxyphins H (62) and I (53) were the exceptions, where the H-10 was β-directed.
2.1.1.4. Daphnane diterpenoid 12,13,14-orthoesters.
Trigonothyrins A–C (63–65) (Fig. 4) were isolated from stems of T. thyrsoideum collected from the Yunnan Province of China by Liu's group.21 Three compounds shared the common characteristics of a 12,13,14-orthoacetate and a unique 4β,6β-oxetanyl moiety. The relative configurations of the 4,6-oxetanyl moiety in the B-ring of 63–65 were first assigned as 4β,6α-oriented, which was indicated to be a wrong assignment shortly afterwards by Yue's group in a related paper.14 Three structures were then revised accordingly by Liu's group.17 Trigothysoid L (66) was isolated from twigs and leaves of T. thyrsoideum collected from the same area by Hao's group quite recently.10
2.1.1.5. Simple daphnane diterpenoids.
The rest 24 compounds are the simple daphnane diterpenoids (67–90) without the macrocyclic polyketide appendages and/or orthoester groups (Fig. 5). In this compound category, trigochinins A (67), B (68),14 D–F (69–71)22 were isolated from the twigs and leaves of T. chinensis, a plant growing in the Yunnan Province of China; trigoheterins A (85) and B (86)18 were obtained from the twigs of T. heterophyllus collected from Hainan Province of China, and trigohownins F–I (87–90)16 were from the twigs of a Hainan plant T. howii. Trigolins A–G (73–79)23 were obtained from the stems of T. lii native to Yunnan. Trigonosin E (72)9 and trigothysoids H–J (80–82)10 were isolated from twigs and leaves of T. thyrsoideum, a plant native to Yunnan Province of China, and trigonothyrins D (83) and E (84)17 were isolated from stems of the same plant. From the structural view, compounds 67–86 featured a unique 4β,6β-oxetanyl group at the B-ring.
2.1.2. Norditerpenoid dimers.
There was no report on the dimeric diterpenoids from the Trigonostemon genus before the last minireview.3 Recently, 15 norditerpenoid dimers have been isolated from three Trigonostemon plants, T. heterophyllus, T. howii, and T. xyphophylloides (Table 2).
Table 2 Norditerpenoid dimers, phenanthrenones, abietanes, 3,4-seco-cleistanthanes, and tigliane diterpenoids
No. |
Compound |
Source |
Part |
Ref. |
|
Norditerpenoid dimers
|
91
|
Trigoflavidol A |
T. flavidus
|
Stems |
24
|
92
|
Trigoflavidol B |
T. flavidus
|
Stems |
24
|
93
|
Trigohowilol C |
T. howii
|
Stems |
25
|
94
|
Trigohowilol D |
T. howii
|
Stems |
25
|
95
|
Trigohowilol E |
T. howii
|
Stems |
25
|
96
|
Trigohowilol F |
T. howii
|
Stems |
25
|
97
|
Neoboutomannin |
T. flavidus
|
Stems |
24
|
98
|
Trigohowilol G |
T. howii
|
Stems |
25
|
99
|
Trigoxyphin S |
T. xyphophylloides
|
Twigs |
26
|
100
|
Trigoxyphin T |
T. xyphophylloides
|
Twigs |
26
|
101
|
Trigoxyphin O |
T. xyphophylloides
|
Twigs |
26
|
102
|
Trigoxyphin R |
T. xyphophylloides
|
Twigs |
26
|
103
|
Trigoxyphin P |
T. xyphophylloides
|
Twigs |
26
|
104
|
Trigoxyphin Q |
T. xyphophylloides
|
Twigs |
26
|
105
|
Trigoxyphin J |
T. xyphophylloides
|
Twigs |
27
|
|
|
Phenanthrenone diterpenoids
|
106
|
Trigoflavidol C |
T. flavidus
|
Stems |
24
|
107
|
Trigonochinene E |
T. flavidus
|
Stems |
24
|
108
|
Thrigonosomone A |
T. lii
|
Roots and stems |
28
|
109
|
Thrigonosomone B |
T. lii
|
Roots and stems |
28
|
110
|
Trigonostemone |
T. reidioides
|
Roots |
2
|
111
|
6,9-O-Dedimethyl-thrigonosomone A |
T. lii
|
Roots and stems |
28
|
112
|
Trigonostemon E |
T. chinensis
|
Stem bark and wood |
29
|
113
|
Trigohowilol A |
T. howii
|
Stems |
25
|
114
|
Trigohowilol B |
T. howii
|
Stems |
25
|
115
|
Trigoxyphin G |
T. xyphophylloides
|
Twigs |
15
|
116
|
Trigoxyphin K |
T. xyphophylloides
|
Twigs |
27
|
117
|
Trigoxyphin L |
T. xyphophylloides
|
Twigs |
27
|
118
|
Trigoxyphin M |
T. xyphophylloides
|
Twigs |
27
|
119
|
Trigoxyphin N |
T. xyphophylloides
|
Twigs |
27
|
|
|
Abietane dinorditerpenoids
|
120
|
12-Hydroxy-13-methylpodocarpa-9,11,13-trien-3-one |
T. howii
|
Stems |
25
|
121
|
Trigonostemon A |
T. chinensis
|
Stem bark and wood |
29
|
122
|
Dihydroheudelotinol |
T. chinensis
|
Stem bark and wood |
29
|
123
|
Trigonostemon B |
T. chinensis
|
Stem bark and wood |
29
|
124
|
5S-Heudelotinone |
T. chinensis
|
Stem bark and wood |
29
|
125
|
Trigonostemon C |
T. chinensis
|
Stem bark and wood |
29
|
126
|
Trigonostemon D |
T. chinensis
|
Stem bark and wood |
29
|
|
|
3,4-Seco cleistanthane diterpenoids
|
127
|
Trigonochinene A |
T. chinensis
|
Stems and leaves |
32
|
128
|
Trigonochinene B |
T. chinensis
|
Stems and leaves |
32
|
129
|
Trigonochinene C |
T. chinensis
|
Stems and leaves |
32
|
130
|
Trigonochinene D |
T. chinensis
|
Stems and leaves |
32
|
131
|
3,4-Seco-sonderianol |
T. chinensis
|
Stems and leaves |
32
|
132
|
3,4-Seco-sonderianic |
T. chinensis
|
Stems and leaves |
32
|
133
|
Trigonoheterene |
T. heterophyllus
|
Stems |
33
|
134
|
Trigonoheterene B |
T. heterophyllus
|
Stems |
34
|
135
|
Trigoflavidone A |
T. flavidus
|
Stems |
35
|
136
|
Trigoflavidone B |
T. flavidus
|
Stems |
35
|
137
|
Trigoflavidone C |
T. flavidus
|
Stems |
35
|
138
|
Trigoflavidone D |
T. flavidus
|
Stems |
35
|
139
|
Trigoflavidone E |
T. flavidus
|
Stems |
35
|
140
|
Trigoheteric acid methyl ester |
T. heterophyllus
|
Twigs |
18
|
|
|
Tigliane diterpenoid
|
141
|
Trigowiin A |
T. howii
|
Bark |
36
|
A chemical study on the stems of Hainan species T. flavidus led to the isolation of two tetranorditerpenoid dimers, trigoflavidols A (91) and B (92), and a homodimeric diterpenoid, neoboutomannin (97) (Fig. 6).24 Four more heterodimers, trigohowilols C–F (93–96), and one diterpenoid homodimer, trigohowilol G (98), were obtained from the stems of T. howii collected in Sanya, Hainan province of China.25 Additionally, seven more degraded diterpenoid dimers, trigoxyphins O–T and J (99–105), were identified from the twigs of a Hainan species of T. xyphophylloides, in which trigoxyphins S (99) and T (100) are homodimers, trigoxyphins O (101) and R (102) belong to heterodimers, and trigoxyphins P, Q, and J (103–105) are the conjugates of a degraded diterpenoid and a phenylpropanoid.26,27
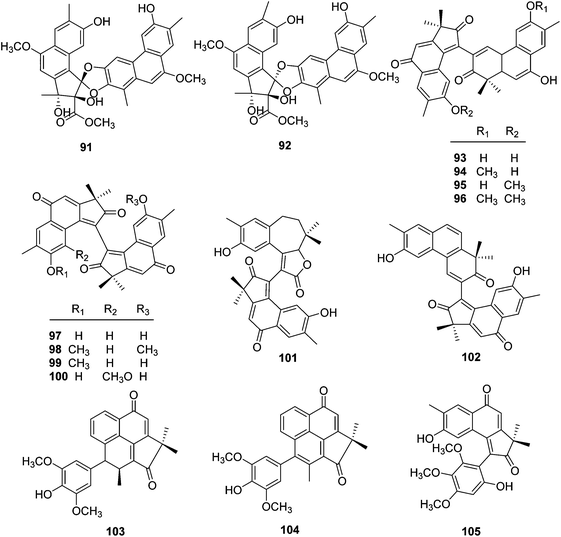 |
| Fig. 6 The structures of norditerpenoid dimers. | |
2.1.3. Phenanthrenone diterpenoids.
In the previous minireview, six phenanthrenone diterpenoids, i.e. thrigonosomones A (108) and B (109),28 trigonostemone (110),2 6,9-O-dedimethyl-thrigonosomone A (111),28 trigonostemon E (112),29 and trigoxyphin G (115),15 were included (Fig. 7). Trigonostemone (110), also named 1,1,7-trimethyl-3,6,9-trimethoxy-2-phenanthrenone, was first isolated from roots of the Thai plant T. reidioides, whose absolute structure was determined by X-ray crystallography.2 Recently, a series of phenanthrenone diterpenoids, e.g. trigoflavidol C (106)24 and trigonochinene E (107)24 from the stems of T. flavidus, and trigohowilols A (113) and B (114)25 from the stems of T. howii were isolated, and both plants were collected from Hainan Island. In addition, four degraded diterpenoids, namely trigoxyphins K–N (116–119), were obtained from the twigs of a Hainan species T. xyphophylloides,27 and they were assigned as neoboutomannin derivatives30 and are structurally related to 1,7-dimethyl-9-methoxyphenanthrene.31
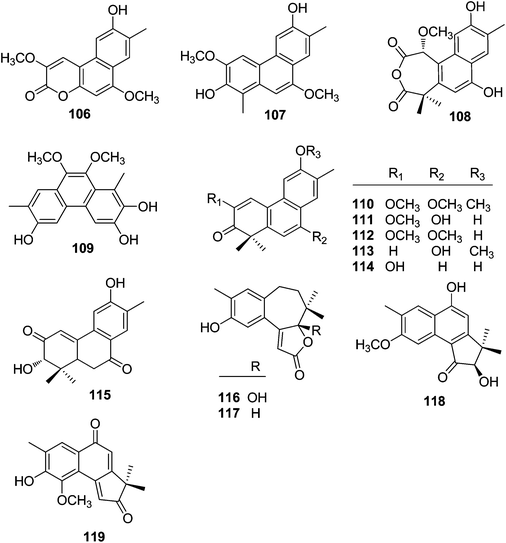 |
| Fig. 7 The structures of phenanthrenone diterpenoids in the genus Trigonostemon. | |
2.1.4. Abietane dinorditerpenoids.
Seven abietane type dinorditerpenoids were obtained from the plants of T. howii and T. chinensis (Table 2, Fig. 8). An abietane dinorditerpenoid, namely 12-hydroxy-13-methylpodocarpa-9,11,13-trien-3-one (120), was isolated from T. howii, a plant that is native to Hainan Province of China.25 Six compounds, trigonostemons A–D (121, 123, 125, and 126, resp.), dihydroheudelotinol (122), and heudelotinone (124) were identified from the stem bark and wood of T. chinensis, which was collected from Guangxi Province of China.29 The absolute configuration of trigonostemon A (121) was determined by X-ray crystallography. Compounds (121–126) possessed a rearranged skeleton of dinorditerpenoid that was suggested to be biosynthetically originated from 12-hydroxy-13-methylpodocarpa-9,11,13-trien-3-one (120).25
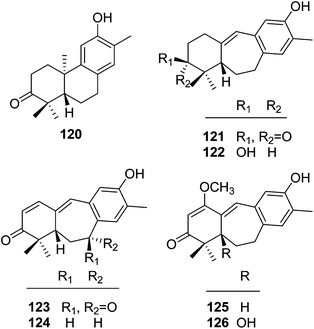 |
| Fig. 8 The structures of abietane dinorditerpenoids. | |
2.1.5. 3,4-Seco cleistanthane diterpenoids.
Except for the isolation of six 3,4-seco cleistanthane diterpenoids (Table 2), namely trigonochinenes A–D (127–130), 3,4-seco-sonderianol (131), and 3,4-seco-sonderianic acid (132), from a Hainan species T. chinensis32 as summarized in the previous minireview, eight more 3,4-seco cleistanthane diterpenoids (Fig. 9), i.e. trigonoheterene (133),33 trigonoheterene B (134)34 and trigoheteric acid methyl ester (140)18 from the stems and twigs of T. heterophyllus, and trigoflavidones A–E (135–139)35 from the stems of T. flavidus were isolated, and both plant samples were collected from Hainan Island of China.
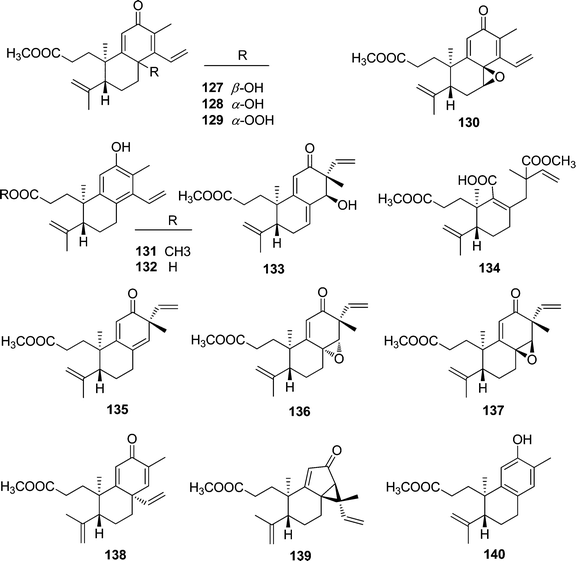 |
| Fig. 9 The structures of 3,4-seco cleistanthane diterpenoids. | |
2.1.6. Tigliane diterpenoid.
Up to now, only one tigliane diterpenoid, trigowiin A (141, Fig. 10), has been isolated from the bark of T. howii,36 which was collected from Vietnam.
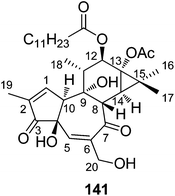 |
| Fig. 10 The structure of tigliane diterpenoid. | |
2.2. Alkaloids
It is particularly interesting that 36 diverse alkaloids of two major classes, β-carboline and indole (simple indole, bisindole, and flavonoidal indole types), were also isolated from Trigonostemon plants. Among them, 11 alkaloids including four types of carboline, simple indole, bisindole and flavonoidal indole were covered in the previous minireview.3 Thereafter, 25 additional alkaloids with diverse carbon skeletons were further identified from the aforementioned Trigonostemon species. In the present review, all the alkaloids isolated from Trigonostemon species were classified into two major classes, namely β-carboline and indole, and are listed in Table 3.
Table 3 Alkaloids in the genus Trigonostemon
No. |
Compound |
Source |
Part |
Ref. |
|
β-Carboline alkaloids
|
142
|
Trigonostemonine A |
T. lii
|
Roots and stems |
38
|
143
|
Trigonostemonine B |
T. lii
|
Roots and stems |
38
|
144
|
Trigonostemonine C |
T. lii
|
Roots and stems |
38
|
145
|
Trigonostemonine D |
T. lii
|
Roots and stems |
38
|
146
|
Trigonostemine E |
T. lii
|
Leaves |
39
|
147
|
Trigonostemine F |
T. lii
|
Leaves |
39
|
148
|
Trigonostemonine E |
T. lii
|
Roots and stems |
38
|
149
|
11-Methoxyrutecarprine |
T. lii
|
Leaves |
39
|
150
|
2-Methoxyrutecarprine |
T. lii
|
Leaves |
39
|
151
|
Trifiline C |
T. filipes
|
Plant |
40
|
152
|
Trigonostemonine F |
T. lii
|
Roots and stems |
38
|
153
|
Trifiline A |
T. filipes
|
Plant |
40
|
154
|
Trifiline B |
T. filipes
|
Plant |
40
|
155
|
Trigonostemine A |
T. lii
|
Leaves |
39
|
156
|
Trigonostemine B |
T. lii
|
Leaves |
39
|
157
|
Trigonostemine C |
T. lii
|
Leaves |
39
|
158
|
Trigonostemine D |
T. lii
|
Leaves |
39
|
159
|
Arenarine C |
T. lii
|
Leaves |
39
|
160
|
Harmicacid methyl ester |
T. lii
|
Leaves |
39
|
161
|
1,2,3,4-Tetrahydro-oxo-β-carboline |
T. lii
|
Leaves |
39
|
162
|
Norharmine |
T. lii
|
Leaves |
39
|
163
|
Harmine |
T. lii
|
Leaves |
39
|
164
|
Trigonoine C |
T. lii
|
Leaves |
40
|
165
|
Trigonoine A |
T. lii
|
Leaves |
41
|
166
|
Trigonoine B |
T. lii
|
Leaves |
41
|
|
|
Indole alkaloids
|
167
|
Trigonoliimine A |
T. lii
|
Leaves |
42
|
168
|
Trigonoliimine B |
T. lii
|
Leaves |
42
|
169
|
Trigonoliimine C |
T. lii
|
Leaves |
42
|
170
|
Trigonostemon F |
T. chinensis
|
Stem bark and wood |
29
|
171
|
Trigolutesin A |
T. lutescens
|
Twigs |
43
|
172
|
Trigolutesin B |
T. lutescens
|
Twigs |
43
|
173
|
Trigolute A |
T. lutescens
|
Twigs |
43
|
174
|
Trigolute B |
T. lutescens
|
Twigs |
43
|
175
|
Trigolute C |
T. lutescens
|
Twigs |
43
|
176
|
Trigolute D |
T. lutescens
|
Twigs |
43
|
177
|
Lotthanongine |
T. reidioides
|
Roots |
44
|
2.2.1. β-Carboline alkaloids.
β-Carbolines are a class of structurally interesting and biologically important alkaloids possessing a common tricyclic pyrido[3,4-b]indole ring system.37 In addition to six β-carboline alkaloids, trigonostemonines A–D (142–145), E (148), and F (152) presented in the former review,3,38 16 additional β-carboline alkaloids (Fig. 11) were further obtained from the leaves of T. lii, which included 11-methoxyrutecarprine (149),39 2-methoxyrutecarprine (150),39 trigonostemines A–F (155–158, 146, and 147, resp.),39 arenarine C (159),39 harmicacid methyl ester (160),39 1,2,3,4-tetrahydro-oxo-β-carboline (161),39 norharmine (162),39 harmine (163),39 trigonoine C (164),40 trigonoines A (165) and B (166).41 Among them, trigonoine A (165) possessed a 2,8-diazabicyclo[3.3.1]nonane ring system, and trigonoine B (166) formed by a combination of 2,3-dihydroquinolin-4-one and 3H-pyrrolo[2,3-c]quinoline that rearranged from the β-carboline skeleton. In addition, three β-carboline alkaloids, namely trifilines A–C (153, 154, and 151, resp.), were also isolated from the plant T. filipes collected from Guangxi Province of China.40
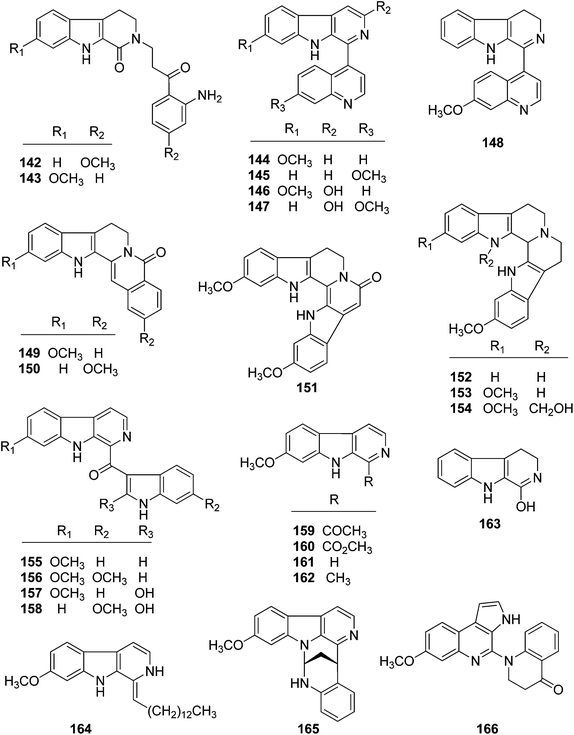 |
| Fig. 11 The structures of β-carboline alkaloids. | |
2.2.2. Indole alkaloids.
11 indole alkaloids (Fig. 12) including trigonoliimines A–C (167–169),42 trigonostemon F (170),29 trigolutesins A (171) and B (172),43 trigolutes A–D (173–176),43 and lotthanongine (177)44 were identified from Trigonostemon plants. Among them, trigolutesins A (171) and B (172), and trifilines A–D (173–176) were the first examples of bisindole alkaloids with a unique spiro ring system isolated from the twigs of T. lutescens reported by Dai's group.43
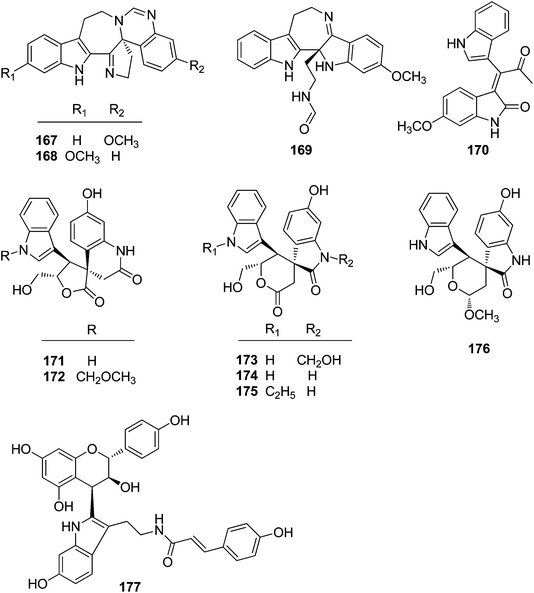 |
| Fig. 12 The structures of indole alkaloids. | |
2.3. Miscellaneous
Except for the two major and most important ingredients of diterpenoids and alkaloids in the Trigonostemon plants, a number of diverse compound classes, including steroids, triterpenoids, sesquiterpenoids, lignans, flavonoids and coumarins, were also isolated. All these compounds are summarized in Table 4.
Table 4 Miscellaneous compound classes in the genus Trigonostemon
No. |
Compound |
Source |
Part |
Ref. |
|
Steroids
|
178
|
Stigmasterol |
T. reidioides
|
Roots |
7
|
179
|
5α-Stigmastane-3,6-dione |
T. reidioides
|
Roots |
45
|
180
|
Δ8,22-Ergostadien-3β-ol-11-one |
T. xyphophylloides
|
Twigs |
46
|
181
|
Mangdesisterol |
T. xyphophylloides
|
Twigs |
47
|
182
|
Ergosterol peroxide |
T. xyphophylloides
|
Twigs |
46,47
|
|
|
Triterpenoids
|
183
|
3-O-Benzoylpluricostatic acid |
T. xyphophylloides
|
Stems |
20
|
184
|
Pluricostatic acid |
T. xyphophylloides
|
Stems |
20
|
185
|
Ursolic acid |
T. xyphophylloides
|
Stems |
20
|
186
|
24(R)-25-Dihydroxy-5α-dammar-20-en-3-one |
T. xyphophylloides
|
Stems |
20
|
|
|
Sesquiterpenoids
|
187
|
Trigohetone |
T. heterophyllus
|
Twigs |
18
|
188
|
Trigonoheterone |
T. heterophyllus
|
Stems |
33
|
|
|
Lignans
|
189
|
Syringaresinol |
T. xyphophylloides
|
Twigs |
46
|
190
|
Pinoresinol |
T. lutescens
|
Twigs |
47
|
191
|
Episyringaresinol |
T. xyphophylloides
|
Twigs |
46
|
192
|
Aviculin |
T. heterophyllus
|
Stems |
48,49
|
193
|
Trigonoheteran |
T. heterophyllus
|
Stems |
48
|
|
|
Flavaloid
|
194
|
Afzelechin-(4α→8)-afzelechin |
T. reidioides
|
Roots |
44
|
|
|
Coumarins
|
195
|
Scopoletin |
T. reidioides
|
Roots |
7
|
196
|
Tomenti |
T. reidioides
|
Roots |
7
|
197
|
5,7-Dihydroxy-6-methoxycoumarin |
T. reidioides
|
Roots |
45
|
198
|
1-(2-Hydroxy-4,5-dimethoxyphenyl)-propan-1-one |
T. xyphophylloides
|
Stems |
50
|
199
|
4-Hydroxy-3,5-dimethoxybenzaldehyde |
T. lutescens
|
Twigs |
47
|
200
|
6-Deoxyjacareubin |
T. xyphophylloides
|
Twigs |
46
|
2.3.1. Steroids.
Five steroids (Table 4, Fig. 13) were obtained in the previous research on Trigonostemon plants. Stigmasterol (178)7 and 5α-stigmastane-3,6-dione (179)45 were discovered from roots of T. reidioides, and Δ8,22-ergostadien-3β-ol-11-one (180),46 mangdesisterol (181),46 and ergosterol peroxide (182)46,47 were obtained from twigs of a Hainan plant T. xyphophylloides.
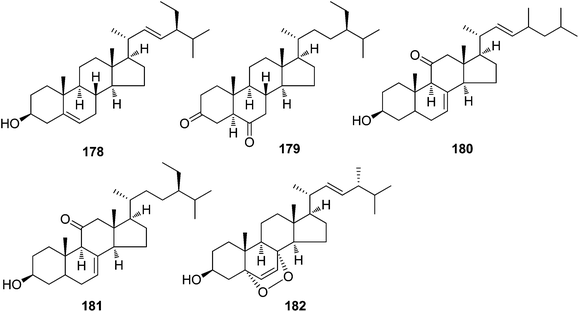 |
| Fig. 13 The structures of steroids. | |
2.3.2. Triterpenoids.
In 2013, four triterpenoids (Table 4, Fig. 14), namely 3-O-benzoylpluricostatic (183), pluricostatic acid (184), ursolic acid (185), and 24(R)-25-dihydroxy-5α-dammar-20-en-3-one (186), were isolated from the stems of T. xyphophylloides, a plant native to Hainan Island of China.20
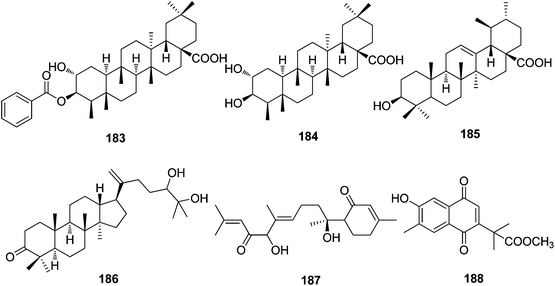 |
| Fig. 14 The structures of triterpenoids and sesquiterpenoids. | |
2.3.3. Sesquiterpenoids.
Trigohetone (187) was the first prenylated bisabolane sesquiterpenoid isolated from the air-dried twigs of a Hainan species T. heterophyllus.18 A sesquiterpenoid, trigonoheterone (188), with a naphthoquinone skeleton (Table 4, Fig. 14) was then isolated from the stems of T. heterophyllus, which was also collected from Hainan Island of China.33
2.3.4. Lignans.
Three lignans (Fig. 15), syringaresinol (189) and episyringaresinol (191) from the twigs of a Hainan plant T. xyphophylloides,46 and pinoresinol (190) from the twigs of a Guangxi plant T. lutescens,47 were reported. Recently, two lignan glycosides (Fig. 15), namely aviculin (192) and trigonoheteran (193), were further isolated from the stems of T. heterophyllus, a plant native to Hainan Island of China.48,49
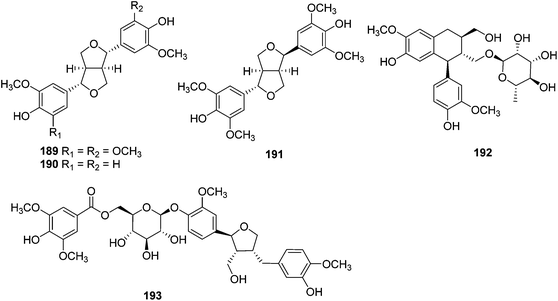 |
| Fig. 15 The structures of lignans. | |
2.3.5. Flavaloid.
One flavaloid dimer, afzelechin-(4α→8)-afzelechin (194), was obtained from the roots of a Thai medicinal plant T. reidioides (Fig. 16).44
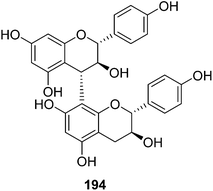 |
| Fig. 16 The structure of flavaloid. | |
2.3.6. Coumarins and other phenolics.
Scopoletin (195),7 tomenti (196),7 and 5,7-dihydroxy-6-methoxycoumarin (197)45 are coumarins isolated from the roots of a Thai plant T. reidioides (Fig. 17). Besides, three other phenolics, 1-(2-hydroxy-4,5-dimethoxyphenyl)-propan-1-one (198) from the stems of T. xyphophylloides,50 4-hydroxy-3,5-dimethoxybenzaldehyde (199) from the twigs of a Guangxi species of T. lutescens,47 and 6-deoxyjacareubin (200) from the twigs of a Hainan species of T. xyphophylloides,46 were isolated recently.
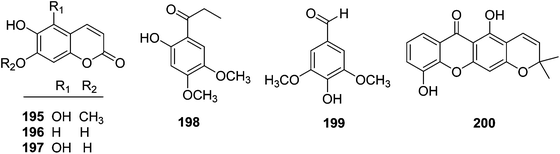 |
| Fig. 17 The structures of coumarins and other phenolics. | |
3. Biosynthetic origin proposed for some isolated compounds
3.1. Phenanthrenone diterpenoids
A possible biosynthetic origin for thrigonosomone A (108) and 6,9-O-demethylthrigonomone A (111) is proposed (Scheme 1). A biogenetic precursor of phenanthrenone diterpenoid 111i subjected to a cascade of methylation, isomerization, and oxidation would produce a ring-expended heptacyclic anhydride, thrigonosomone A (108). A simple methylation of 111i would give 6,9-O-demethylthrigonomone A (111).28
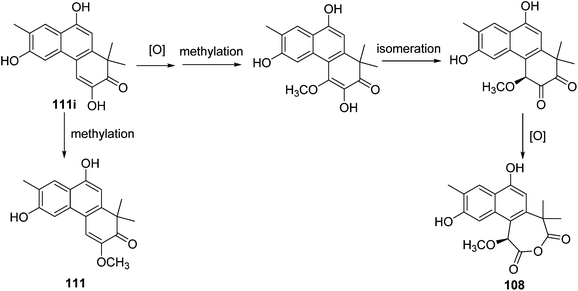 |
| Scheme 1 Plausible biogenetic pathways for thrigonosomone A (108) and 6,9-O-demethylthrigonosomone A (111). | |
3.2. 3,4-Seco cleistanthane diterpenoids
Trigochinenes A–D (127–130), 3,4-seco-sonderianol (131), 3,4-seco-sonderianic (132), trigonoheterene (133), trigonoheterene B (134), trigoflavidones A–E (135–139), and trigoheteric acid methyl ester (140) belonged to the compound class of 3,4-seco cleistanthane diterpenoids, which were likely originated from the cleavage of the C-3–C-4 bond of the 3-keto precursors.35 In Scheme 2, trigoflavidone A (135) would be readily modified by involving a [1,3]-sigmatropic shift of the vinyl group and ring contraction rearrangement to form the trigoflavidones D (138) and E (139). Epoxidation of the 8,14 double bond of 135 would produce trigoflavidones B (136) and C (137).
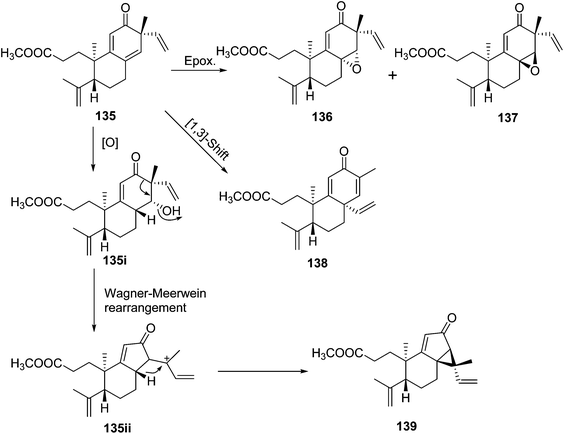 |
| Scheme 2 Plausible biogenetic pathway of trigoflavidones A–E (135–139). | |
3.3. β-Carboline alkaloids
The biosynthetic origin of trigonoine A (165), a carboline alkaloid featuring a 2,8-diazabicyclo[3.3.1]nonane ring system, is proposed as shown in Scheme 3. The key intermediate 165iii would be formed via the Mannich reaction of tryptamine (165i) and quinoline-4-carbaldehyde (165ii). Followed by dehydrogenation and intramolecular nucleophilic reaction, alkaloid 165 would be yielded. Trigonoine B (166) is an adduct of 2,3-dihydroquinolin-4-one (166i) and 3H-pyrrolo[2,3-c]quinolone rearranged from the precursor β-carboline alkaloid (166ii), which was first oxygenated to 166iii, and was then converted to 166iv by an intramolecular condensation. The condensation of 166iv and 166i would yield intermediate 166v, which was finally converted to 166 by dehydrogenation (Scheme 4).41
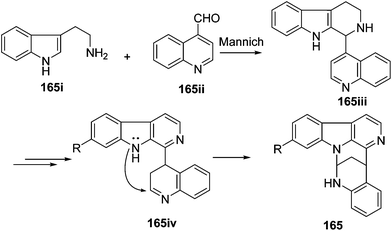 |
| Scheme 3 Plausible biogenetic pathway of trigonoine A (165). | |
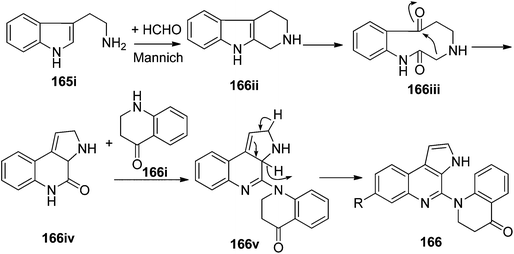 |
| Scheme 4 Plausible biogenetic pathway of trigonoine B (166). | |
3.4. Indole alkaloids
Three indole alkaloids, trigonoliimines A–C (167–169), were proposed to be originated from 201 (Scheme 5), which would yield 202 by oxidation. The key intermediate 202 underwent two alternative acidic catalysis pathways and modifications would give alkaloids trigonoliimine A (167) or B (168), and trigonoliimine C (169), respectively.51
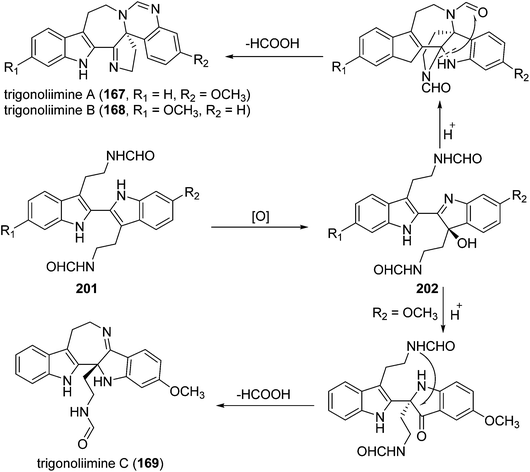 |
| Scheme 5 Plausible biogenetic pathway of trigonoliimines A–C (167–169). | |
The plausible biogenetic pathways for alkaloids 171 and 174 are proposed as shown in Scheme 6,43 where trigonostemon F (170) was supposed to be the biogenetic precursor of two bisindole alkaloids trigolutesin A (171) and trigolute B (174).
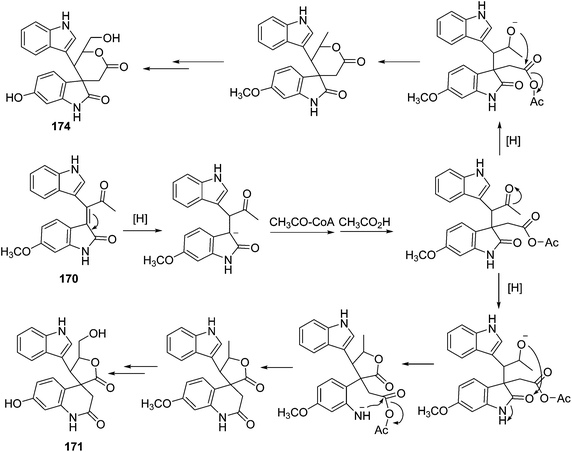 |
| Scheme 6 Plausible biogenetic pathway of trigolutesins A (171) and B (174). | |
4. Biological activities
The former review summarized the biological activities of the compounds isolated from Trigonostemon plants previously, which included insecticidal, insect antifeedant, antimicrobial, cytotoxic, antitumor, anti-HIV-1, and antivenom activities and inhibition of MET tyrosine kinase.3 In addition, it was found that the methanolic extract of the roots of T. reidioides shows anticandidal activity against Candida krusei.52 We described herein only the biological activities of the newly obtained compounds from Trigonostemon species, which were not included in the last review.
4.1. Cytotoxic activity
The cytotoxic activities of the daphnane diterpenoids from Trigonostemon plants against different human tumor cell lines were evaluated. Nine daphnane diterpenoids from twigs of T. howii were evaluated for cytotoxic activity against tumor cell lines HL-60 (human premyelocytic leukemia) and A-549 (human lung adenocarcinoma) using the MTT method and the SRB method, respectively, and the results showed that trigohownins A (30) and D (50) exhibit moderate activities against the HL-60 cell line with the IC50 values of 17.0 and 9.3 μM, respectively.16 Trigoheterin E (51) exhibited moderate cytotoxic activity against both HL-60 and A-549 cell lines with IC50 values of 1.8 and 10.0 μM, respectively.18 Trigoxyphin I (53) showed modest cytotoxicity against SPCA-1 (human lung cancer) and BEL-7402 (human hepatocellular carcinoma) cancer cell lines with IC50 values of 11.07 and 8.73 μM, respectively, and trigoxyphin H (62) exhibited weak activities with IC50 values of 37.30 and 16.13 μM, respectively.19
Two tetranorditerpenoid dimers trigoflavidols A (91) and B (92) and a phenanthrenone diterpenoid trigonochinene E (106) from T. flavidus showed weak cytotoxicities against five human tumor lines, HL-60, SMMC-7721 (human hepatocellular carcinoma), A-549, MCF-7 (human breast cancer), and SW480 (human colon adenocarcinoma).24 Three degraded diterpenoids trigohowilols E (95), F (96), and G (98) from T. howii showed moderate cytotoxic activities against five human tumor cell lines HL-60, SMMC-7721, A-549, MCF-7, and SW480 with IC50 values in the range of 2.33–17.75 μM.25 Trigoxyphin T (100) showed moderate cytotoxicities against SPCA-1 and K-562 tumor cell lines with IC50 values of 1.70 and 2.24 μM, respectively; trigoxyphin O (101) showed significant cytotoxic activities against K-562 (human myelogenous leukaemia) with an IC50 value of 0.37 μM, and exhibited moderate activities against SPCA-1 and SGC-7901 (human gastric cancer) with IC50 values of 1.42 and 2.88 μM, respectively; trigoxyphin R (102) showed significant cytotoxic activity against SPCA-1 with an IC50 value of 0.24 μM and moderate activities against BEL-7402 and SGC-7901 with IC50 values of 3.89 and 5.59 μM, respectively.26 In addition, trigoxyphins J–N (105, 116–119) were evaluated for the cytotoxicities in vitro against the BEL-7402, SPCA-1, and SGC-7901 tumor cell lines, and only trigoxyphin N (119) showed activities against SPCA-1 and SGC-7901 with IC50 values of 4.08 and 5.00 μM, respectively.27
Trigonostemines A–F (155–158, 146, and 147, resp.) are β-carboline alkaloids from T. lii, and were tested against the HL-60, SMMC-7721, A-549, MCF-7 and SW480 tumor cell lines. Trigonostemine A (155) displayed cytotoxic activities against MCF-7 and SW480 cells with IC50 values of 5.30 and 4.86 μM, respectively; trigonostemine B (156) exhibited cytotoxicities against SMMC-7721, A-549, MCF-7, and SW480 cells, with IC50 values of 4.78, 8.67, 2.98, and 3.55 μM, respectively; trigonostemine D (158) showed inhibitory activity against the MCF-7 cell line with the IC50 value of 13.75 μM.39 In addition, three indole alkaloids trigonoliimines A–C (167–169) showed weak activities (10–50 μM) against tumor cell lines (U-937, human lymphoma, and HeLa, human cervical cancer).53
4.2. Antimicrobial activity
Trigoflavidols A–C (91, 92, and 106, resp.) and trigonochinene E (107) were screened for antimicrobial activities against six microbes, Monilia albicans, Pseudomonas aeruginosa, Escherichia coli, Staphylococcus aureus, 8# MRSA (MRSA, methicillin-resistant Staph. aureus), and 82# MRSA, in which trigoflavidols A (91) and B (92) displayed moderate activities against Staph. aureus, 8# MRSA, and 82# MRSA with MIC (minimum inhibitory concentration) values ranging from 1.56 to 6.25 μg mL−1.24 Trigohowilols A–F (113, 114, and 93–96, resp.) showed weak activities against Staph. aureus, 92# MRSA, and 98# MRSA with MIC values ranging from 6.25 to 25 μg mL−1.25 Trigonostemone (110) and trigonochinene (128) showed moderate antibacterial activities against Staphylococcus aureus with MICs of 2.5 and 5.0 mg mL−1, respectively.34
4.3. Antivirus activity
Trigocherriolides A–C (15–17), and trigocherrins A (20), B (21), and E (25) were evaluated in a virus-cell-based assay against three members of the genus Alphavirus (CHIKV; Sindbis virus: SINV; Semliki forest virus: SFV), and in an enzymatic assay against the NS5 RNA-dependent RNA polymerase (RdRp) of dengue virus (DENV) of the genus Flavivirus.11,13 All the tested compounds showed strong anti-CHIKV activities as shown in Table 5, and the selectivity indices (SI) of 20, 21, and 25 (SI = 23, 36, and 8, respectively) are better than those of 15–17 (SI = 2–3).11 Trigocherriolide (19) and trigocherrierin A (47) also showed strong anti-CHIKV activities with EC50 values of 0.6 ± 0.1 and 0.7 ± 0.1 μM, respectively.12 A tigliane diterpenoid, trigowiin A (141), was tested for the antiviral activity in a CHIKV virus-cell-based assay, showing weak antiviral activity with an EC50 value of 43.5 ± 12.8 μM.36
Table 5 Antiviral activities of compounds 15–17, 20, 21, and 25 in Vero cell, against CHIKV, SINV, SFV, and in a DENV RdRp assay (data in μM)a
Compd. |
Cellular assay |
DENV RdRp IC50 |
Vero cells CC50 |
CHIKV EC50 |
SINV EC50 |
SFV EC50 |
n.d. = not determined.
|
15
|
4.6 ± 0.8 |
1.9 ± 0.6 |
3.2 ± 0.1 |
4.0 |
3.1 ± 0.2 |
16
|
5.3 ± 0.2 |
2.5 ± 0.3 |
3.3 ± 0.2 |
4.2 |
16.0 ± 1.3 |
17
|
10.5 ± 0.1 |
3.9 ± 1.0 |
5.3 ± 2.1 |
5.1 ± 0.4 |
n.d. |
20
|
35 ± 8 |
1.5 ± 0.6 |
7.7 ± 1.8 |
16 ± 3 |
12.7 ± 0.2 |
21
|
93 ± 3 |
2.6 ± 0.7 |
21 ± 3 |
45.0 ± 0.6 |
n.d. |
25
|
23.1 ± 0.6 |
3.0 ± 1.2 |
5.6 ± 0.6 |
18 |
n.d. |
Chloroquine |
100 ± 25 |
10 ± 5 |
11.0 ± 2.1 |
13.8 ± 2.3 |
n.d. |
3′-Deoxy-GTP |
n.d. |
n.d. |
n.d. |
n.d. |
0.02 |
In particular, 32 daphnane diterpenoids were tested for anti-HIV activity by an assay of the inhibition of cytopathic effects of HIV-1, in which rediocides A (1), C (2), and F (4), trigonosin F (8), and trigothysoids N (9) and M (46) showed potent anti-HIV-1 activities with the EC50 values at the nanomolar level, and excellent therapy index (TI) as shown in Table 6; trigothysoids O (5), J (82) and L (66) showed very strong anti-HIV-1 activities with EC50 values of 0.358, 2.3, and 4.0 nM (TIs of 80.8, 101.2, and 74.0), respectively;10 trigonothyrin F (48) showed significant activity to prevent the cytopathic effect of HIV-1 with an EC50 value of 0.13 μg mL−1 (TI = 75.1);17 trigolins C (75) and G (80) showed modest anti-HIV-1 activity with EC50 values of 2.04 and 9.17 μg mL−1 (TI = 26.49 and >21.81), respectively.23 It was observed that the presence of macro-lactone moieties in the structurally modified daphnane diterpenoids will significantly enhance the anti-HIV-1 activity of this compound class.
Table 6 Anti-HIV activities of compounds 1, 2, 4, 8, 9, and 46 (data in nM)
Compd. |
Anti-HIV-1 EC50 |
Cytotoxicity CC50 |
TI CC50/EC50 |
1
|
0.004 ± 0.11 × 10−2 |
22.9 ± 0.64 |
6077.3 |
2
|
0.008 ± 0.98 × 10−3 |
27.9 ± 0.02 |
3245.9 |
4
|
0.001 ± 0.60 × 10−3 |
15.2 ± 13.76 |
15 242 |
8
|
0.015 ± 0.85 × 10−3 |
23.8 ± 1.49 |
1618.8 |
9
|
0.001 ± 0.76 × 10−3 |
22.2 ± 0.42 |
17 619 |
46
|
0.358 ± 0.50 × 10−2 |
28.9 ± 5.91 |
80.8 |
Azidothymidine (AZT) |
0.0008 ± — |
1317.4 ± — |
1 646 762.5 |
Some alkaloids isolated from the genus Trigonostemon also showed antiviral activity. Trifilines A (153) and B (154) displayed weak anti-HIV-1 activities with EC50 values of 54.61 and 9.75 μg mL−1 (TI = 1.52 and 1.42), respectively;40 trigonoines A (165) and B (166) also showed weak anti-HIV activities with EC50 values of 13.1 and 17.6 μg mL−1 (TI = 1.28 and 4.48), respectively.41
4.4. Other activities
Rediocide A (1) was reported to have versatile biological functions, such as an antitoxin against cobra venom, acaricidal activity against D. pteronyssinus, insecticidal activities against mosquito larvae (Aedes aegypti) and ectoparasitic flea (Ctenocephalides felis), and cytotoxic effects against tumor cell lines.3–7,54 Recently, it was reported that rediocide A activates conventional PKC (protein kinase C), which induces GPCR (G-protein-coupled receptors) desensitization and internalization, and possibly modulate other cellular events.55 In addition, trigolutesin A (171) showed weak AChE (acetylcholinesterase) inhibitory activity (14.56% inhibition) at 50 μg mL−1.43
5. Chemical synthesis
5.1. Synthesis of daphnane and tigliane diterpenoids
The daphnane and tigliane diterpenoids possess a basic carbon skeleton of the tricyclo[9.3.0.02,7]tetradecane ring system.56 These two compound classes have long been the synthetic topics, and great efforts have been made on the total synthesis of daphnane and tigliane skeletons. In 1989, Wender's group carried out the first total synthesis of a precursor of a tigliane diterpenoid of phorbol ester,57 which involved a 23-step sequence that gave 10% overall yield and 93% overall stereoselectivity. In 1991, the same research group reported a new [5 + 2] cycloaddition method to construct the B, C rings of the daphnane diterpenoids.58 Several years later, they conducted the first asymmetric total synthesis of a daphnane diterpenoid in 1997.59 In 2006, they developed a stereoselective intramolecular oxidopyrylium-alkene [5 + 2] cycloaddition route to furnish the B,C-ring system of 12-hydroxy daphnetoxins.60 One year later, they reported an efficient synthesis of the ABC tricyclic core of macrocyclic daphnane diterpenoids, namely 1α-alkyldaphnanes.61 In the same time period, Page's,56,62,63 Carreira's,64 West's,65 and Evan's66 groups also made tremendous contributions toward the total synthesis of daphnane and tigliane diterpenoids.
Wender's group also made efforts to synthesize bioactive DDOs, which are among the major metabolites of Trigonostemon plants.67 In 2011, they reported a gateway synthesis of daphnane diterpenoid 9,13,14-orthoesters as shown in Schemes 7 and 8. This strategy employed a novel Claisen rearrangement to transfer tartrate-derived chirality to a pro-C11 centre, which in turn controlled the selectivity in [5 + 2] oxidopyrylium cycloaddition. The oxygen-bridged B-ring cycloadduct was then used to control the introduction of C10 and C4 stereochemistry.68
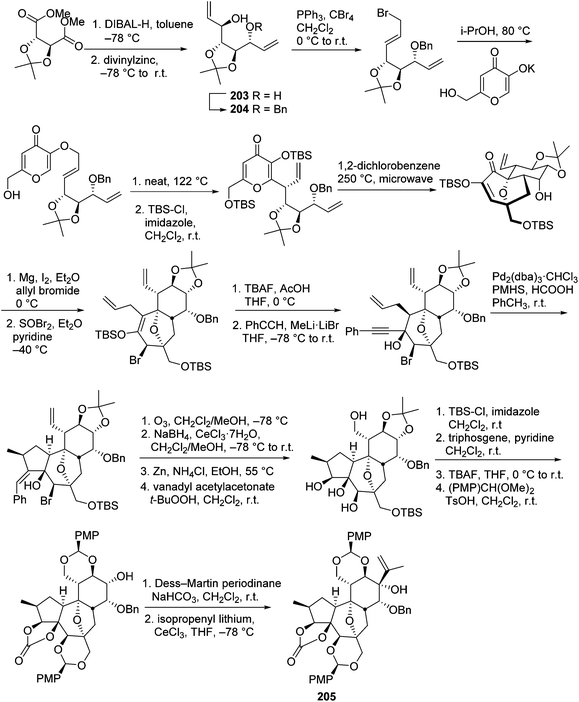 |
| Scheme 7 Synthesis of the daphnane skeleton. | |
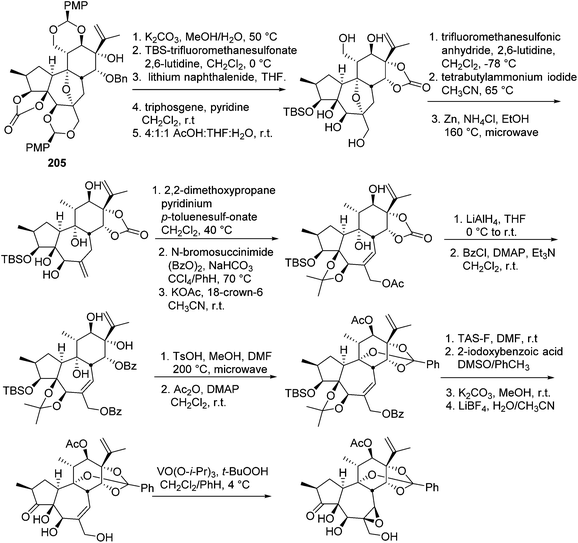 |
| Scheme 8 Synthesis of the daphnane diterpeniod 9,13,14-orthoester. | |
5.2. Synthesis of indole alkaloids: trigonoliimines A–C (167–169)
Shortly after the isolation of trigonoliimines A–C (167–169), a number of synthetic studies on the total synthesis of this class of alkaloids were reported. A retrosynthetic analysis of trigonoliimines was put forward by Movassaghi's group, in which the tryptamine heterodimer (210) (Scheme 9) was served as a common biosynthetic precursor to these alkaloids. The enantioselective total syntheses of trigonoliimines A (167) and B (168) were thus accomplished in seven steps from commercially available materials (Scheme 10), while the total synthesis of trigonoliimine C (169) was completed in eight steps by applying venerable Wagner–Merrwein rearrangement of the hydroxyindolenines (+)-212 (Scheme 11).69 In their next paper in 2014, Movassaghi's group gave full account of the syntheses of the trigonoliimine alkaloids, which remains currently the only solution to access all trigonoliimines in the enantiomerically enriched form.53
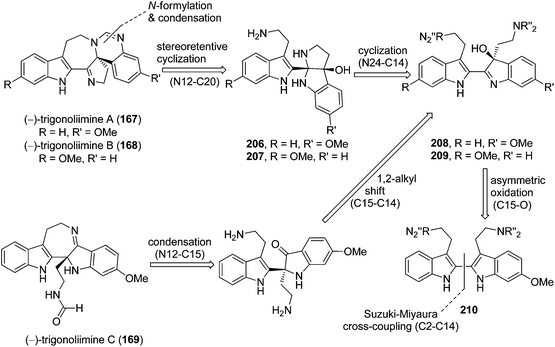 |
| Scheme 9 Retrosynthetic analysis for (−)-trigonoliimines A–C (167–169). | |
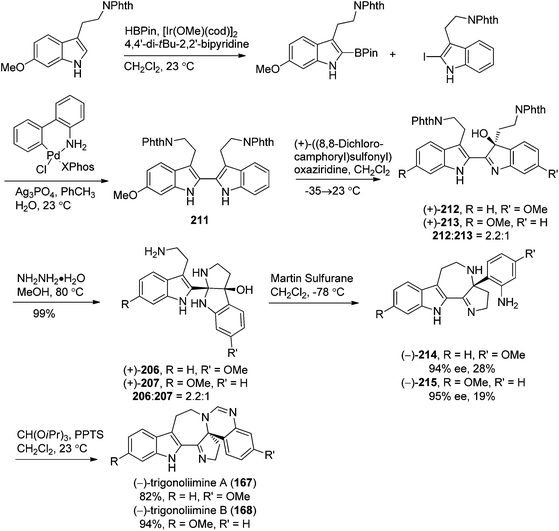 |
| Scheme 10 Movassaghi's synthesis of (−)-trigonoliimines A (167) and B (168). | |
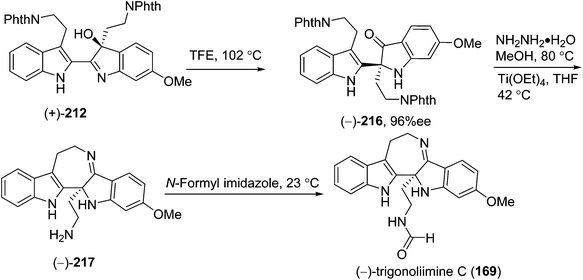 |
| Scheme 11 Movassaghi's synthesis of (−)-trigonoliimine C (169). | |
In 2011, Tambar's group completed the first convergent total synthesis of (±)-trigonoliimine C (169) in 10 steps from tryptamine and 6-methoxytryptamine (Scheme 12). They developed methods to oxidize either the electron-poor or electron-rich indole system in an unsymmetrical conjugated binary indole, which may have a broader impact on the construction of other structurally complex indole alkaloids.70
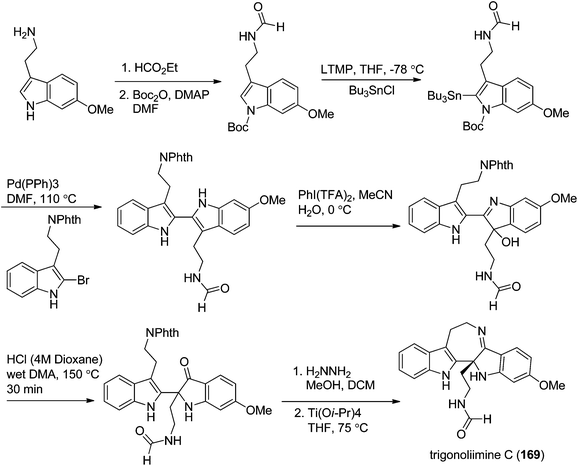 |
| Scheme 12 Tambar's synthesis of (±)-trigonoliimine C (169). | |
In 2011, Shi's group developed a new strategy to construct the hexacyclic skeleton of trigonoliimines A (167) and B (168) (Scheme 13). The key step involves a carbanion-triggered intramolecular cyclization of a seven-membered ring and a subsequent tetrahydropyrimidine ring formation in one pot. The five-membered imine of 218a and 218b was formed via an aza-Wittig reaction.71
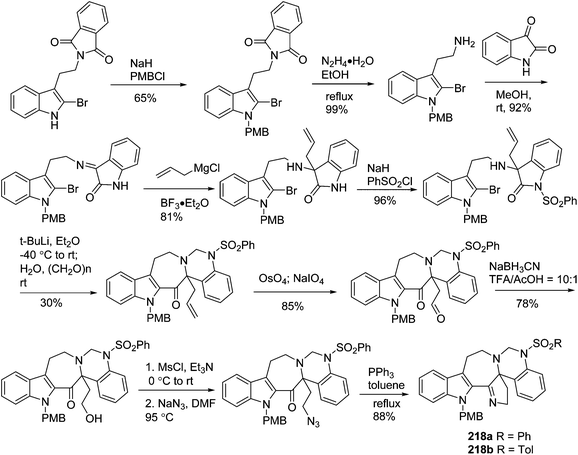 |
| Scheme 13 Shi's synthesis toward trigonoliimines A (167) and B (168). | |
One year later, Zhu's group reported a seven-step synthetic route to trigonoliimine B (168) (Scheme 14), in which they started with the functionalization of commercially available α-isocyanoacetate (219) and 2-fluoronitrobenzene (220), and ended with the unprecedented Bischler–Napieralski reaction implemented for the construction of a seven-membered ring with an overall yield of 12%.72 Further to this, Zhu's group successfully developed a catalytic enantioselective cinchona-alkaloid-catalyzed Michael addition of 222 to 223b for the synthesis of the enantiomerically enriched α,α-disubstituted α-isocyanoacetate 224 and the enantioselective total syntheses of both (+)- and (−)-trigonoliimine A (167) with an overall yield of 7.5% and 6.8%, respectively (Scheme 15).73
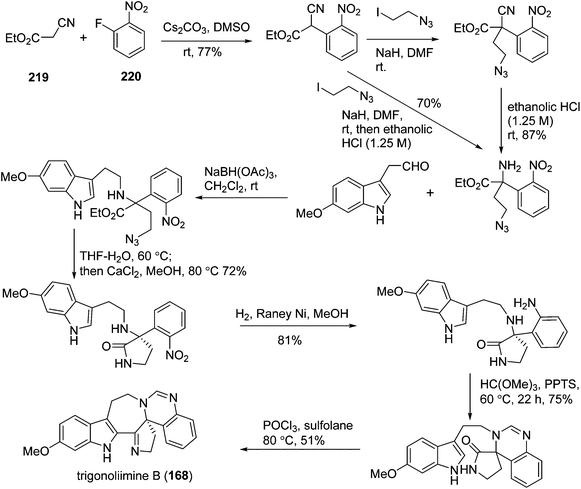 |
| Scheme 14 Zhu's synthesis of (±)-trigonoliimine B (168). | |
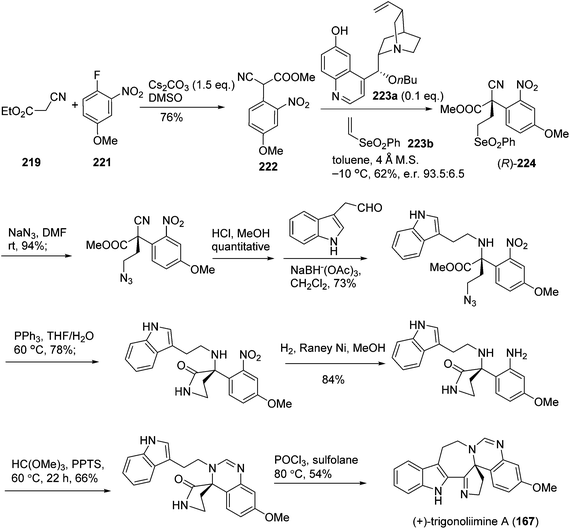 |
| Scheme 15 Zhu's synthesis of (±)-trigonoliimine A (167). | |
In 2011, Hao's group also reported the biomimetic oxidative rearrangement of the bistryptamine framework into the ring system of trigonoliimine C (169) (Scheme 16) after their discovery of these alkaloids.51 Two years later, they delivered a six-step modified synthetic route based on Zhu's work to construct the skeleton of trigonoliimines A (167) and B (168) (Scheme 17).74 Meanwhile, they further reported the total synthesis of trigonoliimine A (167), in which the Houben–Hoesch cyclization for closing the seven-membered ring and the Strecker-type reaction for assembling the precursor were involved (Scheme 18).75
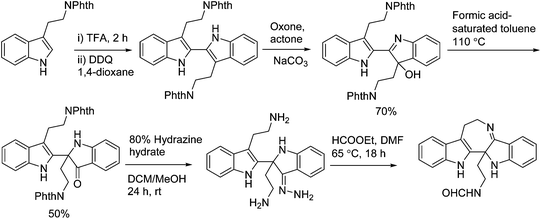 |
| Scheme 16 Hao's biomimetic synthesis toward trigonoliimine C (169). | |
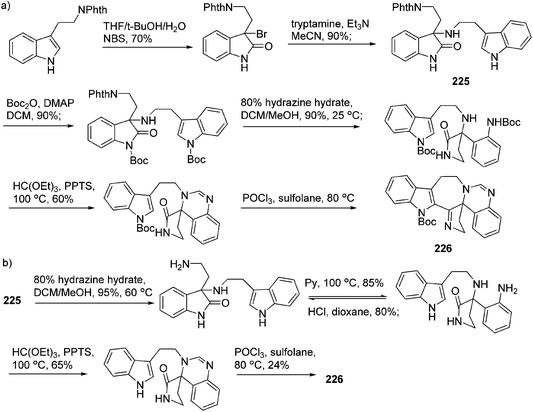 |
| Scheme 17 Hao's two alternative synthetic paths toward trigonoliimines A (167) and B (168). | |
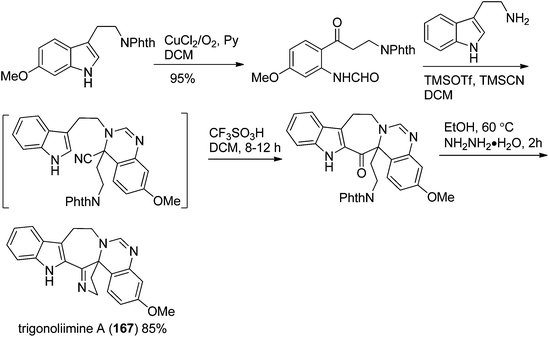 |
| Scheme 18 Hao's rapid total synthesis of (±)-trigonoliimine A (167) via a Strecker/Houben–Hoesch sequence. | |
Recently, Ramana's group reported the total synthesis of (±)-trigonoliimine C (169) which effectively used three catalytic reactions in sequence, including the [Pd]-catalyzed Sonogashira and nitroalkyne cycloisomerization reactions, and the [Au]-catalyzed C2 addition of protected tryptamine to isatogen (Scheme 19).76
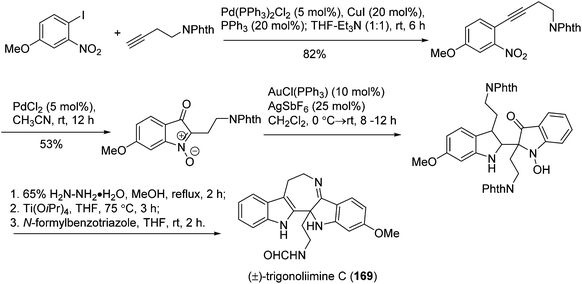 |
| Scheme 19 Ramana's catalytic total synthesis of (±)-trigonoliimine C (169). | |
6. Conclusions
In conclusion, a total of 200 structurally diverse compounds, including terpenoids, alkaloids, phenolics, and steroids, have been isolated from the plant genus of Trigonostemon in the last two decades. Among them, daphnane diterpenoids, norditerpenoid dimers, phenanthrenone diterpenoids, abietane dinorditerpenoids, 3,4-seco cleistanthane deterpenoids, and alkaloids were the major and important components. The biological activities of the diterpenoids and alkaloids were extensively evaluated, and a number of components exhibited promising cytotoxic, antimicrobial, and antivirus activities. Particularly, the anti-HIV macrocyclic daphnane diterpenoid 9,12,14-orthoesters and some alkaloids are noteworthy, and they need to be paid more attention for in-depth studies. Meanwhile, the structurally interesting daphnane diterpenoids and some alkaloids with potent biological activities have also attracted considerable interest from synthetic chemists. All these taken together will make the research on the metabolites of Trigonostemon plants a hot topic for the relevant scientific communities in the future.
Acknowledgements
Financial support from the National Natural Science Foundation (no. U1302222; 81273398) and the Foundation from the MOST (no. 2012CB721105) of the P. R. China is gratefully acknowledged.
Notes and references
-
S. K. Chen and B. Y. Chen, in Chinese Flora (Zhongguo Zhiwu Zhi), Science Press, Beijing, 1997, vol. 44, p. 162 Search PubMed.
- U. Kokpol, S. Thebpatiphat, S. Boonyaratavej, V. Chedchuskulcai, C. Z. Ni, J. Clardy, C. Chaichantipyuth, V. Chittawong and D. H. Miles, J. Nat. Prod., 1990, 53, 1148 CrossRef CAS.
- Y. G. Chen, J. C. Wu, G. Y. Chen, C. R. Han and X. P. Song, Chem. Biodiversity, 2011, 8, 1958 CAS.
- H. Jayasuriya, D. L. Zink, S. B. Singh, R. P. Borris, W. Nanakorn, H. T. Beck, M. J. Balick, M. A. Goetz, L. Slayton, L. Gregory, M. Zakson-Aiken, W. Shoop and S. B. Singh, J. Am. Chem. Soc., 2000, 122, 4998 CrossRef CAS.
- H. Jayasuriya, D. L. Zink, R. P. Borris, W. Nanakorn, H. T. Beck, M. J. Balick, M. A. Goetz, L. Gregory, W. L. Shoop and S. B. Singh, J. Nat. Prod., 2004, 67, 228 CrossRef CAS PubMed.
- N. Soonthornchareonnon, M. Sakayarojkul, M. Isaka, V. Mahakittikun, W. Chuakul and P. Wongsinkongman, Chem. Pharm. Bull., 2005, 53, 241 CrossRef CAS.
- A. Tempeam, N. Thasana, C. Pavaro, W. Chuakul, P. Siripong and S. Ruchirawat, Chem. Pharm. Bull., 2005, 53, 1321 CrossRef CAS.
- H. D. Chen, X. F. He, J. Ai, M. Y. Geng and J. M. Yue, Org. Lett., 2009, 11, 4080 CrossRef CAS PubMed.
- S. F. Li, Y. T. Di, S. L. Li, Y. Zhang, F. M. Yang, Q. Y. Sun, J. M. Simo, H. P. He and X. J. Hao, J. Nat. Prod., 2011, 74, 464 CrossRef CAS PubMed.
- Y. Y. Cheng, H. Chen, H. P. He, Y. Zhang, S. F. Li, G. H. Tang, L. L. Guo, W. Yang, F. Zhu, Y. T. Zheng, S. L. Li and X. J. Hao, Phytochemistry, 2013, 96, 360 CrossRef CAS PubMed.
- P. M. Allard, P. Leyssen, M. T. Martin, M. Bourjot, V. Dumontet, C. Eydoux, J. C. Guillemot, B. Canard, C. Poullain, F. Guéritte and M. Litaudon, Phytochemistry, 2012, 84, 160 CrossRef CAS PubMed.
- M. Bourjot, P. Leyssen, J. Neyts, V. Dumontet and M. Litaudon, Molecules, 2014, 19, 3617 CrossRef CAS PubMed.
- P. M. Allard, M. T. Martin, M. E. Tran Huu Dau, P. Leyssen, F. Gueritte and M. Litaudon, Org. Lett., 2012, 14, 342 CrossRef CAS PubMed.
- H. D. Chen, S. P. Yang, X. F. He, J. Ai, K. Z. Liu, H. B. Liu, M. Y. Geng and J. M. Yue, Org. Lett., 2010, 12, 1168 CrossRef CAS PubMed.
- B. D. Lin, M. L. Han, Y. C. Ji, H. D. Chen, S. P. Yang, S. Zhang, M. Y. Geng and J. M. Yue, J. Nat. Prod., 2010, 73, 1301 CrossRef CAS PubMed.
- S. H. Dong, C. R. Zhang, C. H. Xu, J. Ding and J. M. Yue, J. Nat. Prod., 2011, 74, 1255 CrossRef CAS PubMed.
- L. Zhang, R. H. Luo, F. Wang, Z. J. Dong, L. M. Yang, Y. T. Zheng and J. K. Liu, Phytochemistry, 2010, 71, 1879 CrossRef CAS PubMed.
- S. H. Dong, H. B. Liu, C. H. Xu, J. Ding and J. M. Yue, J. Nat. Prod., 2011, 74, 2576 CrossRef CAS PubMed.
- B. Yang, G. Y. Chen, X. P. Song, L. Q. Yang, C. R. Han, X. Y. Wu, X. M. Li and B. Y. Zou, Bioorg. Med. Chem. Lett., 2012, 22, 3828 CrossRef CAS PubMed.
- L. Yu, W. J. Zuo, W. L. Mei, Z. K. Guo, X. N. Li and H. F. Dai, Phytochem. Lett., 2013, 6, 472 CrossRef CAS PubMed.
- L. Zhang, R. H. Luo, F. Wang, M. Y. Jiang, Z. J. Dong, L. M. Yang, Y. T. Zheng and J. K. Liu, Org. Lett., 2010, 12, 152 CrossRef CAS PubMed.
- H. D. Chen, S. P. Yang, X. F. He, H. B. Liu, J. Ding and J. M. Yue, Tetrahedron, 2010, 66, 5065 CrossRef CAS PubMed.
- S. F. Li, Y. Zhang, N. Huang, Y. T. Zheng, Y. T. Di, S. L. Li, Y. Y. Cheng, H. P. He and X. J. Hao, Phytochemistry, 2013, 93, 216 CrossRef CAS PubMed.
- G. H. Tang, Y. Zhang, Y. C. Gu, S. F. Li, Y. T. Di, Y. H. Wang, C. X. Yang, G. Y. Zuo, S. L. Li, H. P. He and X. J. Hao, J. Nat. Prod., 2012, 75, 996 CrossRef CAS PubMed.
- G. H. Tang, Y. Zhang, C. M. Yuan, Y. Li, Y. C. Gu, Y. T. Di, Y. H. Wang, G. Y. Zuo, S. F. Li, S. L. Li, H. P. He and X. J. Hao, J. Nat. Prod., 2012, 75, 1962 CrossRef CAS PubMed.
- B. Yang, G. Y. Chen, X. P. Song, L. Q. Yang, C. R. Han, X. Y. Wu, C. J. Zheng, X. Ran and R. F. Tang, Tetrahedron Lett., 2013, 54, 6434 CrossRef CAS PubMed.
- B. Yang, G. Y. Chen, X. P. Song, L. Q. Yang, X. Y. Wu, C. R. Han, W. H. Chen, B. Y. Zou and X. M. Li, Bioorg. Med. Chem. Lett., 2013, 23, 5748 CrossRef CAS PubMed.
- X. J. Hu, Y. H. Wang, L. Y. Kong, H. P. He, S. Gao, H. Y. Liu, J. Ding, H. Xie, Y. T. Di and X. J. Hao, Tetrahedron Lett., 2009, 50, 2917 CrossRef CAS PubMed.
- Q. Zhu, C. P. Tang, C. Q. Ke, X. Q. Li, J. Liu, L. S. Gan, H. C. Weiss, E. R. Gesing and Y. Ye, J. Nat. Prod., 2010, 73, 40 CrossRef CAS PubMed.
- A. T. Tchinda, A. Tsopmo, M. Tene, P. Kamnaing, D. Ngnokam, P. Tane, J. F. Ayafor, J. D. Connolly and L. J. Farrugia, Phytochemistry, 2003, 64, 575 CrossRef CAS.
- L. Long, S. K. Lee, H. B. Chai, P. Rasoanaivo, Q. Gao, H. Navarro, M. E. Wall, M. C. Wani, N. R. Farnsworth, G. A. Cordell, J. M. Pezzuto and A. D. Kinghorn, Tetrahedron, 1997, 53, 15663 CrossRef CAS.
- S. Yin, Z. S. Su, Z. W. Zhou, L. Dong and J. M. Yue, J. Nat. Prod., 2008, 71, 1414 CrossRef CAS PubMed.
- Y. X. Li, W. L. Mei, W. J. Zuo, Y. X. Zhao, W. H. Dong and H. F. Dai, Phytochem. Lett., 2012, 5, 41 CrossRef CAS PubMed.
- Y. X. Li, W. J. Zuo, W. L. Mei, H. Q. Chen and H. F. Dai, Chin. J. Nat. Med., 2014, 12, 297 Search PubMed.
- G. H. Tang, H. P. He, Y. C. Gu, Y. T. Di, Y. H. Wang, S. F. Li, S. L. Li, Y. Zhang and X. J. Hao, Tetrahedron, 2012, 68, 9679 CrossRef CAS PubMed.
- M. Bourjot, L. Delang, V. H. Nguyen, J. Neyts, F. Guéritte, P. Leyssen and M. Litaudon, J. Nat. Prod., 2012, 75, 2183 CrossRef CAS PubMed.
- R. H. Cao, W. L. Peng, Z. H. Wang and A. L. Xu, Curr. Med. Chem., 2007, 14, 479 CrossRef CAS.
- X. J. Hu, Y. T. Di, Y. H. Wang, L. Y. Kong, S. Gao, C. S. Li, H. Y. Liu, H. P. He, J. Ding, H. Xie and X. J. Hao, Planta Med., 2009, 75, 1157 CrossRef CAS PubMed.
- S. F. Li, Y. Zhang, Y. Li, X. R. Li, L. M. Kong, C. J. Tan, S. L. Li, Y. T. Di, H. P. He and X. J. Hao, Bioorg. Med. Chem. Lett., 2012, 22, 2296 CrossRef CAS PubMed.
- S. F. Li, Y. Y. Chen, Y. Zhang, S. L. Li, H. P. He and X. J. Hao, Nat. Prod. Bioprospect., 2012, 2, 126 CrossRef CAS PubMed.
- S. F. Li, Y. T. Di, H. P. He, Y. Zhang, Y. H. Wang, J. L. Yin, C. J. Tan, S. L. Li and X. J. Hao, Tetrahedron Lett., 2011, 52, 3186 CrossRef CAS PubMed.
- C. J. Tan, Y. T. Di, Y. H. Wang, Y. Zhang, Y. K. Si, Q. Zhang, S. Gao, X. J. Hu, X. Fang, S. F. Li and X. J. Hao, Org. Lett., 2010, 12, 2370 CrossRef CAS PubMed.
- S. S. Ma, W. L. Mei, Z. K. Guo, S. B. Liu, Y. X. Zhao, D. L. Yang, Y. B. Zeng, B. Jiang and H. F. Dai, Org. Lett., 2013, 15, 1492 CrossRef CAS PubMed.
- T. Kanchanapoom, R. Kasai, P. Chumsri, K. Kraisintu and K. Yamasaki, Tetrahedron Lett., 2002, 43, 2941 CrossRef CAS.
-
T. Wang-amnauyporn, Insect antifeedants from the roots of Trigonostemon reidioides Craib, Thesis (M.Sc.), Chulalongkorn University, 1998 Search PubMed.
- L. Yu, W. L. Mei, W. J. Zuo, H. Wang, Z. K. Guo, X. Q. An and H. F. Dai, Lishizhen Med. Mater. Med. Res., 2013, 24, 591 CAS.
- S. S. Ma, W. L. Mei, Y. B. Zeng, S. B. Liu, W. H. Dong, B. Jiang and H. F. Dai, Chin. J. Med. Chem., 2013, 23, 47 CAS.
- Y. X. Li, W. J. Zuo, X. N. Li, W. L. Mei and H. F. Dai, J. Asian Nat. Prod. Res., 2014, 16, 549 CrossRef CAS PubMed.
- H. J. Kim, E. R. Woo and H. Park, J. Nat. Prod., 1994, 57, 581 CrossRef CAS.
- C. C. Cen, G. Y. Chen, C. R. Han and X. P. Song, Acta Crystallogr., Sect. E: Struct. Rep. Online, 2009, 65, 02815 Search PubMed.
- S. Liu and X. J. Hao, Tetrahedron Lett., 2011, 52, 5640 CrossRef CAS PubMed.
- Y. Rukayadi, J. S. Shim and J. K. Hwang, Mycoses, 2008, 51, 308 CrossRef PubMed.
- S. Han, K. C. Morrison, P. J. Hergenrother and M. Movassaghi, J. Org. Chem., 2014, 79, 473 CrossRef CAS PubMed.
- M. Utsintong, A. Kaewnoi, W. Leelamanit, A. J. Olson and O. Vajragupta, Chem. Biodiversity, 2009, 6, 1404 CAS.
- L. X. Cui, J. Li and X. Xie, J. Nat. Prod., 2012, 75, 1058 CrossRef CAS PubMed.
- P. C. B. Page and D. C. Jennens, J. Chem. Soc., Perkin Trans. 1, 1992, 2587 RSC.
- P. A. Wender, H. Y. Lee, R. S. Wilhelm and P. D. Williams, J. Am. Chem. Soc., 1989, 111, 8954 CrossRef CAS.
- P. A. Wender and J. L. Mascareñas, J. Org. Chem., 1991, 56, 6267 CrossRef CAS.
- P. A. Wender, C. D. Jesudason, H. Nakahira, N. Tamura, A. L. Tebbe and Y. Ueno, J. Am. Chem. Soc., 1997, 119, 12976 CrossRef CAS.
- P. A. Wender, F. Christopher Bi, N. Buschmann, F. Gosselin, C. Kan, J. M. Kee and H. Ohmura, Org. Lett., 2006, 8, 5373 CrossRef CAS PubMed.
- P. A. Wender, N. D'Angelo, V. I. Elitzin, M. Ernst, E. E. Jackson-Ugueto, J. A. Kowalski, S. Mckendry, M. Rehfeuter, R. Sun and D. Voigtlaender, Org. Lett., 2007, 9, 1829 CrossRef CAS PubMed.
- P. C. B. Page, D. C. Jennens and H. McFarland, Tetrahedron Lett., 1997, 38, 5395 CrossRef CAS.
- P. C. B. Page, D. C. Jennens and H. McFarland, Tetrahedron Lett., 1997, 38, 6913 CrossRef CAS.
- S. R. Jackson, M. G. Johenson, M. Mikami, S. Shiokawa and E. M. Carreira, Angew. Chem., Int. Ed., 2001, 40, 2694 CrossRef CAS.
- C. Stewart, R. McDonald and F. G. West, Org. Lett., 2011, 13, 720 CrossRef CAS PubMed.
- A. J. Catino, A. Sherlock, P. Shieh, J. S. Wzorek and D. A. Evans, Org. Lett., 2013, 15, 3330 CrossRef CAS PubMed.
- S. G. Liao, H. D. Chen and J. M. Yue, Chem. Rev., 2009, 109, 1092 CrossRef CAS PubMed.
- P. A. Wender, N. Buschmann, N. B. Cardin, L. R. Jones, C. Kan, J. M. Kee, J. A. Kowalski and K. E. Longcore, Nat. Chem., 2011, 19, 615 CrossRef PubMed.
- S. Han and M. Movassaghi, J. Am. Chem. Soc., 2011, 133, 10768 CrossRef CAS PubMed.
- X. B. Qi, H. L. Bao and U. K. Tambar, J. Am. Chem. Soc., 2011, 133, 10050 CrossRef CAS PubMed.
- P. J. Feng, Y. K. Fan, F. Z. Xue, W. G. Liu, S.
L. Li and Y. A. Shi, Org. Lett., 2011, 13, 5827 CrossRef CAS PubMed.
- T. Buyck, Q. Wang and J. P. Zhu, Org. Lett., 2012, 14, 1338 CrossRef CAS PubMed.
- T. Buyck, Q. Wang and J. P. Zhu, Angew. Chem., Int. Ed., 2013, 52, 12714 CrossRef CAS PubMed.
- J. Qiu, J. X. Zhang, S. Liu and X. J. Hao, Tetrahedron Lett., 2013, 54, 300 CrossRef CAS PubMed.
- B. Zhao, X. Y. Hao, J. X. Zhang, S. Liu and X. J. Hao, Org. Lett., 2013, 15, 528 CrossRef CAS PubMed.
- B. N. Reddy and C. V. Ramana, Chem. Commun., 2013, 49, 9767 RSC.
|
This journal is © the Partner Organisations 2014 |