DOI:
10.1039/D1TB01980E
(Paper)
J. Mater. Chem. B, 2022,
10, 2463-2470
Carboxy-terminal dendrimers with phenylalanine for a pH-sensitive delivery system into immune cells including T cells†
Received
9th September 2021
, Accepted 4th December 2021
First published on 7th December 2021
Abstract
Although T cells play important roles in various immune reactions, there are only a few reports on delivery systems into T cells. Our previous study showed that carboxy-terminal phenylalanine (Phe)-modified polyamidoamine (PAMAM) dendrimers have both temperature- and pH-sensitive properties, which are affected by the chemical structure. The self-assembled structures of Phe, observed in phenylketonuria, enhance the protein aggregation, the association with the cell membrane and the membrane permeability. In this study, we applied the Phe-modified dendrimers to a pH-sensitive drug delivery system into T cells. Dendrimers with different amino acids and acid anhydrides were synthesized, and their pH-responsive association with T cells and their subsets was investigated. The dendrimers modified with Phe and cyclohexanedicarboxylic acid (CHex) showed higher uptake into various cells, including Jurkat cells, CD3+ T cells, CD3 + CD4+ helper T cells and CD3 + CD8+ killer T cells. These dendrimers were internalized into T cells via endocytosis, and their cellular uptake was enhanced under weak acidic conditions (pH 6.5). Our results showed that Phe- and CHex-modified dendrimers have a delivery potential to T cells and their subsets, which may be useful for cancer immunotherapy.
1. Introduction
The application of stimuli-responsive materials to drug delivery systems (DDS) is of great importance, since these materials change their properties when they are exposed to external stimuli, such as temperature, ultrasonication, and magnetic fields, and internal stimuli, such as pH, reduction environments, and environment-specific enzymes.1 pH-responsive DDS have been studied by many researchers because anaerobic glycolysis produces lactic acid in tumor tissues, which creates acidic tumor microenvironments.2 In addition, cells endocytose extracellular substances, whose late endosomes and lysosomes become acidic.2 Therefore, pH-sensitive DDS is useful for tumor-targeted drug delivery as well as for endosomal escape.2 Dendrimers are highly branched macromolecules with well-defined structures, and their unique properties differ from those of linear polymers. Since dendrimers can encapsulate and/or modify various bioactive compounds, such as drug molecules, they are potent nanoplatforms for DDS. Polyamidoamine (PAMAM) dendrimers, which are commercially available, have a large number of inner tertiary amino groups, which can work as pH-sensitive units. Acidic compounds can easily be encapsulated due to their electrostatic interactions with tertiary amino groups, and the binding affinity increases with the decrease in pH.3
Our previous report indicates that the amino-terminal PAMAM dendrimers with phenylalanine (Phe) have temperature- and pH-responsive properties in the ranges larger than pH 7.4 Also, carboxy-terminal Phe-bearing PAMAM dendrimers, which were synthesized by reacting with Phe and succinyl anhydride (Suc), showed temperature- and pH-responsive properties in a different manner.5,6 A PAMAM dendrimer modified with Suc and Phe in this order (PAMAM-Suc-Phe) showed UCST-type thermosensitivity at pH 6,5 but the PAMAM dendrimer modified with Phe and Suc in this order (PAMAM-Phe-Suc) showed both LCST- and UCST-type thermosensitivity at pH 4 and 6, respectively.6 Phenylketonuria (PKU) is a metabolic disorder disease which induces an elevated concentration of Phe.7 Fibril structures are formed in a high concentration of Phe because of the self-assembly. The self-assembled structures of Phe enhance the protein aggregation, the association with the cell membrane and the membrane permeability, which are known to be the cause of PKU.7 These inspired us to use Phe-modified dendrimers as a potent drug carrier with pH-sensitive properties.
Cancer immunotherapy is a cancer treatment method that attacks tumor cells by activating the immune systems of patients.8 Immune surveillance against cancer comprises various kinds of immune cells, in which T cells play important roles. T cells are classified into various kinds of subsets. CD4- and CD8-positive T cells are known as helper T cells and cytotoxic T cells, respectively. Helper and cytotoxic T cells control immune reactions and induce cell death, respectively. These subsets are a possible target in cancer immunotherapy. It has been reported that lactate produced in tumor tissues impairs the migration of T cells by reducing the expression of chemokine receptors. Also, excess lactate regulates macrophage polarization and suppresses the functions of killer cells.9 Thus, the upregulation of T cells in acidic tumor microenvironments is important for efficient cancer immunotherapy. T cells are activated by mediating with phagocytic immune cells, such as macrophages and dendritic cells,10 because direct delivery into T cells is difficult. There are many reports on pH-sensitive DDS into antigen-presenting cells, in which endosomal escape can be induced.10 However, there are only a few reports of delivery systems to T cells.11,12 Schmid et al. and Cao et al. developed CD8- and CD4-conjugated poly(lactic-co-glycolic acid) (PLGA) nanoparticles for T cell-targeted delivery, respectively.11 They were used for the antibodies that can be targeted at the cell surface of T cells. Because efficient delivery into T cells using antibodies is difficult, the delivery systems into T cells still need to be developed.
We previously reported that the anionic terminal PAMAM dendrimers whose generation is greater than generation-4 (G4) were efficiently accumulated in lymph nodes via intradermal injection.13 The sentinel lymph node is the first organ to drain malignant tumor cells. Lymph node-targeted delivery, especially delivery into lymph node-resident T cells, is necessary for cancer immunotherapy. Previously, 2-carboxy-cyclohexanolated (CHex) PAMAM dendrimer with Phe, PAMAM-CHex-Phe, was well associated with lymph node-resident lymphocytes, including T cells.14 In this study, we attempted to construct a pH-sensitive delivery system into T cells by using carboxy-terminal Phe- and CHex-modified dendrimers with different structures, PAMAM-CHex-Phe and PAMAM-Phe-CHex. Other types of hydrophobic carboxy-terminal dendrimers were also synthesized by changing the amino acid (leucine (Leu)), acid anhydrides, and reaction order (Fig. 1), to investigate the relationship between the dendrimer structure and the delivery ability into T cells. The T cell association of these dendrimers was examined at different pHs and temperatures using fluorescence-activated cell sorting (FACS), where murine splenocytes stained with an anti-CD3 antibody were used. Also, we examined the association of PAMAM-CHex-Phe and PAMAM-Phe-CHex with some culture cell lines and T cell subsets, such as CD4-positive helper T cells (CD3+CD4+), CD8-positive killer T cells (CD3+CD8+) and activated T cells (CD3+CD69+). The intracellular distribution of PAMAM-CHex-Phe and PAMAM-Phe-CHex was observed using confocal microscopic imaging. This is the first report on the direct delivery into T cells using pH-sensitive DDS, as far as we know.
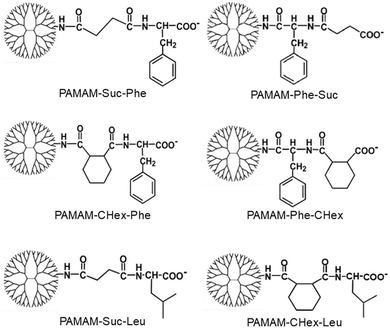 |
| Fig. 1 Structure of the dendrimers used for the delivery into T cells in this study. | |
2. Experimental
2.1 Materials
PAMAM-Suc-Phe, PAMAM-CHex-Phe, and PAMAM-Phe-Suc were synthesized in accordance with our previous reports.5,6 PAMAM-Phe-CHex was synthesized in the same procedure as for PAMAM-Phe-Suc by replacing Suc with CHex. PAMAM-Suc-Leu and PAMAM-CHex-Leu were synthesized using the same procedures as for PAMAM-Suc-Phe and PAMAM-CHex-Phe by replacing Phe with Leu. These carboxy-terminal dendrimers were labeled with fluorescein isothiocyanate (FITC) in accordance with our previous report.14 The bound number of FITC to each dendrimer was estimated from the absorbance of the FITC-conjugated dendrimers at 495 nm. The detailed synthetic procedures are shown in the ESI.† Phycoerythrin (PE)-conjugated antibody (CD3-PE (clone REA641)) and allophycocyanin (APC)-conjugated antibodies (CD69-APC (clone REA937), CD4-APC (clone REA604), and CD8a-APC (clone REA601)) were purchased from Miltenyi Biotec GmbH, Ltd. (Bergish Gladbach, Germany). Concanavalin A (Con. A) was purchased from Nacalai Tesque, Inc. (Kyoto, Japan). 4T1 cells and Jurkat cells were purchased from American Type Culture Collection (ATCC, VA, USA), and RAW264 cells were obtained from RIKEN BRC (Ibaraki, Japan). Jurkat cells were cultured in a humidified incubator at 37 °C with 5% CO2 using Roswell Park Memorial Institute-1640 (RPMI-1640) medium containing 10% of fetal bovine serum (FBS). 4T1 cells and RAW264 cells were cultured using 10% FBS-containing Dulbecco's modified Eagle's medium (DMEM) and minimum essential medium (MEM), respectively. LysoTracker Red DND-99 and Cellstain DAPI solution were purchased from Thermo Fisher Scientific (Waltham, MA, USA) and Dojindo Laboratories (Kumamoto, Japan), respectively.
2.2 Association of dendrimers with T cells
FACS was performed using the same method as in our previous reports except for the immunostaining and the FACS machine.14 Briefly, spleens were collected from BALB/c mice (7–8-week-old, female) after perfusion with phosphate-buffered saline (PBS) containing heparin under anesthesia. The splenocytes were obtained after digestion with collagenase for 30 min at 37 °C. Each FITC-labeled dendrimer (fluorescent dye concentration: 5 μM) was incubated with 2 × 105 cells in an RPMI-1640 medium for 3 h at 37 °C or 4 °C with and without pH adjustment to pH 6.5. The cells were washed and immunostained with CD3-PE, CD4-APC, and CD8a-APC according to the manufacturer's instruction. The stained and unstained cells were evaluated by FACS using BD FACS Aria TM III Cell Sorter (Becton, Dickinson and Company, Franklin Lakes, NJ). The mean green fluorescence intensity of the singly stained cells with CD3-PE and double-stained cells with CD3-PE and CD4-APC or CD8-APC was measured. The cells treated at pH 6.5 were double-stained with CD3-PE and CD69-APC prior to FACS to confirm the T cell activity at pH 6.5.
The splenocytes collected from BALB/c mice were incubated in 10% FBS-containing an RPMI 1640 medium supplemented with 50 μM of 2-mercaptoethanol at a concentration of 3 × 106 cells per mL. Then, these cells were cultured in a medium containing Con. A (1 μg mL−1) for 2 days. Afterward, the FITC-labeled dendrimers were incubated under different conditions, as described above. These cells were double-stained with CD3-PE and CD69-APC according to the manufacturer's instructions. The mean green fluorescence intensity of the double-stained cells with CD3-PE and CD69-APC (CD3 + CD69+ cells) and the singly stained cells with CD3-PE (CD3 + CD69- cells) was measured.
The animal experiments were performed in accordance with the protocols approved by the Animal Care and Use Committees of Osaka Prefecture University.
2.3 Association of dendrimers with various cell lines
25 μL of RPMI solution containing FITC-labeled dendrimers (fluorescent dye concentration: 5 μM) were added to 1 × 105 Jurkat cells and incubated at 37 °C or 4 °C for 3 h. Then, 375 μL of PBS was added to the cell suspension and centrifugated (2000 rpm, 4 °C, 10 min) to collect cells. After washing with 400 μL of PBS, FACS was performed by using GUAVA Incyte, (Luminex, Japan). Adherent RAW264 cells and 4T1 cells were seeded in a 24-well plate at 1.4 × 105 cells per well. After culturing for 24 h, 200 μL of dendrimer solution (fluorescent dye concentration: 5 μM) was added. After the incubation at 37 °C or 4 °C for 3 h, RAW264 cells and 4T1 cells were washed twice with PBS with and without ethylenediaminetetraacetic acid (EDTA, 0.05%), respectively. RAW264 cells and 4T1 cells were detached from the substrate using 200 μL of 0.25% trypsin for 5 min and 2 min, respectively. A further 200 μL of PBS was added to these cell suspensions, and centrifugation was performed (2000 rpm, 4 °C, 10 min) to collect the cells. After washing with 400 μL of PBS, FACS analysis was performed using GUAVA Incyte. The same procedure was performed by using dendrimer-containing medium adjusted to pH 6.0 or 6.5 and in the presence of free Phe.
2.4 Confocal microscopic observation
The association of FITC-labeled dendrimers with Jurkat cells was carried out, as described in 2.3. 75 nM of LysoTracker Red was added to the cells and incubated at 37 °C. After 1.5 h, the DAPI stain was added to the medium whose final concentration of DAPI was 0.7 μg mL−1. After 30 min, cells were collected by centrifugation (3000 rpm, 4 °C, 5 min). After the resuspension of cells using serum-containing RPMI medium, cells were mounted on a glass bottom dish covered with Shi-fix Coverslip (Everest Biotech Ltd, Oxfordshire, UK). After 30 min at 37 °C, unbound cells were removed by washing with PBS. The cells were observed using a FV1200 confocal laser scanning microscope (Olympus, Tokyo, Japan).
3. Results
3.1 Synthesis and pH sensitivity of the carboxy-terminal dendrimers bearing Phe
Different kinds of hydrophobic carboxy-terminal PAMAM dendrimers were synthesized, as shown in Fig. 1, to compare the pH-sensitive properties and the association properties of T cells and their subsets. Previously, PAMAM-CHex-Phe was applied to a delivery into lymph node-resident immune cells.14 PAMAM-CHex-Phe was synthesized, as shown in Fig. 2A.5 Anhydride of CHex was reacted with the PAMAM dendrimer of generation 4 (G4) prior to the reaction of benzyl ester of Phe (Phe-OBzl). The reaction order of Phe and CHex was changed to produce PAMAM-Phe-CHex (Fig. 2B). Amino-terminal protected Phe (Boc-Phe) was first reacted with the PAMAM dendrimer of G4.15 After the deprotection by trifluoroacetic acid (TFA), anhydride of CHex was reacted to produce PAMAM-Phe-CHex. PAMAM-Suc-Phe, PAMAM-CHex-Phe, and PAMAM-Phe-Suc were already synthesized in our previous reports.5,6 PAMAM-Suc-Leu and PAMAM-CHex-Leu, were also synthesized in the same synthetic procedure by replacing Phe with Leu. The synthesized dendrimers were characterized by 1H NMR analyses (Fig. S1–S3 (ESI†), respectively). The bound numbers of Suc and Leu were evaluated using the integral ratios of the signals at 2.4 ppm and 4.2 ppm, respectively, to that of the PAMAM signal in the spectra of PAMAM-Suc-Leu (Fig. S1, ESI†). Since the Suc signal was overlapped with the dendrimer signal, the integral ratio of Suc was calculated by subtracting that of the dendrimer signal. The bound numbers of CHex, Leu, and Phe were evaluated using the integral ratios of the signals at 1.1–1.9 ppm, 0.9 ppm, and 4.2–4.4 ppm, respectively, to those at 2.2–2.4 ppm derived from PAMAM, in the spectra of PAMAM-CHex-Leu and PAMAM-Phe-CHex (Fig. S2 and S3, ESI†). The synthesized dendrimers are listed in Table 1. Almost all the terminal groups of the dendrimer were modified with dicarboxylic acids and amino acids. Each dendrimer was labeled with a green fluorescent dye, FITC, for the detection of the cell-associated dendrimers. The synthetic procedure was also shown in our previous report.14 As listed in Table 1, three to nine FITC molecules were conjugated to the dendrimer. The hydrophobicity indices, log P values, of the terminal groups in PAMAM-Suc-Phe, PAMAM-Suc-Leu, PAMAM-CHex-Phe, and PAMAM-CHex-Leu were calculated as −0.27, −0.71, 1.2, and 0.76, respectively. The log
P values of PAMAM-Phe-CHex and PAMAM-Phe-Suc were the same as those of PAMAM-CHex-Phe and PAMAM-Suc-Phe, respectively (Table 1). Thus, PAMAM-CHex-Phe and PAMAM-Phe-CHex were the most hydrophobic ones among them.
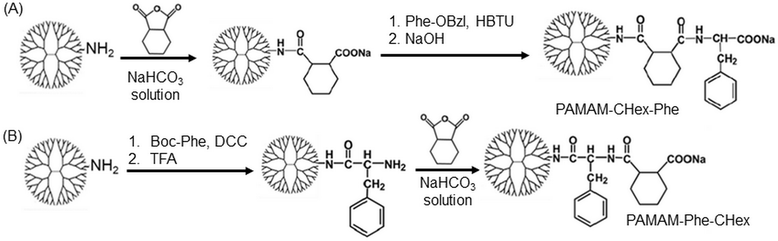 |
| Fig. 2 Synthetic scheme of the two kinds of PAMAM dendrimers bearing Phe and CHex. (A) PAMAM-CHex-Phe and (B) PAMAM-Phe-CHex. DCC and HBTU are dicyclohexyl carbodiimide and 2-(1H-benzotriazole-1-yl)-1,1,3,3-tetramethyluronium hexafluorophosphate, respectively, which are condensation agents. TFA is trifluoroacetic acid. | |
Table 1 Summary of carboxy-terminal dendrimers used in this study
Dendri-mer |
Bound number |
log Pa |
Turbidb |
Suc/CHex |
Phe/Leu |
FITC |
Calculated log P of the dendrimer terminal group using ChemDraw software.
Transmittance became less than 20%.
Not determined.
|
PAMAM-Suc-Phe |
64 |
64 |
7.4 |
−0.27 |
pH4 |
PAMAM-Suc-Leu |
64 |
64 |
6.7 |
−0.71 |
NDc |
PAMAM-CHex-Phe |
64 |
64 |
6.9 |
1.2 |
<pH6 |
PAMAM-CHex-Leu |
64 |
64 |
9.1 |
0.76 |
NDc |
PAMAM-Phe-Suc |
57 |
56 |
3.2 |
−0.27 |
pH5 |
PAMAM-Phe-CHex |
64 |
64 |
3.9 |
1.2 |
<pH7 |
Our previous reports indicate that PAMAM-Suc-Phe, PAMAM-CHex-Phe, and PAMAM-Phe-Suc have efficient pH- and temperature-responsive properties, but Leu/Ile-conjugated dendrimers do not show any significant stimuli-responsive properties.4–6 The carboxy-terminal Phe-bearing dendrimers were soluble under an alkaline condition. However, they were turbid in acidic pH (Fig. S4, ESI†). PAMAM-Suc-Phe and PAMAM-Phe-Suc were turbid below pH 4 and pH 5, respectively. The solution of PAMAM-Phe-Suc, which has both UCST- and LCST-type thermosensitive properties at different pHs, became clear below pH 4.7 PAMAM-CHex-Phe and PAMAM-Phe-CHex were turbid below pH 6 and pH 7, respectively (Fig. S4, ESI†). Since PAMAM-CHex-Phe and PAMAM-Phe-CHex are more hydrophobic compared with the other ones, the carboxylate ions in these dendrimers were protonated at higher pH.6 Also, the Phe-terminal dendrimers, PAMAM-Suc-Phe and PAMAM-CHex-Phe, responded to lower pH than the CHex/Suc-terminal dendrimers, PAMAM-Phe-Suc, and PAMAM-Phe-CHex. This is because the pKa of the carboxy group in Phe is 2.2, which is lower than that of carboxylic acid compounds.
3.2 Association of the carboxy-terminal dendrimers bearing Phe with T cells
The association of the synthesized six kinds of dendrimers with T cells was examined. The splenocytes were collected from mice and incubated with FITC-labeled dendrimers. T cells were immunostained with CD3-PE, and the association of each dendrimer with T cells was examined using FACS. The dendrimer-derived green FITC signal in the CD3-positive (CD3+) cells was examined. Fig. 3 shows that PAMAM-CHex-Phe and PAMAM-Phe-CHex were efficiently associated with T cells, but the other ones were not. Thus, the combination of CHex and Phe in the dendrimer is important for the association with T cells. Selectivity of the cell association is also important in DDS. Fig. S5 (ESI†) shows the cell distribution of CD3-PE-stained splenocytes, which were associated with PAMAM-Phe-CHex and PAMAM-CHex-Phe, in the FACS analysis. FITC signal was detected from both CD3-PE-stiained and unstained cells, suggesting that these dendrimers associated with not only T cells but also other cells. The same experiment was performed at 4 °C, and the mean fluorescence intensities were compared to those at 37 °C. Fig. 4 shows that the fluorescence intensity of all the dendrimers was lower at 4 °C than at 37 °C. This suggests that these dendrimers enter into cells via endocytosis. The fluorescence intensities of PAMAM-CHex-Phe and PAMAM-Phe-CHex were relatively high even at 4 °C compared with the other ones. This suggests that some portions of these dendrimers may be adhered to the cell surface due to hydrophobic interactions. Next, we investigated the association of each dendrimer with T cells at pH 6.5. The mean fluorescence intensities of some dendrimers increased at pH 6.5. Especially, PAMAM-Phe-CHex significantly increased (Fig. 4). The solution containing PAMAM-Phe-CHex became turbid below pH 7, but that containing PAMAM-CHex-Phe did not (Table 1). These suggested that PAMAM-Phe-CHex was assembled at around pH 6.5 via hydrophobic interaction. Enhanced hydrophobicity below pH 7 can promote the T cell association. Since the microenvironments of tumor tissues are known to be weakly acidic (pH 6.3–6.9),2 pH-sensitive properties are useful for the selective association with T cells in and around tumor tissues.
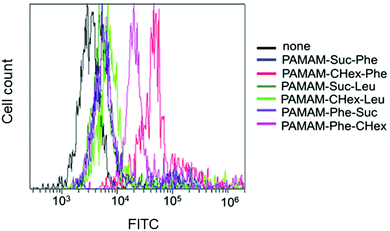 |
| Fig. 3 FACS histograms of the CD3+ T cells at 37 °C. | |
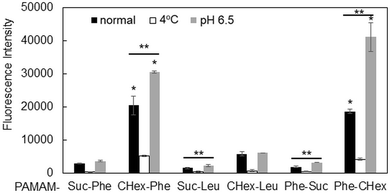 |
| Fig. 4 Mean fluorescence intensity of each dendrimer associated with CD3+ T cells under different conditions. *Higher and p < 0.05 vs. PAMAM-CHex-Phe at 4 °C. **Higher and p < 0.05 vs. each normal condition. | |
3.3 Association of the carboxy-terminal dendrimers bearing Phe with Jurkat cells and other cells
Our results showed that PAMAM-Phe-CHex and PAMAM-CHex-Phe were associated with CD3-PE-stained T cells. Since there are various immune cells including T cells in splenocytes, we used Jurkat cells, human T cell leukemia cells, as a model of T cells in order to further investigate the association of these dendrimers with T cells. Fig. S6a (ESI†) shows that PAMAM-CHex-Phe was efficiently associated with Jurkat cells, but PAMAM-Phe-CHex was not. The fluorescence intensity was lower at 4 °C than at 37 °C, which was consistent with the results using splenocyte-derived T cells. This suggests that these dendrimers enter into cells via endocytosis. But, the pH-sensitive behavior was different: The mean fluorescence intensity of PAMAM-CHex-Phe much increased at acidic pH, but that of PAMAM-Phe-CHex did not. It is possible that the association mechanism of these dendrimers with Jurkat cells may be different from murine splenocytes. Confocal microscopic observations were made to confirm the internalization of these dendrimers into T cells. Fig. 5 shows that both dendrimers were observed inside Jurkat cells as dotted structures, indicating that both dendrimers were internalized into T cells via endocytosis. Interestingly, most of these spots did not co-localize with Lysotracker (Fig. S7, ESI†). This suggests that these dendrimers possibly inhibited the maturation from endosomes to lysosomes. It was reported that an amino-terminal PAMAM dendrimer inhibited the maturation to lysosomes,17 consistent with our results. The association of these dendrimers with Jurkat cells was examined in the presence of free Phe to investigate the effect of Phe to the association. Fig. S8 (ESI†) shows that the dendrimer association slightly increased with increasing Phe up to 100 μM and then decreased at 200 μM. These suggest that free Phe has both positive and negative effects in the association of the dendrimer. The negative effect is the suppression of Phe-based association of the dendrimer. It is known that the self-assembled structures of Phe enhance the association with the cell membrane and the membrane permeability in PKU.7 Thus, free Phe possibly assist the association of the dendrimer with cells, which is a possible positive effect. Further investigation is necessary to elucidate the mechanism of the cellular uptake.
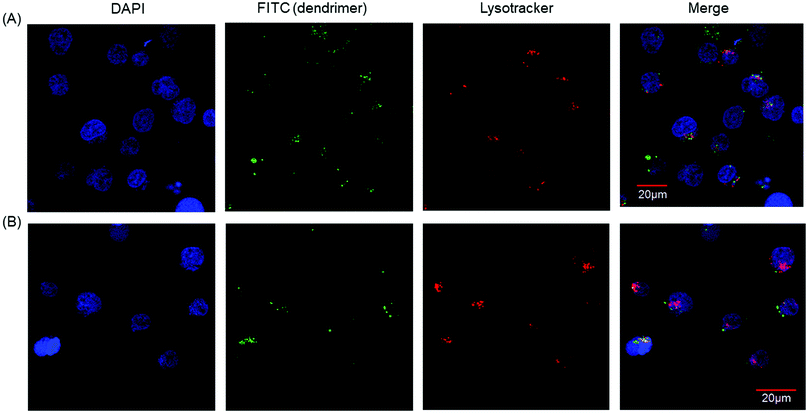 |
| Fig. 5 Confocal microscopic images of Jurkat cells associated with (A) PAMAM-CHex-Phe and (B) PAMAM-Phe-CHex. | |
Next, the association of these dendrimers with RAW264 cells (macrophage-like cells) and 4T1 cells (breast tumor cells) was examined to investigate the cell binding specificity of these dendrimers to T cells. Fig. S6b and c (ESI†) show that both dendrimers were also associated with RAW264 cells and 4T1 cells. The fluorescence intensity of these dendrimers was lower at 4 °C than at 37 °C, suggesting that these dendrimers were internalized into these cells via endocytosis like splenocyte-derived T cells and Jurkat cells. The fluorescence intensity of PAMAM-Phe-CHex increased at low pH, but PAMAM-CHex-Phe did not, which was similar to splenocyte-derived T cells. Because the experimental conditions of these adhesive cells were different from floating splenocytes and Jurkat cells, the direct comparison of the amount of the cell-associated dendrimer was difficult. Further investigation and improvement are required for the selective delivery of the dendrimers into T cells.
3.4 Association of PAMAM-Phe-CHex and PAMAM-CHex-Phe with T cell subsets
Fig. 4 shows that PAMAM-Phe-CHex and PAMAM-CHex-Phe have the ability of pH-sensitive delivery into T cells. T cells are classified into CD4-positive (CD4+) helper T cells and CD8-positive (CD8+) killer T cells, whose functions are different. We examined the delivery of these dendrimers to these T cell subsets after double staining with CD3-PE (yellow) and CD4-APC or CD8a-APC (red). The green fluorescence signals of each dendrimer in CD3+CD4+ and CD3+CD8+ cells were examined using FACS. Fig. 6 shows that the associations of PAMAM-CHex-Phe and PAMAM-Phe-CHex with CD3+CD4+ and CD3+CD8+ cells were similar, indicating that both dendrimers interacted with both the helper and killer T cells. At 4 °C, the associations of both dendrimers with these T cell subsets significantly decreased, indicating that both dendrimers were internalized into helper and killer T cells via endocytosis. At pH 6.5, the associations of both dendrimers with the CD3+CD4+ and CD3+CD8+ cells increased. More PAMAM-Phe-CHex was associated with these T cell subsets at pH 6.5 than PAMAM-CHex-Phe, which is similar to the results shown in Fig. 4. Thus, PAMAM-Phe-CHex and PAMAM-CHex-Phe have a delivery potential into helper T cells and killer T cells in a pH-sensitive manner, and PAMAM-Phe-CHex is more useful for pH-sensitive delivery to these T cell subsets in and around tumor tissues.
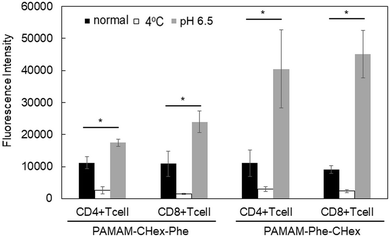 |
| Fig. 6 Association of PAMAM-CHex-Phe and PAMAM-Phe-CHex with CD3+CD4+ helper T cells and CD3+CD8+ killer T cells under different conditions. *Higher and p < 0.05 vs. each normal condition. | |
3.5 Association of PAMAM-Phe-CHex and PAMAM-CHex-Phe with activated T cells
Our results indicated that the associations of both PAMAM-CHex-Phe and PAMAM-Phe-CHex with T cells and their subsets increased at pH 6.5, which is the pH of tumor microenvironments. The function of T cells is known to change under acidic conditions.9 CD69 is a marker of activated T cells. Con. A is often used as an activator of T cells to upregulate CD69 expression.16 After the incubation of the splenocytes at pH 6.5 and/or in the presence of Con. A, they were immunostained with CD3-PE and CD69-APC prior to the FACS analysis. Fig. S9 (ESI†) shows that the Con. A-untreated T cells did not express CD69 even at pH 6.5. And, CD69 was expressed in T cells after the treatment of Con. A. regardless of the adjustment to pH 6.5. This indicates that T cells are not activated at pH 6.5.
The association of PAMAM-Phe-CHex and PAMAM-CHex-Phe with the nonactivated T cells (CD3+CD69-cells) and the activated T cells (CD3+CD69+ cells) was compared. Fig. 7 shows that the mean fluorescence intensities of the dendrimers associated with the activated T cells were higher than those of the dendrimers with nonactivated T cells, suggesting that these dendrimers can be more efficiently associated with activated T cells than with nonactivated T cells. The fluorescence intensity of PAMAM-Phe-CHex in both the nonactivated and activated T cells increased at pH 6.5, suggesting that this is most effectively associated with activated T cells at pH 6.5. Our results suggest that the association of these dendrimers can be controlled using pH and T cell activation.
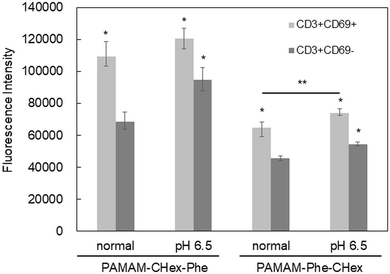 |
| Fig. 7 Association of PAMAM-CHex-Phe and PAMAM-Phe-CHex with CD3+CD69+ and CD3+CD69- cells under different conditions after the Con. A treatment. *p < 0.05 vs. PAMAM-CHex-Phe or PAMAM-Phe-CHex under the normal condition in CD3+CD69-cells. **p < 0.05 between the normal and acidic conditions in CD3 + CD69+ cells. | |
4. Discussion
In this study, we synthesized six carboxy-terminal dendrimers with hydrophobic amino acids (Phe and Leu) and acid anhydrides (Suc and CHex) in different reaction orders to compare their pH-responsive properties and their association with T cells and their subsets. The dendrimers bearing CHex and Phe, PAMAM-Phe-CHex and PAMAM-CHex-Phe, showed a higher association with splenocyte-derived T cells than with the other ones. These dendrimers had the highest log
P values in this study (Table 1), indicating that they are the most hydrophobic ones. This suggests that the hydrophobic effect significantly influences the association of dendrimers with immune cells. We attempted to elucidate the internalization mechanism of these dendrimers with T cells. As shown in Fig. S8 (ESI†), the association of dendrimer with Jurkat cells was slightly enhanced by the addition of Phe. Because the self-assembled structure of Phe enhanced the cell membrane permeability,7 it is possible that Phe aggregates at the dendrimer surface directly interacted with the cell membranes. The adhesion of the dendrimers to the liposomes as a cell membrane model was investigated (Table S1, ESI†). The adsorption (%) of PAMAM-Phe-CHex and PAMAM-CHex-Phe to liposomes was calculated as 55% and 2%, respectively. This suggested that PAMAM-Phe-CHex possibly interacted with the cell membranes at the surfaces of the T cells and their subsets. Fig. S4 (ESI†) shows that the solubility of PAMAM-Phe-CHex was lower than that of PAMAM-CHex-Phe even at neutral pH, which supports the results of the interaction of PAMAM-Phe-CHex with the liposome. Our confocal microscopic analysis indicates that these dendrimers were internalized into the T cells via endocytosis (Fig. 5). Interestingly, these dendrimers were rarely co-localized with lysotracker after the internalization (Fig. S7, ESI†). Further investigation is necessary.
The targeted delivery is important for DDS. Fig. S5 and S6 (ESI†) indicate that our dendrimers were not associated with T cells selectively. Since T cells are classified into various subsets with different functions, the targeted delivery into specific T cell subsets is also important. Both PAMAM-Phe-CHex and PAMAM-CHex-Phe were associated with CD4+ helper T cells as well as CD8+ killer T cells. These dendrimers showed pH-responsive properties, as shown in Fig. S4 (ESI†). Especially, the solubility of PAMAM-Phe-CHex was drastically changed from a physiological pH of 7.4 to pH 6.5 because of the coacervation. Although the coacervation did not occur at the low dendrimer concentration, the dendrimer surface may become hydrophobic. Thus, the association of PAMAM-Phe-CHex with T cells and their subsets improved at pH 6.5 (Fig. 4, 6 and 7). Acidic pH is one of the factors of tumor microenvironments. pH-sensitive properties are useful for selective delivery into cells in and around tumor tissues. Although the acidic pH did not activate T cells, as shown in Fig. S9 (ESI†), the activated T cells were more associated with these dendrimers than with the nonactivated T cells (Fig. 7). The combination of our system with T cell activators might also be useful for the selective delivery to targeted T cells.
We think that our dendrimers are useful for cancer immunotherapy, based on the pH-responsive properties. Activation of lymph node-resident helper T cells and killer T cells as well as suppression of regulatory T cells localized in tumor tissues are necessary in cancer immunotherapy. Our previous reports indicate that anionic terminal dendrimers, including PAMAM-CHex-Phe, are highly accumulated in the lymph node and lymph node-resident T cells.13,14 It is known that small nanoparticles of less than 70 kDa are necessary for the distribution in the lymph node.18 The molecular weight of our dendrimers is less than 40 kDa, which is useful for the delivery into lymph node-resident T cells. This study shows that our dendrimers have the potential for direct delivery to various T cells especially at low pH, that is, around tumor cells. Because dendrimers can load various compounds such as peptides, drugs, and imaging agents, it is expected that these dendrimers are a possible carrier for pH-sensitive delivery to metastatic tumor cell-localized lymph node-resident T cells.
5. Conclusion
In this study, we constructed pH-sensitive delivery systems to T cells and their subsets by using carboxy-terminal dendrimers bearing Phe and hydrophobic acid anhydride, such as PAMAM-CHex-Phe and PAMAM-Phe-CHex. The dendrimers with Suc and Leu were not efficiently associated with T cells, indicating the importance of the combination of CHex and Phe for the association with T cells. These dendrimers were more efficiently associated with CD3+ T cells at pH 6.5, which is observed in tumor microenvironments. This suggests that these dendrimers, especially PAMAM-Phe-CHex, are useful for delivery to T cells around and in tumors. Also, these dendrimers were associated with CD4+ helper T cells and CD8+ killer T cells at a similar level, suggesting that both cell types are possible dendrimer targets. These dendrimers were associated with the activated CD69+ T cells more efficiently. This is the first report on the direct delivery into T cells using pH-sensitive DDS, as far as we know. Our findings are important for the development of nanoplatforms for direct delivery to T cells to control the functions of T cells, which play key roles in cancer immunotherapy.
Funding
This work was supported by JSPS KAKENHI Grant Numbers JP20H05232 (Grant-in-Aid for Scientific Research on Innovative Area: Aquatic Functional Materials) from the Ministry of Education, Culture, Sports, Science and Technology of Japan (MEXT).
Conflicts of interest
H. S. is an employee of Kao Co., Ltd.
Acknowledgements
We are grateful to Prof. Takashi Inui (Osaka Prefecture University) and Dr. Tadahiro Inoue (Osaka Prefecture University) for their assistance in the animal experiments and for the useful discussions, respectively.
References
- S. Mura, J. Nicolas and P. Couvreur, Nat. Mater., 2013, 12, 991–1003 CrossRef CAS PubMed; H. Wang, Q. Huang, H. Chang, J. Xiao and Y. Cheng, Biomater. Sci., 2016, 4, 375–390 RSC; D. Schmaljohann, Adv. Drug Delivery Rev., 2006, 58, 1655–1670 CrossRef PubMed.
- C. Corbet and O. Feron, Nat. Rev. Cancer, 2017, 17, 577–593 CrossRef CAS PubMed; M. Kanamala, W. R. Wilson, M. Yang, B. D. Palmer and Z. Wu, Biomaterials, 2016, 85, 152–167 CrossRef PubMed; T. Haider, K. K. Sandha, V. Soni and P. N. Gupta, Mater. Sci. Eng., C, 2020, 116, 111229 CrossRef PubMed; W. Gao, J. M. Chan and O. C. Farokhzad, Mol. Pharm., 2010, 7, 1913–1920 CrossRef PubMed.
- D. A. Tomalia, A. M. Naylor and W. A. Goddard, Angew. Chem., Int. Ed. Engl., 1990, 29, 138–175 CrossRef CAS; S. Svenson and D. A. Tomalia, Adv. Drug Delivery Rev., 2005, 57, 2106–2129 CrossRef PubMed; C. Kojima, Expert Opin. Drug Delivery, 2010, 7, 307–319 CrossRef PubMed.
- Y. Tono, C. Kojima, Y. Haba, T. Takahashi, A. Harada, S. Yagi and K. Kono, Langmuir, 2006, 22, 4920–4922 CrossRef CAS PubMed.
- M. Tamaki, D. Fukushima and C. Kojima, RSC Adv., 2018, 8, 28147–28151 RSC.
- M. Tamaki and C. Kojima, RSC Adv., 2020, 10, 10452–10460 RSC.
- L. Adler-Abramovich, L. Vaks, O. Carny, D. Trudler, A. Magno, A. Caflisch, D. Frenkel and E. Gazit, Nat. Chem. Biol., 2012, 8, 701–706 CrossRef CAS PubMed; T. D. Do, W. M. Kincannon and M. T. Bowers, J. Am. Chem. Soc., 2015, 137, 10080–10083 CrossRef PubMed; E. C. Griffith, R. J. Perkins, D. M. Telesford, E. M. Adams, L. Cwiklik, H. C. Allen, M. Roeselová and V. Vaida, J. Phys. Chem. B, 2015, 119, 9038–9048 CrossRef PubMed; R. Perkins and V. Vaida, J. Am. Chem. Soc., 2017, 139, 14388–14391 CrossRef PubMed; B. G. Anand, K. Dubey, D. S. Shekhawat and K. Kar, Sci. Rep., 2017, 7, 1–9 CrossRef PubMed.
- I. Mellman, G. Coukos and G. Dranoff, Nature, 2011, 480, 480–489 CrossRef CAS PubMed; S. Spranger, H. K. Koblish, B. Horton, P. A. Scherie, R. Newton and T. F. Gajewski, J. Immunother. Cancer, 2014, 2, 3 CrossRef PubMed.
- V. Hubera, C. Camisaschi, A. Berzi, S. Ferro, L. Lugini, T. Triulzi, A. Tuccitto, E. Tagliabue, C. Castelli and L. Rivoltini, Semin. Cancer Biol., 2017, 43, 74–89 CrossRef CAS PubMed; K. Fischer, P. Hoffmann, S. Voelkl, N. Meidenbauer, J. Ammer and M. Edinger,
et al.
, Blood, 2007, 109, 3812–3819 CrossRef PubMed.
- J. Banchereau and R. M. Steinman, Nature, 1998, 392, 245–252 CrossRef CAS PubMed; O. P. Joffre, E. Segura, A. Savina and S. Amigorena, Nat. Rev. Immunol., 2012, 12, 557–569 CrossRef PubMed; M. D. Joshi, W. J. Unger, G. Storm, Y. Kooyk and E. Mastrobattista, J. Controlled Release, 2012, 161, 25–37 CrossRef PubMed.
- D. Schmid, C. G. Park, C. A. Hartl, N. Subedi, A. N. Cartwright, R. B. Puerto, Y. Zheng, J. Maiarana, G. J. Freeman, K. W. Wucherpfennig, D. J. Irvine and M. S. Goldberg, Nat. Commun., 2017, 8, 1747 CrossRef PubMed; S. Cao, S. D. Slack, C. N. Levy, S. M. Hughes, Y. Jiang, C. Yogodzinski, P. Roychoudhury, K. R. Jerome, J. T. Schiffer, F. Hladik and K. A. Woodrow, Sci. Adv., 2019, 5, 1–14 Search PubMed.
- L. Tang, Y. Zheng, M. B. Melo, L. Mabardi, A. P. Castaño, Y.-Q. Xie, N. Li, S. B. Kudchodkar, H. C. Wong, E. K. Jeng, M. V. Maus and D. J. Irvine, Nat. Biotechnol., 2018, 36, 707 CrossRef CAS PubMed.
- Y. Niki, M. Ogawa, R. Makiura, Y. Magata and C. Kojima, Nanomed. NBM, 2015, 11, 2119–2127 CrossRef CAS PubMed; Y. Nishimoto, S. Nagashima, K. Nakajima, T. Ohira, T. Sato, T. Izawa, J. Yamate, K. Higashikawa, Y. Kuge, M. Ogawa and C. Kojima, Int. J. Pharm., 2020, 576, 119021 CrossRef PubMed.
- Y. Nishimoto, M. Nishio, S. Nagashima, K. Nakajima, T. Ohira, S. Nakai, I. Nakase, K. Higashikawa, Y. Kuge, A. Matsumoto, M. Ogawa and C. Kojima, Polymers, 2020, 12, 1474 CrossRef CAS PubMed.
- K. Kono, H. Akiyama, T. Takahashi, T. Takagshi and A. Harada, Bioconjugate Chem., 2005, 16, 208–214 CrossRef CAS PubMed.
- O. Borges, G. Borchard, A. Sousa, H. E. Junginger and A. C. Silva, Int. J. Pharm., 2007, 337, 254–264 CrossRef CAS PubMed.
- O. P. Perumal, R. Inapagolla, S. Kannan and R. M. Kannan, Biomaterials, 2008, 29, 3469–3476 CrossRef CAS PubMed.
- M. A. Swartz and A. W. Lund, Nat. Rev. Cancer, 2012, 12, 210–219 CrossRef CAS PubMed.
Footnote |
† Electronic supplementary information (ESI) available. See DOI: 10.1039/d1tb01980e |
|
This journal is © The Royal Society of Chemistry 2022 |
Click here to see how this site uses Cookies. View our privacy policy here.