DOI:
10.1039/C8JA00319J
(Critical Review)
J. Anal. At. Spectrom., 2019,
34, 81-103
Applications of laser-induced breakdown spectroscopy in cultural heritage and archaeology: a critical review
Received
28th September 2018
, Accepted 1st November 2018
First published on 1st November 2018
Abstract
In this paper, we present a critical review on the applications of the Laser-Induced Breakdown Spectroscopy (LIBS) technique in cultural heritage and archaeology. The strategies used by the groups involved in this kind of research for the analysis of the typical materials of interest (metals, pigments, pottery, glass, etc.) are discussed in detail, as well as the use of LIBS in combination with other techniques (LIBS and Raman, LIBS and XRF, and LIBS and MS). Specific applications of LIBS as a support for cultural heritage restoration and the application of the technique for the analysis of underwater objects are presented in separate sessions. In conclusion, new trends of LIBS for cultural heritage and archaeology (micro-LIBS analysis, 3D elemental imaging, and surface- and nanoparticle-enhanced LIBS) are introduced and discussed.
1 Introduction
Laser-Induced Breakdown Spectroscopy (LIBS) is an analytical technique based on the use of a pulsed power laser which, focused on the sample surface, produces the ablation of a small amount of material and its excitation.1 The ablated material, in the state of plasma, emits a characteristic spectrum, which gives qualitative and quantitative information about the sample composition. Since its introduction, in the late 80's of the last century,2 LIBS has been successfully applied to the analysis of materials of interest in many different fields, ranging from industrial diagnostics,3–5 environmental protection,6,7 bio-medicine,8,9 forensic analysis,10,11etc. The characteristics of speed, portability, capability of in situ remote analysis without sample treatment and ease of use of the equipment have made LIBS one of the fastest growing analytical techniques in the last century, with the number of LIBS-related papers published per year nearly doubling from 2010 (225 papers) to 2017 (433).† These very same characteristics have made the LIBS technique interesting for Cultural Heritage (CH) and archaeology applications. However, contrarily to other kinds of applications, the intrinsic micro-destructive nature of the LIBS technique has somewhat limited its diffusion in these fields; to date, only about 2% of the LIBS papers (slightly more than 100 papers of a total of more than 4000) deals with LIBS applications in cultural heritage. Since cultural heritage and archaeological objects are, normally, valuable and delicate, in some cases unique, the use of a destructive (although minimally) technique must be carefully considered. Alternative techniques exist, such as X-ray fluorescence, laser-induced fluorescence, multispectral imaging, Raman spectroscopy, etc.,12 which are portable and non-destructive and may also provide structural information on the nature of the materials under study. From case to case, the importance of the potential results that can be obtained by LIBS, and not with other techniques, has to be considered by art conservators and archaeologists against the possible damage to the object of the study. It is evident that, when a non-destructive option exists, it should be preferred to a destructive approach; however, in our opinion, the main obstacle to the application of LIBS in CH and archaeology is more related to a difficulty in the communication between LIBS researchers and art historians, conservators and archaeologists, than to real limitations of the technique itself. It has already been discussed13 how the undoubtable advantages of LIBS have been largely over-boosted in the past against its equally undoubtable drawbacks, creating great expectations, especially in multi-cultural disciplines such as CH and archaeology, that in most of the cases were not actually met. On the other hand, excellent results have been demonstrated when a fruitful dialog between scientists and humanists has been established and the LIBS technique has been applied in response to a real demand of the operators in the field.
This review presents the most outstanding case-studies, which have demonstrated, in the last few decades, the effectiveness of the application of LIBS in cultural heritage and archaeology. In some cases, LIBS has been used in conjunction with other analytical techniques, and these applications will be discussed separately. We have organized our work according to the main materials analyzed by LIBS (metals, stones, ceramics, pigments, glass, bones, etc.). In a few cases, multi-material objects or collections have been studied. In that case, the studies will be quoted in all the relevant chapters. Specific applications, such as LIBS in restoration and in underwater archaeology, will be presented separately, because of their peculiarities (Table 1).
Table 1 Table of applications of LIBS in CH
Section |
Material |
Application |
References |
2.1 |
Bronze |
Authentication |
44
|
Classification |
32 and 34
|
Composition |
33, 34, 43, 45, 48–50 and 55–57
|
Gold |
Composition |
47
|
Gold/copper |
Stratigraphy |
36
|
Copper |
Stratigraphy |
37 and 38
|
Classification |
31
|
Composition |
31
|
Iron |
Classification |
32
|
2.2 |
Paintings |
Composition |
58, 59 and 61–64
|
Fresco |
Mapping |
60
|
Wall paintings |
Composition |
70 and 88
|
Pottery |
Composition |
71
|
Enamel |
Composition |
72
|
Polymers |
Composition |
73
|
Binders |
Composition |
74
|
Pigments |
Mapping |
90
|
2.3 |
Pottery |
Depth profiling |
92 and 93
|
Composition |
94, 95, 98 and 100
|
Provenience |
103
|
Firing temperature |
104
|
Authentication |
105
|
Mapping |
106–108
|
Marble |
Laser cleaning |
109–112 and 114–116
|
Provenience |
117–120
|
Limestone |
Provenience |
121
|
2.4 |
Teeth |
Nutrition |
134 and 138
|
Composition |
135
|
Bones |
Conservation |
136
|
Nutrition |
137–139
|
Foxing |
Distribution |
140
|
Wood |
Composition |
141
|
2.5 |
Glass |
Composition |
142 and 144
|
Classification |
143 and 144
|
Conservation |
139 and 140
|
Precious stones |
Composition |
147 and 149
|
Classification |
148 and 150
|
3.1 |
Pigments |
Composition |
158
|
Pigments |
Mapping |
155
|
3.2 |
Bronze |
Classification |
165
|
Composition |
41, 42, 55, 166 and 168
|
Corrosion |
169
|
Silver |
Composition |
167
|
Pigments |
Composition |
82
|
Rocks |
Composition |
170
|
4.1 |
Construction materials |
Diagnostic |
173
|
Cleaning |
174 and 178
|
Metal |
Cleaning |
182–185 and 187
|
Paintings |
Cleaning |
175–177
|
Glass |
Cleaning |
178
|
Marble |
Cleaning |
111, 115 and 179–181
|
4.2 |
Metal/marble/wood |
Classification |
123
|
Metal |
Composition |
195
|
Metal/pottery/marble |
Classification |
124 and 197
|
5.1 |
Fresco |
2D mapping |
60
|
Mortar |
2D mapping |
128 and 199
|
Pottery |
2D mapping |
106
|
Building materials |
3D mapping |
129
|
Rocks |
3D mapping |
201
|
Pigments |
Classification |
90
|
5.2 |
Dyes |
Classification |
211
|
Several reviews have been published in the past dealing with LIBS applications in CH and archaeology;14–21 our review will thus mostly focus on the most recent applications, not covered by previous studies.
2 Case studies
2.1 LIBS analysis of metals
The LIBS technique is particularly suited for the analysis of metallic objects22 and, therefore, the applications of the technique in CH and archaeology for this kind of study are also numerous. It should be considered, however, that most of the advantages of LIBS are shared, in these applications, with the Energy Dispersive X-Ray Fluorescence (ED-XRF) technique.23,24 ED-XRF is a fast and portable technique, which is capable of precise quantitative analysis without pre-treatment of the samples, in a fully non-destructive way. In most archaeometric applications, thus, ED-XRF should be preferred to LIBS. The intrinsic quickness and non-destructive character of ED-XRF allow for extensive sampling of objects in collections (for authentication and provenience studies,25–28 for example) and/or different points of measurements on single objects, improving the statistical significance of the measurements. There are, however, specific situations in which the LIBS technique might give more information with respect to ED-XRF. Since ED-XRF is, intrinsically, a bulk technique, it is very difficult, if not impossible, to discriminate the contributions to the XRF spectra coming from different depths under the surface. Moreover, due to the differences in X-ray absorption by the elements, the XRF spectra are often a complex combination of fluorescence emitted by the elements at depths of several tens of microns under the surface. This is not a problem for the analysis of homogeneous materials; however, metallic artifacts, especially the archaeological ones, may present surface corrosion layers, which would make the ED-XRF quantitative analysis unreliable. On the other hand, the LIBS analysis is limited to the depth of the crater produced on the sample surface (typically less than one micron in metals); this feature, combined with the micro-destructivity of the technique, allows the use of the laser as a ‘drill’ for sampling the object at different depths. Although the interpretation of the stratigraphic compositional changes is not completely straightforward, because of the possibility of contamination from the border of the crater and the reduction of the signal due to the defocusing of the laser beam, in the analysis of metals for cultural heritage and archaeology the possibility of making a stratigraphic analysis in most of the cases is the only real analytical advantage of the use of LIBS vs. ED-XRF. From a practical point of view, the use of safer optical (LIBS) instead of X-Ray excitation (XRF) should also be mentioned, which should not be neglected, and the possibility of LIBS of making remote analysis using optical fibers or articulated arms (one meter or less)29 or up to several meters in open path configuration.30 On the other hand, ED-XRF analysis must be performed at a close distance; moreover, most of the portable ED-XRF systems have measurement spots of the order of 1 mm2, while the laser beam in LIBS analysis can be focused up to a few microns in diameter, allowing for a much higher spatial resolution. Even in the presence of thick corroded surface layers (in archaeological samples, the thickness of corroded layer can exceed hundreds of microns), the lateral dimensions of the LIBS crater may be kept lower than the typical dimensions of the XRF spot (around 1 mm in diameter).
We will discuss in the following section the combined use of LIBS and ED-XRF, which has been proved, in many cases, to be very effective for maximizing the information and reducing at the minimum the impact on the objects under study.
Among the applications of LIBS for the analysis of metallic objects in CH and archaeology, the following studies are worth mentioning: Corsi et al.,31 who in 2005 made the analysis and classification of copper age weapons (see Fig. 1).
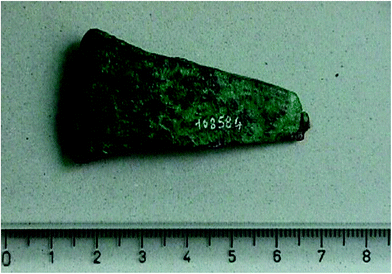 |
| Fig. 1 One of the copper-age weapons studied in ref. 31. | |
Fortes et al.,32 who made, in the same year, a chronocultural classification of bronze and iron-age objects; Burgio et al.,33 for the quantitative analysis of fragments for a bronze statue of Le Seur in 2006; Foresta et al.,34 for the quantitative study and classification of 12 bronze statues at the Museum of Crotona in 2012 (Fig. 2) using Modì,29 a mobile dual pulse LIBS instrument realized by the Pisa group in collaboration with Marwan Scientific Instruments (Pisa).
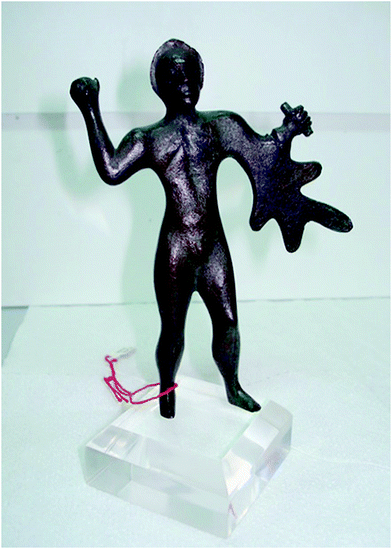 |
| Fig. 2 One of the bronze statues studied in Crotona by LIBS (ref. 28). | |
In all these cases, the samples presented an extended surface corrosion, which made necessary the use of LIBS for the quantitative determination of the alloy composition.35 Similarly, in 2010 Abdelhamid et al. applied LIBS for the stratigraphic analysis of a gold-coated archaeological decorative copper object.36
Agresti et al.37 also realized a mobile LIBS instrument for the analysis of CH and archaeological samples and in 2016, following a previous study by Siano et al.,38 studied the optimization of LIBS depth-profiling for bronze analysis using 3D digital microscopy.39 The same authors also applied LIBS in conjunction with other techniques, for the analysis of archaeological samples (THz imaging and LIBS in ref. 40, neutron tomography and diffraction, XRF, X-Ray diffraction and LIBS in ref. 41 and 42). Further applications involved the analysis of a Donatello bronze statue43 and the LIBS authentication study, combined with micro-structural analysis, of Roman copper–alloy coins.44
Gaudiuso et al. in 2008 worked on copper-based alloy samples from the archaeological site of Minervino Murge (around VII B.C.) using, besides traditional ns LIBS, also fs LIBS, finding that in both cases the LIBS spectra obtained could be used for the quantitative determination of the alloy composition using suitable calibration curves.45
The complexity of the analysis of CH and archaeological metallic alloys often triggered the need for sophisticated methods for quantitative analysis and classification. In 2001, Corsi et al.46 published a paper on standard-less quantitative analysis of gold objects, using the calibration-free LIBS method.47 Fornarini et al. developed in 2009 a theoretical model for the quantitative analysis of quaternary bronze using ns and fs LIBS.48,49 In 2012, Gaudiuso et al.50 published a paper on the application of LIBS for the analysis of copper-based alloy objects from archeological sites in Southern Italy (from VII century B.C. to VII A.D.), introducing a standard-less quantitative approach named the inverse calibration-free method.51 In 2014, D'Andrea et al.52–54 introduced an artificial neural network approach for the quantitative analysis of bronze samples. The same technique was used by Lorenzetti et al.55 for the analysis of the alloy of the Pisa Griffin, a Medieval Islamic statue conserved at Museo dell'Opera del Duomo, in Pisa, Italy (see Fig. 3).
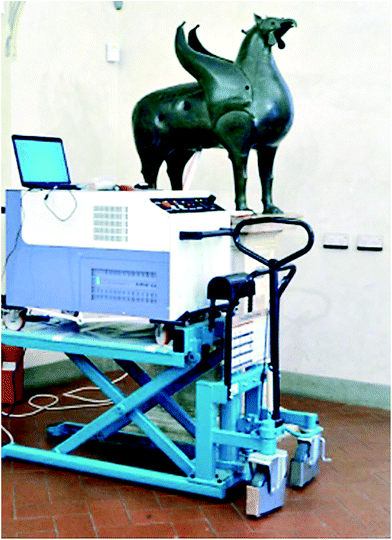 |
| Fig. 3 LIBS measurements on the Pisa Griffin (ref. 55). | |
Pagnotta et al. in 2015 used the self-organizing maps method for the analysis and comparison of two archaeological bronze samples.56 In the same year, Agresti et al.57 developed a depth dependent calibration method for the quantitative analysis of bronze in stratigraphic LIBS studies.
2.2 LIBS analysis of pigments
The first attempt to determine the elemental nature of paintings using a spark of a few milligram mass fraction is dated back to 1924: in the Comptes Rendus des Séances de l'Académie des Science Edmond Bayle and Henry George briefly presented for the first time the idea of using an elemental spectroscopic technique to analyse pigments. This approach should have been considered very challenging, because until 1971 it was hard to find other examples. In fact, thanks to the invention of laser microprobes in the early 1960s and the subsequent technological developments, a significant advancement in the spectrochemical analysis of microscopic specimens was achieved. In 1971, Petrakiev, Samov and Dimitrov identified pigments in old multilayer wall paintings applying the LMAl Zeiss laser microspectral analyser;58 8 years later, Roy published in the National Gallery Technical Bulletin the promising results of a versatile and sensitive analytical tool for the qualitative detection of the characteristic and trace elements found in pigment materials.59 It may be considered as the first case of using LIBS for painting diagnostic: a synthetic ruby resonator rod created laser light pulses with a power density of up to 100 MW cm−2 producing a 95 µm diameter laser crater. Multiple spectra were stacked on the photographic plate, covering the spectral range of 200–850 nm (see Fig. 4).
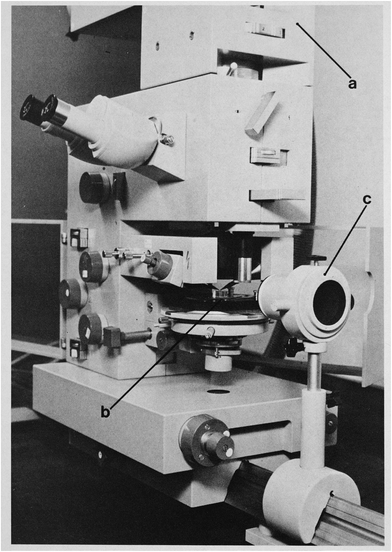 |
| Fig. 4 LMAl Zeiss laser microspectral analyser: (a) optical adaptor, (b) sample stage and spark-gap, (c) imaging quartz lens (ref. 60, courtesy of the National Gallery, London, UK). | |
However, the first paper where the LIBS technique is explicitly mentioned for this purpose is the work by Anglos et al. in 1997.61 The authors collected LIBS spectra from a wide variety of pigments in powder form and in oil color test samples. Appropriate emission lines for the identification of the metallic elements in the pigments were examined and proposed. Optimal experimental parameters were discussed, demonstrating the analytical capabilities of the technique in the field of pigment analysis of painted artworks. Corsi et al.60 in year 2000 performed the first LIBS 2D elemental mapping on a II century A.D. Roman fresco from St. Albans, UK (see Fig. 5).
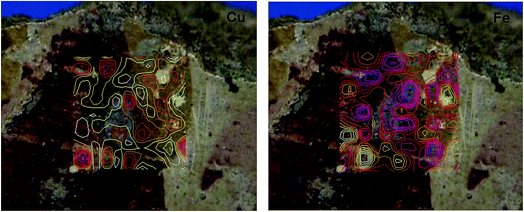 |
| Fig. 5 LIBS elemental mapping (Cu and Fe) on a Roman fresco (ref. 60). Red indicates high concentration of the element and blue low concentration. | |
In the same year, Borgia et al.62 demonstrated the possibility of performing quantitative standardless determination of the composition of pigments using calibration-free LIBS. Later, several examples have been reported in the literature, from the analysis of azurite (Cu3(CO3)2(OH)2) and lapis lazuli, a mixture of minerals with lazurite ((Na,Ca)8[(S,Cl,SO4,OH)2[(Al6Si6O24)]) as the main constituent,63 to byzantine icons,64 Bronze Age painted plaster,65 Roman frescos,66,67 oil paintings,68 grisaille,69 prehistoric rock wall paintings,70 Neolithic painted pottery71 (Fig. 6) and painted enamel.72
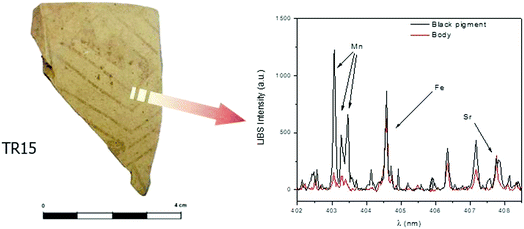 |
| Fig. 6 LIBS analysis on Neolithic pottery, showing the use of Mn oxide as a black pigment (ref. 71). | |
Generally, these studies demonstrate that LIBS can be successfully used to identify not only inorganic, but also organic materials such as protective polymers or binders.73 Comparing the emission of CN and C2 bands in the plasma, in fact, Bai et al.74 discriminated casein, animal glue, oil and egg yolk in mock-up samples painted with a layer of the pigment cinnabar (HgS). To advance the separation, a chemometric method based on the Principal Component Analysis (PCA) was used; 266 nm radiation allowed for a better performance.
The main drawback of the technique, its micro-destructivity, can be turned into an advantage: each laser pulse can expose a new layer of artworks, providing a sort of cross-sectional analysis.75 To better associate the LIBS information with the single layer it is necessary to know the exact depth of the crater after each pulse. For metal alloys76 it has been already demonstrated that it is possible to estimate the depth of the crater after each pulse with Optical Coherence Tomography (OCT), but only assuming a constant ablation rate throughout the whole experiment. This seems not the case for paint layers, but Kaszewska et al.77 suggested anyway the use of a spectral-domain OCT instrument after each laser pulse as a fast profilometer. In this way, they were able to obtain the precise in-depth scaling of elemental concentration profiles, and the recognition of layer boundaries by estimating the corresponding differences in the material ablation rate; the expected statistical errors for the crater-depth determination were about ±4 µm.
Nevertheless, the mass ablation is not the only LIBS weakness; an interesting study by Bruder et al.78 investigated the discoloration phenomenon of lead pigments analysed by LIBS. They found that the pigments faded after a few days of exposure to ambient atmosphere. It was established that the mechanism is due to the formation of lead oxide (PbO) through recombination between atomized lead and oxygen in the plume.79 A threshold for discoloration occurrence was estimated; in particular, if the total amount of energy delivered (fluence-per-shot × number of shots) is less than 35–40 J cm−2, no discoloration is observed. LIBS conditions could then be determined in order not to induce visible discoloration and to ensure a correct preservation of the artwork. In general, for the study of delicate pigments the use of a laser with a shorter pulse duration (picosecond or femtosecond lasers) could reduce the photo-thermal effect.80 Unfortunately, cinnabar even with a very low laser energy value tends to darken, irreparably.81
The elemental content of paint materials was determined in most cases leading to the identification of the pigment used in agreement with data from analyses of the same samples with other established techniques, both elemental and in terms of different detectable elemental contents, such XRF82 and XRD,83 or molecular for complementary information, mainly Raman, by trying to identify the compounds before and after the laser shot,84 but also FT-IR,85 LIF18 and HPLC-UV/VIS.86
As for other LIBS applications, the use of chemometric techniques allows for a semi-automatic pigment recognition. Duchêne et al.87 showed the promising use of Soft Independent Modelling of Class Analogy (SIMCA) and Partial Least-Squares Discriminant Analysis (PLS-DA) for improving the recognition of unknown spectra acquired in the laboratory. The first step lied in the direct spectral comparison of the major lines of the reference and those of the unknown pigment, and then each pigment is associated with one element of its composition. Unfortunately, the method didn't allow for on-site identification, due to the difference of signal intensities between the data and the experimental spectra. Thanks to more robust instrumentation and using a different approach Syvilay et al.88 reached better results. The Standard Normal Variate (SNV) method was applied to overcome undesired LIBS signal variations for in situ depth analyses of painting layers from mural paintings. The results were very promising but, as highlighted by the same authors, it is worth noting that even if the method allows for a better visualization of wavelength intensities and so a better recognition of the peaks, the SNV transformation depends on the mean and the standard deviation of all the spectra, so the efficiency will change according to the specific material. Principal Component Analysis (PCA) was also employed to verify the presence of clusters related to the depth profile analysis of prehistoric paintings,89 determining the main elements characterizing the distinct layers. In several samples, in fact, a layer of impurities due to geological processes was deposited on the surface, and the PCA score plot enabled us to identify unequivocally the spectra belonging only to the pigment layer.
Finally, an interesting approach by Syta et al. is worth mentioning,90 which combined LIBS and Laser-Ablation ICP Mass Spectrometry (LA-ICP-MS) for elemental mapping of inorganic archaeological samples: using a 266 nm Nd:YAG laser, cross sections of mediaeval Nubian objects with specific blue painting layers, including either Egyptian blue or lapis lazuli, were analysed. The distribution of selected elements was mapped with a scan rate of 10 µm s−1.
2.3 LIBS analysis of geological materials
Since the introduction of the LIBS method, the scientific community linked to the study of geological materials and, among them, those used in cultural heritage, has seen in LIBS a valuable tool, in particular for field use. Pottery, among the geological materials of interest for CH, plays a major role in the LIBS application in this field. Pottery is an artificial transformation, through cooking, produced starting from a mixture of geological materials (clays and other materials used to improve the technical characteristics). The principal application on these materials is the study of glazes: the capability to obtain a depth profile that shows qualitatively all the elements in the sample, also the low-atomic number ones, is well suited to these studies. One of the first studies used glass beads for building a calibration curve for the analysis of archaeological glazes on pottery.91 In the analysis of complex and multi-stratified artificial materials such as glazed ceramics, there is a need to understand the contribution made by each layer of the material analyzed to the plasma emission spectrum. The contribution made by the Colao et al.92 and Lazic et al.93 studies is very interesting in that respect. The part of the first paper regarding the glaze pottery from Urbino and the second paper deal with two main problems in this kind of analysis: the inhomogeneity of the material and the contribution of each thin layer to the plasma emission spectrum. The first problem is overcome averaging different spectral lines for each analyzed element; the second problem, as bisque and glaze both contain the same elements, is surpassed by separating the single contribution of bisque and glaze in the emission spectra. Other studies deal with the arguments in a qualitative way to identify the pigments used for pottery decoration,94,95 sometimes comparing the results with those from other techniques such as SEM/EDX for quantitative purposes.96 The studies of Erdem et al.97 shift the focus to the determination of the compositional variation of clay. To overcome the problems presented by the natural lack of homogeneity of the ceramic body, the authors analyzed potsherds and pellets obtained from ground potsherds. A further step forward is the use of a chemometric method (Principal Component Analysis – PCA) for determining similarities and differences between the analyzed fragments. Genc Oztoprak et al.98 proposed the use of PCA and Partial Least Squares-Discriminant Analysis (PLS-DA) to classify some ceramic fragments, also focusing on the possibility of performing the LIBS analysis in situ. Xenogiannopoulou et al.99 carried out a complete study of Attic black pottery by LIBS analysis, comparing the results with those from consolidated techniques such as AAS, SEM/EDX, XRF and XRD. Lasheras et al.100 used LIBS with calibration curves on different standards prepared with KBr and zinc as internal standards for the analysis of Roman pottery and compared their results with those from Atomic Absorption Spectrometry (AAS). Khedr et al.101,102 introduced a combination of LIBS and ED-XRF that provided good results, underlining the possibility of carrying out in situ measurements on glazes.
An aspect not dealt within these studies was the possibility to carry out analyses of Rare Earth Elements (REEs) that in geological materials might be useful to identify their origin. A single attempt in this direction was made by Rai et al.,103 who qualitatively studied pottery coming from the Indian site of Kausambi, identifying precise sets of REEs that have allowed us to confirm the common provenance of the raw materials. Remaining in the Indian geographical area, particularly in the north-east of the country, Singh et al.104 used LIBS and SEM/EDX to determine the chemical composition and the probable firing temperature of eight ceramic fragments (five Neolithic and three belonging to the historical period). They used LIBS for the qualitative characterization of the ceramic body, while the quantitative analysis was entrusted to a SEM micro-probe. In 2013, LIBS was also used by Legnaioli et al.105 for a multi-technique authentication analysis of an archaeological ceramic vase.
A new approach that in the future could potentially fit into the normal analytical routine is that introduced by Pagnotta et al.106 in 2018. Starting from slices of potsherds (negatives of thin sections), the authors mapped a portion of surface samples using a scanning µ-LIBS instrument and analyzed it qualitatively, through the use of image-based techniques. In another paper, Pagnotta et al.107 extended the previous method for segmenting the elemental maps in qualitatively similar areas, through SOM algorithms (see Fig. 7).
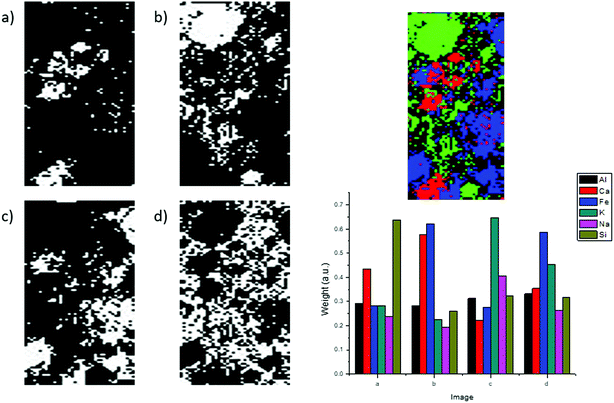 |
| Fig. 7 Reconstruction of the mineralogical composition of a Roman mortar sample from SOM images (ref. 107). | |
The same authors thus performed standard-less analysis by CF-LIBS, in a fast and semi-automated way, on the prototype spectra provided by the SOM analysis, thus solving the problem of analyzing thousands of spectra to overcome the lack of homogeneity of the material. The work was carried out on mortar coming from the Castle of Adrano (Sicily).108
LIBS was also used as a diagnostic tool for the process of laser cleaning of marble, for the first time, by Maravelaki et al. in 1997 and 1999;109,110 in 2001, the same authors also characterized the encrustation layer of marble by LIBS.111 Other studies that used LIBS for monitoring the cleaning processes of marble and stones were published in 2001 by Dickmann et al.112 and Klein et al.,113 who studied the discoloration of marble following the laser cleaning, by Hildenhagen and Dickmann in 2003,114 by Colao et al.115 in 2004 and by Khedr et al.116 in 2011. The LIBS analysis of historical marble for provenience studies was proposed by Lazic et al. in 2004117 and then performed in 2007 by Bakry on marble from Jeddah, Saudi-Arabia,118 Mahmood et al.119 in 2010, on a variety of marble samples extracted from Quetta region of Boluchistan, Pakistan, and by Fahad and Abrar in 2018.120 Columbu et al.121 used LIBS for the analysis of limestone Nuragic statues from the Mont'e Prama archaeological site (XI–IX cent. BC) in Sardinia (see Fig. 8). A blind clustering analysis of the LIBS spectra based on Graph Theory122 helped in determining the provenance of raw materials employed for the artefacts, indicating the compatibility of all analysed statue samples with geological outcrops from the Santa Caterina di Pittinuri area.
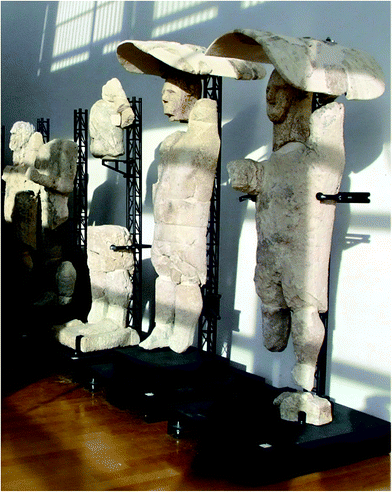 |
| Fig. 8 The ‘Giants’ of Mont'e Prama, analysed by LIBS in ref. 121 (photo S. Carboni). | |
The potential of LIBS for underwater analysis of marble has been demonstrated in 2005 by Lazic et al.123 and by Guirado et al. in.124
An interesting study for the mapping of historic buildings was proposed by Fortes et al.125 in a study performed at the Cathedral of Malaga, in Spain. The authors used a standoff system to reach areas of the building otherwise difficult to access without expensive scaffolding, demonstrating the possibility of remote LIBS characterization and monitoring of stone degradation in historical and modern buildings. In their work, the qualitative characterization of different materials was carried out following a well-defined and structured protocol, in order to reach an accurate description of the construction materials with a good spatial resolution. Brai et al.,126 in their study on bricks and mortar sampled in the ancient Greek-Roman Theatre of Taormina, used XRF and LIBS to distinguish the chemical characteristics and state of degradation of these building materials. Hemeda127 combined different techniques (SEM/EDX, MO, XRD, DT-TGA, etc.) focusing on the study of construction materials at the Kom El-dikka site in Alexandria, Egypt.
Raneri et al.128 studied mortar LIBS elementary maps with image-processing methods, coupling the qualitative analysis to the data provided by optical and scanning electron microscopy. Senesi et al.129 in 2018 presented an innovative application of LIBS micro-mapping for the characterization of the surface degradation of the construction materials of the Castello Svevo in Bari (Italy). The same authors also took advantage in 2018 of the handheld LIBS instrumentation that is now available for the analysis of stone monuments,130 using a Calibration Free (CF-LIBS) approach131 for obtaining a quantitative determination of the elemental composition of the sample.
2.4 LIBS analysis of bones, teeth and other organic materials
The LIBS technique has been proposed, in the past, for the analysis of biological samples for medical diagnostics132 and forensic science.133 The same methods used in that field can be applied to the study of archaeological biological findings (or human or animal origin) such as bones, teeth and other organic materials. Alvira et al.134 in 2010 applied LIBS to the determination of strontium and magnesium in dentin and enamel of Neolithic, middle age, and modern Homo sapiens teeth. The authors noted that the values of the Mg/Ca and Sr/Ca ratios are higher in dentin than in enamel, but also reported the existence of considerable heterogeneity in the distribution of these elements within and between dental tissues. They finally considered that this kind of analysis could be useful for evolutionary anthropology studies, since the data acquired can provide information regarding early nutrition, seasonality, and residential mobility.
Galiová et al.135 in 2010 analyzed by both LIBS and LA-ICP-MS the dentine of a prehistoric bear (Ursus arctos) tooth. Using the LIBS technique Abdel-Salam et al.212 in 2007 demonstrated a simple method for the qualitative and quantitative estimation of the hardness of calcified tissues (enamel of human teeth, shells and eggshell) based on the LIBS plasma ionic-to-atomic line intensity ratios of Mg (or Ca).
Rusak et al.136 in 2011 studied by LIBS the conservation status of 6000 year-old sheep and cattle archaeological bones, finding that the Ca/F ratio was higher in well preserved bones with respect to degraded ones.
Garofano et al.137 in 2011 studied the archaeological case of the ‘Venice vampire lady’. The skull of a female individual was discovered during the excavation of a XVI century mass grave in Venice, with a brick inserted in the mouth for keeping it wide open. The forensic anthropological analysis evidenced that the brick was inserted in the mouth post-mortem, as part of a ritual against vampires believed to survive in the grave eating their own funeral shrouds. The LIBS analysis of the bones for the determination of the paleo-diet of the ‘Venice vampire lady’, whose age at death was determined by the anthropological analysis to be around 60 years, indicated a dominant vegetarian diet, possibly integrated with fish. The LIBS analysis confirmed the hypothesis of a low social status of the deceased, also suggested by the presence of arthritic deformation of the left shoulder probably due to a fatiguing work.
Kasem, Russo and Harith in 2011 and 2014 determined from the LIBS analysis of archaeological bones and teeth the dietary habits of individuals belonging to four different ancient Egyptian dynasties representing the Middle Kingdom (1980–1630 B.C.), Second Intermediate Period (1630–1539/23 B.C.), the Late Period (664–332 B.C.) and the Roman–Greek Period (30 B.C.–A.D. 395).7,138 The authors pointed out that any conclusion about dietary habits must take into account postmortem effects such as biological degradation and diagenetic effects (especially from the surrounding soil).
In a similar study, in 2015 Al-Khafif and El-Banna139 studied the dietary habits of ancient Egyptians through the LIBS analysis of mandibles excavated from Qubbet el Hawa Cemetery in Aswan (Egypt). The authors in particular focused their attention on three successive eras: First Intermediate Period, Middle Kingdom, and Second Intermediate Period. They found that in the First Intermediate period the Sr/Ca ratio was significantly lower with respect to other periods and interpreted this result as the indication of consumption of imported cereals in the years of famine. The increase of this ratio in the Middle Kingdom and Second Intermediate Period was interpreted as the result of the improvement of the climatic, social, economic, and political conditions and a reflection of the amelioration of the agriculture conditions at those times.
Also of interest for cultural heritage and archaeological studies is the LIBS analysis of foxing stains in paper and parchment in 2002 by Bicchieri et al.140 and the analysis of coral and hard wood prayer beads performed by Lie et al. in 2006.141
2.5 LIBS analysis of glass and precious stones
Many other materials of interest for cultural heritage and archaeology have been studies using LIBS. Müller and Stege142 in 2003 evaluated the potential of LIBS for the analysis of historical glass. In 2005, Carmona et al.143 analyzed corroded glass by LIBS, classifying a group of historical glass based on the lead content. The authors found that the composition of the corroded layer and of the bulk glass could be determined with minimal disruptive analysis. Szelagowska et al.144 studied medieval stained glass from St. Mary's Church and Corpus Christi Basilica in Krakow (14th–16th century) and from Polish historical buildings (18th–20th century) coupling the LIBS analysis with SEM/EDX, for correlating the glass composition with the morphology of the samples. The authors were able to distinguish between medieval and modern glass on the basis of their component (soda–lime–silicate for modern glass and potash–lime–silicate for historic glass); on this basis, they were able to classify an unknown stained glass sample as modern glass. Palomar et al.139 and Oujja et al.140 between 2013 and 2015 studied the process of degradation of Roman glass with LIBS stratigraphy, finding that this technique was particularly useful for glass conserved under burial conditions.
LIBS has also been used for the analysis of precious stones by McMillan et al.145 in 2006; the findings of this study were used for demonstrating the possibility of determining the provenience of beryl in 2008.146 Agrosì et al. used standard-less micro-LIBS for the analysis of emeralds in 2014 (ref. 147) and on six samples of a rare red variety of beryl, using calibration free-LIBS analysis.47 The authors demonstrated the capability of LIBS for discriminating between red beryl (Be3Al2(SiO3)6) and pezzottaite (raspberry beryl, Cs(Be2Li)Al2Si6O18) by observing the differences in Cs and Li emission lines.148
Koral et al.149 demonstrated the possibility of performing minimally destructive LIBS analysis on transparent samples and gemstones (glass, tourmaline, aquamarine and ruby) using Nanoparticle-Enhanced LIBS (NELIBS).
Finally, in 2018 McManus et al.150 coined the term Quantagenetics® (from quantum, referring to the quantum properties of light and genesis, or origin) for the process of authentication of materials through their LIBS spectra. The method, in spite of its fancy name, is based on a simple correlation analysis between the LIBS spectra, using the Euclidian distance between them; in any case, with their method the authors were able to classify the provenience of 510 emeralds from 18 Colombian mines, with a success rate of 99.4%.
3 LIBS in combination with other techniques
When analyzing cultural heritage objects, there is the need to obtain as comprehensive as possible information, and it is often reached by using two or more complementary analytical techniques.
Many examples of integrated approaches, based on the use of independent instruments, have been published in the literature, although the realization of hybrid devices sharing common parts represents the most interesting topic in this field. An obvious advantage of this set-up is that objects will not need to be moved from one instrument to the other, reducing analysis time, and more importantly the same spot on the object's surface can be easily probed. In this chapter we will highlight the application where the combination of LIBS with one or more techniques presented evident synergies, giving more information than the ones that might be obtained through the separate application of each technique.
3.1 LIBS and Raman spectroscopy
LIBS can be combined with spectroscopic techniques such as laser-induced fluorescence (LIF) spectroscopy, diffuse reflectance spectroscopy, and hyperspectral imaging; nevertheless the most explored hybridization for the analysis of cultural heritage materials is with Raman spectroscopy.151–153 The required spectral resolution and optimal spectral region for Raman and LIBS measurements are indeed roughly similar, and both techniques utilize similar equipment for spectra induction and detection.
An important concern encountered in this combination is the protection of the sample from excessive energy loads that might lead to thermal damage or even surface ablation, thus hindering Raman analysis. Moreover, the optimal wavelength to fit both LIBS and Raman is the 2nd harmonic (532 nm) of a Nd:YAG laser, which nonetheless may prevent the recording of the Raman signal in CH samples rich in organic binding materials, due to the emission of unwanted fluorescence.
Over the years, several approaches have been suggested to combine Raman and LIBS techniques in a single instrument: two laser sources (CW laser for Raman; pulsed laser for LIBS),83,154–156 the same laser system adjusting the energy of the laser pulse (low energy for Raman; high energy for LIBS);157–161 simultaneous Raman and LIBS measurements in a single pulse differentiated in time (leading edge of the nanosecond laser pulse for Raman; laser pulse tail for LIBS)162 or space (scattering photons from the intact edge for Raman; emitting photons from the inner region for LIBS);163 simultaneous double pulse measurements dividing Raman and LIBS signal acquisition using a few microsecond delay (first low energy pulse for Raman; second high energy pulse for LIBS).164
The first example of the LIBS-Raman instrument applied to the analysis of artworks has been proposed in 2006 by Giakoumaki and coworkers for pigment identification.158 In their hybrid system, a single nanosecond Q-switched Nd:YAG laser working with the 2nd harmonic (532 nm) is used as an excitation source for LIBS and Raman measurements. Raman scattering and atomic emission are collected through the same lens used to focus the laser beam, and then a CCD detector is used operating in the continuous mode for recording the Raman signal and in the gated mode for the LIBS signal. The Raman or LIBS analysis is performed by simply adjusting the energy of the laser pulse below or above the material's ablation threshold, respectively. Working in single pulse mode and with 2–4 mJ as beam energy (fluence: 6–12 J cm−2), the authors demonstrated that almost four cycles of Raman-LIBS analysis could be performed at the same spot, which allows compositional depth-profiling studies. Later, the same configuration has been applied by Osticioli et al.159 to the analysis of three different samples of artistic/architectural interest.
Hoehse et al.155 improved the spatial resolution of the system with a newly designed dual arm echelle spectrometer for both techniques, also reducing measurement time and increasing robustness because no moving parts (gratings or prisms) are required. The performances of the instrument were demonstrated by the mapping of heterogeneous mineral samples and in-depth analysis of pigments.
As the employment of a single pulsed laser for both methods leads to unfavourable signal-to-noise ratios in Raman spectroscopy due to the low duty cycle, recently Lednev et al.164 developed a new approach combining Raman and LIBS within a single laser event running the laser in double pulse mode. The possibility of accurately controlling power density during Raman measurement prevents the thermal alteration of the sample. Moreover, the independent gating (Raman camera is gated during the first laser pulse interaction; LIBS camera is triggered after short delay with the second laser pulse) enables the acquisition of high-quality Raman/LIBS spectra.
3.2 LIBS and X-ray fluorescence
In 2007, Ferretti et al.,165 in the framework of an experimental campaign on the so-called “Porticello Bronzes”, at the Museo of the Magna Grecia in Reggio Calabria (Fig. 9), proposed the use of LIBS and XRF, with the purpose of reducing the impact on the samples caused by the application of the micro-destructive LIBS technique through a preliminary screening performed using non-destructive XRF.
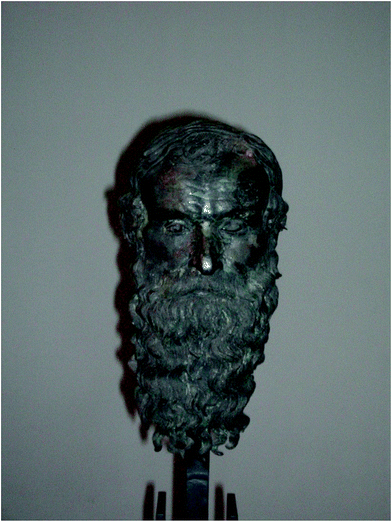 |
| Fig. 9 The “Philosopher Head” of Porticello (ref. 165). | |
The intuition of the authors was confirmed by the finding that, from a qualitative point of view, the elemental composition determined by XRF was equivalent to what was determined by LIBS, thus allowing the use of XRF for general classification, reducing the LIBS measurements to the minimum necessary for the precise quantitative determination of the sample composition. The effectiveness of this approach was confirmed in the subsequent studies on the “Magnificent Crater” of Trebeništa, Macedonia166 (Fig. 10), where LIBS was used for the precise determination of the composition of the ternary bronze alloy on the basis of which some applications were reproduced according to the ancient manufacturing techniques.
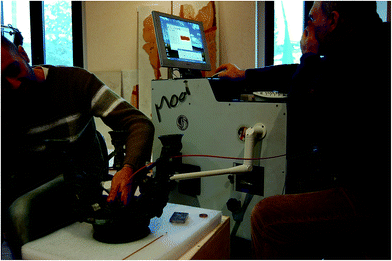 |
| Fig. 10 LIBS measurements on the Trebeništa Crater (ref. 166). | |
LIBS was also used by Pardini et al.167 in 2012, for a preliminary assessment of the validity of XRF quantitative analysis on Roman silver denarii, characterized by a moderate surface patina. Alberghina et al.,168 in contrast, used micro-XRF for validating LIBS quantitative analysis on bronze alloys. The same authors used LIBS and XRF in 2011 for the study of the corrosion process in bronze169 and, subsequently, for the study of the materials from the Greek Theater in Taormina170 and for the stratigraphic analysis of pictorial surfaces.82 As already mentioned in the section devoted to the applications of LIBS to metals, Agresti et al. in 2016 used LIBS and XRF, together with neutrons and X-ray diffraction, for the analysis of three bronze figurines from the collection of the Egyptian Museum of Florence.41,42 Also Lorenzetti et al.55 used X-ray fluorescence and LIBS for the determination of the alloy of the Pisa Griffin. A comparison of the performances of LIBS, XRF and PIXE techniques for CH and archaeology studies has been recently published by Lazic et al.171
4 Specific applications
4.1 LIBS for restoration
LIBS has been frequently proposed and used as a diagnostic tool for monitoring the effectiveness of the laser cleaning process for artwork conservation. Tools such as the naked eye of the conservator or the use of different types of microscopy and spectroscopy can indeed provide good control, but with limited capabilities (i.e. the requirement of experienced operators, the use of off-line instrumentation or even sample collection). In contrast, LIBS can be performed in situ by using pulses from the same cleaning laser, allowing the on-line monitoring of the material removal by recording the corresponding spectra.
On-line monitoring of the restoration process is crucial to define properly the end point of cleaning or to study complex stratigraphies. Here the ability of LIBS to perform depth profile analysis comes in, revealing for example the presence of overpainting or retouching in successive paint layers,61 the extent of contaminant layers on objects of cultural value,172 or even the presence of chlorine useful for monitoring the status of historical buildings.173
In a pioneering study, Gobernado-Mitre and coworkers174 evaluated the use of LIBS to monitor the laser cleaning process of limestone from a historical building. The determination of the different chemical composition of the black crust and the underlying limestone proved to be useful in order to avoid surface over-cleaning. The same principle has been applied on painted artworks,21,61,175–177 stained glass,178 marble,111,115,179–181 sandstone,178 metals and alloys.182–185
The instrumental configurations developed for monitoring of the restoration processes are worth noting more than the specific application. The versatility of LIBS allows indeed designing portable laser cleaning stations for the in situ restoration in museums and art galleries.176 Lentjes et al.,186 to overcome the disadvantages of ICCD detectors (high investment costs, ambience sensitivity and complexity), proposed the use a commercial miniature spectrometer applicable for the detection of “spectral fingerprints”, through the analysis of the correlation between the spectra from the ablated material and reference spectra.
Finally, also the double pulse LIBS configuration has been applied for restoration purposes. Colao and coworkers182 reported the use of collinear double pulse LIBS for the laser cleaning of ancient bronze alloys. In this application, the first pulse was utilized to remove the contamination layer on the sample surface, while the second was used for quantitative analysis. Fortes et al.187 developed an orthogonal double pulse LIBS configuration in a re-heating scheme as a diagnostic tool for the restoration of archaeometallurgical samples. Here the second pulse allows the analysis of the material eroded in the cleaning stage, thus avoiding the damage of the sample.
4.2 Underwater LIBS
One of the reasons for the wide application of LIBS lies in the possibility of virtually performing the analysis of any kind of substance (solid, liquid, gaseous or aerosol) in air, in vacuum, in fluids, and even under extreme conditions such as high temperature and pressure environments. This aspect is of particular interest in the analysis of archeological materials, since most of these pieces are found submerged in sea environments. Experts estimate that more than three million shipwrecks currently lie on sea floors, and of them scientists have explored less than one percent.188
However, the LIBS analysis of solids in liquids generates phenomena like motion, shaking and splashing, which change laser focusing and generate instability in the plasma. These effects lead to unstable atomic emission, resulting in unsatisfactory figures of merit (i.e., sensitivity, precision and limits of detection) as compared to solid or gas sample LIBS, and in spectral emission characterized by a broad spectral continuum.189
Several attempts to improve the sensitivity in liquids using different instrumental developments have been presented. Dual pulse excitation, being the most common approach proposed in the literature for the analysis of submerged solids, has been thoroughly discussed in 2007 by De Giacomo et al., together with the basic aspects of underwater LIBS.189 Briefly, in the double pulse-LIBS (DP-LIBS) approach the first laser pulse produces a gas bubble whereas the second pulse ablates the sample and re-excites the plasma inside the bubble. In two consecutive studies, Lazic et al. examined different processes involved during the laser–water interaction, the dependence of DP-LIBS signal intensity inside liquids on the interpulse delay, and the influence of the optical properties of the vapor bubble induced by the first laser pulse on the formation and detection of the secondary plasma.190,191
Researchers demonstrated the capability of DP-LIBS to perform underwater analysis of a wide range of materials, from metallic alloys (iron, bronze and precious alloys) to non-metallic samples (rocks and wood).192
The DP approach has also its own limitations, related to the use of two optical paths and their laborious alignment, to the applicability limited to liquid media transparent to the laser pulses and to the poor efficiency of the system beyond 100 bar. The pioneering approach proposed by Beddows and coworkers in 2002, aiming to overcome these problems, is based on a fiber optic cable, which delivers the ablation laser pulse and a buffer gas flow to the sample surface, and collects the emitted light.193 The buffer gas blowing onto the sample surface offers a quasi-gaseous environment for the plasma, guaranteeing a temporal evolution of the plasma itself similar to that obtained in ambient-air media. Moreover, the displacement of the water due to gas shielding makes the analysis feasible also in non-transparent aqueous media. The instrument was tested in water at depths of approximately 180 cm, which is notable, but the pressure differential there with respect to the water surface was not critical compared to more plausible and deeper sampling sites under water.
The performances of underwater single pulse-LIBS have been further improved by the use of long-pulse laser excitations, after Sakka et al.194 demonstrated that a good signal quality can be obtained working with a pulse of 150 ns duration as opposed to pulses of <20 ns duration. The mechanisms involved during long-pulse irradiation are surely different from those that take place during double-pulse irradiation, even if still little is known about them.
Regarding the application of underwater LIBS analysis to archeological investigations, the first paper dealing with this topic dates back to 2005, when Lazic and coworkers presented a dual pulse system for the examination of different classes of materials potentially found in undersea archaeological parks (i.e. iron, copper-based alloys, precious alloys, marble and wood).123 Samples were analyzed in the laboratory after immersion in simulated sea water (20 mm water column height above the focal spot). While no particular instrumental set-up was required for this application, the main problems were encountered in the presence of ablated particles, which progressively reduces the water transparency, and because of very weak plasma emission intensities from submerged stones.
For archaeologists, the possibility of performing in situ elemental analysis is of great interest when the submersed artifacts are not immediately recognizable due to their high corrosion degree or to low visibility conditions. Laserna and coworkers from the University of Malaga constitute the most active group in this field, with four publications focused on in situ and stand-off submarine LIBS analysis of solid samples. In 2012 they presented an instrument comprised of a main unit for laser-fiber coupling and signal detection, and of an underwater probe, interconnected by means of a 40 m long umbilical cord.195 Both the laser beam and the signal are transmitted through the same fiber optic cable (45 m long), whereas a coaxial gas flux creates a solid–gas interface and improves the ablation efficiency. Metallic archaeological samples were analyzed underwater at 30 m in the Mediterranean Sea by a professional diver able to operate the hand-held probe.
The technological evolution of this instrument, based on the multi-pulse transmission through the optical fiber cable, was presented in 2015.124 Multi-pulse LIBS opens the possibility to increase the laser beam energy transmitted while avoiding the damage of the fiber, and operating in a collinear configuration it uses a single laser source.196 This configuration was tested for the identification of archeological assets in two real shipwrecks, and for the discrimination of submersed objects having different nature (bronze alloys, metallic pieces, ceramics and marble) through LDA classification.124,197 In the last case, from the initial emission spectrum of 2048 data points, only 10 spectral variables were considered for discriminant analysis, and the resulting model allowed the identification of all considered samples.
The same research group investigated the potential of a stand-off LIBS instrument to work in an underwater open-path environment, thus removing the need to work with a qualified diver.198 Samples were analyzed at distances up to 80 cm from the sensor at a solid–water interface, using a double pulse collinear configuration. Important parameters, such as the effect of water temperature and the influence of path length on the LIBS signal were investigated.
5 New developments and prospects
5.1 Micro-LIBS analysis and 3D compositional mapping
The high spatial resolution of the LIBS technique (<10 µm lateral resolution; <1 µm in depth resolution) can be fruitfully exploited for obtaining 3D compositional maps of cultural heritage objects. The first application of LIBS mapping in cultural heritage was presented by Corsi et al. in the year 2000, on a Roman fresco (II century A.D.) from St. Albans (UK).60 More recently, the coupling of the laser with an optical microscope199 has paved the road to LIBS-based microanalysis also in cultural heritage and archaeology; Pagnotta et al. in 2017 studied ancient Roman mortar (5th century A.D.), using different statistical approaches for the determination of the chemical composition of the binder and aggregate fraction in the compositional maps. A similar study on a set of mortar samples, suitably characterized by the occurrence of volcanic rock fragments with a relevant range in grain sizes, revealed in 2018 the potentiality of the method to provide compositional and spatial distribution data on aggregate grains and hydraulic phases.128 Pagnotta et al. in 2018 also proposed the use of the remains of thin-section realization (‘negative face’) for the surface mapping of Neolithic potsherds, demonstrating that the combination of these images with algorithms derived from image processing techniques may provide interesting information and supporting data to in-depth investigate pottery components detected by optical microscopy observation.106 The capability of performing fast quantitative analysis of the LIBS spectra obtained from 3D mapping was shown by the same group in 2018.108
In 2018, Senesi et al. obtained a full microscale three dimensional (3D) compositional imaging of weathered limestone from Castello Svevo in Bari (Italy). The analysis allowed the creation of ‘virtual thin-sections’ (VTS) of the samples, from which the extent of the alteration processes occurred at the limestone surface was estimated129 (see Fig. 11).
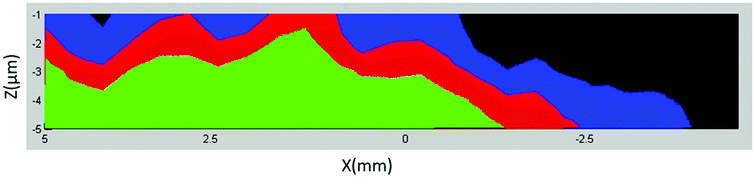 |
| Fig. 11 Virtual thin section of the black crust on the limestone surface from Castello Svevo in Bari, Italy (ref. 129), showing the change of mineralogical composition in the transition from the black crust layer (black) to the limestone underneath (green). | |
An interesting development of the micro-LIBS technique has been its integration with Laser Ablation ICP Mass Spectrometry (LA-ICP-MS), firstly proposed by Meissner et al. in 2004.200 In 2008, Novotný et al. used micro-LIBS and LA-ICP-MS separately, for elemental, large area 3D mapping of granite.201 In general, LA-ICP-MS is more performant with respect to LIBS for what concerns the limits of detection, trueness and precision. However, MS techniques suffer from interference that might make difficult the determination of some elements (H, N, O, Si, Se, As, and S), which are, instead, measurable by LIBS. Tandem LIBS/LA-ICP-MS has been successfully applied to archaeological objects by Syta et al.90 on cross sections of mediaeval Nubian objects, for the study and discrimination of Egyptian blue vs. lapis-lazuli. It is worth noting, however, that the sequential acquisition of LIBS and mass spectra has been preferred by Kokkinaki et al. against simultaneous application of the two methods, for optimizing mass resolution and minimizing the surface damage of the samples, in the case of low ablation threshold materials.202 On the other hand, the micro-destructivity of both LIBS and LA-ICP-MS techniques clearly limits the possibility of obtaining 3D elemental maps of the same regions on the sample, when a sequential approach is applied.
5.2 Surface- and nanoparticle-enhanced LIBS
One of the main advantages of LIBS is the absence of a sample preparation step for the analysis. Most of the proposed techniques for the enhancement of LIBS performances aim to maintain this condition, focusing on the implementation of high-power sources, tunable lasers or high-resolution detectors.
A technique recently introduced by De Giacomo et al.203 leads to a minimal sample pretreatment, based on the deposition of metallic nanoparticles on the sample surface. A review was recently published by Dell'Aglio et al.,204 discussing in depth the mechanisms behind Nanoparticle Enhanced LIBS (NELIBS), its advantages and limits.
It has been demonstrated how the deposition of metallic nanoparticles (NPs) on a sample can strongly influence the interaction between the laser radiation and the sample surface, depending on the dimensions, the concentration and the nature of the NPs.
Assuming a suitable deposition of noble metal nanoparticles and a laser pulse of nanosecond duration, a general explanation of the involved phenomenon can be given as follows.
Upon irradiation, most of the energy delivered by the laser pulse is deposited on the nanoparticles, inducing a coherent and collective oscillation of the electrons on the surface of the NPs. This amplifies the electric field localized on the surface and in the gaps between the NPs, causing an increase in the intensity of the incident laser radiation. This phenomenon causes some electrons to be extracted from the surface of the conductive NPs, inducing a faster breakdown of both the NPs and the sample.
In addition, if the incident laser radiation is resonant with the surface plasmon of the NPs deposited on the samples, another phenomenon takes place. The pulse energy is absorbed by the NPs that are immediately heated as well as the surrounding region. The heat is then transferred to the sample, inducing a localized vaporization and a very efficient plasma excitation, while the damage to the sample surface is confined and of the same order of the NP diameter.
In their studies, De Giacomo et al.149,203,205–208 extensively demonstrated how NELIBS can be applied to a variety of samples, using a Nd:YAG laser operating at 1064 nm with pulses in the nanosecond regime and gold or silver NPs.
The best results have been obtained with the analysis of metallic samples (i.e. a pure titanium target) where an enhancement factor, the ratio between the NELIBS and the LIBS emission line intensity, of up to 100 has been registered. In this case the NPs are simply deposited dropwise on the cleaned sample surface and dried before performing the analysis.
Alternatively, it has been shown how, if the NPs are deposited on a dielectric support such as glass, Teflon or silicon, a substrate for the analysis of liquid samples can be obtained. A drop of the liquid sample is deposited on the dried NPs and the solvent evaporated. This allowed for the detection of sub-ppm analytes and significantly improved the technique's LOD.
Furthermore, NELIBS allowed for the enhancement of also molecular bands. In this case, an enhancement factor of about 10 was obtained and a longer persistence of plasma emission was registered when compared to conventional LIBS analyses.
Being a novel technique, NELIBS has not yet been tested for cultural heritage applications. Nevertheless, it has proven to be a highly versatile technique, suitable for the analysis of samples of different nature.
The most obvious field of application would be the study of metallic and precious artifacts. Due to the well proven capability of NELIBS for highly enhancing the LODs of trace elements in alloys and gemstones and the reduced damage dealt to the sample surface, it can be a valuable tool for the identification and the investigation of artifacts, coins, jewelry, etc.
There are many advantages in using NELIBS when studying transparent materials, such as glass and gemstones. First of all, a transparent or translucent sample can make difficult an optimal focusing of the laser beam on the surface, where a layer of NPs is more easily visible. Moreover, glass and gemstones usually have high breakdown thresholds that, in turn, require a much higher pulse energy to produce a sufficiently energetic plasma and this can lead to an excessive damage of the sample. Due the effect of the NPs, a lower energy is required to generate the plasma, resulting in a more “gentle” ablation, thus preserving the integrity of the sample.
A recent study by Poggialini et al.209 illustrated the possibility of using green-synthetized silver NPs for NELIBS with a low power and mobile instrument. Trace elements in copper samples were analyzed with enhancement factors of about 4 and LODs in the order of ppm. This demonstrated the possibility of using a cost-effective alternative to commercially available NPs for NELIBS analyses. The possibility of using this approach also for in situ analyses makes it more suitable and appealing for CH applications.
One of the criticalities of NELIBS application in cultural heritage would be the contamination of the sample. While the NPs are removed from the measurement spot by the laser pulse, it is not uncommon that the area where the NPs are deposited is larger than the ablated area, leaving an amount of metallic NPs on the sample surface. This could lead to a series of oxidation and contamination processes that are not acceptable with CH artifacts that are, usually, one-of-a-kind. However, due to the very simple method of sample preparation (i.e. dropwise evaporation of a colloidal solution), no chemical reaction is involved in the NP deposition. This suggests that the remaining nanoparticles could be removed from the sample surface with wet swabs.
Surface Enhanced LIBS (SENLIBS) is another method for improving the performances of the technique.210 In this case, a liquid sample is deposited and dried on a suitable solid surface, generally a metal sheet. As opposed to NELIBS for liquid samples, where the laser pulse interacts with the surface plasmon of the NPs, in SENLIBS a dense and hot plasma is generated on the substrate surface and engulfs the dried droplets, enhancing the LIBS signal.
The applicability of SENLIBS to exclusively liquid samples may restrict its use in CH, where the vast majority of the samples are solid. There are, however, some cases in which SENLIBS can be seen as a valuable addition to more conventional techniques.
A study by Campanella et al.211 demonstrated how SENLIBS can be combined with HPLC-DAD analyses in order to obtain information on the inorganic fraction of an extract in addition to the organic fraction data. In their work, a series of textile samples from both reference standards and historical tapestries were subjected to an extraction process. While analyzing organic dyes by LC techniques, the part of the extract containing the inorganic mordant is usually discarded. In this work, microdroplets of the extract were deposited on various substrates and analyzed by LIBS. This allowed for an easy discrimination of the mordant and, in turn, of the dye.
6 Conclusions
LIBS applications in cultural heritage and archaeology are, as we have seen, a small niche in the slightly larger niche that LIBS occupies in the scene of the current analytical techniques. However, when applied correctly, the technique has demonstrated to give interesting results, providing answers to the main problems in the field, which are authentication, dating and conservation. The future of LIBS in cultural heritage and archaeology laboratory studies mainly relies on the development of cheaper and more performant micro-LIBS systems, possibly coupled to mass spectrometers, and on the full comprehension and consequent exploitation of the nanoparticle LIBS enhancement, which might lead to a drastic reduction of the destructivity of the technique. The results recently obtained on precious stones and glass seem very promising. On the other hand, in situ application will benefit the realization of truly portable LIBS/XRF and LIBS/Raman instrumentation, for complementing the positive features of the technique and compensating for its weaknesses.
Conflicts of interest
There are no conflicts to declare.
List of acronyms and abbreviations
3D | Three dimensional |
AAS | Atomic absorption spectrometry |
A.D. | Anno domini |
B.C. | Before christ |
CF-LIBS | Calibration-free LIBS |
CH | Cultural heritage |
DAD | Diode array detector |
DP | Double pulse |
DT | Differential thermal |
ED-XRF | Energy dispersive X-ray fluorescence |
EDX | Energy dispersive X-ray |
HLPC | High performance liquid chromatography |
ICP | Inductively coupled plasma |
FT-IR | Fourier transform infrared |
LA | Laser ablation |
LASER | Light amplification by stimulated emission of radiation |
LC | Liquid chromatography |
LDA | Linear discriminant analysis |
LIBS | Laser-induced breakdown spectroscopy |
LIF | Laser-induced fluorescence |
LOD | Limit of detection |
MS | Mass spectrometry |
MO | Microscope observation |
NELIBS | Nanoparticle enhanced LIBS |
NP | Nanoparticle |
OCT | Optical coherence tomography |
Modì | Mobile dual-pulse instrument |
PCA | Principal component analysis |
PIXE | Particle induced X-ray emission |
PLS-DA | Partial least squares discriminant analysis |
REE | Rare earth elements |
SENLIBS | Surface-enhanced LIBS |
SEM | Scanning electron microscope |
SIMCA | Soft independent modelling of class analogy |
SOM | Self organizing maps |
TGA | Thermal gravimetric analysis |
UV | Ultraviolet |
VIS | Visible |
XRD | X-ray diffraction |
XRF | X-ray fluorescence |
YAG | Yttrium aluminium garnet |
Acknowledgements
The authors thank the National Gallery, London, UK, Museo Nazionale della Magna Grecia, Reggio Calabria, Italy, Museo Archeologico di Crotone, Italy, Museo Archeologico Nazionale di Cagliari, Italy, and Museo dell'Opera del Duomo, Pisa, Italy, for the use of the images.
Notes and references
-
A. W. Miziolek, V. Palleschi and I. Schechter, Laser induced breakdown spectroscopy (LIBS): Fundamentals and applications, 2006, DOI:10.1017/cbo9780511541261.
- L. J. Radziemski, From LASER to LIBS, the path of technology development, Spectrochim. Acta, Part B, 2002, 57(7), 1109–1113, DOI:10.1016/s0584-8547(02)00052-6.
- R. Noll, C. Fricke-Begemann, S. Connemann, C. Meinhardt and V. Sturm, LIBS analyses for industrial applications – an overview of developments from 2014 to 2018, J. Anal. At. Spectrom., 2018, 33(6), 945–956, 10.1039/c8ja00076j.
- D. Bulajic, G. Cristoforetti, M. Corsi, M. Hidalgo, S. Legnaioli, V. Palleschi, A. Salvetti, E. Tognoni, S. Green, D. Bates, A. Steiger, J. Fonseca, J. Martins, J. McKay, B. Tozer, D. Wells, R. Wells and M. A. Harith, Diagnostics of high-temperature steel pipes in industrial environment by laser-induced breakdown spectroscopy technique: The LIBSGRAIN project, Spectrochim. Acta, Part B, 2002, 57(7), 1181–1192, DOI:10.1016/s0584-8547(02)00060-5.
- G. Lorenzetti, S. Legnaioli, E. Grifoni, S. Pagnotta and V. Palleschi, Laser-based continuous monitoring and resolution of steel grades in sequence casting machines, Spectrochim. Acta, Part B, 2015, 112, 1–5, DOI:10.1016/j.sab.2015.07.006.
- K. Y. Yamamoto, D. A. Cremers, M. J. Ferris and L. E. Foster, Detection of Metals in the Environment Using a Portable Laser-Induced Breakdown Spectroscopy Instrument, Appl. Spectrosc., 1996, 50(2), 222–233, DOI:10.1366/0003702963906519.
- M. A. Kasem, R. E. Russo and M. A. Harith, Influence of biological degradation and environmental effects on the interpretation of archeological bone samples with laser-induced breakdown spectroscopy, J. Anal. At. Spectrom., 2011, 26(9), 1733–1739, 10.1039/c1ja10057b.
- L. Sancey, V. Motto-Ros, B. Busser, S. Kotb, J. M. Benoit, A. Piednoir, F. Lux, O. Tillement, G. Panczer and J. Yu, Laser spectrometry for multi-elemental imaging of biological tissues, Sci. Rep., 2015, 4(1), 6065, DOI:10.1038/srep06065.
- M. Corsi, G. Cristoforetti, M. Hidalgo, S. Legnaioli, V. Palleschi, A. Salvetti, E. Tognoni and C. Vallebona, Application of laser-induced breakdown spectroscopy technique to hair tissue mineral analysis, Appl. Opt., 2003, 42, 30, DOI:10.1364/ao.42.006133.
- A. Gupta, J. M. Curran, S. Coulson and C. M. Triggs, Comparison of intra-day and inter-day variation in LIBS spectra, Forensic Chem., 2017, 3, 36–40, DOI:10.1016/j.forc.2016.12.002.
- M. Tofanelli, L. Pardini, M. Borrini, F. Bartoli, A. Bacci, A. D'Ulivo, E. Pitzalis, M. C. Mascherpa, S. Legnaioli, G. Lorenzetti, S. Pagnotta, G. De Holanda Cavalcanti, M. Lezzerini and V. Palleschi, Spectroscopic analysis of bones for forensic studies, Spectrochim. Acta, Part B, 2014, 99, 70–75, DOI:10.1016/j.sab.2014.06.006.
- P. Vandenabeele and M. Kate Donais, Mobile Spectroscopic Instrumentation in Archaeometry Research, Appl. Spectrosc., 2016, 70(1), 27–41, DOI:10.1177/0003702815611063.
- V. Palleschi, If laser-induced breakdown spectroscopy was a brand: Some market considerations, Spectrosc. Eur., 2017, 29, 2 Search PubMed.
- A. Nevin, G. Spoto and D. Anglos, Laser spectroscopies for elemental and molecular analysis in art and archaeology, Appl. Phys. A: Mater. Sci. Process., 2012, 106(2), 339–361, DOI:10.1007/s00339-011-6699-z.
- A. Giakoumaki, K. Melessanaki and D. Anglos, Laser-induced breakdown spectroscopy (LIBS) in archaeological science-applications and prospects, Anal. Bioanal. Chem., 2007, 387(3), 749–760, DOI:10.1007/s00216-006-0908-1.
-
D. Anglos and J. C. Miller, Cultural heritage applications of LIBS, 2006, DOI:10.1017/cbo9780511541261.010.
- D. Anglos, Laser-induced breakdown spectroscopy in art and archaeology, Appl. Spectrosc., 2001, 55(6), 186A–205A, DOI:10.1366/0003702011952398.
- V. Spizzichino and R. Fantoni, Laser Induced Breakdown Spectroscopy in archeometry: A review of its application and future perspectives, Spectrochim. Acta, Part B, 2014, 99, 201–209, DOI:10.1016/j.sab.2014.07.003.
- R. Gaudiuso, M. Dell'Aglio, O. De Pascale, G. S. Senesi and A. De Giacomo, Laser Induced Breakdown Spectroscopy for Elemental Analysis in Environmental, Cultural Heritage and Space Applications: A Review of Methods and Results, Sensors, 2010, 10(8), 7434–7468, DOI:10.3390/s100807434.
- V. Lazic, L. Caneve, F. Colao, R. Fantoni, L. Fornarini and V. Spizzichino, Quantitative elemental analyses of archaeological materials by laser induced breakdown spectroscopy (LIBS) – An overview, Proc. SPIE-Int. Soc. Opt. Eng., 2005, 1–12, DOI:10.1117/12.612510.
-
D. Anglos, S. Couris, A. Mavromanolakis, I. Zergioti, M. Solomidou, W. Q. Liu, T. G. Papazoglou, V. Zafiropulos, C. Fotakis and M. Doulgeridis. Artwork diagnostics: laser induced breakdown spectroscopy (LIBS) and laser induced fluorescence (LIF) spectroscopy, in Proceedings of the first International conference on lasers in the conservation of Artworks, Heraklion, Greece, 1995, pp. 113–118 Search PubMed.
-
S. Legnaioli, G. Lorenzetti, L. Pardini, G. H. Cavalcanti and V. Palleschi, Applications of LIBS to the analysis of metals, 2014, DOI:10.1007/978-3-642-45085-3_7.
- E. Frahm and R. C. P. Doonan, The technological versus methodological revolution of portable XRF in archaeology, J. Archaeol. Sci., 2013, 40(2), 1425–1434, DOI:10.1016/j.jas.2012.10.013.
-
C. Pauna, B. Constantinescu, F. Constantin, R. Bugoi, D. Stan, A. Vasilescu, J. A. Caballero, C. E. Alonso, M. V. Andrés, J. E. García Ramos and F. Pérez-Bernal, Archaeometrical studies using X-ray fluorescence methods, 2010, pp. 187–188, DOI:10.1063/1.3428918.
- C. Arias, S. Bani, F. Catalli, G. Lorenzetti, E. Grifoni, S. Legnaioli, S. Pagnotta and V. Palleschi, X-Ray Fluorescence Analysis and Self-Organizing Maps Classification of the Etruscan Gold Coin Collection at the Monetiere of Florence, Appl. Spectrosc., 2017, 71, 5, DOI:10.1177/0003702816641421.
-
C. Arias, E. Grifoni, S. Legnaioli, G. Lorenzetti, S. Pagnotta and V. Palleschi, X-Ray fluorescence analysis of late Roman imperial coins, in IMEKO International Conference on Metrology for Archeology and Cultural Heritage, MetroArcheo, 2016 Search PubMed.
- E. Caponetti, F. Armetta, L. Brusca, D. Chillura Martino, M. Luisa Saladino, S. Ridolfi, G. Chirco, M. Berrettoni, P. Conti, N. Bruno and S. Tusa, A multivariate approach to the study of orichalcum ingots from the underwater Gela's archaeological site, Microchem. J., 2017, 135, 163–170, DOI:10.1016/j.microc.2017.09.003.
- M. F. Guerra, An overview on the ancient goldsmith's skill and the circulation of gold in the past: the role of x-ray based techniques, X-Ray Spectrom., 2008, 37(4), 317–327, DOI:10.1002/xrs.1013.
- A. Bertolini, G. Carelli, F. Francesconi, M. Francesconi, L. Marchesini, P. Marsili, F. Sorrentino, G. Cristoforetti, S. Legnaioli, V. Palleschi, L. Pardini and A. Salvetti, Modì: A new mobile
instrument for in situ double-pulse LIBS analysis, Anal. Bioanal. Chem., 2006, 385, 2, DOI:10.1007/s00216-006-0413-6.
- B. Sallé, P. Mauchien and S. Maurice, Laser-Induced Breakdown Spectroscopy in open-path configuration for the analysis of distant objects, Spectrochim. Acta, Part B, 2007, 62(8), 739–768, DOI:10.1016/j.sab.2007.07.001.
- M. Corsi, G. Cristoforetti, M. Giuffrida, M. Hidalgo, S. Legnaioli, L. Masotti, V. Palleschi, A. Salvetti, E. Tognoni, C. Vallebona and A. Zanini, Archaeometric Analysis of Ancient Copper Artefacts by Laser-Induced Breakdown Spectroscopy Technique, Microchim. Acta, 2005, 152, 105–111 CrossRef CAS.
- F. J. Fortes, M. Cortés, M. D. Simón, L. M. Cabalín and J. J. Laserna, Chronocultural sorting of archaeological bronze objects using laser-induced breakdown spectrometry, Anal. Chim. Acta, 2005, 554(1–2), 136–143, DOI:10.1016/j.aca.2005.08.081.
- L. Burgio, G. Cristoforetti, S. Legnaioli, V. Palleschi, L. Pardini, A. Salvetti and E. Tognoni, Quantitative LIBS analysis of samples from a le Sueur bronze, Proc. SPIE-Int. Soc. Opt. Eng., 2006, 6162, 616206, DOI:10.1117/12.674949.
- A. Foresta, F. Anabitarte García, S. Legnaioli, G. Lorenzetti, D. Díaz Pace, L. Pardini and V. Palleschi, LIBS analysis of twelve bronze statues displayed in the National Archaeological Museum of Crotone, Opt. Pura Apl., 2012, 45, 3, DOI:10.7149/opa.45.3.277.
-
M. Abdel Harith, Analysis of corroded metallic heritage artefacts using laser-induced breakdown spectroscopy (LIBS), in Corrosion and Conservation of Cultural Heritage Metallic Artefacts, Elsevier, 2013, pp. 100–125, DOI:10.1533/9781782421573.2.100.
- M. Abdelhamid, S. Grassini, E. Angelini, G. M. Ingo and M. A. Harith, Depth profiling of coated metallic artifacts adopting laser-induced breakdown spectrometry, Spectrochim. Acta, Part B, 2010, 65(8), 695–701, DOI:10.1016/j.sab.2010.03.017.
- J. Agresti, A. A. Mencaglia and S. Siano, Development and application of a portable LIPS system for characterising copper alloy artefacts, Anal. Bioanal. Chem., 2009, 395(7), 2255–2262, DOI:10.1007/s00216-009-3053-9.
- S. Siano, I. Cacciari, A. Mencaglia and J. Agresti, Spatially calibrated elemental depth profiling using LIPS and 3D digital microscopy, Eur. Phys. J. Plus, 2011, 126(12), 1–11, DOI:10.1140/epjp/i2011-11120-y.
-
J. Agresti, I. Cacciari and S. Siano, Optimized laser ablation elemental depth profiling of bronzes using 3D microscopy, in IET Conference Publications, 2016, retrieved from https://www.scopus.com/inward/record.uri?eid=2-s2.0-85018316093%26partnerID=40%26md5=f11240d9543c3aa8c34fc79cb64009e2 Search PubMed.
- I. Cacciari, J. Agresti and S. Siano, Combined THz and LIPS analysis of corroded archaeological bronzes, Microchem. J., 2016, 126, 76–82, DOI:10.1016/j.microc.2015.11.051.
- J. Agresti, I. Osticioli, M. C. Guidotti, G. Capriotti, N. Kardjilov, A. Scherillo and S. Siano, Combined neutron and laser techniques for technological and compositional investigations of hollow bronze figurines, J. Anal. At. Spectrom., 2015, 30(3), 713–720, 10.1039/c4ja00447g.
- J. Agresti, I. Osticioli, M. C. Guidotti, N. Kardjilov and S. Siano, Non-invasive archaeometallurgical approach to the investigations of bronze figurines using neutron, laser, and X-ray techniques, Microchem. J., 2016, 124, 765–774, DOI:10.1016/j.microc.2015.10.030.
- S. Siano and J. Agresti, Archaeometallurgical characterisation of Donatello's Florentine copper alloy masterpieces using portable laser-induced plasma spectroscopy and traditional techniques, Stud. Conserv., 2015, 60(suppl. 1), S106–S119, DOI:10.1179/0039363015z.000000000215.
- L. Bartoli, J. Agresti, M. Mascalchi, A. Mencaglia, I. Cacciari and S. Siano, Combined elemental and microstructural analysis of genuine and fake copper-alloy coins, Quantum Electron., 2011, 41(7), 663–668, DOI:10.1070/qe2011v041n07abeh014523.
- A. De Giacomo, M. Dell'Aglio, O. De Pascale, R. Gaudiuso, A. Santagata and R. Teghil, Laser Induced Breakdown Spectroscopy methodology for the analysis of copper-based-alloys used in ancient artworks, Spectrochim. Acta, Part B, 2008, 63(5), 585–590, DOI:10.1016/j.sab.2008.03.006.
- M. Corsi, G. Cristoforetti, V. Palleschi, A. Salvetti and E. Tognoni, A fast and accurate method for the determination of precious alloys caratage by Laser Induced Plasma Spectroscopy, Eur. Phys. J. D, 2001, 13, 3, DOI:10.1007/s100530170255.
- A. Ciucci, M. Corsi, V. Palleschi, S. Rastelli, A. Salvetti and E. Tognoni, New procedure for quantitative elemental analysis by laser-induced plasma spectroscopy, Appl. Spectrosc., 1999, 53, 8, DOI:10.1366/0003702991947612.
- L. Fornarini, R. Fantoni, F. Colao, A. Santagata, R. Teghil, A. Elhassan and M. A. Harith, Theoretical Modeling of Laser Ablation of Quaternary Bronze Alloys: Case Studies Comparing Femtosecond and Nanosecond LIBS Experimental Data, J. Phys. Chem. A, 2009, 113(52), 14364–14374, DOI:10.1021/jp903279h.
- S. Almaviva, R. Fantoni, L. Caneve, F. Colao, L. Fornarini, A. Santagata and R. Teghil, Use of ns and fs pulse excitation in laser-induced breakdown spectroscopy to improve its analytical performances: A case study on quaternary bronze alloys, Spectrochim. Acta, Part B, 2014, 99, 185–192, DOI:10.1016/j.sab.2014.07.009.
- R. Gaudiuso, M. Dell'Aglio, O. De Pascale, A. Santagata and A. De Giacomo, Laser-induced plasma analysis of copper alloys based on Local Thermodynamic Equilibrium: An alternative approach to plasma temperature determination and archeometric applications, Spectrochim. Acta, Part B, 2012, 74–75, 38–45, DOI:10.1016/j.sab.2012.06.034.
- E. Grifoni, S. Legnaioli, G. Lorenzetti, S. Pagnotta, F. Poggialini and V. Palleschi, From Calibration-Free to Fundamental Parameters Analysis: A comparison of three recently proposed approaches, Spectrochim. Acta, Part B, 2016, 124, 40–46, DOI:10.1016/j.sab.2016.08.022.
- E. D'Andrea, S. Pagnotta, E. Grifoni, G. Lorenzetti, S. Legnaioli, V. Palleschi and B. Lazzerini, An artificial neural network approach to laser-induced breakdown spectroscopy quantitative analysis, Spectrochim. Acta, Part B, 2014, 99, 52–58, DOI:10.1016/j.sab.2014.06.012.
-
E. D'Andrea, B. Lazzerini, V. Palleschi and S. Pagnotta, Determining the composition of bronze alloys by means of high-dimensional feature selection and Artificial Neural Networks, in Conference Record – IEEE Instrumentation and Measurement Technology Conference, 2015, DOI:10.1109/I2MTC.2015.7151598.
-
E. D'Andrea, B. Lazzerini and V. Palleschi, Combining multiple neural networks to predict bronze alloy elemental composition, 2016, DOI:10.1007/978-3-319-33747-0_34.
-
G. Lorenzetti, E. Grifoni, S. Legnaioli, S. Pagnotta and V. Palleschi, Analysis of the alloy of the Pisa Griffin, in The Pisa Griffin and the Mari-Cha Lion, ed. A. Contadini, Pacini Editore, Pisa, 2018, pp. 105–112 Search PubMed.
- S. Pagnotta, E. Grifoni, S. Legnaioli, M. Lezzerini, G. Lorenzetti and V. Palleschi, Comparison of brass alloys composition by laser-induced breakdown spectroscopy and self-organizing maps, Spectrochim. Acta, Part B, 2015, 103–104, 70–75, DOI:10.1016/j.sab.2014.11.008.
- J. Agresti and S. Siano, Depth-dependent calibration for quantitative elemental depth profiling of copper alloys using laser-induced plasma spectroscopy, Appl. Phys. A: Mater. Sci. Process., 2014, 117(1), 217–221, DOI:10.1007/s00339-014-8363-x.
- A. Petrakiev, A. Samov and G. Dimitrov, Application of LMAl Zeiss laser microspectral analyser to identification pigments in old multilayer wall paintings, Jena Rev., 1971, 16(4), 251 Search PubMed.
- A. Roy, The Laser Microspectral Analysis of Paint, Natl. Gallery Tech. Bull., 1979, 3, 43–50 Search PubMed.
-
M. Corsi, G. Cristoforetti, V. Palleschi, A. Salvetti and E. Tognoni, Surface Compositional Mapping of Pigments on a Roman Fresco by CF-LIBS, in Proceedings of the first international conference on Laser-Induced Breakdown and Applications, Tirrenia (Italy), October, 2000, vol. 8 to 12, p. 74 Search PubMed.
- D. Anglos, S. Couris and C. Fotakis, Laser Diagnostics of Painted Artworks: Laser-Induced Breakdown Spectroscopy in Pigment Identification, Appl. Spectrosc., 1997, 51(7), 1025–1030, DOI:10.1366/0003702971941421.
- I. Borgia, L. M. F. Burgio, M. Corsi, R. Fantoni, V. Palleschi, A. Salvetti, M. C. Squarcialupi and E. Tognoni, Self-calibrated quantitative elemental analysis by laser-induced plasma spectroscopy: Application to pigment analysis, J. Cult. Herit., 2000, 1, 2, DOI:10.1016/S1296-2074(00)00174-6.
- M. Bicchieri, M. Nardone, P. A. Russo, A. Sodo, M. Corsi, G. Cristoforetti, V. Palleschi, A. Salvetti and E. Tognoni, Characterization of azurite and lazurite based pigments by laser induced breakdown spectroscopy and micro-Raman spectroscopy, Spectrochim. Acta, Part B, 2001, 56, 6, DOI:10.1016/s0584-8547(01)00228-2.
- C. Henry, Taking a closer look at art, Chem. Eng. News, 2000, 78(23), 74 CrossRef.
- A. Brysbaert, K. Melessanaki and D. Anglos, Pigment analysis in Bronze Age Aegean and Eastern Mediterranean painted plaster by laser-induced breakdown spectroscopy (LIBS), J. Archaeol. Sci., 2006, 33(8), 1095–1104, DOI:10.1016/j.jas.2005.11.016.
- B. A. Ali, D. Bulajic, M. Corsi, G. Cristoforetti, S. Legnaioli, L. Masotti, V. Palleschi, A. Salvetti and E. Tognoni, µ-LIBS/µ-raman spectroscopic analysis of pigments in a roman fresco, Proc. SPIE-Int. Soc. Opt. Eng., 2001, 4402, 25–31, DOI:10.1117/12.445670.
- L. Caneve, A. Diamanti, F. Grimaldi, G. Palleschi, V. Spizzichino and F. Valentini, Analysis of fresco by laser induced breakdown spectroscopy, Spectrochim. Acta, Part B, 2010, 65(8), 702–706, DOI:10.1016/j.sab.2010.05.003.
- K. Şerifaki, H. Böke, Ş. Yalçin and B. Ipekoǧlu, Characterization of materials used in the execution of historic oil paintings by XRD, SEM-EDS, TGA and LIBS analysis, Mater. Charact., 2009, 60(4), 303–311, DOI:10.1016/j.matchar.2008.09.016.
- J. Striber, R. Radvan and L.-M. Angheluta, Laser spectroscopy methods for an 18th century grisaille painting investigation, J. Optoelectron. Adv. Mater., 2009, 11(11), 1815–1820 CAS.
- C. Lofrumento, M. Ricci, L. Bachechi, D. De Feo and E. M. Castellucci, The first spectroscopic analysis of Ethiopian prehistoric rock painting, J. Raman Spectrosc., 2012, 43(6), 809–816, DOI:10.1002/jrs.3096.
- L. Angeli, C. Arias, G. Cristoforetti, C. Fabbri, S. Legnaioli, V. Palleschi, G. Radi, A. Salvetti and E. Tognoni, Laser Chem., 2006, 2006, 61607, DOI:10.1155/2006/61607.
- L. Qu, X. Zhang, H. Duan, R. Zhang, G. Li and Y. Lei, The application of LIBS and other techniques on Chinese low temperature glaze, MRS Adv., 2017, 2081–2094, DOI:10.1557/adv.2017.85.
- S. Grégoire, M. Boudinet, F. Pelascini, F. Surma, V. Detalle and Y. Holl, Laser-induced breakdown spectroscopy for polymer identification, Anal. Bioanal. Chem., 2011, 400(10), 3331–3340, DOI:10.1007/s00216-011-4898-2.
- X. Bai, D. Syvilay, N. Wilkie-Chancellier, A. Texier, L. Martinez, S. Serfaty, D. Martos-Levif and V. Detalle, Influence of ns-laser wavelength in laser-induced breakdown spectroscopy for discrimination of painting techniques, Spectrochim. Acta, Part B, 2017, 134, 81–90, DOI:10.1016/j.sab.2017.06.008.
- N. F. C. Mendes, I. Osticioli, J. Striova, A. Sansonetti, M. Becucci and E. Castellucci, Versatile pulsed laser setup for depth profiling analysis of multilayered samples in the field of cultural heritage, J. Mol. Struct., 2009, 924–926, 420–426, DOI:10.1016/j.molstruc.2009.01.047.
- M. M. Amaral, M. P. Raele, A. Z. De Freitas, G. S. Zahn, R. E. Samad, N. D. Vieira Jr and L. V. G. Tarelho, Laser Induced Breakdown Spectroscopy (LIBS) applied to stratigrafic elemental analysis and Optical Coherence Tomography (OCT) to damage determination of cultural heritage Brazilian coins, Proc. SPIE-Int. Soc. Opt. Eng., 2009, 7391, 73910I, DOI:10.1117/12.827724.
- E. A. Kaszewska, M. Sylwestrzak, J. Marczak, W. Skrzeczanowski, M. Iwanicka, E. Szmit-Naud, D. Anglos and P. Targowski, Depth-Resolved Multilayer Pigment Identification in Paintings: Combined Use of Laser-Induced Breakdown Spectroscopy (LIBS) and Optical Coherence Tomography (OCT), Appl. Spectrosc., 2013, 67(8), 960–972, DOI:10.1366/12-06703.
- R. Bruder, D. L'Hermite, A. Semerok, L. Salmon and V. Detalle, Near-crater discoloration of white lead in wall paintings during laser induced breakdown spectroscopy analysis, Spectrochim. Acta, Part B, 2007, 62(12), 1590–1596, DOI:10.1016/j.sab.2007.10.031.
- S. Aze, J.-M. Vallet, A. Baronnet and O. Grauby, The fading of red lead pigment in wall paintings: tracking the physico-chemical transformations by means of complementary micro-analysis techniques, Eur. J. Mineral., 2006, 18(6), 835–843, DOI:10.1127/0935-1221/2006/0018-0835.
- B. N. Chichkov, C. Momma, S. Nolte, F. von Alvensleben and A. Tünnermann, Femtosecond, picosecond and nanosecond laser ablation of solids, Appl. Phys. A: Mater. Sci. Process., 1996, 63(2), 109–115, DOI:10.1007/bf01567637.
- I. Osticioli, M. Wolf and D. Anglos, An Optimization of Parameters for Application of a Laser-Induced Breakdown Spectroscopy Microprobe for the Analysis of Works of Art, Appl. Spectrosc., 2008, 62(11), 1242–1249, DOI:10.1366/000370208786401572.
- M. F. Alberghina, R. Barraco, M. Brai, D. Fontana and L. Tranchina, LIBS and XRF analysis for a stratigraphic study of pictorial multilayer surfaces, Period. Mineral., 2015, 84(3A), 569–589, DOI:10.2451/2015pm0024.
- P. Westlake, P. Siozos, A. Philippidis, C. Apostolaki, B. Derham, A. Terlixi, V. Perdikatsis, R. Jones and D. Anglos, Studying pigments on painted plaster in Minoan, Roman and Early Byzantine Crete. A multi-analytical technique approach, Anal. Bioanal. Chem., 2012, 402(4), 1413–1432 CrossRef CAS PubMed.
- R. Bruder, V. Detalle and C. Coupry, An example of the complementarity of laser-induced breakdown spectroscopy and Raman microscopy for wall painting pigments analysis, J. Raman Spectrosc., 2007, 38(7), 909–915, DOI:10.1002/jrs.1685.
- M. P. Mateo, T. Ctvrtnickova and G. Nicolas, Characterization of pigments used in painting by means of laser-induced plasma and attenuated total reflectance FTIR spectroscopy, Appl. Surf. Sci., 2009, 255(10), 5172–5176, DOI:10.1016/j.apsusc.2008.08.040.
- B. Campanella, E. Grifoni, M. Hidalgo, S. Legnaioli, G. Lorenzetti, S. Pagnotta, F. Poggialini, L. Ripoll-Seguer and V. Palleschi, Multi-technique characterization of madder lakes: A comparison between non- and micro-destructive methods, J. Cult. Herit., 2018 DOI:10.1016/j.culher.2018.01.013.
- S. Duchêne, V. Detalle, R. Bruder and J. Sirven, Chemometrics and Laser Induced Breakdown Spectroscopy (LIBS) Analyses for Identification of Wall Paintings Pigments, Curr. Anal. Chem., 2010, 6(1), 60–65, DOI:10.2174/157341110790069600.
- D. Syvilay, N. Wilkie-Chancellier, B. Trichereau, A. Texier, L. Martinez, S. Serfaty and V. Detalle, Evaluation of the standard normal variate method for Laser-Induced Breakdown Spectroscopy data treatment applied to the discrimination of painting layers, Spectrochim. Acta, Part B, 2015, 114, 38–45, DOI:10.1016/j.sab.2015.09.022.
- F. D. S. L. Borba, J. Cortez, V. K. Asfora, C. Pasquini, M. F. Pimentel, A.-M. Pessis and H. J. Khoury, Multivariate treatment of libs data of prehistoric paintings, J. Braz. Chem. Soc., 2012, 23(5), 958–965, DOI:10.1590/s0103-50532012000500023.
- O. Syta, B. Wagner, E. Bulska, D. Zielińska, G. Z. Żukowska, J. Gonzalez and R. Russo, Elemental imaging of heterogeneous inorganic archaeological samples by means of simultaneous laser induced breakdown spectroscopy and laser ablation inductively coupled plasma mass spectrometry measurements, Talanta, 2018, 179, 784–791, DOI:10.1016/j.talanta.2017.12.011.
- Y. Yoon, T. Kim, M. Yang, K. Lee and G. Lee, Quantitative analysis of pottery glaze by laser induced breakdown spectroscopy, Microchem. J., 2001, 68(2–3), 251–256, DOI:10.1016/s0026-265x(00)00155-7.
- F. Colao, R. Fantoni, V. Lazic and V. Spizzichino, Laser-induced breakdown spectroscopy for semi-quantitative and quantitative analyses of artworks—application on multi-layered ceramics and copper based alloys, Spectrochim. Acta, Part B, 2002, 57(7), 1219–1234, DOI:10.1016/s0584-8547(02)00054-x.
- V. Lazic, F. Colao, R. Fantoni, A. Palucci, V. Spizzichino, I. Borgia, B. G. Brunetti and A. Sgamellotti, Characterisation of lustre and pigment composition in ancient pottery by laser induced fluorescence and breakdown spectroscopy, J. Cult. Herit., 2003, 4(Suppl. 1), 303–308 CrossRef.
- K. Melessanaki, S. C. Ferrence, P. P. Betancourt and D. Anglos, Application of LIBS in the analysis of archaeological objects, Proc. SPIE-Int. Soc. Opt. Eng., 2003, 79–80, DOI:10.1117/12.524915.
- K. Melessanaki, M. Mateo, S. C. Ferrence, P. P. Betancourt and D. Anglos, The application of LIBS for the analysis of archaeological ceramic and metal artifacts, Appl. Surf. Sci., 2002, 197–198, 156–163, DOI:10.1016/s0169-4332(02)00459-2.
- A. J. López, G. Nicolás, M. P. Mateo, V. Piñón, M. J. Tobar and A. Ramil, Compositional analysis of Hispanic Terra Sigillata by laser-induced breakdown spectroscopy, Spectrochim. Acta, Part B, 2005, 60(7–8), 1149–1154, DOI:10.1016/j.sab.2005.05.009.
- A. Erdem, A. Çilingiroğlu, A. Giakoumaki, M. Castanys, E. Kartsonaki, C. Fotakis and D. Anglos, Characterization of Iron age pottery from eastern Turkey by laser- induced breakdown spectroscopy (LIBS), J. Archaeol. Sci., 2008, 35(9), 2486–2494, DOI:10.1016/j.jas.2008.03.019.
- B. G. Oztoprak, M. A. Sinmaz and F. Tülek, Composition analysis of medieval ceramics by laser-induced breakdown spectroscopy (LIBS), Appl. Phys. A: Mater. Sci. Process., 2016, 122, 5, DOI:10.1007/s00339-016-0085-9.
- E. Xenogiannopoulou, C. Andreouli and C. J. Stournaras, Application of LIBS Technique for the Compositional Analysis of an Attic Black Pottery, J. Nano Res., 2009, 8, 61–70, DOI:10.4028/www.scientific.net/jnanor.8.61.
- R.-J. Lasheras, J. Anzano, C. Bello-Gálvez, M. Escudero and J. Cáceres, Quantitative Analysis of Roman Archeological Ceramics by Laser-Induced Breakdown Spectroscopy, Anal. Lett., 2017, 50(8), 1325–1334, DOI:10.1080/00032719.2016.1217000.
- A. Khedr, O. Abdel-Kareem, S. H. Elnabi and M. A. Harith, Compositional analysis of ceramic glaze by laser induced breakdown spectroscopy and energy dispersive X-ray, AIP Conf. Proc., 2011, 87–92, DOI:10.1063/1.3631815.
- A. Khedr and M. A. Harith, In-depth micro-spectrochemical analysis of archaeological Egyptian pottery shards, Appl. Phys. A: Mater. Sci. Process., 2013, 113(4), 835–842, DOI:10.1007/s00339-013-7829-6.
- S. S. Rai, N. K. Rai, A. K. Rai and U. C. Chattopadhyaya, Rare earth elements analysis in archaeological pottery by laser induced breakdown spectroscopy, Spectrosc. Lett., 2016, 49(2), 57–62 CrossRef CAS.
- P. Singh, E. Mal, A. Khare and S. Sharma, A study of archaeological pottery of Northeast India using laser induced breakdown spectroscopy (LIBS), J. Cult. Herit., 2018, 33, 71–82, DOI:10.1016/j.culher.2018.03.011.
- S. Legnaioli, F. A. Garcia, A. Andreotti, E. Bramanti, D. D. Pace, S. Formola, G. Lorenzetti, M. Martini, L. Pardini, E. Ribechini, E. Sibilia, R. Spiniello and V. Palleschi, Multi-technique study of a ceramic archaeological artifact and its content, Spectrochim. Acta, Part A, 2013, 100, 144–148, DOI:10.1016/j.saa.2012.04.009.
- S. Pagnotta, S. Legnaioli, B. Campanella, E. Grifoni, M. Lezzerini, G. Lorenzetti, V. Palleschi, F. Poggialini and S. Raneri, Micro-chemical evaluation of ancient potsherds by µ-LIBS scanning on thin section negatives, Mediterranean Archaeology and Archaeometry, 2018, 18(5), 171–178 Search PubMed.
- S. Pagnotta, M. Lezzerini, L. Ripoll-Seguer, M. Hidalgo, E. Grifoni, S. Legnaioli, G. Lorenzetti, F. Poggialini and V. Palleschi, Micro-Laser-Induced Breakdown Spectroscopy (Micro-LIBS) Study on Ancient Roman Mortars, Appl. Spectrosc., 2017, 71(4), 721–727, DOI:10.1177/0003702817695289.
- S. Pagnotta, M. Lezzerini, B. Campanella, G. Gallello, E. Grifoni, S. Legnaioli, G. Lorenzetti, F. Poggialini, S. Raneri, A. Safi and V. Palleschi, Fast quantitative elemental mapping of highly inhomogeneous materials by micro-Laser-Induced Breakdown Spectroscopy, Spectrochim. Acta, Part B, 2018, 146, 9–15, DOI:10.1016/j.sab.2018.04.018.
- P. V. Maravelaki, V. Zafiropulos, V. Kilikoglou, M. Kalaitzaki and C. Fotakis, Laser-induced breakdown spectroscopy as a diagnostic technique for the laser cleaning of marble, Spectrochim. Acta, Part B, 1997, 52(1), 41–53, DOI:10.1016/s0584-8547(96)01573-x.
- P. Maravelaki-Kalaitzaki, V. Zafiropulos and C. Fotakis, Excimer laser cleaning of encrustation on Pentelic marble: procedure and evaluation of the effects, Appl. Surf. Sci., 1999, 148(1–2), 92–104, DOI:10.1016/s0169-4332(99)00125-7.
- P. Maravelaki-Kalaitzaki, D. Anglos, V. Kilikoglou and V. Zafiropulos, Compositional characterization of encrustation on marble with laser induced breakdown spectroscopy, Spectrochim. Acta, Part B, 2001, 56(6), 887–903 CrossRef.
-
K. Dickmann, S. Klein and V. Zafiropulos, Laser cleaning of marble: discoloration effects using various Nd:YAG laser wavelengths (ω, 2ω, 3ω), 2001, p. 54, DOI:10.1117/12.445675.
- S. Klein, F. Fekrsanati, J. Hildenhagen, K. Dickmann, H. Uphoff, Y. Marakis and V. Zafiropulos, Discoloration of marble during laser cleaning by Nd:YAG laser wavelengths, Appl. Surf. Sci., 2001, 171(3–4), 242–251, DOI:10.1016/s0169-4332(00)00706-6.
- J. Hildenhagen and K. Dickmann, Low-cost sensor system for online monitoring during laser cleaning, J. Cult. Herit., 2003, 4, 343–346, DOI:10.1016/s1296-2074(02)01145-7.
- F. Colao, R. Fantoni, V. Lazic, A. Morone, A. Santagata and A. Giardini, LIBS used as a diagnostic tool during the laser cleaning of ancient marble from Mediterranean areas, Appl. Phys. A: Mater. Sci. Process., 2004, 79(2), 213–219 CrossRef CAS.
- A. Khedr, V. Papadakis, P. Pouli, D. Anglos and M. A. Harith, The potential use of plume imaging for real-time monitoring of laser ablation cleaning of stonework, Appl. Phys. B: Lasers Opt., 2011, 105(2), 485–492, DOI:10.1007/s00340-011-4492-5.
- V. Lazic, R. Fantoni, F. Colao, A. Santagata, A. Morone and V. Spizzichino, Quantitative laser induced breakdown spectroscopy analysis of ancient marbles and corrections for the variability of plasma parameters and of ablation rate, J. Anal. At. Spectrom., 2004, 19(4), 429, 10.1039/b315606k.
- A. Bakry, Spectroscopic analysis of marble samples using laser induced breakdown spectroscopy, Asian J. Spectrosc., 2007, 11(1), 51–59 CAS.
- S. Mahmood, S. A. Abbasi, S. Jabeen and M. A. Baig, Laser-induced breakdown spectroscopic studies of marbles, J. Quant. Spectrosc. Radiat. Transfer, 2010, 111(5), 689–695, DOI:10.1016/j.jqsrt.2009.11.012.
- M. Fahad and M. Abrar, Laser-induced breakdown spectroscopic studies of calcite (CaCO3) marble using the fundamental (1064
nm) and second (532
nm) harmonic of a Nd:YAG laser, Laser Phys., 2018, 28(8), 085701, DOI:10.1088/1555-6611/aac5cd.
- S. Columbu, S. Carboni, S. Pagnotta, M. Lezzerini, S. Raneri, S. Legnaioli, V. Palleschi and A. Usai, Laser-Induced Breakdown Spectroscopy analysis of the limestone Nuragic statues from Mont'e Prama site (Sardinia, Italy), Spectrochim. Acta, Part B, 2018, 149, 62–70, DOI:10.1016/j.sab.2018.07.011.
- E. Grifoni, S. Legnaioli, G. Lorenzetti, S. Pagnotta and V. Palleschi, Application of Graph Theory to unsupervised classification of materials by Laser-Induced Breakdown Spectroscopy, Spectrochim. Acta, Part B, 2016, 118, 40–44, DOI:10.1016/j.sab.2016.02.003.
- V. Lazic, F. Colao, R. Fantoni and V. Spizzicchino, Recognition of archeological materials underwater by laser induced breakdown spectroscopy, Spectrochim. Acta, Part B, 2005, 60(7–8), 1014–1024, DOI:10.1016/j.sab.2005.06.014.
- S. Guirado, F. J. Fortes and J. Javier Laserna, Elemental analysis of materials in an underwater archeological shipwreck using a novel remote laser-induced breakdown spectroscopy system, Talanta, 2015, 137, 182–188, DOI:10.1016/j.talanta.2015.01.033.
- F. J. Fortes, J. Cuñat, L. M. Cabalín and J. J. Laserna,
In Situ Analytical Assessment and Chemical Imaging of Historical Buildings Using a Man-Portable Laser System, Appl. Spectrosc., 2007, 61(5), 558–564, DOI:10.1366/000370207780807722.
- M. Brai, G. Gennaro, T. Schillaci and L. Tranchina, Double pulse laser induced breakdown spectroscopy applied to natural and artificial materials from cultural heritages. A comparison with micro-X-ray fluorescence analysis, Spectrochim. Acta, Part B, 2009, 64(10), 1119–1127, DOI:10.1016/j.sab.2009.07.027.
- S. Hemeda, Laser induced breakdown spectroscopy and other analytical techniques applied on construction materials at Kom El-Dikka, Alexandria, Egypt, Mediterranean Archaeology and Archaeometry, 2013, 13(2), 103–119 Search PubMed.
- S. Raneri, S. Pagnotta, M. Lezzerini, S. Legnaioli, V. Palleschi, S. Columbu, N. Francesco Neri and P. Mazzoleni, Examining the reactivity of volcanic ash in ancient mortars by using a micro-chemical approach, Mediterranean Archaeology and Archaeometry, 2018, 18(5), 147–157 Search PubMed.
- G. S. Senesi, B. Campanella, E. Grifoni, S. Legnaioli, G. Lorenzetti, S. Pagnotta, F. Poggialini, V. Palleschi and O. De Pascale, Elemental and mineralogical imaging of a weathered limestone rock by double-pulse micro-Laser-Induced Breakdown Spectroscopy, Spectrochim. Acta, Part B, 2018, 143, 91–97, DOI:10.1016/j.sab.2018.02.018.
- G. S. Senesi, D. Manzini and O. De Pascale, Application of a laser-induced breakdown spectroscopy handheld instrument to the diagnostic analysis of stone monuments, Appl. Geochem., 2018, 96, 87–91, DOI:10.1016/j.apgeochem.2018.06.008.
- E. Tognoni, G. Cristoforetti, S. Legnaioli and V. Palleschi, Calibration-Free Laser-Induced Breakdown Spectroscopy: State of the art, Spectrochim. Acta, Part B, 2010, 65, 1, DOI:10.1016/j.sab.2009.11.006.
-
O. Samek, M. Liska, J. Kaiser, V. Krzyzanek, D. C. S. Beddows, A. Belenkevitch, G. W. Morris and H. H. Telle, Laser ablation for mineral analysis in the human body: integration of LIFS with LIBS, 1999, p. 263. DOI:10.1117/12.336941.
-
C. N. Rinke-Kneapler and M. E. Sigman, Applications of laser spectroscopy in forensic science, in Laser Spectroscopy for Sensing, Elsevier, 2014, pp. 461–495. DOI:10.1533/9780857098733.3.461.
- F. C. Alvira, F. Ramirez Rozzi and G. M. Bilmes, Laser-Induced Breakdown Spectroscopy Microanalysis of Trace Elements in Homo Sapiens Teeth, Appl. Spectrosc., 2010, 64(3), 313–319, DOI:10.1366/000370210790918328.
- M. Galiová, J. Kaiser, F. J. Fortes, K. Novotný, R. Malina, L. Prokeš, A. Hrdlička, T. Vaculovič, M. Nývltová Fišáková, J. Svoboda, V. Kanický and J. J. Laserna, Multielemental analysis of prehistoric animal teeth by laser-induced breakdown spectroscopy and laser ablation inductively coupled plasma mass spectrometry, Appl. Opt., 2010, 49(13), C191, DOI:10.1364/ao.49.00c191.
- D. A. Rusak, R. M. Marsico and B. L. Taroli, Using laser-induced breakdown spectroscopy to assess preservation quality of archaeological bones by measurement of calcium-to-fluorine ratios, Appl. Spectrosc., 2011, 65(10), 1193–1196, DOI:10.1366/11-06364.
-
L. Garofano, M. Borrini, L. Marchesini, G. Carelli, R. Grassi, F. Sorrentino, F. Francesconi, M. Francesconi, P. Marsili, S. Legnaioli, G. Lorenzetti, L. Pardini and V. Palleschi, Analysis of Archaeological Human Tissues Using Forensic Science Techniques: The Strange Case of the Vampire Lady, in Proceedings of the 5th International Congress on Science and Technology for the Safeguard of Cultural Heritage in the Mediterranean Basin, 22–25 November 2011, Istanbul (Turkey), p. 264 Search PubMed.
- M. A. Kasem, J. J. Gonzalez, R. E. Russo and M. A. Harith, Effect of the wavelength on laser induced breakdown spectrometric analysis of archaeological bone, Spectrochim. Acta, Part B, 2014, 101, 26–31, DOI:10.1016/j.sab.2014.07.010.
- G. D. Al-Khafif and R. El-Banna, Reconstructing Ancient Egyptian Diet through Bone Elemental Analysis Using LIBS (Qubbet el Hawa Cemetery), BioMed Res. Int., 2015, 1–7, DOI:10.1155/2015/281056.
- M. Bicchieri, S. Ronconi, F. P. Romano, L. Pappalardo, M. Corsi, G. Cristoforetti, S. Legnaioli, V. Palleschi, A. Salvetti and E. Tognoni, Study of foxing stains on paper by chemical methods, infrared spectroscopy, micro-X-ray fluorescence spectrometry and laser induced breakdown spectroscopy, Spectrochim. Acta, Part B, 2002, 57, 7, DOI:10.1016/s0584-8547(02)00056-3.
- T. Jie Lie, K. H. Kurniawan, D. P. Kurniawan, M. Pardede, M. Margaretha Suliyanti, A. Khumaeni, S. A. Natiq, S. N. Abdulmadjid, Y. I. Lee, K. Kagawa, N. Idris and M. O. Tjia, Elemental analysis of bead samples using a laser-induced plasma at low pressure, Spectrochim. Acta, Part B, 2006, 61(1), 104–112, DOI:10.1016/j.sab.2005.12.007.
- K. Müller and H. Stege, Evaluation of the analytical potential of laser-induced breakdown spectrometry (LIBS) for the analysis of historical glasses, Archaeometry, 2003, 45, 421–433 CrossRef.
- N. Carmona, M. Oujja, E. Rebollar, H. Römich and M. Castillejo, Analysis of corroded glasses by laser induced breakdown spectroscopy, Spectrochim. Acta, Part B, 2005, 60(7–8), 1155–1162, DOI:10.1016/j.sab.2005.05.016.
-
K. Szelagowska, M. Szymoński, F. Krok, M. Walczak, P. Karaszkiewicz and J. S. Prauzner-Bechcicki. 2008 Comparative study of historic stained glass by LIBS and SEM/EDX. in 7th International Conference on Lasers in the Conservation of Artworks, LACONA 7, Madrid, Spain, 17 September 2007 through 21 September 2007, pp. 141–145 Search PubMed.
- N. J. McMillan, C. E. McManus, R. S. Harmon, F. C. De Lucia and A. W. Miziolek, Laser-induced breakdown spectroscopy analysis of complex silicate minerals—beryl, Anal. Bioanal. Chem., 2006, 385(2), 263–271, DOI:10.1007/s00216-006-0374-9.
- C. E. McManus, N. J. McMillan, R. S. Harmon, R. C. Whitmore, F. C. De Lucia Jr and A. W. Miziolek, Use of laser induced breakdown spectroscopy in the determination of gem provenance: beryls, Appl. Opt., 2008, 47(31), G72, DOI:10.1364/ao.47.000g72.
- G. Agrosì, G. Tempesta, E. Scandale, S. Legnaioli, G. Lorenzetti, S. Pagnotta, V. Palleschi, A. Mangone and M. Lezzerini, Application of Laser Induced Breakdown Spectroscopy to the identification of emeralds from different synthetic processes, Spectrochim. Acta, Part B, 2014, 102, 48–51, DOI:10.1016/j.sab.2014.10.012.
- G. Tempesta and G. Agrosì, Standardless, minimally destructive chemical analysis of red beryls by means of Laser Induced Breakdown Spectroscopy, Eur. J. Mineral., 2016, 28(3), 571–580, DOI:10.1127/ejm/2016/0028-2529.
- A. De Giacomo, C. Koral, G. Valenza, R. Gaudiuso and M. Dellaglio, Nanoparticle Enhanced Laser-Induced Breakdown Spectroscopy for Microdrop Analysis at subppm Level, Anal. Chem., 2016, 88, 10, DOI:10.1021/acs.analchem.6b00324.
- C. E. McManus, J. Dowe and N. J. McMillan, Quantagenetics® analysis of laser-induced breakdown spectroscopic data: Rapid and accurate authentication of materials, Spectrochim. Acta, Part B, 2018, 145, 79–85, DOI:10.1016/j.sab.2018.04.010.
- A. Giakoumaki, K. Melessanaki and D. Anglos, Laser-induced breakdown spectroscopy (LIBS) in archaeological science—applications and prospects, Anal. Bioanal. Chem., 2007, 387(3), 749–760, DOI:10.1007/s00216-006-0908-1.
-
D. Anglos and V. Detalle, Cultural heritage applications of LIBS, in Laser-Induced Breakdown Spectroscopy, Springer, 2014, pp. 531–554 Search PubMed.
-
C. Fotakis, D. Anglos, V. Zafiropulos, S. Georgiou and V. Tornari, Lasers in the preservation of cultural heritage: principles and applications, CRC Press, 2006 Search PubMed.
- G. B. Courreges-Lacoste, B. Ahlers and F. Rull Pérez, Combined Raman spectrometer/laser-induced breakdown spectrometer for the next ESA mission to Mars, Spectrochim. Acta, Part A, 2007, 68(4), 1023–1028 CrossRef PubMed.
- M. Hoehse, D. Mory, S. Florek, F. Weritz, I. Gornushkin and U. Panne, A combined laser-induced breakdown and Raman spectroscopy Echelle system for elemental and molecular microanalysis, Spectrochim. Acta, Part B, 2009, 64(11–12), 1219–1227 CrossRef.
- F. Matroodi and S. H. Tavassoli, Experimental investigation on concurrent laser-induced breakdown spectroscopy Raman spectroscopy, Appl. Opt., 2015, 54(3), 400–407 CrossRef CAS.
- J. Moros, J. A. Lorenzo, P. Lucena, L. Miguel Tobaria and J. J. Laserna, Simultaneous Raman Spectroscopy-Laser-Induced Breakdown Spectroscopy for instant standoff analysis of explosives using a mobile integrated sensor platform, Anal. Chem., 2010, 82(4), 1389–1400 CrossRef CAS PubMed.
- A. Giakoumaki, I. Osticioli and D. Anglos, Spectroscopic analysis using a hybrid LIBS-Raman system, Appl. Phys. A: Mater. Sci. Process., 2006, 83(4), 537–541, DOI:10.1007/s00339-006-3541-0.
- I. Osticioli, N. F. C. Mendes, S. Porcinai, A. Cagnini and E. Castellucci, Spectroscopic analysis of works of art using a single LIBS and pulsed Raman setup, Anal. Bioanal. Chem., 2009, 394(4), 1033–1041, DOI:10.1007/s00216-009-2653-8.
- C. Brian Dreyer, G. S. Mungas, P. Thanh and J. G. Radziszewski, Study of sub-mJ-excited laser-induced plasma combined with Raman spectroscopy under Mars atmosphere-simulated conditions, Spectrochim. Acta, Part B, 2007, 62(12), 1448–1459 CrossRef.
- S. M. Clegg, R. Wiens, A. K. Misra, S. K. Sharma, J. Lambert, S. Bender, R. Newell, K. Nowak-Lovato, S. Smrekar and M. D. Dyar, Planetary geochemical investigations using Raman and laser-induced breakdown spectroscopy, Appl. Spectrosc., 2014, 68(9), 925–936 CrossRef CAS PubMed.
- S. K. Sharma, A. K. Misra, P. G. Lucey and R. C. F. Lentz, A combined remote Raman and LIBS instrument for characterizing minerals with 532 nm laser excitation, Spectrochim. Acta, Part A, 2009, 73(3), 468–476 CrossRef PubMed.
- J. Moros, J. A. Lorenzo and J. J. Laserna, Standoff detection of explosives: critical comparison for ensuing options on Raman spectroscopy–LIBS sensor fusion, Anal. Bioanal. Chem., 2011, 400(10), 3353–3365 CrossRef CAS PubMed.
- V. N. Lednev, S. M. Pershin, P. A. Sdvizhenskii, M. Ya Grishin, A. N. Fedorov, V. V. Bukin, V. B. Oshurko and A. N. Shchegolikhin, Combining Raman and laser induced breakdown spectroscopy by double pulse lasing, Anal. Bioanal. Chem., 2018, 410(1), 277–286 CrossRef CAS PubMed.
- M. Ferretti, G. Cristoforetti, S. Legnaioli, V. Palleschi, A. Salvetti, E. Tognoni, E. Console and P. Palaia,
In situ study of the Porticello Bronzes by portable X-ray fluorescence and laser-induced breakdown spectroscopy, Spectrochim. Acta, Part B, 2007, 62(12), 1512–1518, DOI:10.1016/j.sab.2007.09.004.
-
M. Angelini, O. Colacicchi and G. Bandini, The volute krater on Hypokrateridion of the Trebeniste studies, research and restoration interventions, Bollettino di archeologia, 4, 2011, pp. 1–130, retrieved from http://www.scopus.com/inward/record.url?eid=2-s2.0-84873887660%26partnerID=40%26md5=4e6cd737c2184a9438d64bf125345cdf Search PubMed.
- L. Pardini, A. El Hassan, M. Ferretti, A. Foresta, S. Legnaioli, G. Lorenzetti, E. Nebbia, F. Catalli, M. A. Harith, D. Diaz Pace, F. Anabitarte Garcia, M. Scuotto and V. Palleschi, X-ray fluorescence and laser-induced breakdown spectroscopy analysis of Roman silver denarii, Spectrochim. Acta, Part B, 2012, 74–75, DOI:10.1016/j.sab.2012.06.016.
- M. F. Alberghina, R. Barraco, M. Brai, T. Schillaci and L. Tranchina, Comparison of LIBS and µ-XRF measurements on bronze alloys for monitoring plasma effects, J. Phys.: Conf. Ser., 2011, 275, 012017, DOI:10.1088/1742-6596/275/1/012017.
- M. F. Alberghina, R. Barraco, M. Brai, T. Schillaci and L. Tranchina, Integrated analytical methodologies for the study of corrosion processes in archaeological bronzes, Spectrochim. Acta, Part B, 2011, 66(2), 129–137, DOI:10.1016/j.sab.2010.12.010.
- M. F. Alberghina, R. Barraco, M. Brai, T. Schillaci and L. Tranchina, Double laser LIBS and micro-XRF spectroscopy applied to characterize materials coming from the Greek-Roman theater of Taormina, Proc. SPIE-Int. Soc. Opt. Eng., 2009, 7391, 11, DOI:10.1117/12.827777.
- V. Lazic, M. Vadrucci, R. Fantoni, M. Chiari, A. Mazzinghi and A. Gorghinian, Applications of laser induced breakdown spectroscopy for cultural heritage: A comparison with XRF and PIXE techniques, Spectrochim. Acta, Part B, 2018, 149, 1–14, DOI:10.1016/j.sab.2018.07.012.
-
P. Targowski, M. Iwanicka, B. J. Rouba and C. Frosinini, OCT for Examination of Artwork. Optical Coherence Tomography: Technology and Applications, 2015, pp. 2473–2495 Search PubMed.
- C. D. Gehlen, E. Wiens, R. Noll, G. Wilsch and K. Reichling, Chlorine detection
in cement with laser-induced breakdown spectroscopy in the infrared and ultraviolet spectral range, Spectrochim. Acta, Part B, 2009, 64(10), 1135–1140 CrossRef.
- I. Gobernado-Mitre, A. C. Prieto, V. Zafiropulos, Y. Spetsidou and C. Fotakis, On-line monitoring of laser cleaning of limestone by laser-induced breakdown spectroscopy and laser-induced fluorescence, Appl. Spectrosc., 1997, 51(8), 1125–1129 CrossRef CAS.
- A. Staicu, I. Apostol, A. Pascu, I. Urzica, M. L. Pascu and V. Damian, Minimal invasive control of paintings cleaning by LIBS, Opt. Laser Technol., 2016, 77, 187–192, DOI:10.1016/j.optlastec.2015.09.010.
- J. H. Scholten, J. M. Teule, V. Zafiropulos and R. M. A. Heeren, Controlled laser cleaning of painted artworks using accurate beam manipulation and on-line LIBS-detection, J. Cult. Herit., 2000, 1, S215–S220, DOI:10.1016/s1296-2074(00)00142-4.
- S. Georgiou, V. Zafiropulos, D. Anglos, C. Balas, V. Tornari and C. Fotakis, Excimer laser restoration of painted artworks: procedures, mechanisms and effects, Appl. Surf. Sci., 1998, 127, 738–745 CrossRef.
- S. Klein, T. Stratoudaki, V. Zafiropulos, J. Hildenhagen, K. Dickmann and Th. Lehmkuhl, Laser-induced breakdown spectroscopy for on-line control of laser cleaning of sandstone and stained glass, Appl. Phys. A: Mater. Sci. Process., 1999, 69(4), 441–444, DOI:10.1007/s003390051029.
- R. Salimbeni, R. Pini and S. Siano, Achievement of optimum laser cleaning in the restoration of artworks: expected improvements by on-line optical diagnostics, Spectrochim. Acta, Part B, 2001, 56(6), 877–885 CrossRef.
- G. S. Senesi, I. Carrara, G. Nicolodelli, D. M. B. P. Milori and O. De Pascale, Laser cleaning and laser-induced breakdown spectroscopy applied in removing and characterizing black crusts from limestones of Castello Svevo, Bari, Italy: a case study, Microchem. J., 2016, 124, 296–305 CrossRef CAS.
-
V. Lazic, F. Colao, R. Fantoni, L. Fiorani, A. Palucci, J. Striber, A. Santagata, A. Morone and V. Spizzicchino, Spectroscopic Monitoring of the Laser Cleaning Applied to Ancient Marbles from Mediterranean Areas, in Lasers in the Conservation of Artworks, 2005, Springer, pp. 451–456 Search PubMed.
- F. Colao, R. Fantoni, V. Lazic, L. Caneve, A. Giardini and V. Spizzichino, LIBS as a diagnostic tool during the laser cleaning of copper based alloys: experimental results, J. Anal. At. Spectrom., 2004, 19(4), 502, 10.1039/b315488b.
- V. V. Golovlev, M. J. Gresalfi, J. C. Miller, D. Anglos, K. Melesanaki, V. Zafiropulos, G. Romer and P. Messier, Laser characterization and cleaning of 19th century daguerreotypes II, J. Cult. Herit., 2003, 4, 134–139 CrossRef.
- M. P. Mateo, T. Ctvrtnickova, E. Fernandez, J. A. Ramos, A. Yanez and G. Nicolas, Laser cleaning of varnishes and contaminants on brass, Appl. Surf. Sci., 2009, 255(10), 5579–5583 CrossRef CAS.
- M. Kono, K. G. H. Baldwin, A. Wain and A. V. Rode, Treating the Untreatable in Art and Heritage Materials: Ultrafast Laser Cleaning of “Cloth-of-Gold”, Langmuir, 2015, 31(4), 1596–1604 CrossRef CAS PubMed.
-
M. Lentjes, K. Dickmann and J. Meijer, Low resolution LIBS for online-monitoring during laser cleaning based on correlation with reference spectra, in Lasers in the Conservation of Artworks, Springer, 2007, pp. 437–444 Search PubMed.
- F. J. Fortes, L. M. Cabalín and J. J. Laserna, The potential of laser-induced breakdown spectrometry for real time monitoring the laser cleaning of archaeometallurgical objects, Spectrochim. Acta, Part B, 2008, 63(10), 1191–1197 CrossRef.
- B. Juvelier, Salvaging History: Underwater Cultural Heritage and Commercial Salvage, Am. Univ. Int. Law Rev., 2016, 32, 1023 Search PubMed.
- A. De Giacomo, M. Dell'Aglio, O. De Pascale and M. Capitelli, From single pulse to double pulse ns-Laser Induced Breakdown Spectroscopy under water: Elemental analysis of aqueous solutions and submerged solid samples, Spectrochim. Acta, Part B, 2007, 62(8), 721–738, DOI:10.1016/j.sab.2007.06.008.
- V. Lazic, J. J. Laserna and S. Jovicevic, Insights in the laser induced breakdown spectroscopy signal generation underwater using dual pulse excitation — Part II: Plasma emission intensity as a function of interpulse delay, Spectrochim. Acta, Part B, 2013, 82, 50–59, DOI:10.1016/j.sab.2013.01.009.
- V. Lazic, J. J. Laserna and S. Jovicevic, Insights in the laser-induced breakdown spectroscopy signal generation underwater using dual pulse excitation—Part I: Vapor bubble, shockwaves and plasma, Spectrochim. Acta, Part B, 2013, 82, 42–49 CrossRef CAS.
- F. J. Fortes, J. Moros, P. Lucena, L. M. Cabalín and J. J. Laserna, Laser-induced breakdown spectroscopy, Anal. Chem., 2012, 85(2), 640–669 CrossRef PubMed.
- D. C. S. Beddows, O. Samek, M. Liška and H. H. Telle, Single-pulse laser-induced breakdown spectroscopy of samples submerged in water using a single-fibre light delivery system, Spectrochim. Acta, Part B, 2002, 57(9), 1461–1471 CrossRef.
- T. Sakka, H. Oguchi, S. Masai, K. Hirata, Y. H. Ogata, M. Saeki and H. Ohba, Use of a long-duration ns pulse for efficient emission of spectral lines from the laser ablation plume in water, Appl. Phys. Lett., 2006, 88(6), 61120 CrossRef.
- S. Guirado, F. J. Fortes, V. Lazic and J. J. Laserna, Chemical analysis of archeological materials in submarine environments using laser-induced breakdown spectroscopy. On-site trials in the Mediterranean Sea, Spectrochim. Acta, Part B, 2012, 74–75, 137–143, DOI:10.1016/j.sab.2012.06.032.
- S. Guirado, F. J. Fortes, L. M. Cabalín and J. J. Laserna, Effect of pulse duration in multi-pulse excitation of silicon in laser-induced breakdown spectroscopy (LIBS), Appl. Spectrosc., 2014, 68(9), 1060–1066 CrossRef CAS PubMed.
- M. López-Claros, F. Javier Fortes and J. J. Laserna, Subsea spectral identification of shipwreck objects using laser-induced breakdown spectroscopy and linear discriminant analysis, J. Cult. Herit., 2018, 29, 75–81, DOI:10.1016/j.culher.2016.12.015.
- F. J. Fortes, S. Guirado, A. Metzinger and J. J. Laserna, A study of underwater stand-off laser-induced breakdown spectroscopy for chemical analysis of objects in the deep ocean, J. Anal. At. Spectrom., 2015, 30(5), 1050–1056, 10.1039/c4ja00489b.
- R. Grassi, E. Grifoni, S. Gufoni, S. Legnaioli, G. Lorenzetti, N. Macro, L. Menichetti, S. Pagnotta, F. Poggialini, C. Schiavo and V. Palleschi, Three-dimensional compositional mapping using double-pulse micro-laser-induced breakdown spectroscopy technique, Spectrochim. Acta, Part B, 2017, 127 DOI:10.1016/j.sab.2008.06.011.
- K. Meissner, T. Lippert, A. Wokaun and D. Guenther, Analysis of trace metals in comparison of laser-induced breakdown spectroscopy with LA-ICP-MS, Thin Solid Films, 2004, 453–454, 316–322, DOI:10.1016/j.tsf.2003.11.174.
- K. Novotný, J. Kaiser, M. Galiová, V. Konečná, J. Novotný, R. Malina, M. Liška, V. Kanický and V. Otruba, Mapping of different structures on large area of granite sample using laser-ablation based analytical techniques, an exploratory study, Spectrochim. Acta, Part B, 2008, 63(10), 1139–1144, DOI:10.1016/j.sab.2008.06.011.
-
O. Kokkinaki, C. Mihesana, M. Velegrakis and D. Anglos, Comparative study of laser induced breakdown spectroscopy and mass spectrometry for the analysis of cultural heritage materials, J. Mol. Struct., 2013, 1044, 160–166, DOI:10.1016/j.molstruc.2013.01.069.
- A. De Giacomo, R. Gaudiuso, C. Koral, M. Dell'Aglio and O. De Pascale, Nanoparticle-Enhanced Laser-Induced Breakdown Spectroscopy
of Metallic Samples, Anal. Chem., 2013, 85(21), 10180–10187, DOI:10.1021/ac4016165.
- M. Dell'Aglio, R. Alrifai and A. De Giacomo, Nanoparticle Enhanced Laser Induced Breakdown Spectroscopy (NELIBS), a first review, Spectrochim. Acta, Part B, 2018, 148, 105–112, DOI:10.1016/j.sab.2018.06.008.
- A. De Giacomo, R. Gaudiuso, C. Koral, M. Dell'Aglio and O. De Pascale, Nanoparticle Enhanced Laser Induced Breakdown Spectroscopy: Effect of nanoparticles deposited on sample surface on laser ablation and plasma emission, Spectrochim. Acta, Part B, 2014, 98, 19–27, DOI:10.1016/j.sab.2014.05.010.
- C. Koral, A. De Giacomo, X. Mao, V. Zorba and R. E. Russo, Nanoparticle Enhanced Laser Induced Breakdown Spectroscopy for Improving the Detection of Molecular Bands, Spectrochim. Acta, Part B, 2016, 125, 11–17, DOI:10.1016/j.sab.2016.09.006.
- A. De Giacomo, M. Dell'Aglio, R. Gaudiuso, C. Koral and G. Valenza, Perspective on the use of nanoparticles to improve LIBS analytical performance: nanoparticle enhanced laser induced breakdown spectroscopy (NELIBS), J. Anal. At. Spectrom., 2016, 31(8), 1566–1573, 10.1039/c6ja00189k.
- C. Koral, M. Dell'Aglio, R. Gaudiuso, R. Alrifai, M. Torelli and A. De Giacomo, Nanoparticle-Enhanced Laser Induced Breakdown Spectroscopy for the noninvasive analysis of transparent samples and gemstones, Talanta, 2018, 182, 253–258, DOI:10.1016/j.talanta.2018.02.001.
- F. Poggialini, B. Campanella, S. Giannarelli, E. Grifoni, S. Legnaioli, G. Lorenzetti, S. Pagnotta, A. Safi and V. Palleschi, Green-synthetized silver nanoparticles for Nanoparticle-Enhanced Laser Induced Breakdown Spectroscopy (NELIBS) using a mobile instrument, Spectrochim. Acta, Part B, 2018, 141, 53–58, DOI:10.1016/j.sab.2018.01.005.
- M. A. Aguirre, S. Legnaioli, F. Almodóvar, M. Hidalgo, V. Palleschi and A. Canals, Elemental analysis by surface-enhanced Laser-Induced Breakdown Spectroscopy combined with liquid-liquid microextraction, Spectrochim. Acta, Part B, 2013, 79–80, DOI:10.1016/j.sab.2012.11.011.
- B. Campanella, I. Degano, E. Grifoni, S. Legnaioli, G. Lorenzetti, S. Pagnotta, F. Poggialini and V. Palleschi, Identification of inorganic dyeing mordant in textiles by surface-enhanced laser-induced breakdown spectroscopy, Microchem. J., 2018, 139 DOI:10.1016/j.microc.2018.02.034.
- Z. A. Abdel-Salam, A. H. Galmed, E. Tognoni and M. A. Harith, Estimation of calcified tissues hardness via calcium and magnesium ionic to atomic line intensity ratio in laser induced breakdown spectra, Spectrochim. Acta, Part B, 2007, 62(12), 1343–1347, DOI:10.1016/j.sab.2007.10.033.
Footnote |
† Source: SCOPUS®. |
|
This journal is © The Royal Society of Chemistry 2019 |
Click here to see how this site uses Cookies. View our privacy policy here.