Enhanced summertime PM2.5-suppression of O3 formation over the Eastern U.S. following the O3-sensitivity variations†
Received
21st March 2023
, Accepted 20th November 2023
First published on 21st November 2023
Abstract
The suppression of ozone (O3) formation due to the presence of fine particulate matter (PM2.5) has recently been highlighted for further O3 pollution controls in regions that suffer high ozone concentrations. Here we derive multiple PM2.5-suppression factors for the Eastern United States (U.S.) major cities based on a non-linear fitting of the PM2.5 and O3 relationship from the multiyear surface observations. Our results show that these PM2.5-suppression factors are increasing with time and generally follow the transition of the O3-sensitive regime towards NOx-limited chemistry. A spatial discrepancy of this suppression factor is seen currently with a higher value in the Southeastern U.S. than in the Northeastern U.S. A spatial similarity between urban regions and their downwind locations was observed for the New York City metro area. This more extensive formulation of the PM2.5-suppression factor will further improve the ability of models to help guide O3 and PM2.5 concentration pollution controls.
Environmental significance
Ozone and fine particulate matter (PM2.5) remain troublesome air pollution problems for a large number of areas, including metropolitan areas in the Northeastern U.S. like New York City. The paper's findings shed light on the interplay of these pollutants, namely the role that enhanced PM2.5 can play in suppressing ozone formation. The magnitude of ozone suppression from PM2.5 provides an additional indicator of the sensitivity of ozone formation from its VOC and NOx precursors. The PM2.5 suppression of ozone formation per unit of PM2.5 mass concentration has been increasing over the period 2004–2019 in the Northeast U.S., indicating a transition from VOC-limited to NOx-limited ozone formation sensitivity. It also provides guidance for further O3–PM2.5 studies and pollution control regulations.
|
1. Introduction
The Eastern United States (U.S.) has been marked as a region persistently suffering from the co-occurrence of summertime ozone (O3) and particulate matter (with a diameter under 2.5 μm, PM2.5) pollution during the summertime.1 However, these summertime concentrations of O3 and PM2.5 in the region's major cities have shown decreasing trends since the 1970s as a result of the implementation of emission control policies.2–6 While the region has seen reductions in both pollutants, extreme concentrations of O3 and PM2.5 (defined as the top 5% of measured values in a given year) in New York City (NYC) have shown different overall reductions with a more significant reduction for PM2.5 than O3. This can be attributed to the different reduction rates of their precursors, with the control policies targeting sulfur dioxide (SO2) and primary PM2.5, than volatile organic compounds (VOCs) and nitrogen oxides (NOx).7
Co-occurrence of summertime maximum daily 8 h average O3 (hereafter: MDA8 O3) and the daily 24 h average PM2.5 (hereafter: DA24 PM2.5 for simplification) based on ground measurements in NYC has shown a direct relationship between the pollutants,7 with a monotonically increasing near-linear relationship for low PM concentrations. A leveling-off or even decreasing relationship for high PM concentrations was observed in megacity clusters in China.7–10 This flat or declining relationship has been partly attributed to the scavenging of hydroperoxyl (HO2)/nitrate radicals (NO3) by high concentrations of PM2.5 that inhibits the photochemical production of O3,11–15 or reduced photolysis rates with PM2.5 increasing.16 A number of model simulations have used a uniform reactive uptake coefficient for HO2 on aerosols (γHO2 = 0.2)9,17–21 to focus on (1) studying emission control policies for O3 pollution reduction with the understanding that reduced PM2.5 concentration promotes more efficient O3 formation9,17–20 and (2) possible consideration of a third ‘aerosol inhibited’ regime for O3 formation in addition to the regular VOC-limited and NOx-limited regimes.21
According to Zhang et al. (2022),7 a non-linear polynomial function can be used to depict the O3–PM2.5 co-relationships for NYC based on surface measurements, with a positive linear term reflecting the O3/PM2.5 co-occurrence and a negative power function term reflecting the O3 formation suppression by PM2.5 (hereafter PM2.5-suppression factor). The PM2.5-suppression factors were also identified and likely to change along with the O3-sensitivity of the chemical regime in NYC.7 To verify the connection between the PM2.5-suppression factors and the O3-sensitive regime existing over a larger region, major cities of the Eastern U.S. and their downwind regions are selected in this study, with a focus on the Long Island Sound and surrounding area located just downwind of NYC.22–24 This study will focus on the spatial variation of the PM2.5-suppression factors over several major Eastern U.S. cities and also from urban to downwind regions (NYC as an example) and the temporal variation from 2004 to 2019 to explore the relationship between the PM2.5-suppression factors and the corresponding O3-sensitivity regimes over this regional scale.
2. Methods
2.1 Study locations and periods
Five air quality sites in NYC and downwind locations over Long Island Sound (Fig. S1† for the locations) were chosen as examples of the PM2.5-suppression factor variation from urban to downwind regions. These five sites include (a) two urban sites: the IS52 site in Bronx County of NYC and the Queens College (QC) site in Queens County of NYC in New York State, and (b) three downwind sites: the Eisenhower Park (EP) site in western Long Island in New York State, the Holtsville (HOL) site in central Long Island, and the Criscuolo Park (CP) site in New Haven in Connecticut state, which was chosen because it is frequently influenced by sea breezes that transport NYC urban outflow plumes.22–24 The IS52, QC, EP, and HOL sites belong to the New York State Department of Environmental Conservation (NYSDEC), and the CP site belongs to the Connecticut Department of Energy and Environmental Protection. In addition to these five sites for NYC and downwind locations, another 11 major Eastern U.S. cities with the U.S. Environmental Protection Agency measurement sites for O3 and PM2.5 in the urban regions were chosen to investigate the spatial/temporal variation of the PM2.5-suppression factor. To better cover the study period (2004–2019) with a daily report for O3 and PM2.5 of each city, we generally select the data from 2–3 sites in or near (within 10 km) the downtown regions, with the location of the representative site of each city shown in Table S1.† The study period is from 2004 to 2019 during summertime (June, July, and August), which was separated into three subperiods based on the PM2.5 variation (Fig. S2,† SP1: 2004–2008; SP2: 2009–2013, SP3: 2014–2019). These MDA8 O3 and the PM2.5 from the above sites can be found at https://www.epa.gov/outdoor-air-quality-data/download-daily-data.
2.2 Non-linear fitting of the O3–PM2.5 relationship
Eqn (1) includes (1) a positive linear term to reflect the O3/PM2.5 co-occurrence with its slope influenced by the aerosol chemical composition (this slope varies as a function of the atmospheric chemical composition, which in turn varies with emission controls), (2) a negative power function term with an exponent of 5/3 reflecting the suppression of O3 formation by PM2.5, (i.e., the uptake of HO2/NO3 by PM2.5, etc.), and (3) a constant with the possibility of implying the background O3 without PM2.5.7 The power function exponent was set to 5/3, based on the consideration that (a) the uptake coefficients of the radicals related to the aerosol surface concentration which is expected to be proportional to the 2/3 power of PM2.5 mass concentration, and (b) the radical concentrations were simply assumed to relate with the O3 concentration, which is proportional to the PM2.5 mass concentration as mentioned in the above positive linear term. | O3 = aPM2.5 − b(PM2.5)5/3 + c | (1) |
The coefficient a is the slope of the linear term, b is the power function coefficient, and c is the constant. These three factors will be obtained through non-linear fitting. It should be noted that eqn (1) only represents a very idealized solution for the non-linear O3–PM2.5 relationship, with oversimplified terms and factors, and will cause some uncertainty for the results. More specifically, the impact of aerosol on the O3 formation could be much more complicated with additional or different processes, including other heterogeneous reactions besides the uptake of HO2/NO3 by PM2.5, changes to photolysis rates and direct radiative forcing, changes to meteorological conditions, i.e., boundary layer height and ventilation, temperature and wind speed, etc.25,26 All of these would complicate the equation and introduce new terms to better describe the relationship between PM2.5 and O3. Further studies related to the mechanism are warranted to explore a more accurate function.
The power function coefficient is defined as the “PM2.5-suppression factor”, which indicates the magnitude of suppression of O3 formation by PM2.5 at the same PM2.5 level when doing spatial/temporal comparisons, and will be the focus of this study. In this study, the MDA8 O3 and the DA24 PM2.5 concentrations were used for the O3 and PM2.5 values. The PM2.5 data were initially binned following the approach in Li et al.8 and Buysse et al.27 with increments of 5 μg m−3, which was used to ensure enough statistical points in each bin. However, any site with only four bins when using the increment of 5 μg m−3 was binned in increments of 4 μg m−3 to ensure sufficient points for fitting. In addition, discrete bins having only one or two highest PM2.5 mass concentrations were left out, as they are considered quite probably to be due to the influence of other factors, such as the extreme wildfire smoke plumes. It should be noted that the binned dataset used in this study would constitute a simplified but empirically valid mechanism for the MDA8 O3 and DA24 PM2.5 relationship.
2.3 O3-sensitivity regime
The ratio of the HCHO column concentration and NO2 column concentration from the Ozone Monitoring Instrument (OMI)28,29 was used as the indicator for the early afternoon O3-sensitivity regime (considering the OMI overpass time around 01
:
30 pm at local time) for each site as described by Jin et al. (2020),27 and the data can be found from https://giovanni.gsfc.nasa.gov/giovanni. Jin et al. (2020)30 determined that the high ozone probability (over 70 ppb 8 hour average) peaks at an HCHO/NO2 ratio near 3.6 with a range of [3.2–4.1] for the average of the 7 cities they studied (Los Angeles, New York, Chicago, Washington, Pittsburgh, Atlanta, Houston). The ratios below this are roughly considered as VOC-limited chemistry, and above as NOx-limited chemistry. In this study, a spatial range of 0.5° × 0.5° was used for each city to get the area-averaged column concentration of HCHO and NO2, which were used further to obtain the seasonal averaged HCHO/NO2 ratio.
3. Results and discussion
3.1 Enhanced summertime PM2.5-suppression on O3 formation over the NYC metro area from urban to downwind
The MDA8 O3 and the DA24 PM2.5 concentrations were used to derive their relationships for these subperiods (SP1: 2004–2008; SP2: 2009–2013, SP3: 2014–2019) of the study period (2004–2019), and these relationships were further fitted using eqn (1) as mentioned in Section 2.2. The subperiods were divided based on the variation of the DA24 PM2.5 mass concentration, and the standard deviation of the annual summertime average DA24 PM2.5 in each period was below 1 μg m−3.7 A detailed description of the polynomial equation for fitting the non-linear relationship between O3 and PM2.5 can be found in Zhang et al. (2022).7 Over the past 16 years, the linear slope of the O3–PM2.5 relationship increased with time for both NYC urban sites and its downwind ones (Fig. 1a–e), which was verified to be related to the increased mass fraction of the secondary organic aerosol (SOA) and ammonium nitrate (NH4NO3), caused by the more significant reductions in the emissions of SO2 and PM2.5 than those of VOCs and NOx.7
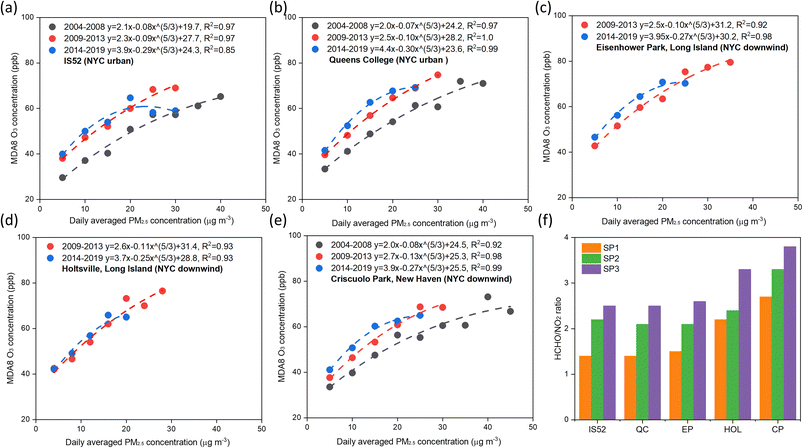 |
| Fig. 1 (a–e) The O3vs. PM2.5 relationships over the NYC (a): the IS52 site of NYC, (b): the Queens College site of NYC and its downwind regions (c): the Eisenhower Park site of Long Island, (d): the Holtsville site of Long Island, and €: the Criscuolo Park site of New Haven), and (f) the OMI HCHO/NO2 ratios for the subperiods of each site (QC: Queens College; EP: Eisenhower Park; HOL: Holtsville; CP: Criscuolo Park). (SP1: 2004–2008; SP2: 2009–2013, SP3: 2014–2019). | |
For the highlighted PM2.5-suppression factor in this study, a clear trend was observed in the NYC urban sites (IS52 and Queens College, Fig. 1a and b) over the past 16 years, with a slight increase from SP1 to SP2 (both near 0.1) and a significantly enhanced step from SP2 to SP3 (near 0.3). The enhanced PM2.5-suppression factor is consistent with the increased HCHO/NO2 ratio (Fig. 1f), which is used to indicate the O3-sensitivity regime through satellite observations.27 An increase in the value of the HCHO/NO2 ratio indicates a shift of the O3-sensitivity regime towards NOx-limited chemistry, and could be (1) a complete change in O3 chemistry sensitivity from VOC-limited to NOx-limited, (2) a shift towards NOx sensitivity (VOC-limited to weakly VOC-limited/transitional), or (3) increasingly NOx-limited O3 chemistry. Fig. 1f shows that the NYC urban region (IS52 and QC) shifted from a strong VOC-limited regime at SP1 (HCHO/NO2 ∼ 1.5) to a weak VOC-limited regime near the lower end of the transitional regime at SP3 (HCHO/NO2 ∼ 2.7). However, the variation of the HCHO/NO2 ratio comparing SP2 to SP1 (SP2 vs. SP1: 2.2 vs. 1.5, 46% increasing compared to SP1) was larger than the variation of the PM2.5-suppression factor (both near 0.1, Fig. 1a and b), and this generally matches the current model simulation result about the Ox–NOx relationship considering the PM2.5 effect over Chinese urban regions from Li et al. (2022),31 which indicated that the PM2.5-suppression effect was weaker at higher NOx concentrations in the VOC-limited regime, but strengthened as the O3-sensitivity approached the transitional regime. The weak PM2.5-suppression effect in the VOC-limited regime could be due to the competition for the consumption of HOx by NOx rather than PM2.5, making the NOx concentration the dominant factor for O3 concentration sensitivity under these conditions.31
For the NYC downwind sites (Eisenhower Park site, Fig. 1c; Holtsville site, Fig. 1d, and Criscuolo Park site, Fig. 1e), their PM2.5-suppression factors for each subperiod were similar to the NYC urban sites (IS51 site, Fig. 1a and Queens College site, Fig. 1b). This can be attributed to urban plume transport, which has been discussed in some detail from recent and current studies based on the 2018 Long Island Sound Tropospheric Ozone Study (LISTOS),22–24 and the formed O3 and PM2.5 and some unreacted precursors can be carried to downwind regions. Meanwhile, based on the fact that the averaging time period for MDA8 O3 (8 hours) and DA24 PM2.5 (24 hours) is generally much longer than the time scale of the photochemical reactions, it is reasonable to believe that the urban plume transport could result in the similar PM2.5-suppression factors for both urban and downwind sites. However, except for the Eisenhower Park site which had an HCHO/NO2 ratio for each subperiod similar to the Queens College site as their proximity to each other (<20 km), the HCHO/NO2 ratios of each subperiod for the Holtsville site (around 70 km from Queens College site) and the Criscuolo Park site (around 100 km from the Queens College site) were much higher than the values of the NYC urban sites (Fig. 1f). Based on satellite observations, this indicates that Holtsville and Criscuolo Park were closer to the NOx-limited regime than NYC urban sites. These differences between the PM2.5-suppression factors and the HCHO/NO2 ratios could come from the discrepancy between the hourly/daily averaged ground measurements and the early afternoon much shorter time period of satellite data capture.
3.2 Enhanced summertime PM2.5-suppression on O3 formation over major cities in the Eastern U.S.
The analysis of the increased PM2.5-suppression effect was also expanded to other major cities of the Eastern U.S., as shown in Fig. 2. More detailed information about the O3vs. PM2.5 relationships of each city and their fitting results are shown in Fig. S3.† The PM2.5-suppression factors of these major cities were below/near 0.1 in SP1 (Fig. 2a and 3) and over 0.2 in SP3 (Fig. 2c and 3), and generally increased following the O3-sensitivity regime moving toward a stronger NOX-limited chemistry, being indicated by their increased HCHO/NO2 ratios from SP1 to SP3 (Fig. 3). Meanwhile, the PM2.5-suppression factors showed a clear spatial discrepancy throughout the Eastern U.S. comparing north and south, especially in SP3 (2014–2019). The PM2.5-suppression factors of the southern cities (i.e., Nashville, Atlanta, Charlotte, and Jacksonville) in SP3 were near or over 0.4, which were clearly higher than the ones of the northern cities (Chicago, Pittsburgh, Boston, NYC, Philadelphia, Baltimore, and Washington, D.C.) with a major range of [0.2–0.3]. The PM2.5-suppression factors of these southern cities, to some extent, matched their stronger NOx-limited chemistry, as shown by their relatively higher HCHO/NO2 ratios. Under a stronger NOx-limited chemistry, the O3 concentration is more sensitive to the NOx variation, which will enhance the effect of the NO3 uptake by PM2.5 on O3 formation and match a higher PM2.5-suppression factor.
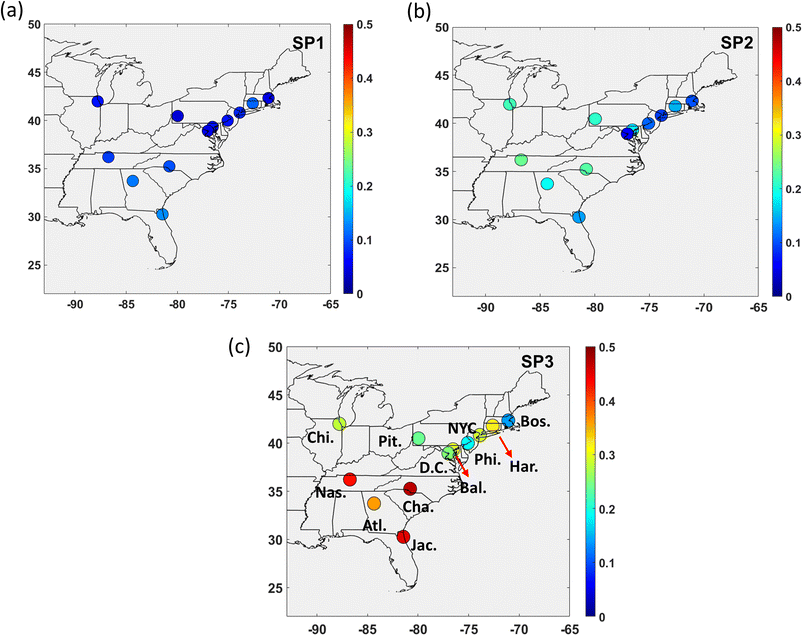 |
| Fig. 2 The PM2.5-suppression factor distribution map for the twelve major cities in the Eastern U.S. for (a) Subperiod 1 (SP1:2004–2008), (b) Subperiod 2 (SP2: 2009–2013), and (c) Subperiod 3 (SP3: 2014–2019). These twelve major cities include Chicago (Chi., Illinois), Pittsburgh (Pit., Pennsylvania), Boston (Bos., Massachusetts), Hartford (Har., Connecticut), NYC (New York), Philadelphia (Phi., Pennsylvania), Baltimore (Bal., Maryland), Washington, D.C. (District of Columbia), Charlotte (Cha., North Carolina), Atlanta (Atl., Georgia), Jacksonville (Jac., Florida), and Nashville (Nas., Tennessee). | |
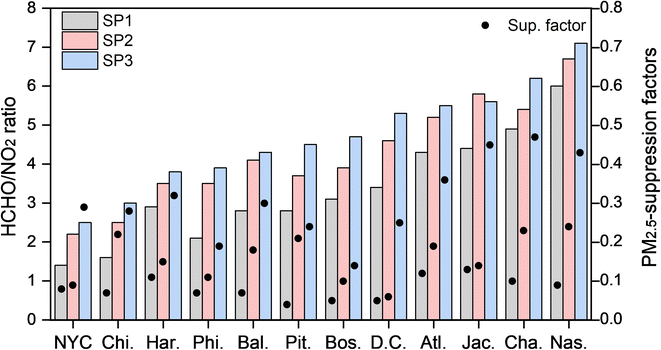 |
| Fig. 3 The OMI HCHO/NO2 ratios and PM2.5-suppression factors for the subperiods of each city mentioned in Fig. 2. | |
Based on the fact that (1) the PM2.5-suppression factor increases as the O3-sensitivity regime shifts toward stronger NOX-limited chemistry and (2) the relatively small variation range of the PM2.5-suppression factors for the cities with similar urban conditions (i.e., those northeast urban cities with a range of [0.2–0.3] in SP3), it is reasonable to suppose the summertime PM2.5-suppression factor derived from the polynomial fits can be used provide information for identifying the O3-sensitivity regimes based on the ground measurement, in addition to the satellite measurements using the HCHO/NO2 ratios. However, it is hard to build a highly correlated relationship between the PM2.5-suppression factor and the HCHO/NO2 ratios (as shown in Fig. S4†), considering the facts that (1) PM2.5-suppression factor changed little in the VOC-limited regime, (2) local atmospheric chemistry varied, (3) the HCHO/NO2 ratios are for early afternoon photochemistry while the PM2.5-suppression factor for a daily average, etc. More studies are warranted for exploring the usage of the PM2.5-suppression factor for O3-sensitivity regimes and its relationship with the satellite-measured HCHO/NO2 ratios in a world range. More especially, the future datasets from the TEMPO satellite (https://tempo.si.edu/) with hourly fluctuations in HCHO/NO2 values will largely promote the understanding the hourly variation of the O3-sensitivity regimes and the daily averaged O3-sensitivity regimes, which could build a better relationship to the ground measurements/derived suppression factors from this study.
3.3 Atmospheric implications of the increased summertime PM2.5-suppression factor
The relationship of the PM2.5-suppression factor with the O3-sensitivity regime also indicates the necessity of considering the effect of the HO2 uptake coefficient (γHO2) on aerosol surfaces, which is most commonly implemented as a constant value, i.e., γHO2 = 0.2 for a number of previous studies.15–21 The increased PM2.5-suppression factor following the O3-sensitivity regime from VOC-limited to NOx-limited can also partly explain the discrepancy between the ambient measurement of HO2 uptake from a rural site in North China Plain (Wangdu County, Hebei province, China) by Tan et al. (2020)32 and the model result for Chinese urban areas from Li et al. (2019).8 Given that Wangdu County was located at a VOC-limited regime in 2014,32,33 it is reasonable to infer that its PM2.5-suppression factor was relatively low, considering (1) the rural Wangdu measurement site in 2014 was highly influenced by polluted regions upwind (Baoding City with a distance of about 35 km and Shijiazhuang City with a distance of about 85 km), making it similar to the conditions of the NYC downwind sites, and (2) a derived PM2.5-suppression factor of approximately 0.02 was determined for North China Plain major cities (including Shijiazhuang City and Baoding City, Zhang et al., 2022).7 This quite low PM2.5-suppression factor implies little influence of HOx uptake by aerosol and matches the derived low HO2 uptake coefficient during daytime (∼0.08) from Tan et al. (2020)32 and Song et al. (2022).33 Following the increase in PM2.5-suppression factor from 0.02 to 0.06 from 2014–2016 to 2017–2019 for North China Plain major cities,7 the γHO2 would be expected to increase to be nearer to the value (γHO2 = 0.2) used in the model.15–21 However, the uptake coefficients γHO2 vary significantly depending on both aerosol size and composition, and ambient conditions such as the humidity. Its variation related to the change in PM2.5-suppression factor under different O3-sensitivity regimes, as well as the underlying mechanism needed to be verified and explored through more field measurements, especially in the highly air polluted regions.
4. Conclusion
The PM2.5-suppression factors on the surface ozone production of Eastern US major cities were derived based on non-linear fitting of the PM2.5 and O3 relationship. These factors derived from urban regions showed increasing trends over 16 years of continuous ground measurements and increased from a value below 0.1 during 2004–2008 to over 0.2 during 2014–2019, and generally followed the transition of the O3-sensitivity regime from VOC-limited toward NOx-limited chemistry.
A spatial similarity for the PM2.5-suppression factors between urban regions and their downwind locations was shown for the New York City metro area. However, the spatial distribution of the PM2.5-suppression factors of different urban regions showed generally larger values over cities with higher HCHO/NO2 values – which implies a more NOx-limited regime. The temporal and spatial variation of these PM2.5-suppression factors was consistent with the variation in the O3-sensitivity regime and provides a feasible way of identifying the O3-sensitivity regimes through this factor with the possibility of better representing the near ground O3-sensitivity chemistry than the current satellite-measured HCHO/NO2 column concentration ratios. The variation of PM2.5-suppression factors raises the possibility of a changeable HO2/NO3 uptake coefficient on aerosol surfaces for the model simulation of O3 pollution, and more studies/measurements are required to verify this possibility. The results from this study will provide useful guidance for further O3–PM2.5 studies considering a range of PM2.5-suppression factors, which in turn will constrain/evaluate model simulations for O3 and PM2.5 concentrations after considering the varied PM2.5-suppression factors and/or the varied HO2/NO3 uptake coefficients. These also benefit the ability of models to develop accurate O3 and PM2.5 concentration pollution control policies.
Conflicts of interest
There are no conflicts of interest to declare.
Acknowledgements
This work has been supported through the New York State Energy Research and Development Authority (NYSERDA) Contract 48971. We acknowledge the free use of ozone and PM2.5 data from the US EPA, the OMI HCHO, and NO2 data from the US NASA. Special thanks to Dr Lukas Valin from the US EPA for providing valuable comments for this study.
References
- J. L. Schnell and M. J. Prather, Co-occurrence of extremes in surface ozone, particulate matter, and temperature over eastern North America, Proc. Natl. Acad. Sci. U.S.A., 2017, 114(11), 2854–2859 CrossRef CAS PubMed.
- S. A. Strode, J. M. Rodriguez, J. A. Logan, O. R. Cooper, J. C. Witte and L. N. Lamsal,
et al., Trends and variability in surface ozone over the United States, J. Geophys. Res. Atmos., 2015, 120(17), 9020–9042 CrossRef.
- T. J. Sullivan, C. T. Driscoll, C. M. Beier, D. Burtraw, I. J. Fernandez and J. N. Galloway,
et al., Air pollution success stories in the United States: The value of long-term observations, Environ. Sci. Policy, 2018, 84, 69–73 CrossRef CAS.
-
EPA, Our Nation's Air: Status and Trends through 2021, 2021. https://gispub.epa.gov/air/trendsreport/2021/ Search PubMed.
- C. L. Blanchard, S. L. Shaw, E. S. Edgerton and J. J. Schwab, Emission influences on air pollutant concentrations in New York State: I. ozone, Atmos. Environ. X, 2019, 3, 100033 CAS.
- C. L. Blanchard, S. L. Shaw, E. S. Edgerton and J. J. Schwab, Emission influences on air pollutant concentrations in New York state: II. PM2.5 organic and elemental carbon constituents, Atmos. Environ. X, 2019, 3, 100039 CAS.
- J. Zhang, J. Wang, Y. Sun, J. Li, M. Ninneman and J. Ye,
et al., Insights from ozone and particulate matter pollution control in New York City applied to Beijing, NPJ Clim. Atmos. Sci., 2022, 5(85), 1–7 CAS.
- K. Li, D. J. Jacob, H. Liao, J. Zhu, V. Shah and L. Shen,
et al., A two-pollutant strategy for improving ozone and particulate air quality in China, Nat. Geosci., 2019, 12(11), 906–910 CrossRef CAS.
- X. Chen, Z. Jiang, Y. Shen, R. Li, Y. Fu and J. Liu,
et al., Chinese regulations are working—why is surface ozone over industrialized areas still high? Applying lessons from Northeast US air quality evolution, Geophys. Res. Lett., 2021, 48(14), e2021GL092816 CrossRef.
- J. Zhu, L. Chen, H. Liao and R. Dang, Correlations between PM2.5 and ozone over China and associated underlying reasons, Atmosphere, 2019, 10(7), 352 CrossRef CAS.
- D. J. Jacob, Heterogeneous chemistry and tropospheric ozone, Atmos. Environ., 2000, 34(12–14), 2131–2159 CrossRef CAS.
- Y. Kanaya, P. Pochanart, Y. Liu, J. Li, H. Tanimoto and S. Kato,
et al., Rates and regimes of photochemical ozone production over Central East China in June 2006: a box model analysis using comprehensive measurements of ozone precursors, Atmos. Chem. Phys., 2009, 9(20), 7711–7723 CrossRef CAS.
- L. K. Whalley, K. L. Furneaux, A. Goddard, J. D. Lee, A. Mahajan and H. Oetjen,
et al., The chemistry of OH and HO2 radicals in the boundary layer over the tropical Atlantic Ocean, Atmos. Chem. Phys., 2010, 10(4), 1555–1576 CrossRef CAS.
- Y. Sakamoto, Y. Sadanaga, J. Li, K. Matsuoka, M. Takemura and T. Fujii,
et al., Relative and absolute sensitivity analysis on ozone production in Tsukuba, a city in Japan, Environ. Sci. Technol., 2019, 53(23), 13629–13635 CrossRef CAS PubMed.
- S. Stadtler, D. Simpson, S. Schröder, D. Taraborrelli, A. Bott and M. Schultz, Ozone impacts of gas–aerosol uptake in global chemistry transport models, Atmos. Chem. Phys., 2018, 18(5), 3147–3171 CrossRef CAS.
- R. R. Dickerson, S. Kondragunta, G. Stenchikov, K. L. Civerolo, B. G. Doddridge and B. N. Holben, The impact of aerosols on solar ultraviolet radiation and photochemical smog, Science, 1997, 278(5339), 827–830 CrossRef CAS PubMed.
- K. Li, D. J. Jacob, H. Liao, L. Shen, Q. Zhang and K. H. Bates, Anthropogenic drivers of 2013–2017 trends in summer surface ozone in China, Proc. Natl. Acad. Sci. U.S.A., 2019, 116(2), 422–427 CrossRef CAS.
- F. Taketani, Y. Kanaya, P. Pochanart, Y. Liu, J. Li and K. Okuzawa,
et al., Measurement of overall uptake coefficients for HO2 radicals by aerosol particles sampled from ambient air at Mts. Tai and Mang (China), Atmos. Chem. Phys., 2012, 12(24), 11907–11916 CrossRef CAS.
- L. K. Xue, T. Wang, J. Gao, A. J. Ding, X. H. Zhou and D. R. Blake,
et al., Ground-level ozone in four Chinese cities: precursors, regional transport and heterogeneous processes, Atmos. Chem. Phys., 2014, 14(23), 13175–13188 CrossRef.
- W. Wang, D. D. Parrish, X. Li, M. Shao, Y. Liu and Z. Mo,
et al., Exploring the drivers of the increased ozone production in Beijing in summertime during 2005–2016, Atmos. Chem. Phys., 2020, 20, 15617–15633 CrossRef CAS.
- P. D. Ivatt, M. J. Evans and A. C. Lewis, Suppression of surface ozone by an aerosol-inhibited photochemical ozone regime, Nat. Geosci., 2022, 15(7), 536–540 CrossRef CAS.
- J. Zhang, M. Ninneman, E. Joseph, M. J. Schwab, B. Shrestha and J. J. Schwab, Mobile laboratory measurements of high surface ozone levels and spatial heterogeneity during LISTOS 2018: Evidence for sea breeze influence, J. Geophys. Res. Atmos., 2020, 125(11), e2019JD031961 CrossRef CAS.
- M. H. Couillard, M. J. Schwab, J. J. Schwab, C. H. Lu, E. Joseph and B. Stutsrim,
et al., Vertical Profiles of Ozone Concentrations in the Lower Troposphere Downwind of New York City During LISTOS 2018–2019, J. Geophys. Res. Atmos., 2021, 126(23), e2021JD035108 CrossRef CAS.
- J. Zhang, A. Catena, B. Shrestha, J. Freedman, E. McCabe and M. J. Schwab,
et al., Unraveling the interaction of urban emission plumes and marine breezes involved in the formation of summertime coastal high ozone on Long Island, Environ. Sci. Atmos., 2022, 2(6), 1438–1449 CAS.
- J. Xing, R. Mathur, J. Pleim, C. Hogrefe, C. M. Gan and D. C. Wong,
et al., Air pollution and climate response to aerosol direct radiative effects: a modeling study of decadal trends across the northern hemisphere, J. Geophys. Res., 2015, 120, 12221–12236 Search PubMed.
- J. Xing, J. Wang, R. Mathur, S. Wang, G. Sarwar and J. Pleim,
et. al., Impacts of aerosol direct effects on tropospheric ozone through changes in atmospheric dynamics and photolysis rates, Atmos. Chem. Phys., 2017, 17, 9869–9883 CrossRef CAS PubMed.
- C. E. Buysse, A. Kaulfus, U. Nair and D. A. Jaffe, Relationships between particulate matter, ozone, and nitrogen oxides during Urban smoke events in the Western US, Environ. Sci. Technol., 2019, 53, 12519–12528 CrossRef CAS PubMed.
-
K. F. Boersma, H. Eskes, A. Richter, I. D. Smedt, A. Lorente, S. Beirle, et al., QA4ECV NO2 Tropospheric and Stratospheric Column Data from OMI (2017), 2017, https://doi.org/10.21944/qa4ecv-no2-omi-v1.1 Search PubMed.
- I. De Smedt, N. Theys, H. Yu, T. Danckaert, C. Lerot and S. Compernolle,
et al., Algorithm theoretical baseline for formaldehyde retrievals from S5P TROPOMI and from the QA4ECV project, Atmos. Meas. Tech., 2018, 11, 2395–2426 CrossRef CAS.
- X. Jin, A. Fiore, K. F. Boersma, I. D. Smedt and L. Valin, Inferring changes in summertime surface Ozone–NOx–VOC chemistry over US urban areas from two decades of satellite and ground-based observations, Environ. Sci. Technol., 2020, 54(11), 6518–6529 CrossRef CAS PubMed.
- C. Li, Q. Zhu, X. Jin and R. C. Cohen, Elucidating Contributions of Anthropogenic Volatile Organic Compounds and Particulate Matter to Ozone Trends over China, Environ. Sci. Technol., 2022, 56(18), 12906–12916 CrossRef CAS.
- Z. Tan, A. Hofzumahaus, K. Lu, S. S. Brown, F. Holland and L. G. Huey,
et al., No evidence for a significant impact of heterogeneous chemistry on radical concentrations in the North China Plain in summer 2014, Environ. Sci. Technol., 2020, 54(10), 5973–5979 CrossRef CAS.
- H. Song, K. Lu, H. Dong, Z. Tan, S. Chen and L. Zeng,
et al., Reduced aerosol uptake of hydroperoxyl radical may increase the sensitivity of ozone production to volatile organic compounds, Environ. Sci. Technol. Lett., 2021, 9(1), 22–29 CrossRef.
|
This journal is © The Royal Society of Chemistry 2024 |
Click here to see how this site uses Cookies. View our privacy policy here.