DOI:
10.1039/D3AY02258G
(Critical Review)
Anal. Methods, 2024,
16, 2777-2809
Paper-based sensors: affordable, versatile, and emerging analyte detection platforms
Received
18th December 2023
, Accepted 19th March 2024
First published on 22nd March 2024
Abstract
Paper-based sensors, often referred to as paper-based analytical devices (PADs), stand as a transformative technology in the field of analytical chemistry. They offer an affordable, versatile, and accessible solution for diverse analyte detection. These sensors harness the unique properties of paper substrates to provide a cost-effective and adaptable platform for rapid analyte detection, spanning chemical species, biomolecules, and pathogens. This review highlights the key attributes that make paper-based sensors an attractive choice for analyte detection. PADs demonstrate their versatility by accommodating a wide range of analytes, from ions and gases to proteins, nucleic acids, and more, with customizable designs for specific applications. Their user-friendly operation and minimal infrastructure requirements suit point-of-care diagnostics, environmental monitoring, food safety, and more. This review also explores various fabrication methods such as inkjet printing, wax printing, screen printing, dip coating, and photolithography. Incorporating nanomaterials and biorecognition elements promises even more sophisticated and sensitive applications.
1. Introduction
In the era of the internet and technological advancement, life is perpetually accelerating, and the demand for swift solutions to a wide array of challenges, problems, and health issues has never been more pressing.1 In an increasingly interconnected world, individuals across different domains, from homemakers juggling household appliances to scientists conducting cutting-edge research, engineers overseeing complex construction sites, and healthcare professionals diagnosing and treating patients, all rely on a common but often unseen hero: sensors. Sensors, ranging from the commonplace to the highly specialized,2 have become the linchpin of modern life, orchestrating machines and equipment with precision and enabling the success of various endeavours. They underpin a data-driven world, translating external inputs into meaningful outputs, thereby ensuring the smooth operation of an array of systems.
Broadly, a sensor can be defined as a device, module, or machine that interfaces with its environment, adeptly responding to external inputs, detecting these inputs, quantifying them, and, most crucially, transforming them into data or actions that can be stored, transmitted, displayed, or employed for diverse purposes.3 The significance of accessible and easily operated sensors cannot be overstated in the context of today's world, where data acquisition is pivotal across a range of sectors, from healthcare to environmental monitoring, industrial automation, disaster management, and scientific research.
While there is a multitude of sensors, each with specific applications,4 this article places a spotlight on a rapidly emerging and highly versatile category: paper-based sensors. These sensors have garnered immense attention due to their unique attributes and transformative potential. With roots dating back to earlier research endeavors,5 paper-based sensors epitomize a novel frontier in sensing technology. They have charmed scientists and industries alike with their simplicity, cost-effectiveness, and easy portability, all of which are redefining the landscape of analytical chemistry and diagnostic applications. At the heart of paper-based sensors lies an elegantly straightforward principle.
1.1 Principle of operation
1.1.1 Chemical or biochemical impregnation.
The paper substrate is treated or impregnated with specialized chemical or biochemical reagents. These reagents exhibit a remarkable ability to selectively react with specific target analytes present in a sample. When such a reaction occurs, it triggers a visible change in color or intensity in the paper.
1.1.2 Quantitative indication.
Crucially, the magnitude of the color change or intensity shift is directly proportional to the concentration of the analyte within the sample.
These attributes render paper-based sensors an ideal choice for a wide range of applications, particularly in settings where access to sophisticated laboratory equipment is limited or impractical. These sensors have found their place in a multitude of applications, from environmental monitoring to track pollution levels to healthcare, offering diagnostic solutions and point-of-care testing. Additionally, they are instrumental in ensuring food safety by providing rapid and accessible quality assessment. Their myriad advantages make them highly appealing:
Cost-efficiency: compared to conventional sensing technologies, paper-based sensors are more affordable and cost effective due to a number of reasons such as;
Material cost: the most common and affordable material used in paper-based sensors is cellulose-based paper. Paper is substantially less expensive to produce and get than other substrates used in typical sensors, such silicon6 or glass.7
Printing techniques: simple pen-on-paper techniques, screen printing, inkjet printing, and other printing techniques can all be used to create paper-based sensors. Due to their scalability and affordability, these methods enable the low cost per unit mass manufacture of sensors.
Less complexity in manufacturing: compared to their conventional counterparts, paper-based sensors frequently call for less complicated manufacturing procedures. Typically, the fabrication process entails printing or depositing sensing devices onto paper substrates, which are then simply encapsulated using techniques. The production costs are reduced as a result of this optimized manufacturing method.
Paper-based sensors are frequently made with the intention of being used only once or thrown away. The necessity for expensive cleaning and maintenance processes connected with reusable sensors is eliminated by this disposability. In addition, paper sensors are reasonably priced for one-time usage in fields including environmental monitoring, food safety testing, and medical diagnostics.8
Integration and miniaturization: developments in microfabrication methods have made it possible to integrate and minimize sensing components on paper substrates. This integration keeps or even improves sensitivity and specificity while lowering the total cost by reducing the amount of sensing material needed for each sensor.
Customization and versatility: paper-based sensors are flexible in both their functional and design aspects. Customization is possible at a reasonable cost because researchers can modify the characteristics of paper substrates and sensor devices to fit certain applications. Because of its adaptability, sensors with reasonable prices can be developed for a variety of uses and sectors.
Low power consumption: a large number of paper-based sensors function without the need for complicated electronic components or external power sources. Rather, to identify analytes, they rely on passive methods like capillary action or colorimetric changes. Paper sensors are appropriate for environments with limited resources, such as those with expensive or restricted access to electricity, due to their low energy consumption, which also lowers operational expenses.
Paper-based sensors are generally less expensive because of their low-cost components, streamlined production methods, disposable nature, and adaptability in terms of design and integration. These qualities render paper-based sensors a desirable choice for a range of applications, especially those requiring scalable and reasonably priced sensing solutions.9,10 In Table 1, a comprehensive comparison between paper and conventional substrates highlights the superiority of paper as an optimal choice for sensor development.
Table 1 The comparison of traditional substrate with paper for making sensors
S. no. |
Property |
Material |
Paper |
PDMS |
Glass |
Silicon |
1 |
Surface profile |
Moderate |
Very low |
Very low |
Very low |
2 |
Flexibility |
Yes |
Yes |
No |
No |
3 |
Structure |
Fibrous |
Solid, gas-permeable |
Solid |
Solid |
4 |
Surface-to-volume ratio |
High |
Low |
Low |
Low |
5 |
Fluid flow |
Capillary action |
Forced |
Forced |
Forced |
6 |
Sensitivity to moisture |
Yes |
No |
No |
No |
7 |
Biocompatibility |
Yes |
Yes |
Yes |
Yes |
8 |
Disposability |
Yes |
No |
No |
No |
9 |
Biodegradability |
Yes |
To some extent |
No |
No |
10 |
High-throughput fabrication |
Yes |
No |
Yes |
Yes |
11 |
Functionalization |
Easy |
Difficult |
Difficult |
Moderate |
12 |
Spatial resolution |
Low to moderate |
High |
High |
Very high |
13 |
Homogeneity of the material |
No |
Yes |
Yes |
Yes |
14 |
Price |
Low |
Moderate |
Moderate |
High |
15 |
Initial investment |
Low |
Moderate |
Moderate |
High |
2. Selection of different substrates and nanoparticles for the synthesis of paper sensors
The selection of paper substrate and blending with different nanomaterials plays a pivotal role in the development of paper sensors, significantly influencing their performance, sensitivity, and overall reliability. The scientific community has explored a wide array of paper substrates and nanoparticles, nanotubes to design paper sensors that cater to diverse applications.
2.1 Cellulose paper
Regular cellulose paper, when modified with functional groups or coatings, becomes a versatile substrate for various paper sensors. This versatility allows scientists to tailor cellulose paper to meet specific application requirements. For instance, the addition of functional groups or coatings can enable cellulose paper to participate in colorimetric assays. In these assays, the paper interacts with analytes, resulting in detectable color changes that are proportional to analyte concentration. Moreover, cellulose paper can be employed in electrochemical sensors where chemical reactions at the paper's surface generate electrical signals, making it ideal for a wide range of electrochemical applications. Further the cellulose paper can be further designed to obtain filter paper and nitrocellulose paper as discussed below.
2.1.1 Cellulose filter paper.
Filter paper's exceptional porosity and fluid-wicking capabilities make it an ideal choice for various paper-based sensors. The high porosity ensures efficient sample absorption and distribution, while its capacity to wick fluids allows for rapid and uniform interactions with analytes. Filter paper finds its application in diverse fields, including pH sensors and glucose sensors. In the case of pH sensors, filter paper can effectively detect pH changes by interacting with pH-indicating reagents. Similarly, in glucose sensors, filter paper can serve as a matrix for glucose-specific enzymes, facilitating the rapid quantification of glucose levels.
2.1.2 Nitrocellulose paper.
Nitrocellulose paper has gained significant recognition for its role in lateral flow assays, particularly in widely-used diagnostic tests like pregnancy tests. Its standout feature is its exceptional capillary action, enabling swift liquid progression. This attribute is especially advantageous in diagnostic tests, where quick results are crucial. Nitrocellulose paper can efficiently immobilize biomolecules, enhancing its usability in diagnostic applications. When samples interact with nitrocellulose paper, they trigger specific biochemical reactions, leading to visible results, such as the appearance of lines or color changes.
2.1.3 Chitosan coated cellulose paper.
Chitosan-coated paper is tailored for the detection of heavy metals such as lead and mercury. Chitosan, derived from chitin, possesses metal-binding properties, making it an effective material for capturing and quantifying metal ions in samples. The specific interaction between chitosan and metal ions results in visible changes, such as color shifts or precipitation, indicating the presence and concentration of heavy metals.
2.1.4 Graphene oxide-infused cellulose paper.
The infusion of paper with graphene oxide or other nanomaterials has ushered in a new era of enhanced sensor performance. Graphene oxide-infused paper is often utilized in the creation of sensors designed to detect heavy metals and organic compounds. The inclusion of nanomaterials, such as graphene oxide, amplifies the sensor's sensitivity. These sensors can efficiently recognize specific analytes in complex sample matrices due to their enhanced affinity, leading to more precise and reliable results.
2.1.5 Carbon nanotube-infused cellulose paper.
Carbon nanotube-infused paper plays a unique role in the realm of paper-based sensors. It excels in creating conductive electrodes, which are indispensable in electrochemical and electronic sensors. The paper's interaction with analytes generates electrical responses, allowing for the quantification of analyte concentrations. In electronic sensors, the electrical properties of carbon nanotubes can be harnessed to enable electronic readouts and data transmission, expanding the scope of potential applications.
2.1.6 Gold nanoparticle deposited cellulose paper.
Gold nanoparticles are often incorporated into paper substrates for creating colorimetric sensors. These sensors utilize the unique optical properties of gold nanoparticles to detect and quantify various analytes, including ions and biomolecules. The interactions between gold nanoparticles and analytes induce noticeable color changes, providing a straightforward and easily interpretable means of analysis. This makes them particularly valuable in applications where rapid and on-site testing is required.
2.1.7 Silver nanoparticle deposited cellulose paper.
Silver nanoparticle-modified paper shares similarities with its gold counterpart and is also employed in colorimetric assays. These sensors can detect specific molecules by triggering color changes when the analyte interacts with the silver nanoparticles. This intuitive method of detection is valuable for various applications, including environmental monitoring and point-of-care diagnostics.
2.2 Polymer cellulose blended paper
The blending of various polymers with paper substrates enhances their properties, contributing to improved durability, flexibility, and stability. This blending is often used in microfluidic paper-based devices (μPADs), which require these characteristics. The μPADs find applications in various fields, from medical diagnostics to environmental monitoring, as their durability ensures they withstand complex sample matrices and rugged field conditions.
The selection of paper substrate plays a pivotal role in shaping the performance and applications of paper sensors. Researchers meticulously consider the nature of the target analyte, the sensitivity required, and the operational conditions when choosing the appropriate paper. In recent years, the utilization of different papers for colorimetric detection has been a growing focus for research groups. The choice of paper significantly influences the capabilities and versatility of paper-based sensors, further driving innovation in this field. Table 2 demonstrates a list of different types of paper used for designing paper sensors in the last two decades.
Table 2 Application of different types of paper sensors in detecting analytes in present
S. no. |
Type of metal ion detected |
Paper substrate |
Sensing element |
Sample/matrix |
Detection limit |
Ref. |
1 |
Hg(II) |
Whatman paper no. 1 |
Rhodamine functionalized polymeric probe |
Drinking water tap water |
0.2 ppm |
11
|
2 |
Hg(II) |
Cellulose nanofiber |
AuNPs |
Water |
0.0002 ppm |
12
|
3 |
Hg(II) |
Filter paper |
CysA@AuNPs |
Aqueous solution |
0.001 ppm |
13
|
4 |
Hg(II) |
Filter paper |
Terpyridine–quinolinium iodide |
Aqueous solutions |
0.2 ppm |
14
|
5 |
Hg(II) |
Whatman paper no. 1 |
Oxalicalix[4]arene–AgNPs |
Waters |
0.014 ppm |
15
|
6 |
Hg(II) |
Cellulose filter paper |
SiO2–AgNPs |
Aqueous media |
2.2 × 10−4 ppm |
16
|
7 |
Hg(II) |
Filter paper |
CdSe/ZnS and TMB |
Tap water |
0.018 ppm |
17
|
8 |
Cu(II) |
Whatman filter paper |
(8-Methoxy-1,3′,3′-trimethylspiro[chromene-2,2'indoline]) |
Tap water |
38 ppm |
18
|
9 |
Cu(II) |
Whatman filter paper 2 |
Chrome azurol S pyrocatechol violet |
Rain water and tap water |
1.7 ppm |
19
|
1.9 ppm |
10 |
Cu(II) |
Filter paper |
ZnSe nanoframes |
Environmental water |
50 ppm |
20
|
11 |
Cu(II) |
Chromatography paper |
Rhodamine B with silica NPs |
Drinking water |
0.7 ppm |
21
|
12 |
Cu(II) |
Whatman cellulose filter paper |
Tricyanofuranhydrazone |
— |
50 ppm |
22
|
13 |
Cu(II) |
Whatman filter paper |
Multi-dye system |
— |
2.23 ppm |
23
|
14 |
Cu(II) |
Filter paper |
Rhodamine–fluorene |
Water |
0.072 ppm |
24
|
15 |
Cr(VI) |
Glass fiber filter paper |
1,5-Diphenylcarbazide |
Tap water, river water, surface water, and electroplating wastewater |
0.010 ppm |
25
|
Cr(III) |
0.015 ppm |
16 |
Cr(VI) |
Nylon paper |
Chitosan with 1,5-diphenylcarbazide |
Water |
0.06 ppm |
26
|
17 |
Cr(III) + Cr(VI) |
Filter paper no. 1 |
Diphenyl carbazide |
Drinking, mineral, and tap waters |
0.003 and 0.002 ppm |
27
|
18 |
Total Cr |
Chromatography paper 1 CHR |
Diphenyl carbazide |
Water and soil |
0.25 ppm |
28
|
19 |
Cr(III) + Cr(VI) |
Whatman no. 1 |
Diphenyl carbazide |
Water and wastewater samples |
0.5 and 0.7 ppm |
29
|
20 |
Pb(II) |
Whatman no. 1 |
Dithizone |
Water |
10 ppm |
30
|
21 |
Pb(II) |
Quantitative filter paper |
Rhodamine 6G hydrazide |
Tap water |
0.5 ppm |
31
|
22 |
As(III) |
Glass microfiber filter paper |
Glucose-functionalized AuNPs |
Environmental water samples |
5.6 ppm |
32
|
Pb(II) |
7.7 ppm |
23 |
Ni(II) |
Whatman paper no. 1 |
Dimethylglyoxime |
River samples |
29 ppm |
33
|
24 |
Ni(II) |
Whatman 1 filter paper |
Dimethylglyoxime |
Industrial wastewater and river water |
2.9 ppm |
34
|
25 |
Ni(II) |
Whatman no. 1, 3, 4 and chromatography paper no. 1 |
Boric acid–polyvinyl alcohol with dimethylglyoxime |
— |
0.92 ppm |
35
|
26 |
Tuberculosis DNA |
Whatman chromatography no. 3 |
AuNPs |
Human disc tissue |
0.0195 ng mL−1 |
36
|
27 |
E. coli
|
Cellulose paper |
Zr-MOF |
Mice wound tissue |
104 CFU mL−1 |
37
|
28 |
HIV-1 p24 antigen |
Whatman chromatography no. 1 and 3 |
Anti-HIV |
Human blood plasma |
0.03 ng mL−1 |
38
|
29 |
Uric acid |
Fiberglass and TLC papers |
AuNPs |
Urine |
1.0 μmol L−1 |
39
|
30 |
Dopamine |
Nitrocellulose membrane |
AuNPs with 20T linkers |
Artificial urine |
10 ng mL−1 |
40
|
31 |
Lactoferrin |
Whatman qualitative filter paper 541 |
2-(5-Bromo-2-pyridylazo)-5-diethylamino phenol |
— |
110 μg mL−1 |
41
|
32 |
Pesticide (methyl paraoxon and chlorpyrifos-oxon) |
Whatman filter paper no. 4 |
Ceria nanoparticles |
Vegetables |
18 and 5.3 ng L−1 |
42
|
33 |
Chlorpyrifos |
Hyundai micro filter paper no. 51 |
Acetylcholinesterase and 3-indoxyl acetate |
Food products |
8.6–12.46 ppm |
43
|
34 |
Organophosphate and carbamate pesticide |
Canson paper |
5,5′-Dithiobis(2-nitrobenzoic)acid |
Food products |
0.27 mM and 6.16 mM |
44
|
35 |
Nitrite and nitrate |
Whatman qualitative filter paper 1 and 5 |
Griess reagent and sulfanilic acid |
Food products |
0.1 mg L−1 and 0.4 mg L−1 |
45
|
36 |
Norfloxacin |
Whatman qualitative filter paper 1, 2, 4, 5, and 42 |
Iron(III) nitrate nonahydrate |
Meat and fish |
50 ppm per 5 ppm |
46
|
3. Different methods opted to fabricate paper sensors
In the field of analytical chemistry, paper sensors have emerged as a transformative technology due to their simplicity, cost-effectiveness, and versatility across a wide range of applications. Various methods have been applied to design and fabricate paper sensors, each offering unique advantages and opportunities for innovation. Paper sensors have emerged as a revolutionary technology in analytical chemistry, gaining widespread recognition due to their simplicity, cost-effectiveness, and remarkable versatility in diverse applications. Over the last two to three decades, various methods have been employed to fabricate paper sensors, each method bringing unique advantages and applications. The following section provides an extensive overview of some of these methods and highlights key recent advancements.
3.1 Inkjet printing
Inkjet printing has ushered in a new era in paper sensor fabrication. This technique involves the precise ejection of tiny droplets of functional ink or sensing materials onto the paper surface using an inkjet printer. This method offers a level of control over deposition that is particularly suited for creating complex patterns and structures. Inkjet-printed paper sensors have found applications in diverse fields, including flexible electronics and wearable devices. In 2019, Ponram et al. introduced a different application of inkjet printing by depositing phosphorescent iridium(III) on paper surfaces. This innovation enabled the highly specific detection of Hg(II) ions.47 This work demonstrated the method's effectiveness in addressing environmental and health-related challenges. In 2020, Lee et al. showcased the potential of inkjet printing by creating a colorimetric technique on photo paper. This technique enabled the detection of 2,4,6-trinitrotoluene (TNT) in contaminated soil with an impressive detection limit of 14 ppm.48 This application exemplified the utility of inkjet-printed paper sensors in environmental monitoring and safety assessments. In 2021, Shrivas et al. harnessed the power of inkjet printing to fabricate a colorimetric sensor using silver nanoparticles (AgNPs) on paper substrates. This sensor was specifically designed for on-site detection of mercuric ions (Hg2+) in water samples, further highlighting the adaptability and accessibility of inkjet-printed paper sensors.49 In 2023, Lim et al. extended the capabilities of inkjet printing by fabricating paper-based sensors using graphene as a printing ink. These sensors were designed for breath monitoring, showcasing the potential applications of inkjet-printed paper sensors in the field of healthcare a schematic way as shown in Fig. 1.50
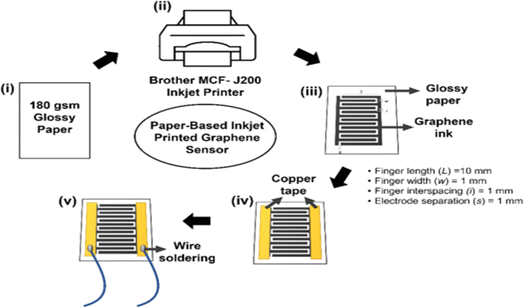 |
| Fig. 1 Schematic representation of a paper-based inkjet-printed graphene sensor [reprinted with permission from ref. 50 Lim W. Y., Goh C. H., Yap K. Z. and Ramakrishnan N., One-step fabrication of paper-based inkjet-printed graphene for breath monitor sensors, Biosensors, 2023, 13(2), 209, https://doi.org/10.3390/bios13020209. Copyright@MDPI]. | |
Inkjet printing has paved the way for precise and customizable paper sensors, enabling applications that range from environmental monitoring to advanced healthcare solutions. These sensors, with their fine-tuned features, are at the forefront of addressing real-world challenges and improving quality of life. Table 3 gives the recent work done in paper sensors using inkjet printing.
Table 3 The recent work done in paper sensor using inkjet printing method
S. no. |
Analyte |
Detector |
Printer |
Methods of detection |
Detection limit |
Ref. |
1 |
pH, glucose, protein |
Scanner |
Picojet-2000 |
Colorimetry |
Glucose-2.8–28 mM |
51
|
pH – 5–9 |
Human serum albumin-0.46–46 μM |
2 |
pH, human IgG, mouse IgG |
Scanner |
Picojet-2000 |
Colorimetry |
Human IgG – at least 10 ng mL−1 |
52
|
3 |
Paraoxon |
Digital camera, smartphone camera |
Dimatix DMP-2800 |
Colorimetry |
Paraoxon – 100 nM |
53 and 54 |
Aflatoxin B1 |
Aflatoxin B1 – 30 nM |
4 |
Paraoxon |
Digital camera |
Dimatix DMP-2800 |
Colorimetry |
Paraoxon – 1 nM |
55
|
Bendiocarb |
Bendiocarb – 1 nM |
Carbaryl |
Carbaryl – 10 nM |
Malathion |
Malathion – 10 nM |
5 |
Hg(II), Ag(I), Cu(II), Cd(II), Pb(II), Cr(IV), Ni(II) |
Digital camera, scanner |
Dimatix DMP-2800 |
Colorimetry |
Hg(II)-0.001 ppm |
56
|
Cu(II)-0.020 ppm |
Ag(I)-0.002 ppm |
Cd(II)-0.020 ppm |
Pb(II)-0.140 ppm |
Cr(IV)-0.150 ppm |
Ni(II)-0.230 ppm |
6 |
ATP |
Human nose |
Dimatix DMP-2800 |
Odour |
1.16 μM in buffer, 1.36 μM in orange punch |
57
|
7 |
pH, H2O2 |
Scanner |
Epson PX-101 |
Colorimetry |
pH: 4–9 |
58
|
H2O2: 14.4 μM |
8 |
Volatile primary amines |
Scanner |
Dimatix DMP-2831 |
Colorimetry |
Discrimination of n-CnH2n−1NH2 (n = 1–7) |
59
|
9 |
Nitrite, ALP |
Scanner |
Canon PIXMA iP4500 |
Colorimetry |
Nitrite (NO2−): 0–5 mM |
60
|
10 |
DBAE, NADH |
Smartphone camera |
Canon PIXMA iP4500 |
Electrochemiluminescence |
DBAE: 3 μM–5 mM (0.9 μM) |
61
|
NADH: 0.2–10 mM (200 μM) |
11 |
Nitrite, nitrate |
Scanner |
Canon P4700 |
Colorimetry |
Nitrite (NO2−): 10–150 μM |
62
|
Nitrate (NO3−): 50–1000 μM |
12 |
Reactive phosphate |
Scanner |
Canon P4700 |
Colorimetry |
0.2–10 mg L−1 P |
63
|
13 |
Metabolic volatiles from food products |
Hg lamp, epifluorescence microscope |
Hp Desk-jet 4280 |
Fluorescence |
Monitoring of meats, dairy, fruit, grain, vegetables and fruit spoilage |
64
|
14 |
Phenolic compounds |
Scanner |
Dimatix DMP-2800 |
Colorimetry |
BPA, p-cresol: 1–200, dopamine, catechol: 1–300, phenol: 1–400, m-cresol: 1–500 μg L−1 |
65
|
15 |
hCG |
Digital camera |
SLT0505-HKF |
Colorimetry |
1 ng mL−1 in buffer solution, 4 ng mL−1 in urine sample |
66
|
16 |
Volatile organic compounds |
UV lamp, digital camera, microscope |
HP Desk-jet D2360 |
Colorimetry, fluorescence |
Discrimination of 10 organic solvent vapours |
67
|
17 |
Morphine |
Scanner |
Dimatix DMP-2831 |
Colorimetry |
1 ng mL−1 |
68
|
18 |
Cationic surfactants, anionic polymers |
Digital camera |
Details not available |
Colorimetry |
Anionic polymers, cationic surfactants: at least 1 mM |
69
|
19 |
Glucose |
Spectrophotometer |
Epson ME1+ |
Colorimetry |
0–100 mM in urine |
70
|
20 |
B. subtilius, E. coli |
Scanner |
Epson Artisan 50 |
Colorimetry |
B. subtilius, E. coli – 103 CFU |
71
|
3.1.1 Advantages and disadvantages of inkjet printing.
Paper sensor design benefits greatly from the use of inkjet printing technology, which is inexpensive, flexible, and capable of producing intricate patterns at high resolution. But there are a few drawbacks to employing inkjet printing for this kind of work.
3.1.1.1 Limitations on resolution.
Inkjet printers can produce high resolutions,72 but for some complex sensor designs—particularly those that call for extremely minute details or exact patterning—the resolution might not be enough.
3.1.1.2 Limited ink compatibility.
While most inkjet printers can use a wide variety of inks, not all of them are compatible with sensor materials or appropriate for printing on paper substrates. This may limit the variety of materials that can be utilized in sensor fabrication.73 Inkjet-printed inks have the potential to penetrate or spread unevenly on paper substrates, which could result in differences in the accuracy and performance of the sensors. This can be especially troublesome for sensors that need exact control over the layering of ink.74
3.1.1.3 Limited compatibility with materials.
The varieties of sensor materials that can be utilized may be limited by the fact that inkjet printing is mostly appropriate for printing on porous or absorbent substrates like paper.75 Certain sensor materials could not print well in an inkjet printer or stick to paper poorly.
3.1.1. 4Durability issues.
Compared to sensors made using alternative methods or materials, inkjet-printed sensors on paper substrates could be less robust.76 Environmental factors, mechanical stress, and moisture can all have an impact on how long inkjet-printed paper sensors last and how reliable they are.
3.1.1.5 Post-processing requirements.
To improve performance, stability, and longevity, inkjet-printed paper sensors may need to undergo extra post-processing procedures like drying, curing, or coating. These procedures may increase the fabrication process's complexity and expense.77
3.2 Wax printing: a versatile technique for microfluidic paper-based devices
In the realm of paper sensor fabrication, wax printing stands as a versatile and essential technique. This method revolves around the precise application of a hydrophobic wax to paper through various means, such as inkjet printing, screen printing, or using wax pens. The hydrophobic wax serves as a crucial barrier, dictating the flow of liquids within specific regions on the paper. The significance of wax printing lies in its role in creating microfluidic paper-based devices (μPADs) as shown in Fig. 2, a cornerstone in diagnostic applications.
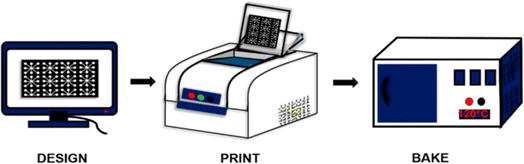 |
| Fig. 2 Schematic representation of wax printing on paper microfluidic devices [reprinted with permission from ref. 78 Prasad A., Tran T. and Gartia M. R., Multiplexed paper microfluidics for titration and detection of ingredients in beverages, Sensors, 2019, 19(6), 1286, https://doi.org/10.3390/s19061286. Copyright©MDPI]. | |
In the year 2019, the wax printing technique experienced significant advancements, led by the work of Strong and colleagues. They made a remarkable leap in the precision and resolution of wax printing by fabricating microfluidic paths on paper.79 The team achieved this feat by strategically altering the iodate concentration and initiating a series of chemical reactions. This breakthrough enhanced the technique's ability to achieve high-resolution designs, a crucial aspect for microfluidic paper devices. Wax printing boasts numerous advantages, which have contributed to its popularity. It is known for its cost-effectiveness, efficiency, rapid prototyping capabilities, and straightforwardness in usage. In fact, the prototyping of microfluidic devices can be completed in as little as five minutes, with subsequent adjustments being made even more swiftly.80
Wax printing remains at the forefront of paper sensor fabrication due to its precision, cost-effectiveness, and rapid prototyping capabilities. While challenges related to the availability of wax printers persist, the innovative spirit of researchers ensures that alternative approaches will continue to be explored, guaranteeing the continued utility of this versatile technique in the development of paper-based microfluidic devices and sensors. The whole fabrication process and some parameters that can be used in wax printing are given in Table 4.
Table 4 Parameters for wax printing
S. no. |
Paper |
Printer |
Time |
Temperature (°C) |
Design software |
Heating equipment |
Ref. |
1 |
Filter paper 202 and 102 |
Xerox Phaser 8560DN |
2 min |
150 |
— |
Oven |
81
|
2 |
Whatman chromatography paper no. 1 |
Xerox Phaser 8560DN |
2 min |
150 |
CleWin (PhoeniX B.V.) |
Hot plate |
82
|
3 |
Whatman chromatography paper no. 1 |
Xerox ColorQube 8570DN |
1 min |
125 |
CorelDRAW (Corel Corp.) |
Oven |
83
|
4 |
Whatman filter paper no. 114 |
Xerox Phaser 8560 |
2 min |
150 |
Illustrator (Abode Systems Inc.) |
Oven |
84
|
5 |
Whatman filter paper no. 4 |
Xerox ColorQube 8750 |
30 s |
150 |
Illustrator (Abode Systems Inc.) |
Oven |
85
|
6 |
Whatman filter paper no. 4 |
Xerox ColorQube 8750N |
5 min |
120 |
— |
Hot plate |
86
|
7 |
Whatman chromatography paper no. 1 |
Xerox ColorQube 8570 |
45 s |
120 |
— |
Oven |
87
|
8 |
Whatman chromatography paper no. 1 |
Xerox ColorQube 8750 |
— |
160 |
Microsoft Paint (Microsoft corp.) |
Laminator |
88
|
9 |
Whatman chromatography paper no. 1 |
Xerox Phaser 8560DN |
30 s |
150 |
Illustrator (Abode System Inc.) |
Oven |
89
|
10 |
Whatman chromatography paper no. 1 |
Xerox ColorQube 8750 |
2 min |
150 |
Photoshop (Abode System Inc.) |
Hot plate |
90
|
11 |
Advantec no. 2 filter paper (Advantec MFS) |
Xerox ColorQube 8570N |
10 min |
100 |
AutoCAD (Autodesk Inc.) |
Hot plate |
91 and 92 |
12 |
Whatman filter paper no. 114 |
Xerox Phaser 8000DP |
2 min |
120 |
— |
Oven |
93
|
13 |
Whatman chromatography paper no. 1 |
Xerox ColorQube 8570 |
50 s |
175 |
Illustrator (Abode Systems Inc.) |
Hot plate |
94 and 95 |
Recognizing the potential of wax printing, Chiang et al. introduced a 3D printing process in 2019 to fabricate paper-based microfluidic devices using wax as a pivotal element.96 In the same year, Wisang and colleagues harnessed the wax printing technique to construct microfluidic paper-based analytical devices designed for the colorimetric detection of lead(II).97
3.2.1 Advantages and disadvantages of wax printing.
It is imperative to acknowledge the present challenge faced by the wax printing technique. The production of wax printers has seen a decline, which poses a hurdle to this otherwise advantageous method.98 Along with this wax printing is often sensitive to temperature variations, which can affect the quality and consistency of the printed patterns. This may pose challenges in environments where temperature control is difficult.
In response to this challenge, researchers are diligently exploring alternative approaches to fabricating microfluidic paper-based devices using wax as a hydrophobic substance. One innovative solution is a hybrid wax printing process that combines a mini CO2 laser machine with a wax printer. This method allows for the simultaneous melting, heating, and piercing of wax for the design of μPADs. The commercial acceptance of μPADs continues to grow, encouraging manufacturers to consider the reproduction of wax printers, ultimately revitalizing this critical technique.99
3.3 Screen printing/stencil printing: precision crafting of paper-based sensors
In the realm of paper-based sensors, the art of screen printing or stencil printing is harnessed to create meticulously designed sensor patterns on paper. This method involves placing a stencil on the paper substrate and then meticulously depositing sensing materials through the openings in the stencil.100 The technique offers unmatched precision in controlling the pattern's creation, enabling the formation of well-defined structures on the paper surface. The foundational principles of screen printing are elegantly illustrated in Fig. 3, providing a comprehensive visual representation of this process.101
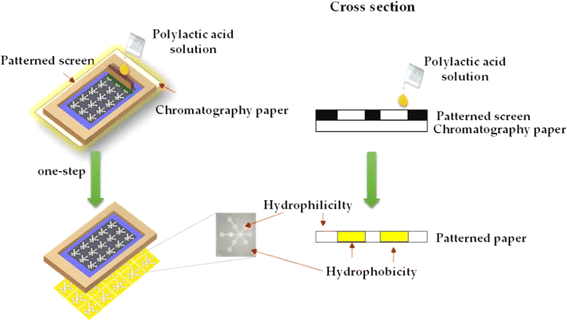 |
| Fig. 3 The fundamental processing model of screen printing [reprinted with permission from ref. 101 Teepoo S., Arsawiset S. and Chanayota P., One-step polylactic acid screen-printing microfluidic paper-based analytical device: application for simultaneous detection of nitrite and nitrate in food samples, Chemosensors, 2019, 7(3), 44, https://doi.org/10.3390/chemosensors7030044. Copyright©MDPI]. | |
In 2016, Cinti and colleagues showcased the capabilities of this technique by developing a paper-based electrochemical sensor that could detect phosphate levels with remarkable sensitivity.102 Three years later, in 2019, Jarujamrus and team demonstrated how screen printing could be employed to craft a hydrophobic barrier on paper using a specially formulated rubber solution.103 Within the same year, Caratelli et al. brought the advantages of screen printing to the construction of a three-electrode-based electrochemical sensor, catering to the detection of cholinesterase inhibitors, an essential endeavor for healthcare and environmental monitoring.104
3.3.1 Advantages and disadvantages of screen printing.
Screen printing is recognized for its holistic approach to sensor preparation. The necessary equipment and materials are readily available, contributing to its popularity. Additionally, the technique is known for its cost-effectiveness, making it an ideal choice for producing small batches of modified paper-based devices.105 Notably, screen printing's suitability extends to bulk production, further underscoring its versatility and applicability in crafting paper-based sensors for various applications.
Compared to other printing methods like inkjet printing or photolithography, screen printing might not be able to produce patterns with a high level of resolution. This may restrict the intricacy and accuracy of patterns that may be printed, which may have an impact on the sensitivity and functionality of the sensor.106 In addition to this, while using screen printing, it might be difficult to obtain exact control over the thickness of printed layers. For paper-based sensors to remain homogeneous and sensitive, layer thickness management is essential.107 Changes in layer thickness could have an impact on the sensors' repeatability and performance. Multilayer registration: to construct functional elements like electrodes, sensing layers, and insulating layers, printing many layers of distinct materials is a common process in the fabrication of paper sensors. It can be challenging to precisely align these layers during the screen printing process,108 which can result in misalignment problems that could impair sensor performance.
3.4 Dip-coating: precision crafting of functional paper-based sensors
In the realm of crafting advanced paper-based sensors, the dip-coating process emerges as a powerful technique. This method involves immersing a substrate, such as paper, into a solution or suspension, typically containing functional materials like nanoparticles, polymers, or chemical reagents. The moving unfolds as the substrate is gently withdrawn from the solution, orchestrated by the delicate dance of capillary and wetting forces.109 This process creates a delicate and controlled deposition of a thin film of the functional material onto the paper's surface. The control over the film's thickness is achieved by manipulating parameters like withdrawal speed, solution concentration, and the number of coating cycles, granting precision and fine-tuning capabilities to this method.
The utilization of dip-coating in creating paper-based sensors has garnered significant attention in recent years, largely owing to a multitude of advantages it offers. These sensors stand out for being lightweight, flexible, and disposable, attributes that render them exceptionally suitable for an array of applications, ranging from environmental monitoring to medical diagnostics and food safety.110
One of the defining strengths of this technique lies in its adaptability. By skilfully tailoring the composition of the coating material, paper-based sensors can be meticulously designed to detect a wide spectrum of analytes. This versatility equips them to respond to diverse analytical needs, from identifying chemical pollutants and biomolecules to detecting ions, thereby serving as dynamic analytical tools.110 Beyond its fundamental advantages, dip-coating bestows upon the sensor designer the ability to orchestrate intricate and multifaceted sensor designs. This enables the creation of paper-based sensors comprising multiple layers, each meticulously engineered to serve a distinct function. These layers can collectively capture target analytes, transduce signals, or facilitate the readout of critical data, showcasing the vast creative possibilities within this methodology.111
3.4.1 Advantages and disadvantages of dip coating.
As the world of paper-based sensors continues to advance, the dip-coating process stands as a cornerstone in achieving sophisticated and multifunctional paper-based sensor designs. Its lightweight, flexible, and adaptable nature, coupled with the ease of manipulation,112 makes it an indispensable tool for the development of sensors catering to multifaceted applications.
Taking about its disadvantages, certain materials used in dip coating formulae might not work well with paper substrates or might change the paper's characteristics in an unfavourable way, including reduction its flexibility or porosity.113 Further, dip coating procedures can take a while, particularly if complicated sensor designs or several coating layers are needed. This may affect the production efficiency and scalability of paper-based sensors.114 In addition to this, dip coating frequently calls for the use of chemicals and solvents, which could lead to issues with waste disposal, worker safety, and solvent emissions.115
3.5 Photolithography: crafting precision patterns for advanced paper sensors
In the world of sensor fabrication, photolithography, often referred to as “photolitho” or “photopatterning,” shines as a versatile and precise manufacturing technique that has, historically, been the stronghold of the semiconductor industry. However, its capabilities have transcended boundaries, finding resonance in a multitude of applications, including the development of paper sensors.116 Photolithography takes center stage in the creation of these sensors by empowering the precise patterning of materials onto the paper substrate.
The core of photolithography lies in the manipulation of light, usually in the form of ultraviolet (UV) rays, to transfer a meticulously designed pattern onto a photosensitive material. This photosensitive material, often a photoresist or light-sensitive polymer, is evenly coated onto the paper substrate, forming a blank canvas awaiting intricate details. The pivotal component of the process is a photomask, a meticulously crafted guide that maps out the pattern to be transferred onto the paper. These photomasks are born of computer-aided design (CAD) software, each possessing transparent and opaque regions corresponding to the desired sensor features.
The alchemy of photolithography unfolds as the coated paper is exposed to the brilliance of UV light, a moment of transformation. The areas of the paper exposed to this luminous energy undergo a chemical metamorphosis, typically in the form of cross-linking or dissolution processes, depending on the specifics of the design.117 The shaded areas, however, remain unchanged, forming the negative of the intended pattern. After the illumination, a developer solution enters the stage, removing the unexposed or uncross-linked regions. This development reveals the precise and intricate sensor features, etched onto the paper like an artistic masterpiece.
The beauty of photolithography extends beyond pattern creation; it encapsulates a world of possibilities. Post-patterning, the paper becomes a canvas for functionalization with specific chemicals or materials to facilitate the sensor's primary function – the detection of target analytes. This could involve the introduction of enzymes, nanoparticles, or conductive materials that breathe life into the paper sensors, allowing them to interact with the chosen analytes.117
The symphony of photolithography unfolds with precision and exactitude, granting paper sensors well-defined patterns that underpin their ability to deliver accurate and reliable sensing.118
3.5.1 Advantages and disadvantages of photolithography.
This technique is not only precise but also scalable, making it suitable for the mass production of sensors. Furthermore, it offers excellent repeatability, a critical aspect in ensuring consistent sensor performance, thereby marking it as a stalwart in the world of advanced paper sensors.119
But if we discuss its drawbacks, the acquisition and upkeep of photolithography equipment can be costly, which limits its availability for small-scale or low-budget projects like those using paper sensors.120 Further, Photolithography operations necessitate a regulated setting with specific tools, materials, and skilled workers. Incorporating this intricacy into the production of paper-based sensors could not be practical or economical. Photoresists and etchants used in photolithography are frequently incompatible with the materials used in paper sensors.121 These compounds' chemical interactions with the paper substrate may result in unintended reactions or a decline in sensor function. In addition to this, if photolithography is not done correctly, the usage of different chemicals and processes could have an adverse effect on the environment.122 The use of these methods to the production of paper sensors may give rise to questions regarding sustainability and the management of chemical waste.
3.6 Laser cutting: crafting precision and innovation in paper-based devices
Laser cutting has emerged as a formidable tool for creating meticulously designed patterns and intricate microfluidic channels on paper, giving rise to a new era of precise and rapid fabrication in the world of paper-based sensors. In 2018, Mahmud et al. introduced an innovative concept where they harnessed the precision of laser technology to fabricate microfluidic paper-based analytical devices (μPADs) capable of measuring the smallest feature sizes essential for the unhindered flow of fluids.123 As depicted in Fig. 4, this achievement has opened up exciting possibilities in the field of paper sensors, promising to revolutionize their design and capabilities.
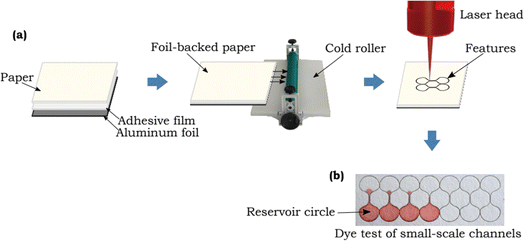 |
| Fig. 4 Schematic representation of small scale channels generated by using laser cutting method [reprinted with permission from ref. 123 Mahmud M. A., Blondeel E. J., Kaddoura M. and MacDonald B. D., Features in microfluidic paper-based devices made by laser cutting: how small can they be?, Micromachines, 2018, 9(5), 220, https://doi.org/10.3390/mi9050220. Copyright@MDPI]. | |
One of the remarkable techniques emerging from the world of laser-based fabrication involves the controlled heating of paper, pre-coated with hydrophobic materials. This inventive approach allows for the creation of aquaphobic barriers at high temperatures, effectively rendering the paper water-resistant. In 2019, Zhang et al. demonstrated their pioneering work by uniformly distributing a layer of wax on paper and utilizing laser-induced heating to engineer intricate microfluidic paths and water-resistant barriers.124 This innovative method not only showcases the adaptability of laser technology but also enhances the functionality and durability of paper-based sensors.
Furthermore, laser cutting plays a pivotal role in the creation of aquaphobic paths by removing hydrophobic materials from the paper. In 2020, Hiep et al. utilized this methodology for the quantification of nitrite, marking a significant milestone in the evolution of paper-based sensor fabrication.125
3.6.1 Advantages and disadvantages of laser cutting.
The dynamic nature of laser cutting and its ability to adapt to various sensor requirements and applications are pushing the boundaries of sensor technology.126 The integration of laser technology into sensor fabrication is ushering in a new era of precision and innovation, promising a brighter future for paper-based sensors.127
Further, it has some limitation such as material compatibility. The variety of materials can be laser-cut, however not all papers are best suited for this technique. Certain papers might have coatings or additives that, when cut with a laser, release harmful vapours that could harm the machinery or cause health problems.128 In addition to this, when laser cutting technique is used for fragile materials like paper, burnt edges may result. This may have an impact on the sensor's appearance as well as its ability to function, especially if the burnt edges weaken the paper's structural integrity or change its characteristics.129
4. Design and development of 3D paper-based sensors
The study on sensors made of paper has recently extended from sensors based on paper substrate with two dimensions (2D) to those with three dimensions (3D) coupled with different preparation techniques. In comparison to 2D sensors, more difficult multichannel analyses can be performed by 3D paper sensors.
Additionally, compared to its transverse flow, the longitudinal fluid flow device requires fewer samples and shorter flow times, allowing quicker and more effective detection and analysis. Currently, the manufacturing procedure of 3D paper-based devices mostly consists of stacking discrete 2D devices using techniques like folding and adhesion.130 In year 2008, Martinez et al. demonstrated a technique to create 3D microfluidic devices utilising dual-sided tape and decorative paper. The fluids were spread vertically and horizontally by the 3D paper-based equipment, so they could straddle one another without blending, and the samples were then dispersed to the detection area, permitting concurrent and effective detection.131 In year 2014, Wang et al. created a 3D paper-based sensor using wax printing and dual sided tape to detect several heavy metal ions concurrently.132 In year 2020, Guan et al. created a three-dimensional paper based sensor using glue adhesion, setting distinct functions on each layer to create a platform that could perform many operations including detection of blood fibrinogen and plasma separation.133 However, employing double-sided adhesive tape to construct 3D paper-based devices has some disadvantages viz. difficult alignment, bonding, and punching procedures, which have negative impact on fluid flow. Additionally, a few scientists created 3D paper sensor using origami to attain numerous tests and simultaneous shunting.134 In year 2016, Ding et al. constructed a 3D equipment using folding technique. The equipment could be utilised for a variety of bioassays by unfold and fold the structure of paper.135 In year 2022, Lazzarini et al. created a 3D paper sensor using origami and wax printed ink for the detection of allergens in food sample.136 It describes a sensor made of origami paper that uses magnetic microbeads to functionalize the paper substrate and conducts a competitive chemiluminescence immunoassay for ovalbumin in food samples. The schematic representation of the sensor as shown below in Fig. 5.
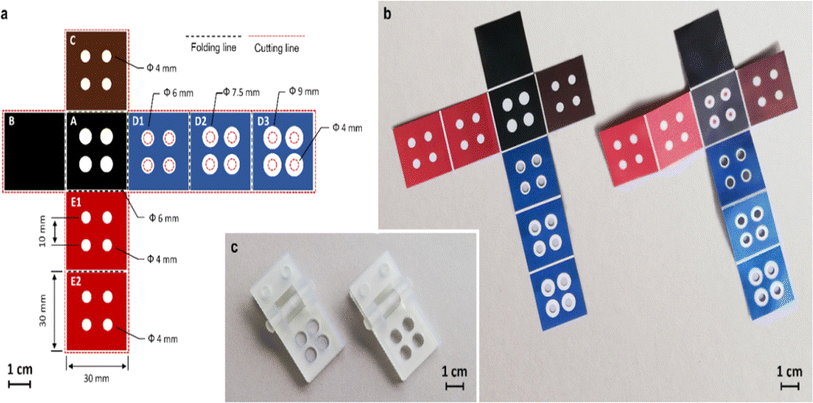 |
| Fig. 5 The schematic representation of 3D paper based sensor using origami and wax printed ink method [reprinted with permission from ref. 136 Lazzarini E., Pace A., Trozzi I., Zangheri M., Guardigli M., Calabria D. and Mirasoli M., An Origami Paper-Based Biosensor for Allergen Detection by Chemiluminescence Immunoassay on Magnetic Microbeads, Biosensors, 2022, 12(10), 825, https://doi.org/10.3390/bios12100825. Copyright@MDPI]. | |
The construction of 3D paper-based sensors using the origami technique is easy, quick, and enables various 3D shapes to execute various tasks.137 The preparation of 3D paper-based devices via stacking necessitates accurate alignment of several layers individually and appropriate interaction between hydrophilic channels in each layer.138
Researchers have developed 3D paper sensors on single paper by employing different preparation techniques. On two sides of the same piece of paper, different hydrophobic patterns are printed in order to implement the preparation process. In year 2016, He et al. used a laser-based direct writing technology to construct and made a three-dimensional (3D) structure on paper substrate, utilising the 3D structure to enable multi-step analysis.130 This approach regulates the hydrophobic barrier depth formed in the substrate by adjusting laser writing parameters. It is possible to build three-dimensional flow routes after thorough design and integration. In year 2021, Jeong et al. suggested 3D-μPAD formation on one piece of paper by managing the paper absorption of several wax inks.139 In year 2014, Li et al. suggested a novel approach for creating 3D paper sensors, instead of using sticky tape or origami. Wax printing was used to create patterns on both sides of a piece paper. By altering the printed wax's density and heating time, multi-layer patterned routes in the substrate were produced with varying wax penetration depths.140 The method reduces the amount of paper layers required to create 3D sensors, facilitating the device quality control process simpler. It is suitable for 3D paper sensors and regular production processes. In year 2018, Punpattanakul et al. effectively generated a three-dimensional network of fluid channels with many fluids channel crossing each other using inkjet printer. Inkjet printer as a shield is used to create hydrophilic design on both sides of the paper, and then submerged in a non-polar solution that contains a water-resistant material to create a barrier that repels water. The mask design assisted in separating the printed area into hydrophobic and hydrophilic regions by preventing the non-polar solution from adhering to it. The mask design assisted to avoid the non-polar solution adhering to the printed section,141 therefore make sections of the paper that are hydrophobic and hydrophilic. In conclusion, the 3D modeling method enables effective integration of additional fluid flow channels, enabling more sophisticated samples analysis on a single device.134 Currently, 3D paper-based sensors are being used for food safety, illness diagnosis and environmental monitoring research and detection.
5. Colorimetry detection method
Colorimetric detection methods are extensively used in paper designed sensors virtue of their cost-effectiveness, simplicity and ease of interpretation.142 These methods rely on changes in color to indicate the presence of a specific analyte or target molecule. Here are some common colorimetric detection techniques used in paper sensors.
5.1 Indicator dyes
Indicator dyes are molecules that undergo a color change in response to a specific analyte. These dyes can be immobilized on the paper substrate or within the detection zone. When the target analyte reacts with the indicator dye, it triggers a chemical or physical change that results in a visible color shift. Examples of indicator dyes include pH indicators (e.g., litmus paper), metal ion indicators (e.g., metallochromic dyes), and enzyme substrates. In year 2017, Gunda et al. detect the E. coli in water sample using a litmus paper test (dip test) in which the enzymatic reaction is performed on the surface of a porous paper substrate. The detection limit of E. coli was143 2 × 105 to 4 × 104 CFU mL−1. In year 2018, Idros et al. developed a multidimensional colorimetric paper based sensor for the detection heavy metal ions i.e., mercury, lead, iron, copper, nickel and chromium ions using triple indicator. The triple indicator changes color upon addition of metal ions and seen by naked eye. The detection limit for Cu2+, Hg2+, Pb2+, Ni2+, Cr2+ and Fe+ were 15, 0.1, 0.3, 0.5, 0.8 and 3.58 μM respectively.144
5.2 Nanoparticles and nanomaterials
Nanoparticles such as gold nanoparticles (AuNPs), silver nanoparticles (AgNPs), and quantum dots can be used in paper sensors. These nanoparticles exhibit unique optical properties that can be tuned to change color in the presence of specific analytes.145 Functionalized nanoparticles can interact with the analyte and cause aggregation, leading to a color shift that is visible to the naked eye. In year 2021, Borthakur et al. synthesized copper sulfide porous reduced graphene oxide nanocomposite to detect the Hg(II) ions. The paper strips dip in Hg(II) ion solution it initiated the TMB oxidation and shows the faded bluish-green color with the detection limit of 0.010 ppm.146 In the same year, Sahu et al. synthesized glucose functionalized AuNPs and deposited on paper substrate for the visual identification of arsenic (As(III)) and lead (Pb(II)) ions. The detection limits for As(III) and Pb(II) were 5.6 ppm and 7.7 ppm.34
5.3 Enzyme substrates
Enzyme-based colorimetric assays involve using enzymes that catalyse reactions causing color shifts.147 For example, in the presence of a target analyte, an enzyme–substrate complex can produce a colored product. This approach is commonly used in bioassays, where the analyte concentration determines how strongly the colour changes. In year 2023, Guan et al. developed a colorimetric paper based sensor for the real time detection of ascorbic acid based on AuNPs nanozyme activity. Its detection limit was 0.406 μM.148 In year 2023, Gao et al. fabricated a DNAzyme colorimetric paper based sensor for the highly sensitive detection of antibiotic-resistant genes in different microorganisms' pathogens. The antibiotic resistance genes can be detected in femtomolar level.149
5.4 Lateral flow assays (LFAs)
LFAs, also known as lateral flow immunoassays, are a type of paper-based sensors, which work on the basis of capillary action and are commonly used for rapid diagnostic tests.150 LFAs typically include four zones viz.; a sample application zone, a conjugate zone (where labeled antibodies or other recognition elements are immobilized), a reaction zone (where target analytes bind to the labeled antibodies), and a detection zone (where a color change occurs). In year 2017, Roh et al. developed an immunochromatographic strip using polydiacetylene on nitrocellulose membrane for the detection of Hepatitis B surface Antibody (HBsAb). A red line can be seen by naked eye on nitrocellulose membrane for up to 1 ng mL−1 of HBsAb.151 In year 2018, Loynachan et al. developed a lateral flow immunoassay (LFIAs) for the sensitive detection of p24 which is the important biomarker for HIV. The detection level of p24 is in the femtomolar range.152
Colorimetric detection methods offer several advantages, including simplicity, rapid response, and visual interpretation. They are particularly suitable for point-of-care testing, environmental monitoring, and resource-limited settings. The design of a colorimetric paper sensor depends on the specific analyte of interest and the desired sensitivity and specificity of the assay.
6. Instrument-free readouts
Instrument-free in paper sensors refer to the ability of a paper-based sensor to provide a measurable response or signal without the need for specialized or complex instrumentation. These sensors are designed to be affordable, transportable and simple, making them particularly useful for point-of-care diagnostics, environmental monitoring, and resource-limited settings. Numerous approaches have been used for this kind of analysis such as radius-based, counting, and detection based on distance.153 In any case, no external equipment is used for semiquantitative determination. In the year, 2018 Hofstetter et al., used radius-based detection technique for the detection of Cu2+, Fe2+, Fe3+, and Zn2+ metal ions using metal complexing reagents. The key benefit of radius/diameter-based detection technique was that it is quite time saving and takes only two to three minutes. Detection based on counting depends on specific components of a device to create colour. Number of coloured materials overall or wetted spots shows how much sample is there. This detection type has been utilised to measure albumin, glucose, antibodies, and hydrogen peroxide.155,156 In year 2012 Lewis et al. used the counting-based technique by developing certain compound to detect H2O2.154
Heavy metals, proteins, DNA, haematocrit levels, small molecules such as glucose, microbes have all been detected using distance-based technique.157–164 Most of the time, distance-based detection depends on an interaction between the target and chemicals that have been dried in the paper channel. Upon complete consumption of the target, colouring will not continue to develop, and the distance at which it stops can specify the targets concentration. In year 2021, Katelakha et al. fabricated a distance paper-based analytical device for the detection of lead in food products. Hydrophilic and hydrophobic barriers were produced by printing the specified pattern onto filter paper using the wax printing process. The schematic representation of distance based paper sensor for lead as shown in Fig. 6.165
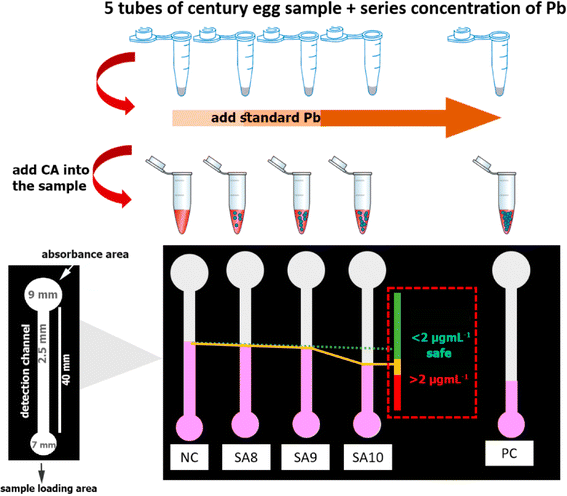 |
| Fig. 6 The schematic representation of distance based paper sensor to identify lead in food samples [reprinted with permission from ref. 165 Katelakha K., Nopponpunth V., Boonlue W. and Laiwattanapaisal W., A simple distance paper-based analytical device for the screening of lead in food matrices, Biosensors, 2021, 11(3), 90, https://doi.org/10.3390/bios11030090. Copyright@MDPI]. | |
In year 2023, Giménez-Gómez et al. created a distance paper based colorimetric sensor for the real time monitoring of dissolved inorganic carbon (DIC) concentration in freshwater. The measurement of DIC concentration between166 50 to 1000 mg L−1. Table 5 shows the various analytes to be detected using paper sensor with distance based detection.
Table 5 The various analytes to be detected using paper sensor with distance based detection
S. no. |
Analyte |
Sample |
Linearity range |
Detection limit |
Ref. |
1 |
Cocaine |
Urine |
— |
1.8 μM |
167
|
2 |
Chloride |
Tap water |
— |
1.7 mg L−1 |
168
|
3 |
DNA |
Parasitic worm |
1 aM to 1 pM |
1 aM |
169
|
4 |
Potassium ion |
Serum |
1–6 mM |
1 mM |
170
|
5 |
Glucose |
Tear |
0.1–1.2 mM |
0.1 mM |
171
|
6 |
H2O2 |
— |
117.5–587.5 mM |
65.2 mM |
172
|
7 |
Chloride |
Mineral water |
— |
2 mg L−1 |
172
|
8 |
Aluminium |
Water |
100 μM–1 mM |
100 μM |
173
|
9 |
Bromide and bromate |
Water, cake, rice, flour, bread flour |
25 μg L−1 to 2 mg L−1 for Br− and 0.5–50 μg L−1 for BrO3− |
10 μg L−1 for Br− and 0.5 μg L−1 for BrO3− |
174
|
10 |
KIO3 |
Milk and salt |
0.05–0.5 mM |
0.05 mM |
175
|
11 |
Tartaric acid |
Wine |
2.5–150 mm |
2.5 mm |
176
|
12 |
Lead ion |
Gunshot residue |
50–500 mg L−1 |
50 mg L−1 |
177
|
13 |
RBCs |
Whole blood |
28–57% |
— |
178
|
14 |
Lactoferrin |
Tear |
0.1–4 mg mL−1 |
0.1 mg mL−1 |
179
|
15 |
Nickel |
Combustion ash |
0.7–92 μg m−3 |
— |
180
|
Instrument-free readouts in paper-based sensors are advantageous for their ease of use, low cost, and portability. They enable rapid and accessible testing in diverse scenarios, from medical diagnostics to environmental monitoring and food safety.
7. Applications of paper-based sensors
Paper-based sensors have gained significant attention in recent years due to their numerous advantages, including low cost, portability, biodegradability, and ease of fabrication. These sensors have found applications in various fields, and some of the key applications include.
7.1 Medical diagnostics
Paper sensors have gained significant attention in recent years for their potential applications in various fields, including medical diagnostics. These sensors are typically inexpensive, easy to fabricate, portable, and environmentally friendly, making them attractive for point-of-care testing and resource-limited settings.181 Here are some medical diagnostic applications of paper sensors.
7.1.1 Glucose monitoring.
Sensors based on paper substrate can be developed to measure the glucose levels in blood, similar to traditional glucose test strips. The paper-based sensors may contain reagents that changes color in glucose presence, allowing individuals with diabetes to monitor their blood sugar levels easily. In year 2017, Gabriel created a colorimetric paper-based sensor using the wax printing method and 3,3′,5,5′-tetramethylbenzydine (TMB) reagent for the detection of glucose in human tear sample as shown in Fig. 7.182 The detection limit of glucose was 84 AU mM−1 and 50 μM and the linear concentration range of glucose was between 0.1 and 1.0 mM.
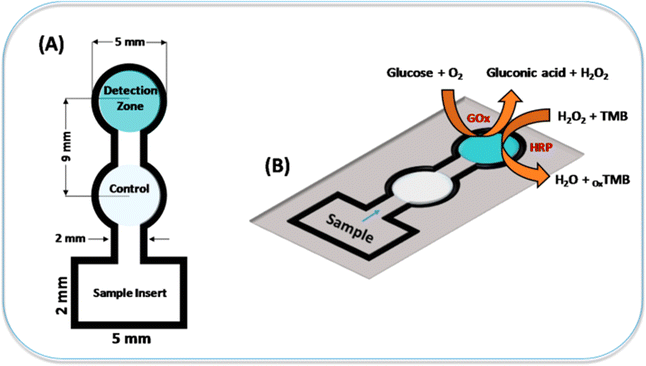 |
| Fig. 7 The representation of colorimetric sensor to detect glucose using a paper substrate [reprinted with permission from ref. 182 Gabriel E. F. M., Garcia P. T., Lopes F. M. and Coltro W. K. T., Based colorimetric biosensor for tear glucose measurements, Micromachines, 2017, 8(4), 104. Copyright@MDPI]. | |
In year 2019, Park et al. fabricated a colorimetric 3D paper based microfluidic sensor with plasma separation membranes for the detection of glucose in whole blood. The detection limit of glucose in whole blood was 0.3 mM.183 In year 2019, Erenas et al. fabricated a combined microfluidic sensor of thread and paper for the colorimetric determination of glucose in blood sample. The 3 μL of whole blood sample is only used for the detection of glucose.184 In year 2023, Khachornsakkul et al. created an enzymatic free colorimetric paper based sensor using nanoparticles for the monitoring of glucose and diagnosis of diabetes in humans. The gold nanoparticles are deposited on one end of paper substrate for oxidation of glucose and silver nanoparticles on other end for colorimetric detection. The limit of detection was 340 μM.185
7.1.2 Urinalysis.
Paper sensors can be used to perform basic urinalysis tests, such as detecting the presence of proteins, glucose, ketones, and other substances in urine. This can aid in the diagnosis of various conditions, including kidney disorders and diabetes. In year 2019, Abarghoei et al. developed a colorimetric paper based sensor based on gold nanoparticles for the detection of citrate, which are employed in identifying the phases of prostate tumours and diagnosing prostate cancer. The detection limit of citrate found in urine sample was186 0.4 mmol L−1. In year 2021, Lewinska et al. created a colorimetric sensor using paper substrate for the analysis of creatinine in artificial urine. Its detection limit was187 0.27 mmol L−1. In year 2021, Mukhopadhyay et al. fabricated a colorimetric sensor on paper substrate for the same time detection of ketone and glucose in urine samples, which are important biomarkers for the identification of diabetic ketoacidosis (DKA). The detection limit for ketone and glucose was 1 mM.188 In year 2021, Rahbar et al. created a colorimetric immunosensor as a new generation of point-of-care platforms that use paper substrate to detect the human chorionic gonadotrophin (hCG) hormone in urine samples and then showing whether a pregnancy is positive or negative. The detection limit of hCG hormone was189 10 ng mL−1.
7.1.3 Saliva testing.
Paper sensors can be designed to analyze components in saliva, such as biomarkers or pathogens. This could be valuable for diagnosing oral health conditions, infectious diseases, and monitoring certain hormonal levels. In year 2021, Pomili et al. created a multiplexed colorimetric paper based sensor for the simultaneously detection of three salivary biomarkers i.e., lactate, glucose and cholesterol. The schematic representation of sensor as depicted in Fig. 8 in which the detection zones were loaded with gold nanoparticles and their respective enzymes and shows the blue to pink color change.190
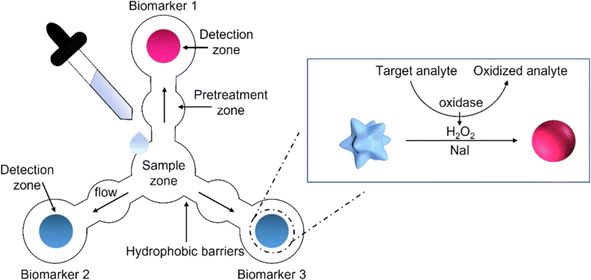 |
| Fig. 8 The schematic representation of paper based sensor for the simultaneously detection of three salivary biomarkers [reprinted with permission from ref. 190 Pomili T., Donati P., Pompa P. P., Paper-based multiplexed colorimetric device for the simultaneous detection of salivary biomarkers, Biosensors, 2021, 11, https://doi.org/10.3390/bios11110443. Copyright@MDPI]. | |
In year 2021, Davidson et al. created a colorimetric paper based sensor that uses loop-mediated isothermal amplification (LAMP) to identify pathogen nucleic acids in complicated samples. This paper based sensor is used to detect the SARS-CoV-2 in human saliva without preprocessing. The detection limit was191 200 genomic copies μL−1. In year 2021, Pungjunun et al. created a microcapillary grooved colorimetric paper sensor for analysis of thiocyanate present in saliva. The detection limit of thiocyanate was 0.2 mM.192Table 6 shows various analytes detection in saliva using paper based colorimetric sensor.
Table 6 Various analytes detection in saliva using paper based colorimetric sensor
S. no. |
Materials & structures |
Fabrication methods |
Analyte |
Detection limit |
Ref. |
1 |
Filter paper, 2D |
Wax printing |
Glucose |
0.37 dg mL−1 |
193
|
2 |
Filter paper, 3D |
Wax printing + laser cutting |
Glucose |
0.09 mM |
194
|
3 |
Filter paper, 2D |
Wax printing |
Glucose |
0.3 mM |
195
|
Nitrite |
14.8 μM |
4 |
Filter paper, 2D |
PMMA masking |
Nitrite |
7.8 μM |
196
|
5 |
Filter paper, 2D and 3D |
Inkjet printing + screen printing |
Thiocyanate nitrite |
1 mM |
197
|
0.2 mM |
6 |
Filter paper, 3D |
Cutting + gel bonding |
Nitrite |
0.05 μM |
198
|
Nitrate |
80 μM |
7 |
Filter paper, 3D |
Wax printing |
H1N1 |
1.34 PFU mL−1 |
199
|
8 |
Filter paper, 2D |
Cutting |
Hepatitis B |
104 copies mL−1 |
200
|
9 |
Filter paper, 3D |
Laser cutting |
Tuberculosis |
100 bacteria mL−1 |
201
|
10 |
Filter paper, 2D |
Cutting |
Cyanide |
0.053 mg L−1 |
202
|
11 |
Filter paper, 2D |
Cutting |
Cu2+ |
1.2 ppm |
203
|
12 |
Filter paper, 3D |
Laser jet printing |
Urea |
0.18 mg dL−1 |
204
|
Ammonia |
0.03 mg dL−1 |
CO2 |
0.06 mg dL−1 |
7.2 Food monitoring and food safety
When it comes to food monitoring and food safety, paper sensors offer several promising applications.
7.2.1 Food contaminant detection.
Paper sensors can be designed to detect contaminants such as pathogens (e.g., bacteria, viruses),205 chemical residues (e.g., pesticides, antibiotics), and adulterants (e.g., melamine in milk). The sensor's components are chosen to specifically interact with the target contaminants, resulting in color changes or other visual signals that can be easily interpreted by users. In year 2019, Govindarajalu et al. fabricated a portable colorimetric sensor on cellulosic paper for the detection of starch in milk.206 In year 2021, Dena et al. used bromothymol blue (BTB) and bromocresol purple (BCP) to construct lab-on-paper colorimetric sensor for bacterial meat deterioration.207Table 7 highlights some colorimetric paper sensors for detecting adulterants in different useful products.
Table 7 The paper-based colorimetric sensors used for detection of adulterants in different useful products
S. no. |
Source of analysis |
Adulterants |
Paper substrate |
Detection limit |
Ref. |
1 |
Milk samples |
17β-Estradiol |
Whatman AE99 |
0.25 μg L−1 |
208
|
2 |
Alcoholic beverages |
Ketamine |
Whatman filter paper no. 1 |
0.001 M |
209
|
3 |
Milk samples |
Clenbuterol |
Whatman cellulose chromatography paper |
0.2 ppb |
210
|
4 |
Milk samples |
Melamine |
Common filter paper |
5.1 nM |
211
|
5 |
Milk samples |
Urea |
Filter paper |
Urea-5 mg |
212
|
Starch |
Starch-17 mg |
Salt |
Salt-29 mg |
Detergent |
Detergent- 20 mg in 10 mL of milk |
6 |
Milk samples |
Milk adulterants |
Whatman filter paper no. 1 |
NA |
213
|
7 |
Commercial food samples |
Benzoic acid |
Whatman qualitative filter paper no. 3 |
Multiple values for the different samples |
214
|
8 |
Wheat flour |
Iron fortificant |
Whatman 1PS paper |
3.691 μg mL−1 |
215
|
9 |
Milk samples |
Starch |
Whatman qualitative 1 |
NA |
206
|
10 |
Milk samples |
Melamine |
Chromatographic paper |
0.1 ppm |
216
|
11 |
Food samples |
Sulphite |
Cellulose paper |
0.04 μg L−1 |
217
|
12 |
Fish (salmon) |
Mercury |
— |
15 nM |
218
|
13 |
Food products |
Nitrite and nitrate |
Whatman qualitative filter paper grade 1 and grade 5 |
0.1 and 0.4 mg L−1 |
219
|
14 |
Food samples |
Borax |
Whatman filter paper no. 1 |
10 mg L−1 |
220
|
Salicylic acid |
35 mg L−1 |
Nitrite and nitrate |
0.4 mg L−1 |
15 |
Meat samples |
Nitrite |
Whatman qualitative filter paper 1, 2, 4, 5 and 42 |
1.1 mg kg−1 of meat |
221
|
7.2.2 Freshness and shelf-life assessment.
Enzymes and indicators can be integrated into paper sensors to assess the freshness and spoilage of food products.222 For example, enzymatic reactions that produce color changes as food deteriorates can be incorporated onto the paper, allowing consumers to determine if a product is still safe to consume. In year 2017, Chen et al. demonstrate a low-cost approach that uses the barcode on the meal as a colorimetric sensor array to keep track of the food condition. This colorimetric sensor is used for monitoring chicken aging and eventual spoiling at various temperatures.223 In year 2018, Chen et al. developed a newly colorimetric food package label to check the freshness of lean pork. These indicator packaging label consisting the three different pH-sensitive dyes groups, including a mixture of bromothymol blue and methyl red, bromocresol purple and bromothymol blue.224 In the same year, the same group constructed a colorimetric indicator label for checking the freshness of packed fresh-cut green bell pepper based on pH responsive dyes. The indicator label made of methyl red and bromothymol blue mixture solution was used to check the pepper decay and it shows the color change from yellow-green to orange.225
7.2.3 Foodborne pathogen monitoring.
Paper sensors can be designed to detect specific pathogens responsible for foodborne illnesses, such as Salmonella, E. coli, and Listeria. The sensors can utilize antibodies or DNA probes that bind to target pathogen biomarkers, leading to a visible signal upon binding. In year 2019, Kim et al. developed a paper-based diagnostic device for point-of-need testing, used duplex coloration to detect fecal-indicating Escherichia coli (E. coli) and highly pathogenic E. coli. The target bacteria 10 cfu mL−1 could be detected in milk sample.226 In year 2020, Asif et al. fabricating an analytical device on paper to detect the Escherichia coli (E. coli), Staphylococcus aureus (S. aureus) and their antibiotic resistant in milk samples using chromogenic substrate. Detection limits were found227 to be 106 cfu mL−1.
7.2.4 Point-of-care testing (POCT).
For food handlers and regulatory bodies, paper sensors can serve as efficient point-of-care testing tools to rapidly screen food samples for potential safety hazards, leading to quicker decision-making and improved food safety management as detailed in Table 8.
Table 8 Emerging point-of care (POC) colorimetric sensor for food safety testing
POC sensors |
Sample type |
Analytes |
Assay time |
Limit of detection |
Ref. |
Paper-based sensor |
Chinese cabbage |
E. coli O157: H7 |
1 h |
10 000 CFU mL−1 |
228
|
Milk |
Clenbuterol |
1 h |
0.2 ppb |
229
|
Water |
Cronobacter spp. |
1 h |
10 cfu cm−2 |
230
|
Water |
NO2− |
5 min |
0.5 nM |
231
|
Water |
Benzoic acid |
1 h |
500 ppm |
232
|
Water, tomato juices |
Copper ions |
2 min |
0.3 ng mL−1 |
233
|
Phosphate buffered saline, milk, water and apple juice |
E. coli O157:H7 |
35 min |
10 CFU mL−1 |
234
|
Paper-based sensor |
Milk |
Alkaline phosphate |
10 min |
0.1 U L−1 |
235
|
Water |
Nitrite |
15 min |
73 ng mL−1 |
236
|
Water |
Pseudomonas aeruginosa
|
50 min |
200 CFU mL−1 |
237
|
Milk, water, spinach |
E. coli
|
1 h |
10–1000 CFU mL−1 |
238
|
Milk, juice |
Salmonella typhimurium
|
1 h |
100–1000 CFU mL−1 |
239
|
|
Phosphate buffered saline |
(i) E. coli |
10 min |
(i)105 cfu mL−1 |
240
|
(ii) S. typhimurium |
i(i)106 cfu mL−1 |
Chip-based sensor |
Corn |
Aflatoxin B1 |
1 h |
3 ppb |
241
|
(i) Wheat |
(i) Gluten |
15–20 min |
(i) 4.7 ng mL−1 |
242
|
(ii) Peanut |
(ii) Ara h |
(ii) 15.2 ng mL−1 |
Water |
(i) Pb(II) ion |
8–10 min |
(i) 30 ppb |
243
|
(ii) Al(III) ion |
(ii) 89 ppb |
Apple |
Malathion |
20 min |
100 ppb |
244
|
Water |
Tetrabromodiphenyl ether |
12 min |
0.01 μg L−1 |
245
|
It is important to note that while paper sensors offer numerous advantages, they may not always replace traditional laboratory methods for highly sensitive or complex analyses. However, they can complement existing techniques, especially in resource-limited settings or for rapid preliminary screening.
7.3 Environmental monitoring and control
7.3.1 Water quality monitoring.
Paper sensors can be designed to detect and quantify pollutants in water sources, such as heavy metals ions (e.g., lead, mercury, cadmium), pH levels, nitrate, and microbial contaminants.246 These sensors can provide real-time monitoring of water quality in both natural bodies of water and water treatment facilities. In year 2014, Wang et al. designed a paper colorimetric sensor using dual-sided tape layers of wax-patterned paper to identify metals ions in water samples with high selectivity.132 In year 2018, Idros et al. prepared an affordable, portable, paper-based microfluidic analytical device, for the detection of Pb(II), Cr(VI), Hg(II), Cu(II), Fe(II) and Ni(II) ions.144 Colorimetric indicators resulted observable colour change when metal ions were applied. Red, green, and blue (RGB) RGB's colour properties in three dimensions were quantitatively analysed by digital imaging and colour calibration techniques.246 In year 2019, Firdaus et al. developed a low cost, straightforward, and portable analytical technique paired with a smartphone for quantifying mercury ions. Silver nanoparticles (AgNPs) in small quantities were used as a colorimetric agent, when mercury ions were added on the AgNPs the yellowish-brown color changed to colourless. It was detected over a lower limit of 0.86 ppb. The schematic representation for the fabrication procedure and acquisition of digital images for determination of mercury ions are shown in Fig. 9.247
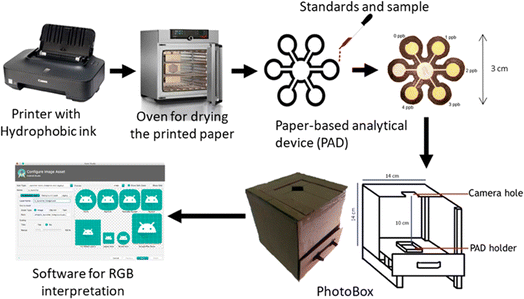 |
| Fig. 9 The schematic representation for the fabrication procedure and acquisition of digital images for determination of mercury ions [reprinted with permission from ref. 247 Firdaus M. L., Aprian A., Meileza N., Hitsmi M., Elvia R., Rahmidar L. and Khaydarov R., Smartphone coupled with a paper-based colorimetric device for sensitive and portable mercury ion sensing, Chemosensors, 2019, 7(2), 25, https://doi.org/10.3390/chemosensors7020025. Copyright@MDPI]. | |
In year 2020, Moniz et al. used 3-hydroxy-4-pyridinone chelators produced from ether as chromogenic reagents, constructed a paper-based microfluidic device to detect Fe(III) ions in water samples. Utilising this new colour reagent increased the device detection limit, the detecting signal remained constant for four hours and the device can be stored for a minimum of one month.248
7.3.2 Air pollution detection.
Paper-based sensors can be used to monitor air pollutants, including gases like nitrogen dioxide (NO2), sulphur dioxide (SO2), carbon monoxide (CO), and volatile organic compounds (VOCs). These sensors can be deployed in urban areas to assess air quality and pollution levels. In year 2012, Wang et al. developed a colorimetric sensor that combine with microfluidic to detect the total NOx (NO + NO2). The created sensor could continually track the quality of the air for up to 18 hours with a detection limit of 50 parts per billion per volume (ppbV).249 In year 2016, Tang and Sun et al. have created a colorimetric sensor made of paper with a high sensitivity for airborne methyl isothiocyanate (MITC) detection. The detection limit was 100 ppb.250 In year 2018, Guo et al. created a microfluidic colorimetric sensor to analyse the gaseous formaldehyde using 4-aminohydrazine-5-mercapto-1,2,4-triazole (AHMT) technique. The detection limit was 0.01 ppm. The schematic representation of the fabricated microfluidic sensor with their calibration is shown in Fig. 10.251
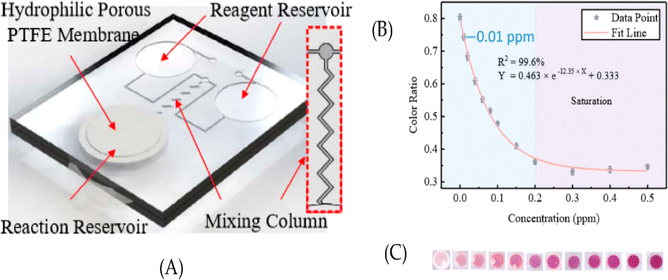 |
| Fig. 10 The schematic representation of the fabricated microfluidic sensor with their calibration [reprinted with permission from ref. 251 Guo X. L., Chen Y., Jiang H. L., Qiu X. B. and Yu D. L., Smartphone-based microfluidic colorimetric sensor for gaseous formaldehyde determination with high sensitivity and selectivity, Sensors, 2018, 18(9), 3141, https://doi.org/10.3390/s18093141. Copyright@MDPI]. | |
In year 2019, Nguyen et al. created a disposable paper-based sensor for chemically detecting gaseous with high selective and accuracy. Seven rings were used in the probe designed, which was wax-printed and coloured with various pH indicators. The probes colour was evaluated and RGB values were retrieved from pictures obtained with a handmade smart-phone applications.252
These applications highlight the versatility and potential impact of paper-based sensors in environmental monitoring and control. Table 9 highlights the paper based colorimetric sensor for the detection of pollutants found in environment monitoring. However, it's important to note that while paper-based sensors offer many advantages, they also have limitations, such as sensitivity and durability. Therefore, the choice of sensor technology should be carefully considered based on the specific monitoring requirements and environmental conditions.
Table 9 The various paper-based colorimetric sensors used for the detection of pollutants found in the environment monitoring
S. no. |
Type of paper |
Analyte |
Methods of fabrication |
Detection limit |
Ref. |
1 |
Chromatography paper |
Explosive residue |
Wax ink |
0.39 μg |
253
|
2 |
Filter paper |
Br− |
Wax printing |
11.25 nM |
254
|
Nd3+ |
0.65 nM |
As3+ |
11.53 nM |
3 |
Filter paper |
Reactive phosphate |
Inkjet printing |
0.05 M |
255
|
4 |
Filter paper |
Ammonia |
Inkjet printing |
0.8 mg N per L |
256
|
5 |
Filter paper |
Acid volatile sulphides |
Cutting |
0.1 μmol g−1 |
257
|
6 |
Filter paper |
S. chartarum
|
Wax printing |
10 spores per mL |
258
|
7 |
Filter paper |
Methyl isothiocyanate |
N/R |
100 ppb |
250
|
8 |
Filter paper |
Bisphenol A |
Inkjet printing |
0.28 μg g−1 |
259
|
9 |
Filter paper |
Hypochlorite |
N/R |
2 g Cl2 per L |
260
|
10 |
Filter paper |
Chromium |
Wax printing |
0.12 μg |
261
|
11 |
Filter paper |
Methyl-paraoxon |
Screen printing |
8 ng mL−1 |
262
|
Chlorpyrifos-oxon |
5.3 ng mL−1 |
12 |
Filter paper |
Paraoxon |
Inkjet printing |
0.01 ng mL−1 |
263
|
Trichlorfon |
0.04 ng mL−1 |
13 |
Filter paper |
Methiocarb |
Wax printing |
5 ng mL−1 |
264
|
14 |
Filter paper |
Carbofuran dichlorvos carbaryl |
Punching |
0.003 ppm |
265
|
0.3 ppm |
0.5 ppm |
Paraoxon |
0.6 ppm |
Pirimicarb |
0.6 ppm |
15 |
Filter paper |
Methyl paraoxon |
Jet printing |
10 μM |
266
|
16 |
Art paper |
Pb2+ |
Microcontact printing |
10 nM |
267
|
17 |
Filter paper |
Pb2+ |
CO2 laser cutting |
0.7 nM |
268
|
18 |
Filter paper |
Ag+ |
Hand drawn |
10 μM |
269
|
19 |
Filter paper |
Cu2+ |
Inkjet printing |
0.08 μM |
270
|
20 |
Filter paper |
Ni2+ |
Wax printing |
4.8 mg L−1 |
271
|
Cu2+ |
1.6 mg L−1 |
Cr2+ |
0.18 mg L−1 |
21 |
Chromatography paper |
Cr6− |
Photolithography |
30 ppm |
272
|
22 |
Chromatography paper |
Ni2− |
Wax and screen printing |
0.24 ppm |
273
|
Cr6− |
0.18 ppm |
Hg2+ |
0.19 ppm |
23 |
Chromatography paper |
Cu, Fe, Zn |
Wax printing |
0.1 ppm |
274
|
24 |
Filter paper |
Fe3+ |
Laser printer and coating |
0.2 mM |
275
|
Ni2+ |
0.4 mM |
25 |
Filter paper |
Ca2+, Mg2+ |
Wax printing |
0.5 mM |
276
|
26 |
Filter paper |
Calcium |
Hand drawn |
8.3 mg L−1 |
277
|
Magnesium |
1.0 mg L−1 |
27 |
Filter paper |
Co(II) |
Immersed |
10 mg L−1 |
278
|
28 |
Filter paper |
Cl |
Wax printing |
1.3 mg L−1 |
279
|
8. The REASSURED criteria for new POCTs and their updates
In 2002, the World Health Organization (WHO) and the Special Programme for Research and Training in Tropical Diseases (TDR) recognized the urgent need for innovative, useful, and cost-effective diagnostic methods for sexually transmitted infections in humans, especially in developing countries. ASSURED was a set of criteria that described the desired features for new POCTs. Among these requirements are affordability, sensitivity, specificity, ease of use, fast and dependable performance, equipment-free operation, and deliverability to people in need.280 The ASSURED standards have had a significant impact on the development, validation, and regulation of POCTs for human healthcare over the last decades. In response to the advancements in wireless technology and the potential for tests to be given by untrained persons, an updated set of standards known as REASSURED was established. The requirement of streamlining sample collection and facilitating real-time communication is emphasized in this updated standard.281 The REASSURED criteria are as follows: affordable (less than $10 USD for a molecular assay); sensitive (minimizing false negatives) and specific (minimizing false positives); user-friendly (requiring only two steps and minimal training); real-time connectivity (feedback for patient care and connection to surveillance systems); easy specimen collection; and environmentally friendly (non-invasive specimen collection, use of recyclable materials, and reduction of hazardous waste); fast and reliable (15–60 minutes from sample receipt to answer; withstands a range of weather and environmental conditions without refrigeration); equipment-free (or runs on solar energy or batteries); and deliverable to end users (guarantees delivery to LMIC users).282 The table below displays the various point-of-care tests together with the REASSURED criteria that were evaluated in the various LMICs.
9. Disposal of paper sensors
The right way to dispose of paper sensors relies on a number of variables, such as the kind of sensor, any chemicals or other materials it might contain, and local trash disposal laws. Here are a few broad recommendations:
Review the guidelines provided by the manufacturer: the manufacturer often includes disposal instructions with many paper sensors. You can find this information in the product paperwork or on the package. Use these instructions if they are accessible.
Divide components: if the paper sensors are made of several materials (paper, plastic, electronics, etc.), think about dividing them apart so that they may be disposed of or recycled using the proper techniques for each type of material.
Eliminate any batteries or electronic parts from the sensors: make sure that any batteries or electronic parts are taken out and disposed of appropriately in accordance with local laws.283 Programs for recycling electronics are frequently offered for the appropriate disposal of such goods.
Look for potentially dangerous materials: certain paper sensors might have chemicals or other materials that should be handled with caution. If you have any suspicions about this, find out more about the materials the sensors are made of or speak with the manufacturer for advice on appropriate disposal techniques.284
Recycling: see whether your local recycling program can accept the paper sensors if their main constituents are recyclable materials (such paper or some plastics). Prior to recycling, make sure that all non-recyclable parts are eliminated.284
Trash disposal: if recycling is not a possibility, dispose of the paper sensors in accordance with local waste disposal regulations by throwing them in the usual trash. Think about employing a garbage disposal company that conforms with laws regarding the safe handling of potentially dangerous substances.285
Chemical disposal: seek advice from waste management agencies or local authorities on appropriate disposal techniques if the sensors contain chemicals or materials that need special handling, such as biohazardous compounds.286
Incineration: incineration may be a viable disposal strategy in certain situations, particularly for sensors that contain biohazardous chemicals.287 But only authorized facilities with the necessary equipment to handle these kinds of materials securely should be used for this.
Paper sensors, like any other electrical equipment or materials, should be disposed of with consideration for the environment and local rules. It's usually a good idea to ask the manufacturer or the appropriate municipal authorities for advice if you're unclear about the correct disposal procedure.
10. Conclusion: the promise, challenges, and future prospects of paper-based sensors
In a world where innovation has led to groundbreaking advancements in various fields, paper-based sensors have emerged as a beacon of hope, offering an economical, environmentally friendly, and user-friendly alternative to traditional sensing technologies. This innovative approach in sensor design leverages the simplicity of paper and the intelligence of scientific research to create versatile and accessible tools with broad applications. In this concluding section, we reflect on the significance of paper-based sensors, the challenges they face, and the promising trends that may shape their future.
10.1 The significance of paper-based sensors
10.1.1 Economic viability.
Paper-based sensors have garnered immense attention and appreciation due to their economic feasibility. The simple materials, primarily paper and ink, are readily available and cost-effective. This affordability factor has made these sensors highly accessible, especially in resource-constrained settings. In developing countries where advanced sensor technology is often financially out of reach, paper-based sensors provide an affordable and reliable solution. They can empower local healthcare workers and first responders to conduct rapid on-site diagnostics and environmental monitoring without straining limited budgets.
10.1.2 User-friendly nature.
Designed with a focus on simplicity, paper-based sensors have revolutionized the concept of user-friendliness. They are intuitive to use and require minimal training for operation. This simplicity eliminates the need for specialized technicians or elaborate instruments, thereby democratizing access to critical diagnostic tools. These sensors are particularly valuable in emergencies and scenarios where time is of the essence, such as during disease outbreaks or environmental crises.
10.1.3 Eco-friendly attributes.
Paper-based sensors exemplify eco-friendliness in sensor technology. Unlike their electronic counterparts, which often contain hazardous materials and contribute to electronic waste, paper sensors are biodegradable. This feature aligns with global efforts to adopt more sustainable and environmentally friendly practices. The production and disposal of paper sensors are less harmful to the planet, addressing concerns about environmental impact.
10.1.4 Rapid diagnostics in healthcare.
One of the most notable applications of paper-based sensors is in the realm of rapid diagnostics. They have transformed point-of-care testing for various diseases, from infectious diseases to chronic conditions. Speed and reliability in diagnosis can be life-saving, especially in healthcare settings where immediate action is crucial. These sensors have played a pivotal role in expanding access to diagnostics, even in remote or underserved areas, by offering fast and accurate results.
10.1.5 Versatile applications.
Paper-based sensors have proven their versatility across different domains. Their applications span across healthcare, where they are used for disease detection and monitoring, to environmental monitoring for water quality testing and pollution detection. Additionally, they have made significant contributions to ensuring food safety by detecting contaminants and pathogens. These sensors are instrumental in addressing real-world challenges.
10.2 Challenges/research gaps
10.2.1 Tensile strength and stretchability.
While paper is an ideal substrate for its cost-effectiveness, it presents challenges related to tensile strength and stretchability. Ensuring that paper-based sensors can withstand challenging conditions, such as physical stress or exposure to various environmental factors, requires ongoing research and development.
10.2.2 Device adaptability.
Designing paper-based sensors that can adapt to diverse environments and scenarios is an evolving challenge. Ensuring that sensors function consistently under different conditions is critical. Researchers are working towards creating sensors that can be seamlessly integrated into various settings, from urban healthcare facilities to remote fieldwork.
10.2.3 Mass production.
As the demand for paper-based sensors grows, the need for mass production methods has become evident. Balancing cost-effectiveness and quality in scaling up production processes is a challenge. Developing scalable manufacturing methods that maintain the accuracy and effectiveness of the sensors is essential to meet the increasing demand.
10.3 Future trends
10.3.1 Improved sensitivity and selectivity.
Researchers are committed to enhancing the sensitivity and selectivity of paper-based sensors. This means that paper sensors will become capable of detecting even lower concentrations of analytes with precision. This development opens up new possibilities in fields like environmental monitoring288 and early disease detection, where detecting minute quantities of substances is paramount.
10.3.2 Integration with electronics.
The integration of electronic components into paper-based sensors is a promising direction.289 This advancement may involve incorporating microcontrollers, wireless communication, or data analysis capabilities directly into the paper substrate. By doing so, paper sensors can become more versatile and adaptable for various applications, including remote monitoring and real-time data transmission.
10.3.3 Multiplexing capabilities.
Future paper sensors may be engineered to detect multiple analytes simultaneously. This capability, known as multiplexing, can significantly enhance testing efficiency. It enables the simultaneous detection of various substances in a single test,290 streamlining processes and enabling comprehensive analysis.
10.3.4 Application diversification.
The applications of paper-based sensors could expand into various fields beyond healthcare and environmental monitoring. Industries such as agriculture, food quality control,291 and safety, as well as wearable health monitoring devices,292 stand to benefit from specialized paper-based sensors. These sensors could address unique challenges and bring cost-effective solutions to these domains.
10.3.5 Printable electronics.
Advancements in printable electronics, including conductive inks and flexible circuits, have the potential to revolutionize paper-based sensors. Researchers are exploring the possibility of printing complex electronic components directly onto paper substrates. This innovation could enable the development of more sophisticated sensors that can perform intricate tasks and provide real-time data.
10.3.6 Regulatory approval.
As paper-based sensors mature and gain wider acceptance, they may undergo regulatory approval processes to ensure their safety and reliability for use in critical applications. This step is vital in healthcare settings and any other context where the accuracy of results is paramount.
In conclusion, paper-based sensors have not only made their mark but have also ushered in a new era of possibility in the realm of sensor technology. As research continues to break new ground, the future is incredibly promising. Paper sensors are poised to become more accurate, adaptable, and applicable in various domains. With their affordability, accessibility, and eco-friendliness, paper-based sensors have the potential to revolutionize the way we approach diagnostics, monitoring, and data collection. In the coming years, we anticipate the emergence of novel solutions that will address global challenges and provide opportunities for further advancements in science and technology.
Conflicts of interest
There are no conflicts to declare.
Acknowledgements
The authors are thankful to the STEM Pioneers Training Lab, Najran, Kingdom of Saudi Arabia for supporting this work.
References
- C. Dincer, R. Bruch, E. Costa-Rama, M. T. Fernández-Abedul, A. Merkoçi, A. Manz, G. A. Urban and F. Güder, Disposable sensors in diagnostics, food, and environmental monitoring, Adv. Mater., 2019, 31(30), 1806739 CrossRef PubMed.
- V. Naresh and N. Lee, A review on biosensors and recent development of nanostructured materials-enabled biosensors, Sensors, 2021, 21(4), 1109 CrossRef CAS PubMed.
-
A. A. Ensafi, An introduction to sensors and biosensors, in Electrochemical Biosensors, ed. Ensafi, A. A., Elsevier, Cambridge, MA, USA, 1st edn, 2019, pp. 1–10 Search PubMed.
- S. Malik, J. Singh, R. Goyat, Y. Saharan, V. Chaudhry, A. Umar, A. A. Ibrahim, S. Akbar, S. Ameen and S. Baskoutas, Nanomaterials-based biosensor and their applications: A review, Heliyon, 2023, 9, e19929 CrossRef CAS PubMed.
- E. W. Nery and L. T. Kubota, Sensing approaches on paper-based devices: a review, Anal. Bioanal. Chem., 2013, 405, 7573–7595 CrossRef CAS PubMed.
- E. Noviana, T. Ozer, C. S. Carrell, J. S. Link, C. McMahon, I. Jang and C. S. Henry, Microfluidic paper-based analytical devices: from design to applications, Chem. Rev., 2021, 121(19), 11835–11885 CrossRef CAS PubMed.
- C. T. Kung, C. Y. Hou, Y. N. Wang and L. M. Fu, Microfluidic paper-based analytical devices for environmental analysis of soil, air, ecology and river water, Sens. Actuators, B, 2019, 301, 126855 CrossRef CAS.
- W. Zhao, M. M. Ali, S. D. Aguirre, M. A. Brook and Y. Li, based bioassays using gold nanoparticle colorimetric probes, Anal. Chem., 2008, 80(22), 8431–8437 CrossRef CAS PubMed.
- E. Noviana, D. B. Carrão, R. Pratiwi and C. S. Henry, Emerging applications of paper-based analytical devices for drug analysis: A review, Anal. Chim. Acta, 2020, 1116, 70–90 CrossRef CAS PubMed.
- K. Mao, X. Min, H. Zhang, K. Zhang, H. Cao, Y. Guo and Z. Yang, based microfluidics for rapid diagnostics and drug delivery, J. Controlled Release, 2020, 322, 187–199 CrossRef CAS PubMed.
- N. Choudhury, B. Ruidas, C. D. Mukhopadhyay and P. De, Rhodamine-appended polymeric probe: an efficient colorimetric and fluorometric sensing platform for Hg2+ in aqueous medium and living cells, ACS Appl. Polym. Mater., 2020, 2(11), 5077–5085 CrossRef CAS.
- J. Fu, J. Zhu, Y. Tian, K. He, H. Yu, L. Chen, D. Fang, D. Jia, J. Xie, H. Liu and J. Wang, Green and transparent cellulose nanofiber substrate-supported luminescent gold nanoparticles: A stable and sensitive solid-state sensing membrane for Hg (II) detection, Sens. Actuators, B, 2020, 319, 128295 CrossRef CAS.
- P. Abdollahiyan, M. Hasanzadeh, P. Pashazadeh-Panahi and F. Seidi, Application of Cys A@ AuNPs supported amino acids towards rapid and selective identification of Hg (II) and Cu (II) ions in aqueous solution: An innovative microfluidic paper-based (μPADs) colorimetric sensing platform, J. Mol. Liq., 2021, 338, 117020 CrossRef CAS.
- L. Chen, X. Tian, D. Xia, Y. Nie, L. Lu, C. Yang and Z. Zhou, Novel colorimetric method for simultaneous detection and identification of multimetal ions in water: Sensitivity, selectivity, and recognition mechanism, ACS Omega, 2019, 4(3), 5915–5922 CrossRef CAS PubMed.
- S. Dey, A. Kumar, A. Mahto, I. H. Raval, K. M. Modi, S. Haldar and V. K. Jain, Oxacalix [4] arene templated silver nanoparticles as dual readout sensor: Developing portable kit for rapid detection of methylmercury and its speciation, Sens. Actuators, B, 2020, 317, 128180 CrossRef CAS.
- A. A. Azmi, A. I. Daud, W. M. Khairul, S. Hamzah, W. W. Khalik and N. H. Hairom, Silica–silver core–shell nanoparticles incorporated with cellulose filter paper as an effective colorimetric probe for mercury ion detection in aqueous media: Experimental and computational evaluations, Environ. Nanotechnol., Monit. Manage., 2023, 19, 100762 CAS.
- H. Li, W. Wang, Z. Wang, Q. Lv, H. Bai and Q. Zhang, Analyte-enhanced photocatalytic activity of CdSe/ZnS quantum dots for paper-based colorimetric sensing of Hg2+ under visible light, Microchem. J., 2021, 164, 106037 CrossRef CAS.
- A. Kumar, A. Bera and S. Kumar, A smartphone-assisted sensitive, selective and reversible recognition of copper ions in an aqueous medium, ChemistrySelect, 2020, 5(3), 1020–1028 CrossRef CAS.
- H. Sharifi, J. Tashkhourian and B. Hemmateenejad, A 3D origami paper-based analytical device combined with PVC membrane for colorimetric assay of heavy metal ions: Application to determination of Cu (II) in water samples, Anal. Chim. Acta, 2020, 1126, 114–123 CrossRef CAS PubMed.
- R. E. Dong, P. Kang, X. L. Xu, L. X. Cai and Z. Guo, Cation-exchange strategy for a colorimetric paper sensor: Belt-like ZnSe nanoframes toward visual determination of heavy metal ions, Sens. Actuators, B, 2020, 312, 128013 CrossRef CAS.
- T. Sannok, K. Wechakorn, J. Jantra, N. Kaewchoay and S. Teepoo, Silica nanoparticle–modified paper strip–based new rhodamine B chemosensor for highly selective detection of copper ions in drinking water, Anal. Bioanal. Chem., 2023, 415(19), 4703–4712 CrossRef CAS PubMed.
-
W. Sharmoukh, M. S. Abdelrahman, S. Elkhabiry and T. Khattab, Novel Metallochromic Hydrazone-Based Chemosensor Toward Colorimetric Paper Strip For Selective Detection Cu2+ Search PubMed.
- M. Geetha, K. K. Sadasivuni, M. Al-Ejji, N. Sivadas, B. Bhattacharyya, F. N. Musthafa, S. Alfarwati, T. J. Promi, S. A. Ahmad, S. Alabed and D. A. Hijazi, Design and development of inexpensive paper-based chemosensors for detection of divalent copper, J. Fluoresc., 2023, 33(6), 2327–2338 CrossRef CAS PubMed.
- N. A. Pungut, H. M. Saad, K. S. Sim and K. W. Tan, A turn on fluorescent sensor for detecting Al3+ and colorimetric detection for Cu2+: Synthesis, cytotoxicity and on-site assay kit, J. Photochem. Photobiol., A, 2021, 414, 113290 CrossRef CAS.
- H. M. Zhai, T. Zhou, F. Fang and Z. Y. Wu, Colorimetric speciation of Cr on paper-based analytical devices based on field amplified stacking, Talanta, 2020, 210, 120635 CrossRef CAS PubMed.
- R. Rajamanikandan, M. Ilanchelian and H. Ju, Smartphone-enabled colorimetric visual quantification of highly hazardous trivalent chromium ions in environmental waters and catalytic reduction of p-nitroaniline by thiol-functionalized gold nanoparticles, Chemosphere, 2023, 340, 139838 CrossRef CAS PubMed.
- H. Tabani, F. Dorabadi Zare, W. Alahmad and P. Varanusupakul, Determination of Cr (III) and Cr (VI) in water by dual-gel electromembrane extraction and a microfluidic paper-based device, Environ. Chem. Lett., 2020, 18(1), 187–196 CrossRef CAS.
- A. Muhammed, A. Hussen, M. Redi and T. Kaneta, Remote investigation of total chromium determination in environmental samples of the kombolcha industrial zone, Ethiopia, using microfluidic paper-based analytical devices, Anal. Sci., 2021, 37(4), 585–592 CrossRef CAS PubMed.
- H. Tabani, K. Khodaei, P. Varanusupakul and M. Alexovič, Gel electromembrane extraction: Study of various gel types and compositions toward diminishing the electroendosmosis flow, Microchem. J., 2020, 153, 104520 CrossRef CAS.
-
A. Tantayanon, J. Temsangsukmanee and T. Assawawinyadet, Based Sensor for Preliminary Screening of Lead in Industrial Wastewater Search PubMed.
- N. Khunkhong, N. Kitchawengkul, Y. Wongnongwa, S. Jungsuttiwong, T. Keawin, V. Promarak, P. Nalaoh, K. Suttisintong, K. Chansaenpak and P. Jarujamrus, A novel spirooxazine derivative as a colorimetric probe for Fe2+ and Pb2+ determination on microfluidic paper-based analytical device (μPAD) for maintaining in photochromic efficiency, Dyes Pigm., 2023, 208, 110869 CrossRef.
- C. A. Chen, H. Yuan, C. W. Chen, Y. S. Chien, W. H. Sheng and C. F. Chen, An electricity-and instrument-free infectious disease sensor based on a 3D origami paper-based analytical device, Lab Chip, 2021, 21(10), 1908–1915 RSC.
- X. Xie, M. Pan, L. Hong, K. Liu, J. Yang, S. Wang and S. Wang, An “off–on” rhodamine 6G hydrazide-based output platform for fluorescence and visual dual-mode detection of lead (II), J. Agric. Food Chem., 2021, 69(25), 7209–7217 CrossRef CAS PubMed.
- B. Sahu, R. Kurrey, M. K. Deb, K. Shrivas, I. Karbhal and B. R. Khalkho, A simple and cost-effective paper-based and colorimetric dual-mode detection of arsenic (iii) and lead (ii) based on glucose-functionalized gold nanoparticles, RSC Adv., 2021, 11(34), 20769–20780 RSC.
- X. Xiong, J. Zhang, Z. Wang, C. Liu, W. Xiao, J. Han and Q. Shi, Simultaneous multiplexed detection of protein and metal ions by a colorimetric microfluidic paper-based analytical device, BioChip J., 2020, 14, 429–437 CrossRef CAS PubMed.
- Y. Chen, A. Nilghaz, R. Liu, S. Liu, L. Li, Y. Kong, X. Wan and J. Tian, Suppressing infiltration and coffee-ring effects of colorimetric reagents on paper for trace-level detection of Ni (II), Cellulose, 2023, 30(8), 5273–5288 CrossRef CAS.
- T. T. Tsai, C. Y. Huang, C. A. Chen, S. W. Shen, M. C. Wang, C. M. Cheng and C. F. Chen, Diagnosis of tuberculosis using colorimetric gold nanoparticles on a paper-based analytical device, ACS Sens., 2017, 2(9), 1345–1354 CrossRef CAS PubMed.
- Y. Sun, C. Zhao, J. Niu, J. Ren and X. Qu, Colorimetric band-aids for point-of-care sensing and treating bacterial infection, ACS Cent. Sci., 2020, 6(2), 207–212 CrossRef CAS PubMed.
- A. Saadati, F. Farshchi, M. Hasanzadeh, Y. Liu and F. Seidi, Colorimetric and naked-eye detection of arsenic (iii) using a paper-based microfluidic device decorated with silver nanoparticles, RSC Adv., 2022, 12(34), 21836–21850 Search PubMed.
- S. Dalirirad and A. J. Steckl, Lateral flow assay using aptamer-based sensing for on-site detection of dopamine in urine, Anal. Biochem., 2020, 596, 113637 CrossRef CAS PubMed.
- H. Kudo, K. Maejima, Y. Hiruta and D. Citterio, Microfluidic paper-based analytical devices for colorimetric detection of lactoferrin, SLAS Technol., 2020, 25(1), 47–57 CrossRef CAS PubMed.
- S. Nouanthavong, D. Nacapricha, C. S. Henry and Y. Sameenoi, Pesticide analysis using nanoceria-coated paper-based devices as a detection platform, Analyst, 2016, 141(5), 1837–1846 RSC.
- H. J. Kim, Y. Kim, S. J. Park, C. Kwon and H. Noh, Development of colorimetric paper sensor for pesticide detection using competitive-inhibiting reaction, BioChip J., 2018, 12, 326–331 CrossRef CAS.
- M. E. Badawy and A. F. El-Aswad, Bioactive paper sensor based on the acetylcholinesterase for the rapid detection of organophosphate and carbamate pesticides, Int. J. Anal. Chem., 2014, 18, 2014 Search PubMed.
- T. Thongkam and K. Hemavibool, An environmentally friendly microfluidic paper-based analytical device for simultaneous colorimetric detection of nitrite and nitrate in food products, Microchem. J., 2020, 159, 105412 CrossRef CAS.
- E. Trofimchuk, A. Nilghaz, S. Sun and X. Lu, Determination of norfloxacin residues in foods by exploiting the coffee-ring effect and paper-based microfluidics device coupling with smartphone-based detection, J. Food Sci., 2020, 85(3), 736–743 CrossRef CAS PubMed.
- M. Ponram, U. Balijapalli, B. Sambath, S. K. Iyer, K. Kakaraparthi, G. Thota, V. Bakthavachalam, R. Cingaram, J. Sung-Ho and K. N. Sundaramurthy, Inkjet-printed phosphorescent Iridium (III) complex based paper sensor for highly selective detection of Hg2+, Dyes Pigm., 2019, 163, 176–182 CrossRef CAS.
- M. G. Lee, H. W. Yoo, S. H. Lim and G. R. Yi, Inkjet-printed low-cost colorimetric tickets for TNT detection in contaminated soil, Korean J. Chem. Eng., 2020, 37, 2171–2178 CrossRef CAS.
- K. Shrivas, T. Kant, S. Patel, R. Devi, N. S. Dahariya, S. Pervez, M. K. Deb, M. K. Rai and J. Rai, Inkjet-printed paper-based colorimetric sensor coupled with smartphone for determination of mercury (Hg2+), J. Hazard. Mater., 2021, 414, 125440 CrossRef PubMed.
- W. Y. Lim, C. H. Goh, K. Z. Yap and N. Ramakrishnan, One-step fabrication of paper-based inkjet-printed graphene for breath monitor sensors, Biosensors, 2023, 13(2), 209 CrossRef CAS PubMed.
- K. Abe, K. Suzuki and D. Citterio, Inkjet-printed microfluidic multianalyte chemical sensing paper, Anal. Chem., 2008, 80(18), 6928–6934 CrossRef CAS PubMed.
- K. Abe, K. Kotera, K. Suzuki and D. Citterio, Inkjet-printed paperfluidic immuno-chemical sensing device, Anal. Bioanal. Chem., 2010, 398, 885–893 CrossRef CAS PubMed.
- S. Z. Hossain, R. E. Luckham, A. M. Smith, J. M. Lebert, L. M. Davies, R. H. Pelton, C. D. Filipe and J. D. Brennan, Development of a bioactive paper sensor for detection of neurotoxins using piezoelectric inkjet printing of sol− gel-derived bioinks, Anal. Chem., 2009, 81(13), 5474–5483 CrossRef CAS PubMed.
- J. Wang, D. Bowie, X. Zhang, C. Filipe, R. Pelton and J. D. Brennan, Morphology and entrapped enzyme performance in inkjet-printed sol–gel coatings on paper, Chem. Mater., 2014, 26(5), 1941–1947 CrossRef CAS.
- S. Z. Hossain, R. E. Luckham, M. J. McFadden and J. D. Brennan, Reagentless bidirectional lateral flow bioactive paper sensors for detection of pesticides in beverage and food samples, Anal. Chem., 2009, 81(21), 9055–9064 CrossRef CAS PubMed.
- S. Z. Hossain and J. D. Brennan, β-Galactosidase-based colorimetric paper sensor for determination of heavy metals, Anal. Chem., 2011, 83(22), 8772–8778 CrossRef CAS PubMed.
- Z. Zhang, J. Wang, R. Ng, Y. Li, Z. Wu, V. Leung, S. Imbrogno, R. Pelton, J. D. Brennan and C. D. Filipe, An inkjet-printed bioactive paper sensor that reports ATP through odour generation, Analyst, 2014, 139(19), 4775–4778 RSC.
- K. Maejima, S. Tomikawa, K. Suzuki and D. Citterio, Inkjet printing: an integrated and green chemical approach to microfluidic paper-based analytical devices, RSC Adv., 2013, 3(24), 9258–9263 RSC.
- T. Soga, Y. Jimbo, K. Suzuki and D. Citterio, Inkjet-printed paper-based colorimetric sensor array for the discrimination of volatile primary amines, Anal. Chem., 2013, 85(19), 8973–8978 CrossRef CAS PubMed.
- X. Li, J. Tian, G. Garnier and W. Shen, Fabrication of paper-based microfluidic sensors by printing, Colloids Surf., B, 2010, 76(2), 564–570 CrossRef CAS PubMed.
- J. L. Delaney, C. F. Hogan, J. Tian and W. Shen, Electrogenerated chemiluminescence detection in paper-based microfluidic sensors, Anal. Chem., 2011, 83(4), 1300–1306 CrossRef CAS PubMed.
- B. M. Jayawardane, S. Wei, I. D. McKelvie and S. D. Kolev, Microfluidic paper-based analytical device for the determination of nitrite and nitrate, Anal. Chem., 2014, 86(15), 7274–7279 CrossRef CAS PubMed.
- B. M. Jayawardane, I. D. McKelvie and S. D. Kolev, A paper-based device for measurement of reactive phosphate in water, Talanta, 2012, 100, 454–460 CrossRef CAS PubMed.
- H. Kwon, F. Samain and E. T. Kool, Fluorescent DNAs printed on paper: Sensing food spoilage and ripening in the vapor phase, Chem. Sci., 2012, 3(8), 2542–2549 RSC.
- R. S. Alkasir, M. Ornatska and S. Andreescu, Colorimetric paper bioassay for the detection of phenolic compounds, Anal. Chem., 2012, 84(22), 9729–9737 CrossRef CAS PubMed.
- A. Apilux, Y. Ukita, M. Chikae, O. Chailapakul and Y. Takamura, Development of automated paper-based devices for sequential multistep sandwich enzyme-linked immunosorbent assays using inkjet printing, Lab Chip, 2013, 13(1), 126–135 RSC.
- B. Yoon, I. S. Park, H. Shin, H. J. Park, C. W. Lee and J. M. Kim, A litmus-type colorimetric and fluorometric volatile organic compound sensor based on inkjet-printed polydiacetylenes on paper substrates, Macromol. Rapid Commun., 2013, 34(9), 731–735 CrossRef CAS PubMed.
- T. Teerinen, T. Lappalainen and T. Erho, A paper-based lateral flow assay for morphine, Anal. Bioanal. Chem., 2014, 406, 5955–5965 CrossRef CAS PubMed.
- A. Swerin and I. Mira, Ink-jettable paper-based sensor for charged macromolecules and surfactants, Sens. Actuators, B, 2014, 195, 389–395 CrossRef CAS.
- Y. Zhang, F. Lyu, J. Ge and Z. Liu, Ink-jet printing an optimal multi-enzyme system, Chem. Commun., 2014, 50(85), 12919–12922 RSC.
- B. Creran, X. Li, B. Duncan, C. S. Kim, D. F. Moyano and V. M. Rotello, Detection of bacteria using inkjet-printed enzymatic test strips, ACS Appl. Mater. Interfaces, 2014, 6(22), 19525–19530 CrossRef CAS PubMed.
- I. Barbulovic-Nad, M. Lucente, Y. Sun, M. Zhang, A. R. Wheeler and M. Bussmann, Bio-microarray fabrication techniques—a review, Crit. Rev. Biotechnol., 2006, 26(4), 237–259 CrossRef CAS PubMed.
- P. Lv, L. Song, Y. Li, H. Pang and W. Liu, Hybrid ternary rice paper/polypyrrole ink/pen ink nanocomposites as components of flexible supercapacitors, Int. J. Hydrogen Energy, 2021, 46(24), 13219–13229 CrossRef CAS.
- W. Shen, Y. Filonenko, Y. B. Truong, I. H. Parker, N. Brack and P. Pigram,
et al., Contact angle measurement and surface energetics of sized and unsized paper, Colloids Surf., A, 2000, 173(1–3), 117–126 CrossRef CAS.
- M. Rahbar, P. N. Nesterenko, B. Paull and M. Macka, High-throughput deposition of chemical reagents via pen-plotting technique for microfluidic paper-based analytical devices, Anal. Chim. Acta, 2019, 1047, 115–123 CrossRef CAS PubMed.
- K. Maejima, S. Tomikawa, K. Suzuki and D. Citterio, Inkjet printing: an integrated and green chemical approach to microfluidic paper-based analytical devices, RSC Adv., 2013, 3(24), 9258–9263 RSC.
- K. Yamada, S. Takaki, N. Komuro, K. Suzuki and D. Citterio, An antibody-free microfluidic paper-based analytical device for the determination of tear fluid lactoferrin by fluorescence sensitization of Tb3+, Analyst, 2014, 139(7), 1637–1643 RSC.
- A. Prasad, T. Tran and M. R. Gartia, Multiplexed paper microfluidics for titration and detection of ingredients in beverages, Sensors, 2019, 19(6), 1286 CrossRef CAS PubMed.
- E. Carrilho, A. W. Martinez and G. M. Whitesides, Understanding wax printing: a simple micropatterning process for paper-based microfluidics, Anal. Chem., 2009, 81(16), 7091–7095 CrossRef CAS PubMed.
- M. Gutiérrez-Capitán, A. Baldi and C. Fernández-Sánchez, Electrochemical paper-based biosensor devices for rapid detection of biomarkers, Sensors, 2020, 20(4), 967 CrossRef PubMed.
- Y. Lu, W. Shi, L. Jiang, J. Qin and B. Lin, Rapid prototyping of paper-based microfluidics with wax for low-cost, portable bioassay, Electrophoresis, 2009, 30(9), 1497–1500 CrossRef CAS PubMed.
- E. Carrilho, A. W. Martinez and G. M. Whitesides, Understanding wax printing: a simple micropatterning process for paper-based microfluidics, Anal. Chem., 2009, 81(16), 7091–7095 CrossRef CAS PubMed.
- C. Renault, M. J. Anderson and R. M. Crooks, Electrochemistry in hollow-channel paper analytical devices, J. Am. Chem. Soc., 2014, 136(12), 4616–4623 CrossRef CAS PubMed.
- R. Derda, S. K. Tang, A. Laromaine, B. Mosadegh, E. Hong, M. Mwangi, A. Mammoto, D. E. Ingber and G. M. Whitesides, Multizone paper platform for 3D cell cultures, PLoS One, 2011, 6(5), e18940 CrossRef CAS PubMed.
- J. E. Schonhorn, S. C. Fernandes, A. Rajaratnam, R. N. Deraney, J. P. Rolland and C. R. Mace, A device architecture for three-dimensional, patterned paper immunoassays, Lab Chip, 2014, 14(24), 4653–4658 RSC.
- F. F. Tao, X. Xiao, K. F. Lei and I. C. Lee, Based cell culture microfluidic system, BioChip J., 2015, 9, 97–104 CrossRef CAS.
- C. Renault, J. Koehne, A. J. Ricco and R. M. Crooks, Three-dimensional wax patterning of paper fluidic devices, Langmuir, 2014, 30(23), 7030–7036 CrossRef CAS PubMed.
- K. L. Peters, I. Corbin, L. M. Kaufman, K. Zreibe, L. Blanes and B. R. McCord, Simultaneous colorimetric detection of improvised explosive compounds using microfluidic paper-based analytical devices (μPADs), Anal. Methods, 2015, 7(1), 63–70 RSC.
- N. R. Pollock, J. P. Rolland, S. Kumar, P. D. Beattie, S. Jain, F. Noubary, V. L. Wong, R. A. Pohlmann, U. S. Ryan and G. M. Whitesides, A paper-based multiplexed transaminase test for low-cost, point-of-care liver function testing, Sci. Transl. Med., 2012, 4, 152ra129 Search PubMed.
- J. Noiphung, K. Talalak, I. Hongwarittorrn, N. Pupinyo, P. Thirabowonkitphithan and W. Laiwattanapaisal, A novel paper-based assay for the simultaneous determination of Rh typing and forward and reverse ABO blood groups, Biosens. Bioelectron., 2015, 67, 485–489 CrossRef CAS PubMed.
- K. F. Lei, C. H. Huang and N. M. Tsang, Impedimetric quantification of cells encapsulated in hydrogel cultured in a paper-based microchamber, Talanta, 2016, 147, 628–633 CrossRef CAS PubMed.
- K. F. Lei, C. H. Huang, R. L. Kuo, C. K. Chang, K. F. Chen, K. C. Tsao and N. M. Tsang, based enzyme-free immunoassay for rapid detection and subtyping of influenza A H1N1 and H3N2 viruses, Anal. Chim. Acta, 2015, 883, 37–44 CrossRef CAS PubMed.
- M. Funes-Huacca, A. Wu, E. Szepesvari, P. Rajendran, N. Kwan-Wong, A. Razgulin, Y. Shen, J. Kagira, R. Campbell and R. Derda, Portable self-contained cultures for phage and bacteria made of paper and tape, Lab Chip, 2012, 12(21), 4269–4278 RSC.
- P. Teengam, W. Siangproh, A. Tuantranont, C. S. Henry, T. Vilaivan and O. Chailapakul, Electrochemical paper-based peptide nucleic acid biosensor for detecting human papillomavirus, Anal. Chim. Acta, 2017, 952, 32–40 CrossRef CAS PubMed.
- P. Teengam, W. Siangproh, A. Tuantranont, T. Vilaivan, O. Chailapakul and C. S. Henry, Multiplex paper-based colorimetric DNA sensor using pyrrolidinyl peptide nucleic acid-induced AgNPs aggregation for detecting MERS-CoV, MTB, and HPV oligonucleotides, Anal. Chem., 2017, 89(10), 5428–5435 CrossRef CAS PubMed.
- C. K. Chiang, A. Kurniawan, C. Y. Kao and M. J. Wang, Single step and mask-free 3D wax printing of microfluidic paper-based analytical devices for glucose and nitrite assays, Talanta, 2019, 194, 837–845 CrossRef CAS PubMed.
- Y. F. Wisang, H. Sulistyarti, U. Andayani and A. Sabarudin, Microfluidic Paper-based Analytical Devices (μPADs) For Analysis Lead Using Naked Eye and Colorimetric Detections, IOP Conf. Ser. Mater. Sci. Eng., 2019, 546(3), 032033 CrossRef CAS.
- J. S. Ng and M. Hashimoto, Fabrication of paper microfluidic devices using a toner laser printer, RSC Adv., 2020, 10(50), 29797–29807 RSC.
- S. Altundemir, A. K. Uguz and K. Ulgen, A review on wax printed microfluidic paper-based devices for international health, Biomicrofluidics, 2017, 11(4), 041501 CrossRef CAS PubMed.
- W. Dungchai, O. Chailapakul and C. S. Henry, A low-cost, simple, and rapid fabrication method for paper-based microfluidics using wax screen-printing, Analyst, 2011, 136(1), 77–82 RSC.
- S. Teepoo, S. Arsawiset and P. Chanayota, One-step polylactic acid screen-printing microfluidic paper-based analytical device: application for simultaneous detection of nitrite and nitrate in food samples, Chemosensors, 2019, 7(3), 44 CrossRef CAS.
- S. Cinti, D. Talarico, G. Palleschi, D. Moscone and F. Arduini, Novel reagentless paper-based screen-printed electrochemical sensor to detect phosphate, Anal. Chim. Acta, 2016, 919, 78–84 CrossRef CAS PubMed.
- P. Jarujamrus, R. Meelapsom, P. Naksen, N. Ditcharoen, W. Anutrasakda, A. Siripinyanond, M. Amatatongchai and S. Supasorn, Screen-printed microfluidic paper-based analytical device (μPAD) as a barcode sensor for magnesium detection using rubber latex waste as a novel hydrophobic reagent, Anal. Chim. Acta, 2019, 1082, 66–77 CrossRef CAS PubMed.
- V. Caratelli, A. Ciampaglia, J. Guiducci, G. Sancesario, D. Moscone and F. Arduini, Precision medicine in Alzheimer's disease: An origami paper-based electrochemical device for cholinesterase inhibitors, Biosens. Bioelectron., 2020, 165, 112411 CrossRef CAS PubMed.
- J. Sitanurak, N. Fukana, T. Wongpakdee, Y. Thepchuay, N. Ratanawimarnwong, T. Amornsakchai and D. Nacapricha, T-shirt ink for one-step screen-printing of hydrophobic barriers for 2D-and 3D-microfluidic paper-based analytical devices, Talanta, 2019, 205, 120113 CrossRef CAS PubMed.
- W. Dungchai, O. Chailapakul and C. S. Henry, A low-cost, simple, and rapid fabrication method for paper-based microfluidics using wax screen-printing, Analyst, 2011, 136(1), 77–82 RSC.
- Y. Xu, M. Liu, N. Kong and J. Liu, Lab-on-paper micro-and nano-analytical devices: Fabrication, modification, detection and emerging applications, Microchim. Acta, 2016, 183, 1521–1542 CrossRef CAS.
- Y. Sameenoi, P. N. Nongkai, S. Nouanthavong, C. S. Henry and D. Nacapricha, One-step polymer screen-printing for microfluidic paper-based analytical device (μPAD) fabrication, Analyst, 2014, 139(24), 6580–6588 RSC.
- F. Sun, K. Wu, H. C. Hung, P. Zhang, X. Che, J. Smith, X. Lin, B. Li, P. Jain, Q. Yu and S. Jiang, Paper sensor coated with a poly (carboxybetaine)-multiple DOPA conjugate via dip-coating for biosensing in complex media, Anal. Chem., 2017, 89(20), 10999–11004 CrossRef CAS PubMed.
- S. Kumar, C. M. Pandey, A. Hatamie, A. Simchi, M. Willander and B. D. Malhotra, Nanomaterial-modified conducting paper: fabrication, properties, and emerging biomedical applications, Global Challenges, 2019, 3(12), 1900041 CrossRef PubMed.
- V. Kedambaimoole, K. Harsh, K. Rajanna, P. Sen, M. M. Nayak and S. Kumar, MXene wearables: properties, fabrication strategies, sensing mechanism and applications, Mater. Adv., 2022, 3(9), 3784–3808 RSC.
- T. Songjaroen, W. Dungchai, O. Chailapakul and W. Laiwattanapaisal, Novel, simple and low-cost alternative method for fabrication of paper-based microfluidics by wax dipping, Talanta, 2011, 85(5), 2587–2593 CrossRef CAS PubMed.
- X. Tang and X. Yan, Dip-coating for fibrous materials: mechanism, methods and applications, J. Sol-Gel Sci. Technol., 2017, 81, 378–404 Search PubMed.
- E. W. Nery and L. T. Kubota, Sensing approaches on paper-based devices: a review, Anal. Bioanal. Chem., 2013, 405, 7573–7595 CrossRef CAS PubMed.
- H. Liu, H. Jiang, F. Du, D. Zhang, Z. Li and H. Zhou, Flexible and degradable paper-based strain sensor with low cost, ACS Sustain. Chem. Eng., 2017, 5(11), 10538–10543 CrossRef CAS.
- A. W. Martinez, S. T. Phillips, G. M. Whitesides and E. Carrilho, Diagnostics for the Developing World: Microfluidic Paper-Based Analytical Devices, Anal. Chem., 2010, 82(1), 3–10 CrossRef CAS PubMed.
- A. W. Martinez, S. T. Phillips, M. J. Butte and G. M. Whitesides, Patterned paper as a platform for inexpensive, low-volume, portable bioassays, Angew. Chem., 2007, 119(8), 1340–1342 CrossRef.
- K. V. Nemani, K. L. Moodie, J. B. Brennick, A. Su and B. Gimi, In vitro and in vivo evaluation of SU-8 biocompatibility, Mater. Sci. Eng. C, 2013, 33(7), 4453–4459 CrossRef CAS PubMed.
- L. S. Busa, M. Maeki, A. Ishida, H. Tani and M. Tokeshi, Simple and sensitive colorimetric assay system for horseradish peroxidase using microfluidic paper-based devices, Sens. Actuators, B, 2016, 236, 433–441 CrossRef CAS.
- A. W. Martinez, S. T. Phillips, M. J. Butte and G. M. Whitesides, Patterned paper as a platform for inexpensive, low-volume, portable bioassays, Angew. Chem., 2007, 119(8), 1318–1320 CrossRef PubMed.
- A. T. Singh, D. Lantigua, A. Meka, S. Taing, M. Pandher and G. Camci-Unal, based sensors: Emerging themes and applications, Sensors, 2018, 18(9), 2838 CrossRef PubMed.
- Y. Xia, J. Si and Z. Li, Fabrication techniques for microfluidic paper-based analytical devices and their applications for biological testing: A review, Biosens. Bioelectron., 2016, 77, 774–789 CrossRef CAS PubMed.
- M. A. Mahmud, E. J. Blondeel, M. Kaddoura and B. D. MacDonald, Features in microfluidic paper-based devices made by laser cutting: How small can they be?, Micromachines, 2018, 9(5), 220 CrossRef PubMed.
- Y. Zhang, J. Liu, H. Wang and Y. Fan, Laser-induced selective wax reflow for paper-based microfluidics, RSC Adv., 2019, 9(20), 11460–11464 RSC.
- D. H. Hiep, Y. Tanaka, H. Matsubara and S. Ishizaka, Fabrication of paper-based microfluidic devices using a laser beam scanning technique, Anal. Sci., 2020, 36(10), 1275–1278 CrossRef CAS PubMed.
- E. Fu, P. Kauffman, B. Lutz and P. Yager, Chemical signal amplification in two-dimensional paper networks, Sens. Actuators, B, 2010, 149(1), 325–328 CrossRef CAS PubMed.
- M. A. Mahmud, E. J. Blondeel, M. Kaddoura and B. D. MacDonald, Creating compact and microscale features in paper-based devices by laser cutting, Analyst, 2016, 141(23), 6449–6454 RSC.
-
T. Muangpool and S. Pullteap, Reviews on laser cutting technology for industrial applications, InThird International Conference on Photonics Solutions (ICPS2017), SPIE, 2018, vol. 10714, pp. 184–191 Search PubMed.
- R. Ghosh, S. Gopalakrishnan, R. Savitha, T. Renganathan and S. Pushpavanam, Fabrication of laser printed microfluidic paper-based analytical devices (LP-μPADs) for point-of-care applications, Sci. Rep., 2019, 9(1), 7896 CrossRef PubMed.
- P. J. He, I. N. Katis, R. W. Eason and C. L. Sones, Laser direct-write for fabrication of three-dimensional paper-based devices, Lab Chip, 2016, 16(17), 3296–3303 RSC.
- A. W. Martinez, S. T. Phillips and G. M. Whitesides, Three-dimensional microfluidic devices fabricated in layered paper and tape, Proc. Natl. Acad. Sci. U. S. A., 2008, 105(50), 19606–19611 CrossRef CAS PubMed.
- H. Wang, Y. J. Li, J. F. Wei, J. R. Xu, Y. H. Wang and G. X. Zheng, Based three-dimensional microfluidic device for monitoring of heavy metals with a camera cell phone, Anal. Bioanal. Chem., 2014, 406, 2799–2807 CrossRef CAS PubMed.
- Y. Guan, K. Zhang, F. Xu, R. Guo, A. Fang, B. Sun, X. Meng, Y. Liu and M. Bia, An integrated platform for fibrinogen quantification on a microfluidic paper-based analytical device, Lab Chip, 2020, 20(15), 2724–2734 RSC.
- Q. H. Nguyen and M. I. Kim, Nanomaterial-mediated paper-based biosensors for colorimetric pathogen detection, TrAC, Trends Anal. Chem., 2020, 132, 116038 CrossRef CAS PubMed.
- J. Ding, B. Li, L. Chen and W. Qin, A three-dimensional origami paper-based device for potentiometric biosensing, Angew. Chem., Int. Ed., 2016, 55(42), 13033–13037 CrossRef CAS PubMed.
- E. Lazzarini, A. Pace, I. Trozzi, M. Zangheri, M. Guardigli, D. Calabria and M. Mirasoli, An origami paper-based biosensor for allergen detection by chemiluminescence immunoassay on magnetic microbeads, Biosensors, 2022, 12(10), 825 CrossRef CAS PubMed.
- H. Lim, A. T. Jafry and J. Lee, Fabrication, flow control, and applications of microfluidic paper-based analytical devices, Molecules, 2019, 24(16), 2869 CrossRef CAS PubMed.
- A. K. Yetisen, M. S. Akram and C. R. Lowe, Based microfluidic point-of-care diagnostic devices, Lab Chip, 2013, 13(12), 2210–2251 RSC.
- S. G. Jeong, D. H. Kim, J. Kim, J. H. Kim, S. Song and C. S. Lee, Programmable microfluidic flow for automatic multistep digital assay in a single-sheet 3-dimensional paper-based microfluidic device, Chem. Eng. J., 2021, 411, 128429 CrossRef CAS.
- X. Li and X. Liu, Fabrication of three-dimensional microfluidic channels in a single layer of cellulose paper, Microfluid. Nanofluid., 2014, 16, 819–827 CrossRef CAS.
- K. Punpattanakul, S. Kraduangdej, N. Jiranusornkul, M. Chiaranairungroj, A. Pimpin, T. Palaga and W. Srituravanich, A novel patterning method for three-dimensional paper-based devices by using inkjet-printed water mask, Cellulose, 2018, 25, 2659–2665 CrossRef.
- G. G. Morbioli, T. Mazzu-Nascimento, A. M. Stockton and E. Carrilho, Technical aspects and challenges of colorimetric detection with microfluidic paper-based analytical devices (μPADs)-A review, Anal. Chim. Acta, 2017, 970, 1–22 CrossRef CAS PubMed.
- N. S. Gunda, S. Dasgupta and S. K. Mitra, DipTest: A litmus test for E. coli detection in water, PLoS One, 2017, 12(9), e0183234 CrossRef PubMed.
- N. Idros and D. Chu, Triple-indicator-based multidimensional colorimetric sensing platform for heavy metal ion detections, ACS Sens., 2018, 3(9), 1756–1764 CrossRef CAS PubMed.
- P. C. Chen, Y. C. Li, J. Y. Ma, J. Y. Huang, C. F. Chen and H. T. Chang, Size-tunable copper nanocluster aggregates and their application in hydrogen sulfide sensing on paper-based devices, Sci. Rep., 2016, 6(1), 24882 CrossRef CAS PubMed.
- P. Borthakur, P. K. Boruah and M. R. Das, CuS and NiS nanoparticle-decorated porous-reduced graphene oxide sheets as efficient peroxidase nanozymes for easy colorimetric detection of Hg (II) ions in a water medium and using a paper strip, ACS Sustain. Chem. Eng., 2021, 9(39), 13245–13255 CrossRef CAS.
- X. Kou, L. Tong, Y. Shen, W. Zhu, L. Yin, S. Huang, F. Zhu, G. Chen and G. Ouyang, Smartphone-assisted robust enzymes@ MOFs-based paper biosensor for point-of-care detection, Biosens. Bioelectron., 2020, 156, 112095 CrossRef CAS PubMed.
- H. Guan, S. Du, B. Han, Q. Zhang and D. Wang, A rapid and sensitive smartphone colorimetric sensor for detection of ascorbic acid in food using the nanozyme paper-based microfluidic chip, Lebensm. Wiss. Technol., 2023, 184, 115043 CrossRef CAS.
- H. Gao, Y. Li, Y. Li, K. Qu, K. Zhang and J. Li, Detection of antibiotic-resistance genes in bacterial pathogens using a Cas12a/3D DNAzyme colorimetric paper sensor, Fundam. Res., 2023 DOI:10.1016/j.fmre.2023.04.011.
- H. Kim, D. R. Chung and M. Kang, A new point-of-care test for the diagnosis of infectious diseases based on multiplex lateral flow immunoassays, Analyst, 2019, 144(8), 2460–2466 RSC.
- J. Roh, S. Y. Lee, S. Park and D. J. Ahn, Polydiacetylene/anti-HBs complexes for visible and fluorescent detection of hepatitis B surface antigen on a nitrocellulose membrane, Chem.–Asian J., 2017, 12(16), 2033–2037 CrossRef CAS PubMed.
- C. N. Loynachan, M. R. Thomas, E. R. Gray, D. A. Richards, J. Kim, B. S. Miller, J. C. Brookes, S. Agarwal, V. Chudasama, R. A. McKendry and M. M. Stevens, Platinum nanocatalyst amplification: redefining the gold standard for lateral flow immunoassays with ultrabroad dynamic range, ACS Nano, 2018, 12(1), 279–288 CrossRef CAS PubMed.
- Y. T. Chen and J. T. Yang, Detection of an amphiphilic biosample in a paper microchannel based on length, Biomed. Microdevices, 2015, 17, 1–8 CrossRef PubMed.
- G. G. Lewis, M. J. DiTucci and S. T. Phillips, Quantifying analytes in paper-based microfluidic devices without using external electronic readers, Angew. Chem., Int. Ed., 2012, 51(51), 12707–12710 CrossRef CAS PubMed.
- M. A. Mahmud, E. J. Blondeel and B. D. MacDonald, Counting-based microfluidic paper-based devices capable of analyzing submicroliter sample volumes, Biomicrofluidics, 2020, 14(1), 014107 CrossRef CAS PubMed.
- J. C. Hofstetter, J. B. Wydallis, G. Neymark, T. H. Reilly III, J. Harrington and C. S. Henry, Quantitative colorimetric paper analytical devices based on radial distance measurements for aqueous metal determination, Analyst, 2018, 143, 3085–3090 RSC.
- D. M. Cate, S. D. Noblitt, J. Volckens and C. S. Henry, Multiplexed paper analytical device for quantification of metals using distance-based detection, Lab Chip, 2015, 15(13), 2808–2818 RSC.
- Y. Shimada and T. Kaneta, Highly sensitive paper-based analytical devices with the introduction of a large-volume sample via continuous flow, Anal. Sci., 2018, 34(1), 65–70 CrossRef CAS PubMed.
- X. Wei, T. Tian, S. Jia, Z. Zhu, Y. Ma, J. Sun, Z. Lin and C. J. Yang, Microfluidic distance readout sweet hydrogel integrated paper-based analytical device (μDiSH-PAD) for visual quantitative point-of-care testing, Anal. Chem., 2016, 88(4), 2345–2352 CrossRef CAS PubMed.
- A. G. Wang, T. Dong, H. Mansour, G. Matamoros, A. L. Sanchez and F. Li, based DNA reader for visualized quantification of soil-transmitted helminth infections, ACS Sens., 2018, 3(1), 205–210 CrossRef CAS PubMed.
- C. W. Quinn, D. M. Cate, D. D. Miller-Lionberg, I. I. I. T. Reilly, J. Volckens and C. S. Henry, Solid-phase extraction coupled to a paper-based technique for trace copper detection in drinking water, Environ. Sci. Technol., 2018, 52(6), 3567–3573 CrossRef CAS PubMed.
- T. Tian, Y. An, Y. Wu, Y. Song, Z. Zhu and C. Yang, Integrated distance-based origami paper analytical device for one-step visualized analysis, ACS Appl. Mater. Interfaces, 2017, 9(36), 30480–30487 CrossRef CAS PubMed.
- L. Cai, Y. Fang, Y. Mo, Y. Huang, C. Xu, Z. Zhang and M. Wang, Visual quantification of Hg on a microfluidic paper-based
analytical device using distance-based detection technique, AIP Adv., 2017, 7(8), 085214 CrossRef.
- S. B. Berry, S. C. Fernandes, A. Rajaratnam, N. S. DeChiara and C. R. Mace, Measurement of the hematocrit using paper-based microfluidic devices, Lab Chip, 2016, 16(19), 3689–3694 RSC.
- K. Katelakha, V. Nopponpunth, W. Boonlue and W. Laiwattanapaisal, A simple distance paper-based analytical device for the screening of lead in food matrices, Biosensors, 2021, 11(3), 90 CrossRef CAS PubMed.
- P. Giménez-Gómez, I. Hättestrand, S. Sjöberg, C. Dupraz, S. Richardson and N. Pamme, Distance-based paper analytical device for the determination of dissolved inorganic carbon concentration in freshwater, Sens. Actuators, B, 2023, 385, 133694 CrossRef.
- T. Tian, Y. An, Y. Wu, Y. Song, Z. Zhu and C. Yang, Integrated distance-based origami paper analytical device for one-step visualized analysis, ACS Appl. Mater. Interfaces, 2017, 9(36), 30480–30487 CrossRef CAS PubMed.
- L. Cai, Z. Ouyang, J. Song and L. Yang, Indicator-free argentometric titration for distance-based detection of chloride using microfluidic paper-based analytical devices, ACS Omega, 2020, 5(30), 18935–18940 CrossRef CAS PubMed.
- A. G. Wang, T. Dong, H. Mansour, G. Matamoros, A. L. Sanchez and F. Li, Based DNA reader for visualized quantification of soil-transmitted helminth infections, ACS Sens., 2018, 3(1), 205–210 CrossRef CAS PubMed.
- Y. Soda, D. Citterio and E. Bakker, Equipment-free detection of K+ on microfluidic paper-based analytical devices based on exhaustive replacement with ionic dye in ion-selective capillary sensors, ACS Sens., 2019, 4(3), 670–677 CrossRef CAS PubMed.
- S. Allameh and M. Rabbani, A distance-based microfluidic paper-based biosensor for glucose measurements in tear range, Appl. Biochem. Biotechnol., 2022, 194(5), 2077–2092 CrossRef CAS PubMed.
- N. S. Moreira, C. L. Chagas, K. A. Oliveira, G. F. Duarte-Junior, F. R. de Souza, M. Santhiago, C. D. Garcia, L. T. Kubota and W. K. Coltro, Fabrication of microwell plates and microfluidic devices in polyester films using a cutting printer, Anal. Chim. Acta, 2020, 1119, 1 CrossRef CAS PubMed.
- M. P. Nguyen, S. P. Kelly, J. B. Wydallis and C. S. Henry, Read-by-eye quantification of aluminum (III) in distance-based microfluidic paper-based analytical devices, Anal. Chim. Acta, 2020, 1100, 156–162 CrossRef CAS PubMed.
- K. Phoonsawat and W. Dungchai, Highly sensitive, selective and naked-eye detection of bromide and bromate using distance–based paper analytical device, Talanta, 2021, 221, 121590 CrossRef CAS PubMed.
- C. Chen, L. Zhao, H. Zhang, X. Shen, Y. Zhu and H. Chen, Novel wax valves to improve distance-based analyte detection in paper microfluidics, Anal. Chem., 2019, 91(8), 5169–5175 CrossRef CAS PubMed.
- M. Rahbar, A. R. Wheeler, B. Paull and M. Macka, Ion-exchange based immobilization of chromogenic reagents on microfluidic paper analytical devices, Anal. Chem., 2019, 91(14), 8756–8761 CrossRef CAS PubMed.
- S. Buking, P. Saetear, W. Tiyapongpattana, K. Uraisin, P. Wilairat, D. Nacapricha and N. Ratanawimarnwong, Microfluidic paper-based analytical device for quantification of lead using reaction band-length for identification of bullet hole and its potential for estimating firing distance, Anal. Sci., 2018, 34(1), 83–89 CrossRef CAS PubMed.
- S. B. Berry, S. C. Fernandes, A. Rajaratnam, N. S. DeChiara and C. R. Mace, Measurement of the hematocrit using paper-based microfluidic devices, Lab Chip, 2016, 16(19), 3689–3694 RSC.
- K. Yamada, T. G. Henares, K. Suzuki and D. Citterio, Distance-based tear lactoferrin assay on microfluidic paper device using interfacial interactions on surface-modified cellulose, ACS Appl.
Mater. Interfaces, 2015, 7(44), 24864–24875 CrossRef CAS PubMed.
- D. M. Cate, W. Dungchai, J. C. Cunningham, J. Volckens and C. S. Henry, Simple, distance-based measurement for paper analytical devices, Lab Chip, 2013, 13(12), 2397–2404 RSC.
- S. S. Nadar, P. D. Patil, M. S. Tiwari and D. J. Ahirrao, Enzyme embedded microfluidic paper-based analytic device (μPAD): a comprehensive review, Crit. Rev. Biotechnol., 2021, 41(7), 1046–1080 CrossRef CAS PubMed.
- E. F. Gabriel, P. T. Garcia, F. M. Lopes and W. K. Coltro, Based colorimetric biosensor for tear glucose measurements, Micromachines, 2017, 8(4), 104 CrossRef.
- C. Park, H. R. Kim, S. K. Kim, I. K. Jeong, J. C. Pyun and S. Park, Three-dimensional paper-based microfluidic analytical devices integrated with a plasma separation membrane for the detection of biomarkers in whole blood, ACS Appl. Mater. Interfaces, 2019, 11(40), 36428–36434 CrossRef CAS PubMed.
- M. M. Erenas, B. Carrillo-Aguilera, K. Cantrell, S. Gonzalez-Chocano, I. M. de Vargas-Sansalvador, I. de Orbe-Payá and L. F. Capitan-Vallvey, Real time monitoring of glucose in whole blood by smartphone, Biosens. Bioelectron., 2019, 136, 47–52 CrossRef CAS PubMed.
- K. Khachornsakkul, F. J. Rybicki and S. Sonkusale, Nanomaterials integrated with microfluidic paper-based analytical devices for enzyme-free glucose quantification, Talanta, 2023, 260, 124538 CrossRef CAS PubMed.
- S. Abarghoei, N. Fakhri, Y. S. Borghei, M. Hosseini and M. R. Ganjali, A colorimetric paper sensor for citrate as biomarker for early stage detection of prostate cancer based on peroxidase-like activity of cysteine-capped gold nanoclusters, Spectrochim. Acta, Part A, 2019, 210, 251–259 CrossRef CAS PubMed.
- I. Lewińska, M. Speichert, M. Granica and Ł. Tymecki, Colorimetric point-of-care paper-based sensors for urinary creatinine with smartphone readout, Sens. Actuators, B, 2021, 340, 129915 CrossRef.
-
M. Mukhopadhyay, S. G. Subramanian, K. V. Durga, D. Sarkar and S. DasGupta, Design, Fabrication, and Theoretical Investigation of a Cost-Effective Laser Printing Based Colorimetric Paper Sensor for Non-Invasive Glucose and Ketone Detection, bioRxiv, 2021, preprint, vol. 24, DOI:10.1101/2021.09.23.461386.
- M. Rahbar, S. Zou, M. Baharfar and G. Liu, A customized microfluidic paper-based platform for colorimetric immunosensing: demonstrated via hCG assay for pregnancy test, Biosensors, 2021, 11(12), 474 CrossRef CAS PubMed.
- T. Pomili, P. Donati and P. P. Pompa, Based multiplexed colorimetric device for the simultaneous detection of salivary biomarkers, Biosensors, 2021, 11(11), 443 CrossRef CAS PubMed.
- J. L. Davidson, J. Wang, M. K. Maruthamuthu, A. Dextre, A. Pascual-Garrigos, S. Mohan, S. V. Putikam, F. O. Osman, D. McChesney, J. Seville and M. S. Verma, A paper-based colorimetric molecular test for SARS-CoV-2 in saliva, Biosens. Bioelectron.: X, 2021, 9, 100076 CAS.
- K. Pungjunun, A. Yakoh, S. Chaiyo, N. Praphairaksit, W. Siangproh, K. Kalcher and O. Chailapakul, Laser engraved microapillary pump paper-based microfluidic device for colorimetric and electrochemical detection of salivary thiocyanate, Microchim. Acta, 2021, 188, 1 CrossRef PubMed.
- L. A. Santana-Jiménez, A. Márquez-Lucero, V. Osuna, I. Estrada-Moreno and R. B. Dominguez, Naked-eye detection of glucose in saliva with bienzymatic paper-based sensor, Sensors, 2018, 18(4), 1071 CrossRef PubMed.
- Y. Jia, H. Sun, H. Dong, C. Wang, X. Lin and D. Dong, Scalable and parallelized biochemical assays in paper devices integrated with a programmable binary valve matrix, Sens. Actuators, B, 2020, 321, 128466 CrossRef CAS.
- C. K. Chiang, A. Kurniawan, C. Y. Kao and M. J. Wang, Single step and mask-free 3D wax printing of microfluidic paper-based analytical devices for glucose and nitrite assays, Talanta, 2019, 194, 837–845 CrossRef CAS PubMed.
- Y. Jiang, Z. Hao, Q. He and H. Chen, A simple method for fabrication of microfluidic paper-based analytical devices and on-device fluid control with a portable corona generator, RSC Adv., 2016, 6(4), 2888–2894 RSC.
- J. Sitanurak, N. Fukana, T. Wongpakdee, Y. Thepchuay, N. Ratanawimarnwong, T. Amornsakchai and D. Nacapricha, T-shirt ink for one-step screen-printing of hydrophobic barriers for 2D-and 3D-microfluidic paper-based analytical devices, Talanta, 2019, 205, 120113 CrossRef CAS PubMed.
- F. T. Ferreira, R. B. Mesquita and A. O. Rangel, Novel microfluidic paper-based analytical devices (μPADs) for the determination of nitrate and nitrite in human saliva, Talanta, 2020, 219, 121183 CrossRef CAS PubMed.
- J. Bhardwaj, A. Sharma and J. Jang, Vertical flow-based paper immunosensor for rapid electrochemical and colorimetric detection of influenza virus using a different pore size sample pad, Biosens. Bioelectron., 2019, 126, 36–43 CrossRef CAS PubMed.
- R. Tang, H. Yang, J. R. Choi, Y. Gong, J. Hu, T. Wen, X. Li, B. Xu, Q. Mei and F. Xu, based device with on-chip reagent storage for rapid extraction of DNA from biological samples, Microchim. Acta, 2017, 184, 2141–2150 CrossRef CAS.
- D. Das, A. Dsouza, N. Kaur, S. Soni and B. J. Toley, Paper stacks for uniform rehydration of dried reagents in paper microfluidic devices, Sci. Rep., 2019, 9(1), 15755 CrossRef PubMed.
- L. Lvova, G. Pomarico, F. Mandoj, F. Caroleo, C. Di Natale, K. M. Kadish and S. Nardis, Smartphone coupled with a paper-based optode: Towards a selective cyanide detection, J. Porphyrins Phthalocyanines, 2020, 24(05n07), 964–972 CrossRef CAS.
- G. Vyas, S. Bhatt, M. K. Si, S. Jindani, E. Suresh, B. Ganguly and P. Paul, Colorimetric dual sensor for Cu (II) and tyrosine and its application as paper strips for detection in water and human saliva as real samples, Spectrochim. Acta, Part A, 2020, 230, 118052 CrossRef CAS PubMed.
- A. Sheini, A paper-based device for the colorimetric determination of ammonia and carbon dioxide using thiomalic acid and maltol functionalized silver nanoparticles: application to the enzymatic determination of urea in saliva and blood, Microchim. Acta, 2020, 187, 1 CrossRef PubMed.
- M. Srisa-Art, K. E. Boehle, B. J. Geiss and C. S. Henry, Highly sensitive detection of Salmonella typhimurium using a colorimetric paper-based analytical device coupled with immunomagnetic separation, Anal. Chem., 2018, 90(1), 1035–1043 CrossRef CAS PubMed.
- A. K. Govindarajalu, M. Ponnuchamy, B. Sivasamy, M. V. Prabhu and A. Kapoor, A cellulosic paper-based sensor for detection of starch contamination in milk, Bull. Mater. Sci., 2019, 42, 1–6 CrossRef CAS.
- A. S. Dena, S. A. Khalid, A. F. Ghanem, A. I. Shehata and I. M. El-Sherbiny, User-friendly lab-on-paper optical sensor for the rapid detection of bacterial spoilage in packaged meat products, RSC Adv., 2021, 11(56), 35165–35173 RSC.
- L. Xiao, Z. Zhang, C. Wu, L. Han and H. Zhang, Molecularly imprinted polymer grafted paper-based method for the detection of 17β-estradiol, Food Chem., 2017, 221, 82–86 CrossRef CAS PubMed.
- J. Narang, N. Malhotra, C. Singhal, A. Mathur, D. Chakraborty, A. Anil, A. Ingle and C. S. Pundir, Point of care with micro fluidic paper based device integrated with nano zeolite–graphene oxide nanoflakes for electrochemical sensing of ketamine, Biosens. Bioelectron., 2017, 88, 249–257 CrossRef CAS PubMed.
- L. Ma, A. Nilghaz, J. R. Choi, X. Liu and X. Lu, Rapid detection of clenbuterol in milk using microfluidic paper-based ELISA, Food Chem., 2018, 246, 437–441 CrossRef CAS PubMed.
- N. Gao, P. Huang and F. Wu, Colorimetric detection of melamine in milk based on Triton X-100 modified gold nanoparticles and its paper-based application, Spectrochim. Acta, Part A, 2018, 192, 174–180 CrossRef CAS PubMed.
- M. Salve, A. Wadafale, G. Dindorkar and J. Kalambe, Quantifying colorimetric assays in μPAD for milk adulterants detection using colorimetric android application, Micro Nano Lett., 2018, 13(11), 1520–1524 CrossRef CAS.
- Y. Fan, H. Wang, S. Liu, B. Zhang and Y. Zhang, Milk carton with integrated paper-based microfluidics for milk quality rapid test, J. Food Saf., 2018, 38(6), e12548 CrossRef.
- C. C. Liu, Y. N. Wang, L. M. Fu and K. L. Chen, Microfluidic paper-based chip platform for benzoic acid detection in food, Food Chem., 2018, 249, 162–167 CrossRef CAS PubMed.
- A. W. Waller, M. Toc, D. J. Rigsby, M. Gaytan-Martínez and J. E. Andrade, Development of a paper-based sensor compatible with a mobile phone for the detection of common iron formulas used in fortified foods within resource-limited settings, Nutrients, 2019, 11, 7 CrossRef PubMed.
- L. Xie, X. Zi, H. Zeng, J. Sun, L. Xu and S. Chen, Low-cost fabrication of a paper-based microfluidic using a folded pattern paper, Anal. Chim. Acta, 2019, 1053, 131–138 CrossRef CAS PubMed.
- A. Shahvar, M. Saraji, H. Gordan and D. Shamsaei, Combination of paper-based thin film microextraction with smartphone-based sensing for sulfite assay in food samples, Talanta, 2019, 197, 578–583 CrossRef CAS PubMed.
- S. Shariati and G. Khayatian, Microfluidic paper-based analytical device using gold nanoparticles modified with N, N′-bis (2-hydroxyethyl) dithiooxamide for detection of Hg (ii) in air, fish and water samples, New J. Chem., 2020, 44(43), 18662–18667 RSC.
- T. Thongkam and K. Hemavibool, An environmentally friendly microfluidic paper-based analytical device for simultaneous colorimetric detection of nitrite and nitrate in food products, Microchem. J., 2020, 159, 105412 CrossRef CAS.
- N. Ratnarathorn and W. Dungchai, Based analytical device (PAD) for the determination of borax, salicylic acid, nitrite, and nitrate by colorimetric methods, J. Anal. Chem., 2020, 75, 487–494 CrossRef CAS.
- E. Trofimchuk, Y. Hu, A. Nilghaz, M. Z. Hua, S. Sun and X. Lu, Development of paper-based microfluidic device for the determination of nitrite in meat, Food Chem., 2020, 316, 126396 CrossRef CAS PubMed.
- X. W. Huang, X. B. Zou, J. Y. Shi, Y. Guo, J. W. Zhao, J. Zhang and L. Hao, Determination of pork spoilage by colorimetric gas sensor array based on natural pigments, Food Chem., 2014, 145, 549–554 CrossRef CAS PubMed.
- Y. Chen, G. Fu, Y. Zilberman, W. Ruan, S. K. Ameri, Y. S. Zhang, E. Miller and S. R. Sonkusale, Low cost smart phone diagnostics for food using paper-based colorimetric sensor arrays, Food Control, 2017, 82, 227–232 CrossRef CAS.
- H. Z. Chen, M. Zhang, B. Bhandari and C. H. Yang, Development of a novel colorimetric food package label for monitoring lean pork freshness, Lwt, 2019, 99, 43–49 CrossRef CAS.
- H. Z. Chen, M. Zhang, B. Bhandari and Z. Guo, Applicability of a colorimetric indicator label for monitoring freshness of fresh-cut green bell pepper, Postharvest Biol. Technol., 2018, 140, 85–92 CrossRef CAS.
- H. J. Kim, C. Kwon, B. S. Lee and H. Noh, One-step sensing of foodborne pathogenic bacteria using a 3D paper-based device, Analyst, 2019, 144(7), 2248–2255 RSC.
- M. Asif, F. R. Awan, Q. M. Khan, B. Ngamsom and N. Pamme, based analytical devices for colorimetric detection of S. aureus and E. coli and their antibiotic resistant strains in milk, Analyst, 2020, 145(22), 7320–7329 RSC.
- B. Pang, C. Zhao, L. Li, X. Song, K. Xu, J. Wang, Y. Liu, K. Fu, H. Bao, D. Song and X. Meng, Development of a low-cost paper-based ELISA method for rapid Escherichia coli O157: H7 detection, Anal. Biochem., 2018, 542, 58–62 CrossRef CAS PubMed.
- L. Ma, A. Nilghaz, J. R. Choi, X. Liu and X. Lu, Rapid detection of clenbuterol in milk using microfluidic paper-based ELISA, Food Chem., 2018, 246, 437–441 CrossRef CAS PubMed.
- L. Sun, Y. Jiang, R. Pan, M. Li, R. Wang, S. Chen, S. Fu and C. Man, A novel, simple and low-cost paper-based analytical device for colorimetric detection of Cronobacter spp, Anal. Chim. Acta, 2018, 1036, 80–88 CrossRef CAS PubMed.
- B. Wang, Z. Lin and M. Wang, Fabrication of a paper-based microfluidic device to readily determine nitrite ion concentration by simple colorimetric assay, J. Chem. Educ., 2015, 92(4), 733–736 CrossRef CAS.
- C. C. Liu, Y. N. Wang, L. M. Fu and K. L. Chen, Microfluidic paper-based chip platform for benzoic acid detection in food, Food Chem., 2018, 249, 162–167 CrossRef CAS PubMed.
- S. Chaiyo, W. Siangproh, A. Apilux and O. Chailapakul, Highly selective and sensitive paper-based colorimetric sensor using thiosulfate catalytic etching of silver nanoplates for trace determination of copper ions, Anal. Chim. Acta, 2015, 866, 75–83 CrossRef CAS PubMed.
- W. Wu, S. Zhao, Y. Mao, Z. Fang, X. Lu and L. Zeng, A sensitive lateral flow biosensor for Escherichia coli O157: H7 detection based on aptamer mediated strand displacement amplification, Anal. Chim. Acta, 2015, 861, 62–68 CrossRef CAS PubMed.
- L. Yu, Z. Shi, C. Fang, Y. Zhang, Y. Liu and C. Li, Disposable lateral flow-through strip for smartphone-camera to quantitatively detect alkaline phosphatase activity in milk, Biosens. Bioelectron., 2015, 69, 307–315 CrossRef CAS PubMed.
- X. X. Zhang, Y. Z. Song, F. Fang and Z. Y. Wu, Sensitive paper-based analytical device for fast colorimetric detection of nitrite with smartphone, Anal. Bioanal. Chem., 2018, 410, 2665–2669 CrossRef CAS PubMed.
- Y. Chen, N. Cheng, Y. Xu, K. Huang, Y. Luo and W. Xu, Point-of-care and visual detection of P. aeruginosa and its toxin genes by multiple LAMP and lateral flow nucleic acid biosensor, Biosens. Bioelectron., 2016, 81, 317–323 CrossRef CAS PubMed.
- J. R. Choi, J. Hu, R. Tang, Y. Gong, S. Feng, H. Ren, T. Wen, X. Li, W. A. Abas, B. Pingguan-Murphy and F. Xu, An integrated paper-based sample-to-answer biosensor for nucleic acid testing at the point of care, Lab Chip, 2016, 16(3), 611–621 RSC.
- R. Tang, H. Yang, Y. Gong, M. You, Z. Liu, J. R. Choi, T. Wen, Z. Qu, Q. Mei and F. Xu, A fully disposable and integrated paper-based device for nucleic acid extraction, amplification and detection, Lab Chip, 2017, 17(7), 1270–1279 RSC.
- J. Park, J. H. Shin and J. K. Park, Pressed paper-based dipstick for detection of foodborne pathogens with multistep reactions, Anal. Chem., 2016, 88(7), 3781–3788 CrossRef CAS PubMed.
- X. Li, F. Yang, J. X. Wong and H. Z. Yu, Integrated smartphone-app-chip system for on-site parts-per-billion-level colorimetric quantitation of aflatoxins, Anal. Chem., 2017, 89(17), 8908–8916 CrossRef CAS PubMed.
- X. Weng, G. Gaur and S. Neethirajan, Rapid detection of food allergens by microfluidics ELISA-based optical sensor, Biosensors, 2016, 6(2), 24 CrossRef PubMed.
- C. Zhao, G. Zhong, D. E. Kim, J. Liu and X. Liu, A portable lab-on-a-chip system for gold-nanoparticle-based colorimetric detection of metal ions in water, Biomicrofluidics, 2014, 8(5), 052107 CrossRef PubMed.
- X. Meng, C. W. Schultz, C. Cui, X. Li and H. Z. Yu, On-site chip-based colorimetric quantitation of organophosphorus pesticides using an office scanner, Sens. Actuators, B, 2015, 215, 577–583 CrossRef CAS.
- A. Chen, R. Wang, C. R. Bever, S. Xing, B. D. Hammock and T. Pan, Smartphone-interfaced lab-on-a-chip devices for field-deployable enzyme-linked immunosorbent assay, Biomicrofluidics, 2014, 8(6), 064101 CrossRef PubMed.
- L. L. Shen, G. R. Zhang, W. Li, M. Biesalski and B. J. Etzold, Modifier-free microfluidic electrochemical sensor for heavy-metal detection, ACS Omega, 2017, 2(8), 4593–4603 CrossRef CAS PubMed.
- M. L. Firdaus, A. Aprian, N. Meileza, M. Hitsmi, R. Elvia, L. Rahmidar and R. Khaydarov, Smartphone coupled with a paper-based colorimetric device for sensitive and portable mercury ion sensing, Chemosensors, 2019, 7(2), 25 CrossRef CAS.
- T. Moniz, C. R. Bassett, M. I. Almeida, S. D. Kolev, M. Rangel and R. B. Mesquita, Use of an ether-derived 3-hydroxy-4-pyridinone chelator as a new chromogenic reagent in the development of a microfluidic paper-based analytical device for Fe (III) determination in natural waters, Talanta, 2020, 214, 120887 CrossRef CAS PubMed.
- R. Wang, A. Prabhakar, R. A. Iglesias, X. Xian, X. Shan, F. Tsow, E. S. Forzani and N. Tao, A microfluidic-colorimetric sensor for continuous monitoring of reactive environmental chemicals, IEEE Sens. J., 2011, 12(5), 1529–1535 Search PubMed.
- P. Tang and G. Sun, Highly sensitive colorimetric paper sensor for methyl isothiocyanate (MITC): Using its toxicological reaction, Sens. Actuators, B, 2018, 261, 178–187 CrossRef CAS.
- X. L. Guo, Y. Chen, H. L. Jiang, X. B. Qiu and D. L. Yu, Smartphone-based microfluidic colorimetric sensor for gaseous formaldehyde determination with high sensitivity and selectivity, Sensors, 2018, 18(9), 3141 CrossRef PubMed.
- T. T. Nguyen, B. T. Huy and Y. I. Lee, Disposable colorimetric paper-based probe for the detection of amine-containing gases in aquatic sediments, ACS Omega, 2019, 4(7), 12665–12670 CrossRef CAS PubMed.
- K. L. Peters, I. Corbin, L. M. Kaufman, K. Zreibe, L. Blanes and B. R. McCord, Simultaneous colorimetric detection of improvised explosive compounds using microfluidic paper-based analytical devices (μPADs), Anal. Methods, 2015, 7(1), 63–70 RSC.
- P. G. Sutariya, H. Soni, S. A. Gandhi and A. Pandya, Single-step fluorescence recognition of As3+, Nd3+ and Br− using pyrene-linked calix [4] arene: application to real samples, computational modelling and paper-based device, New J. Chem., 2019, 43(2), 737–747 RSC.
- B. M. Jayawardane, W. Wongwilai, K. Grudpan, S. D. Kolev, M. W. Heaven, D. M. Nash and I. D. McKelvie, Evaluation and Application of a Paper-Based Device for the Determination of Reactive Phosphate in Soil Solution, J. Environ. Qual., 2014, 43(3), 1081–1085 CrossRef PubMed.
- B. M. Jayawardane, I. D. McKelvie and S. D. Kolev, Development of a gas-diffusion microfluidic paper-based analytical device (μPAD) for the determination of ammonia in wastewater samples, Anal. Chem., 2015, 87(9), 4621–4626 CrossRef CAS PubMed.
- E. Pellegrini, M. Contin, L. Vittori Antisari, G. Vianello, C. Ferronato and M. De Nobili, A new paper sensor method for field analysis of acid volatile sulfides in soils, Environ. Toxicol. Chem., 2018, 37(12), 3025–3031 CrossRef CAS PubMed.
- G. A. Suaifan and M. Zourob, Portable paper-based colorimetric nanoprobe for the detection of Stachybotrys chartarum using peptide labeled magnetic nanoparticles, Microchim.
Acta, 2019, 186, 1 CrossRef CAS PubMed.
- R. S. Alkasir, A. Rossner and S. Andreescu, Portable colorimetric paper-based biosensing device for the assessment of bisphenol A in indoor dust, Environ. Sci. Technol., 2015, 49(16), 9889–9897 CrossRef CAS PubMed.
- J. Sitanurak, N. Wangdi, T. Sonsa-Ard, S. Teerasong, T. Amornsakchai and D. Nacapricha, Simple and green method for direct quantification of hypochlorite in household bleach with membraneless gas-separation microfluidic paper-based analytical device, Talanta, 2018, 187, 91–98 CrossRef CAS PubMed.
- S. Nouanthavong, D. Nacapricha, C. S. Henry and Y. Sameenoi, Pesticide analysis using nanoceria-coated paper-based devices as a detection platform, Analyst, 2016, 141(5), 1837–1846 RSC.
- Y. Wu, Y. Sun, F. Xiao, Z. Wu and R. Yu, Sensitive inkjet printing paper-based colormetric strips for acetylcholinesterase inhibitors with indoxyl acetate substrate, Talanta, 2017, 162, 174–179 CrossRef CAS PubMed.
- A. Mohammadi, F. Ghasemi and M. R. Hormozi-Nezhad, Development of a paper-based plasmonic test strip for visual detection of methiocarb insecticide, IEEE Sens. J., 2017, 17(18), 6044–6049 CAS.
- A. Apilux, C. Isarankura-Na-Ayudhya, T. Tantimongcolwat and V. Prachayasittikul, based acetylcholinesterase inhibition assay combining a wet system for organophosphate and carbamate pesticides detection, Exp. Clin. Sci. J., 2015, 14, 307 Search PubMed.
- A. T. Jafry, H. Lee, A. P. Tenggara, H. Lim, Y. Moon, S. H. Kim, Y. Lee, S. M. Kim, S. Park, D. Byun and J. Lee, Double-sided electrohydrodynamic jet printing of two-dimensional electrode array in paper-based digital microfluidics, Sens. Actuators, B, 2019, 282, 831–837 CrossRef CAS.
- H. Sun, W. Li, Z. Z. Dong, C. Hu, C. H. Leung, D. L. Ma and K. Ren, A suspending-droplet mode paper-based microfluidic platform for low-cost, rapid, and convenient detection of lead (II) ions in liquid solution, Biosens. Bioelectron., 2018, 99, 361–367 CrossRef CAS PubMed.
- N. Fakhri, M. Hosseini and O. Tavakoli, Aptamer-based colorimetric determination of Pb2+ using a paper-based microfluidic platform, Anal. Methods, 2018, 10(36), 4438–4444 RSC.
- J. Dhavamani, L. H. Mujawar and M. S. El-Shahawi, Hand drawn paper-based optical assay plate for rapid and trace level determination of Ag+ in water, Sens. Actuators, B, 2018, 258, 321–330 CrossRef CAS.
- Y. Cui, X. Wang, Q. Zhang, H. Zhang, H. Li and M. Meyerhoff, Colorimetric copper ion sensing in solution phase and on paper substrate based on catalytic decomposition of S-nitrosothiol, Anal. Chim. Acta, 2019, 1053, 155–161 CrossRef CAS PubMed.
- X. Sun, B. Li, A. Qi, C. Tian, J. Han, Y. Shi, B. Lin and L. Chen, Improved assessment of accuracy and performance using a rotational paper-based device for multiplexed detection of heavy metals, Talanta, 2018, 178, 426–431 CrossRef CAS PubMed.
- H. Asano and Y. Shiraishi, Microfluidic paper-based analytical device for the determination of hexavalent chromium by photolithographic fabrication using a photomask printed with 3D printer, Anal. Sci., 2018, 34(1), 71–74 CrossRef CAS PubMed.
- J. P. Devadhasan and J. Kim, A chemically functionalized paper-based microfluidic platform for multiplex heavy metal detection, Sens. Actuators, B, 2018, 273, 18–24 CrossRef CAS.
- J. C. Hofstetter, J. B. Wydallis, G. Neymark, I. I. I. T. H. Reilly, J. Harrington and C. S. Henry, Quantitative colorimetric paper analytical devices based on radial distance measurements for aqueous metal determination, Analyst, 2018, 143(13), 3085–3090 RSC.
- W. Xu, X. Chen, S. Cai, J. Chen, Z. Xu, H. Jia and J. Chen, Superhydrophobic titania nanoparticles
for fabrication of paper-based analytical devices: An example of heavy metals assays, Talanta, 2018, 181, 333–339 CrossRef CAS PubMed.
- S. Karita and T. Kaneta, Chelate titrations of Ca2+ and Mg2+ using microfluidic paper-based analytical devices, Anal. Chim. Acta, 2016, 924, 60–67 CrossRef CAS PubMed.
- M. A. Ostad, A. Hajinia and T. Heidari, A novel direct and cost effective method for fabricating paper-based microfluidic device by commercial eye pencil and its application for determining simultaneous calcium and magnesium, Microchem. J., 2017, 133, 545–550 CrossRef CAS.
- X. Liu, Y. Yang, Q. Li, Z. Wang, X. Xing and Y. Wang, Portably colorimetric paper sensor based on ZnS quantum dots for semi-quantitative detection of Co2+ through the measurement of grey level, Sens. Actuators, B, 2018, 260, 1068–1075 CrossRef CAS.
- A. Yakoh, P. Rattanarat, W. Siangproh and O. Chailapakul, Simple and selective paper-based colorimetric sensor for determination of chloride ion in environmental samples using label-free silver nanoprisms, Talanta, 2018, 178, 134–140 CrossRef CAS PubMed.
- J. F. Silveira Petruci, P. C. Hauser and A. A. Cardoso, Colorimetric paper-based device for gaseous hydrogen cyanide quantification based on absorbance measurements, Sens. Actuators, B, 2018, 268, 392–397 CrossRef.
-
H. Kettler, K. White and S. J. Hawkes, Mapping the Landscape of Diagnostics for Sexually Transmitted Infections: Key Findings and Recommendations, World Health Organization, 2004 Search PubMed.
- J. A. Otoo and T. S. Schlappi, REASSURED multiplex diagnostics: a critical review and forecast, Biosensors, 2022, 12(2), 124 CrossRef CAS PubMed.
- K. J. Land, D. I. Boeras, X. S. Chen, A. R. Ramsay and R. W. Peeling, REASSURED diagnostics to inform disease control strategies, strengthen health systems and improve patient outcomes, Nat. Microbiol., 2019, 4(1), 46–54 CrossRef CAS PubMed.
- J. H. Cho, Y. Gao, J. Ryu and S. Choi, Portable, disposable, paper-based microbial fuel cell sensor utilizing freeze-dried bacteria for in situ water quality monitoring, ACS Omega, 2020, 5(23), 13940–13947 CrossRef CAS PubMed.
- J. H. Cho, Y. Gao and S. Choi, A portable, single-use, paper-based microbial fuel cell sensor for rapid, on-site water quality monitoring, Sensors, 2019, 19(24), 5452 CrossRef CAS PubMed.
- R. Kahhat, J. Kim, M. Xu, B. Allenby, E. Williams and P. Zhang, Exploring e-waste management systems in the United States, Resour., Conserv. Recycl., 2008, 52(7), 955–964 CrossRef.
- S. L. Percival, L. Suleman, C. Vuotto and G. Donelli, Healthcare-associated infections, medical devices and biofilms: risk, tolerance and control, J. Med. Microbiol., 2015, 64(4), 323–334 CrossRef PubMed.
- M. Tahernia, M. Mohammadifar, D. J. Hassett and S. Choi, A fully disposable 64-well papertronic sensing array for screening electroactive microorganisms, Nano Energy, 2019, 65, 104026 CrossRef CAS.
- L. Marle and G. M. Greenway, Microfluidic devices for environmental monitoring, TrAC, Trends Anal. Chem., 2005, 24(9), 795–802 CrossRef CAS.
- R. Fobel, A. E. Kirby, A. H. Ng, R. R. Farnood and A. R. Wheeler, Paper microfluidics goes digital, Adv. Mater., 2014, 26(18), 2838–2843 CrossRef CAS PubMed.
- M. Amatatongchai, J. Sitanurak, W. Sroysee, S. Sodanat, S. Chairam, P. Jarujamrus, D. Nacapricha and P. A. Lieberzeit, Highly sensitive and selective electrochemical paper-based device using a graphite screen-printed electrode modified with molecularly imprinted polymers coated Fe3O4@ Au@ SiO2 for serotonin determination, Anal. Chim. Acta, 2019, 1077, 255–265 CrossRef CAS PubMed.
- O. Skurtys and J. M. Aguilera, Applications of microfluidic devices in food engineering, Food Biophys., 2008, 3, 1–5 CrossRef.
- E. Noviana, D. B. Carrão, R. Pratiwi and C. S. Henry, Emerging applications of paper-based analytical devices for
drug analysis: A review, Anal. Chim. Acta, 2020, 1116, 70–90 CrossRef CAS PubMed.
Footnote |
† Adjunct Professor at the Department of Materials Science and Engineering, The Ohio State University, Columbus 43210, OH, USA. |
|
This journal is © The Royal Society of Chemistry 2024 |