Improving the performance of portable aerosol size spectrometers for building dense monitoring networks†
Received
29th November 2022
, Accepted 27th December 2022
First published on 28th December 2022
Abstract
The ideal particle number size distribution measurement instruments for building dense networks would be compact, easily maintained, and able to produce accurate results. This is challenging to achieve because when reducing the size of size spectrometers, their accuracy is often reduced as well. For existing portable size spectrometers, a large source of uncertainty is unipolar chargers due to their instability in the achieved charge distributions. To address this issue, we modified a set of commercial portable size spectrometer such that its unipolar charger is discarded and it measures charged particles of both polarities, a method that has proven to be efficient in reducing charging-related uncertainty for size spectrometers. We used indoor, outdoor, and NaCl aerosols as test aerosols and compared size distributions obtained using the modified and the original spectrometers with those measured using a set of reference size spectrometer. We demonstrate that size distributions obtained using the modified spectrometer were in better agreement with those obtained using the reference size spectrometer than the original one. By using the new charging method in portable size spectrometers, we improved their performance and reduced their size at the same time, making them a more suitable choice for building dense monitoring networks.
Environmental significance
Building dense networks for aerosol size distributions requires spectrometers that are compact, easily maintained, and able to produce accurate results. Portable SMPSs, a miniaturized version of the most state-of-the-art size spectrometers, have been developed. Unfortunately, these portable SMPSs often face untraceable uncertainty due to unipolar charging. Here we present a new charging method that enables such instruments to discard the chargers and measure size distributions of aerosols conditioned by natural air ions, with improved data accuracy. This method can be implemented in various portable SMPSs, enabling better applications in building dense networks, occupational hygiene, mobile exposure monitoring, and aircraft measurement.
|
1. Introduction
Aerosols are ubiquitous in the environment and have great impacts on air quality,1 climate change,2 and human health.3 When studying these effects, particle number size distribution (PNSD) is key information as many properties of aerosols are size dependent; for example, the potential of aerosols to act as cloud condensation nuclei (CCN).4 A commonly used instrument to conduct PNSD measurements is an electrical mobility size spectrometer (e.g., SMPS or DMPS).5,6 These state-of-the-art instruments that are designed for scientific uses can provide accurate size distributions, but are usually expensive, bulky, heavy and require high maintenance.7 These features have hindered them from being deployed in dense monitoring networks to obtain monitoring data with high spatial and temporal resolution, which is a future trend for aerosol size distribution monitoring. Building such networks would help collect consistent epidemiologic evidence to support the independent health effects of ultrafine particles,8 as well as improve aerosol-related mechanisms in weather and climate models.9
Compact, easily maintained size spectrometers that can produce accurate results would be ideal for building a dense network, although at this stage the former two features are achieved often by sacrificing accuracy to a certain extent.10 One type of instrument is based on the working principle of SMPSs, which consists of a charger that conditions the charge state of particles to a known distribution, a differential mobility analyzer (DMA) to classify positively or negatively charged particles according to their electrical mobilities, and a condensation particle counter (CPC) to count the selected particles in each mobility bin.6 These portable SMPSs have minimized the size of their charger, DMA, and CPC (some chose to replace the CPC with an electrometer), including a TSI NanoScan 3910,11 Kanomax portable aerosol mobility spectrometer (PAMS),12 Grimm mini wide range aerosol spectrometer (mini WRAS), and non-commercialized miniature electrical ultrafine particle sizer (mini-eUPS).7 Another type of instrument is based on the principle that the average charge on particles is approximately proportional to their diameters, and can be reduced to handheld size due to their simple constructions.13 These instruments can only obtain number concentrations and a number-weighted mean diameter,13,14 or measure size distribution in a limited size range with low resolution.15 In principle, portable SMPSs still measure particle concentrations for each known particle size selected using a DMA, rather than using the average charge to approximate the particle diameters which is performed using the second type of instrument. This enables portable SMPSs to provide size distributions with better accuracy, wider size range, and higher resolution, making them a more suitable choice for building a dense network.
For portable SMPS instruments, the sacrifice in accuracy is mostly caused by unipolar chargers, which are widely used in portable instruments because of their small size and high charging efficiency (Table 1). However, unlike bipolar chargers (also referred to as neutralizers) that can condition aerosols to a relatively stable charge distribution, the charge distribution achieved using unipolar chargers is rather unstable and strongly affected by the variation in the flow residence time, ion concentrations and properties, particle morphology, as well as the pre-existing charge on the measured aerosols.17,18 This could lead to discrepancies between the actual charge fractions and those used in size distribution data inversion, thus causing uncertainty in the retrieved size distributions. This has been observed in several intercomparison studies between portable SMPS instruments and reference instruments.19 Stabile et al.20 compared a NanoScan SMPS with a reference SMPS with fresh aggregated particles and found that the total particle concentrations are overestimated by one-fold, very likely caused by the inability of unipolar chargers to properly charge the aggregated particles. Fonseca et al.21 reported similar results that a NanoScan SMPS overestimated the number concentrations by over 20% of those measured using the reference CPC for agglomerated particles such as ZnO, spark-generated soot, and diesel soot particles. Uncertainty brought by unipolar chargers is usually large and untraceable, so reducing it would make portable SMPSs closer to the ideal pick for building dense networks.
Table 1 A summary of portable SMPSs
|
TSI nanoscan 3910 |
Kanomax PAMSa |
Grimm mini WRAS |
mini-eUPS |
PAMS provides two modes for measuring size distributions (denoted as ‘wide’ and ‘high’ here). In wide mode, the sheath flow rate of the DMA is set at 0.2 lpm and a wider size range can be measured, whereas the high mode provides users with higher size resolution and the sheath flow rate of the DMA is set at 0.4 lpm.16
|
Charger |
Unipolar corona charger |
Bipolar corona charger |
Unipolar charger |
Unipolar mini-plate particle charger |
Particle detector |
Isopropanol CPC |
Isopropanol CPC |
Faraday cup electrometer |
Mini-particle faraday cage with an electrometer |
Size range |
10–420 nm |
14.5–862.3 nm (wide), 10–433.7 nm (high) |
10–193 nm |
5–200 nm (sheath at 1.5 lpm) |
Size bins |
13 |
14 (wide), 27 (high) |
10 |
20 |
Time resolution |
60 s |
56 s (wide), 108 s (high) |
60 s |
60 s |
Dimensions (W × H × D) |
45 × 23 × 39 cm |
23 × 23 × 15 cm |
31 × 12 × 34 cm |
16.5 × 12.7 × 10 cm |
Weight |
8 kg |
5 kg |
7.6 kg |
1 kg |
Reference |
Tritscher et al.11 |
Kulkarni et al.12 |
Product datasheet |
Liu et al.7 |
A solution to solving charging-related uncertainty is by measuring charged particles of both polarities. The key is that during bipolar diffusion charging, the measured raw size distributions of both polarities can reflect the charging process and reveal the actual charge fractions.22 By using the actual charge fractions in data inversion, it can greatly reduce the uncertainty in retrieved PNSDs. It is further demonstrated that since natural air ions constantly condition the charge state of atmospheric aerosols,23 it is feasible to retrieve charge fractions of aerosols that are naturally charged and utilize this process to measure size distributions of outdoor aerosols.24 This new method has been supported by two years of atmospheric measurements, and natural air ions have shown rather stable properties and the capability of replacing conventional neutralizers. These findings lead to a possible solution to the uncertainty brought about by unipolar chargers for portable SMPSs. By measuring charged particles of both polarities, charge states of aerosols conditioned with natural air ions can be obtained and unipolar chargers will no longer be needed.
In this study, we demonstrate that by discarding the unipolar charger and using the new charging method, the performance of portable SMPS instruments can be improved. A TSI NanoScan SMPS was modified so that its unipolar charger is discarded and it measures charged particles of both polarities. A NanoScan SMPS was chosen as our object of modification because it has been more often studied in intercomparisons and more often used in various environments. Indoor, outdoor, and generated NaCl aerosols were used as test aerosols. Size distributions measured using the NanoScan SMPS before and after modification were compared with those measured using a bipolar SMPSs equipped with a neutralizer as the reference. It is shown that with the modified NanoScan SMPS we can obtain size distributions that are in better agreement with those obtained using the reference SMPS.
2. Methods
2.1 Modification of the NanoScan SMPS
The NanoScan SMPS (Model 3910, TSI corp.) is composed of an opposed flow unipolar diffusion charger,25 a radial DMA,26,27 and an isopropanol CPC (Fig. 1a). The sampled aerosol flow of 0.75 lpm is preconditioned with a cyclone (Dp50 = 550 nm) to remove larger particles. Then it enters the unipolar charger where it joins an opposed charger flow of 0.45 lpm that is filtered with both an active carbon filter and a HEPA filter. The aerosols are charged with positive ions in the unipolar charger, and 0.25 lpm of the sample flow then enters the radial DMA to be classified. The sheath flow of the radial DMA is 0.75 lpm. The size-classified particles then enter the CPC to be counted and exhausted. The NanoScan SMPS could measure particles of a certain size (single mode) or in the size range of 11.5–365.2 nm (geometric midpoints of the first and last bins) which are divided into 13 channels (scanning mode). In scanning mode, the voltage of the radial DMA was scanned upwards in 45 seconds (measurement) and downward in 15 seconds (retrace), enabling a time resolution of 60 seconds. The voltage was negative so that positively charged particles are measured, and a data inversion routine is used to retrieve the size distribution of sampled aerosols.
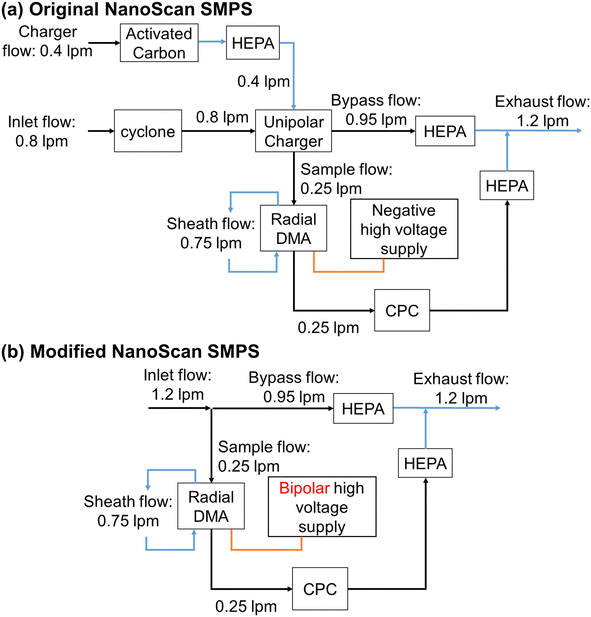 |
| Fig. 1 Flow schematic of (a) the original NanoScan SMPS with the main components, and (b) the modified NanoScan SMPS after discarding the unipolar charger and replacing the unipolar high voltage supply with a bipolar one. | |
To enable the NanoScan SMPS to measure charged particles of both polarities conditioned by natural air ions, we discarded the unipolar charger and replaced the unipolar high voltage supply with a bipolar one (Fig. 1b). This high voltage supply (Model 5HVA24-BP1, Advanced Energy Industries Inc.) provides both positive and negative high voltages in the range of −5000 to +5000 V. We also made a control system to regulate the voltage of the radial DMA and collect data from the CPC. After modification, the bipolar NanoScan can measure both positively and negatively charged particles in the size range of 11.8–297.1 nm, which are divided into 13 channels. During each cycle, the voltage was scanned from 5000 V to 10 V for 30 seconds, then −10 V to −5000 V for 30 seconds with 5 seconds in between for the polarity to switch, and 10 seconds of zeroing after the positive scanning, enabling a time resolution of 75 seconds.
To test whether our modification is successful, we calibrated the voltage of the power supply, the plumb time of the CPC, and the sizing accuracy of the DMA before use. We also confirmed that the collected CPC readings (analog pulses) and those downloaded from the instrument were consistent with each other. A combined factor of the transfer function, CPC detection efficiency, and penetration efficiency through the modified NanoScan SMPS were also measured with the setup shown in Fig. S1,† which will be incorporated into the data inversion routine.
2.2 Measurements
We compared the NanoScan SMPS with a set of well-calibrated bipolar SMPS as reference.24 It consisted of a soft X-ray neutralizer (Model 3087, TSI corp.), a long DMA (Model 3081, TSI corp.), and a butanol-based CPC (Model 3772, TSI corp.). The aerosol flow rate is 1 lpm, and the sheath flow rate for the long DMA is 10 lpm and controlled by a homemade sheath flow control box. The power supply (Model 10HVA24-BP1, Advanced Energy Industries Inc.) provides both positive and negative high voltages for the DMA and both negatively and positively charged particles in the size range of 7.4–286 nm are measured, which are divided into 30 channels. A comparison of the original NanoScan SMPS, the modified NanoScan SMPS, and the reference SMPS in terms of their operation specifications is shown in Table 2.
Table 2 Specifications of instruments used in this study
ID |
Size rangea (nm) |
Time resolution |
Sample flow rate (lpm) |
Sheath flow rate (lpm) |
High voltage supply |
Other settings |
The geometric midpoints of the first and last size channels are used here to denote the size range.
|
Original NanoScan SMPS |
11.5–365.2 |
60 s |
0.25 |
0.75 |
Unipolar |
Unipolar charger |
Modified NanoScan SMPS |
11.8–297.1 |
75 s |
0.25 |
0.75 |
Bipolar |
No charger |
Reference SMPS |
7.4–286 |
5 min |
1 |
10 |
Bipolar |
Bipolar charger |
Three types of aerosols were tested as the reference SMPS and NanoScan SMPS were operated in parallel. The NanoScan SMPS was tested both before and after modification. Indoor aerosols in the lab, outdoor aerosols, and polydisperse NaCl aerosols were chosen as test aerosols, representing aerosols in different environments with varying concentration levels. Both indoor and outdoor aerosols were sampled in a laboratory located on the campus of Tsinghua University.28 The polydisperse NaCl aerosols were generated using a home-made collision atomizer with a diffusion dryer (Fig. 2). A neutralizer that conditions the charge state of the NaCl aerosols can be turned on or off, representing two different charging schemes. The NaCl aerosols are charged by colliding with bipolar ions (bipolar diffusion charging) with the neutralizer turned on, and by spray electrification with the neutralizer turned off.29 Note that bipolar diffusion charging of the measured aerosols is a prerequisite for our method of obtaining charge fractions from measured raw size distributions of both polarities to work.
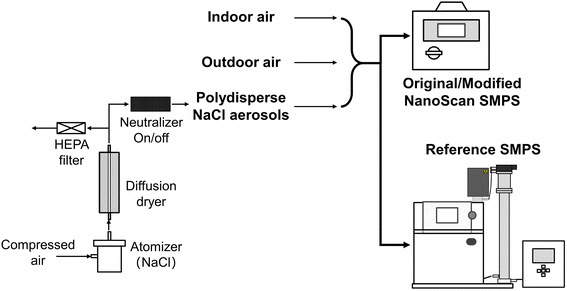 |
| Fig. 2 Schematic of the measurement setup in this study. Indoor aerosols, outdoor aerosols, and polydisperse NaCl aerosols were tested in turn as either the original or the modified NanoScan SMPS were operated in parallel with the reference SMPS. | |
To compare the original NanoScan SMPS and the reference SMPS, indoor aerosols and outdoor aerosols were measured for 48 hours each. For polydisperse NaCl aerosols, the measurement lasted for 15 minutes so that three replicate tests were obtained for the reference SMPS. When comparing the modified NanoScan SMPS and the reference SMPS, indoor aerosols and outdoor aerosols were measured for 46 hours each, and polydisperse NaCl aerosols were measured for 15 minutes.
2.3 Data analysis
With the bipolar data from the reference SMPS and the modified NanoScan SMPS, we retrieved PNSDs with actual charge fractions calculated using measured raw particle number concentrations of both polarities. We first obtained the ion mobility ratio weighted using ion concentrations, denoted as x: | 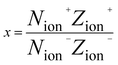 | (1) |
where N±ion is the concentration of positive or negative ions (cm−3) and Z±ion is the mobility of positive or negative ions (cm2 V−1 s−1).
With the obtained ion mobility ratios, charge fractions are then calculated using a modified Gunn and Woessner formula proposed by Chen & Jiang,22 and PNSDs in the form of dN/dlogDp are retrieved with a linear inversion algorithm.30 Note that the charge fractions were calculated for each scan, and the whole inversion routine would take less than 3 seconds for the modified NanoScan SMPS (less than its voltage retrace time of 15 seconds), which can be conveniently incorporated into existing SMPSs. For the original NanoScan SMPS, we downloaded the stored number concentrations of each size bin from the instrument. Loss correction was performed and the PNSDs in the form of dN/dlogDp were calculated from the concentration data.
To compare the PNSDs in detail, we calculated several parameters that can characterize them. Number concentrations in nucleation mode, Aitken mode, and accumulation mode as well as the whole measured size range are integrated from the PNSDs. Geometric mean diameters and geometric standard deviations are also calculated based on the obtained PNSDs. More details can be found in the ESI.†
3. Results and discussion
3.1 Improved indoor and outdoor PNSDs using the modified NanoScan SMPS
For both indoor and outdoor aerosols, the PNSDs measured using the modified NanoScan SMPS showed good consistency with those measured using the reference SMPS (Fig. S3† and Fig. 3). As shown by the outdoor aerosol results of all collected data (46 hours in total), the modified NanoScan SMPS and the reference SMPS both detected new particle formation events and captured the time evolution of the PNSDs (Fig. 3a and b). More specifically, both instruments are consistent with each other in terms of averaged size distribution and integrated particle number concentrations in the size ranges of 12–25 nm, 25–90 nm, 90–297 nm and 12–297 nm (Fig. 3c–g). Number concentrations in different size ranges are mostly within ±20% of those measured using the reference SMPS. The same level of consistency was also observed when indoor aerosols were measured (Fig. S3†), with the occasional exception when a new particle formation event was observed indoors (measurement conducted on April 22nd). The cause of this deviation remains uncertain, and more studies targeting the measurement of indoor aerosols during new particle formation events are needed. Nevertheless, PNSDs measured using the modified NanoScan SMPS are generally consistent with those measured using the reference SMPS.
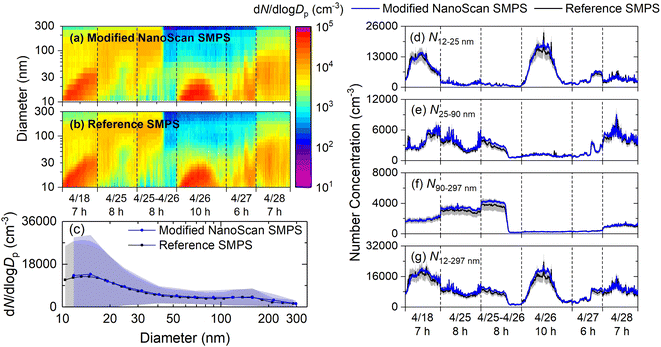 |
| Fig. 3 PNSDs of outdoor aerosols for a total of 46 hours using (a) the modified NanoScan SMPS and (b) the reference SMPS. (c) The averaged size distributions and standard deviations during the measurement period, as denoted by the line and shaded area, respectively. Integrated number concentrations in the size ranges of (d) 12–25 nm, (e) 25–90 nm, (f) 90–297 nm, and (g) 12–297 nm of measured size distributions are shown, and the shaded area represents the ±20% range of the reference SMPS. The time resolution for the PNSDs and thus the calculated parameters measured using the original NanoScan SMPS and the reference SMPS are 75 s and 5 min, respectively. | |
The PNSDs of both indoor and outdoor aerosols measured using the original NanoScan SMPS showed poor consistency with those measured using the reference SMPS. The original NanoScan SMPS tends to underestimate particles in the nucleation mode and accumulation mode, and overestimate particles in the Aitken mode compared to the reference SMPS (Fig. S4 and S5†). Size-segregated number concentrations in different size ranges can deviate much from those measured using the reference SMPS, but the total number concentrations in the whole size range are mostly within ±20% of those measured using the reference SMPS. Similar results were observed by Ahlawat et al.19 when comparing ten sets of NanoScan SMPSs with a reference SMPS during four hours of outdoor aerosol measurement. Their averaged PNSD was bimodal, with an underestimation of 60% in the PNC of the first mode (around 20–25 nm) and an overestimation of 120% in the PNC of the second mode (around 70–80 nm). They also reported that the total number concentrations measured using the NanoScan SMPS instruments were mostly within the ±20% range of those measured using the reference CPC.
These collected data for both indoor and outdoor aerosols are further analyzed to illustrate the improved accuracy of the modified NanoScan SMPS. For the studied parameters (denoted as P) including integrated number concentrations in different size ranges, geometric mean diameters, and geometric standard deviations, Pmodified/Preference are mostly within the range of 0.9–1.1 (Fig. 4a), while Poriginal/Preference deviated much from 1.0 (Fig. 4b). Obvious underestimation for nucleation mode particle concentrations (median value for Poriginal/Preference is 0.72) and overestimation for accumulation mode particle concentrations (median value for Poriginal/Preference is 1.40) can be observed. When these data are presented in scatter plots, the data points for the modified NanoScan SMPS and the reference SMPS are also distributed near the equal dashed lines (Fig. S6†), while for the original NanoScan SMPS and the reference SMPS the data points are more scattered and away from the equal dashed lines (Fig. S7†).
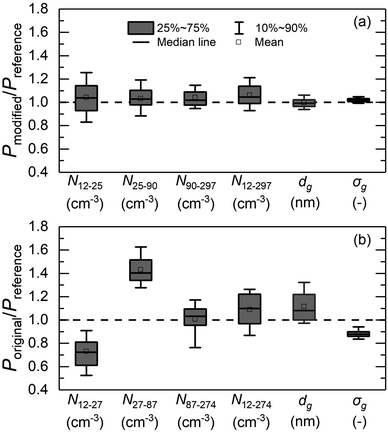 |
| Fig. 4 The ratio of different parameters between (a) the modified NanoScan SMPS and the reference SMPS and (b) the original NanoScan SMPS and the reference SMPS for all periods when outdoor and indoor aerosols were measured. The compared parameter for each box (denote as P) is shown in the x-axis. The dashed lines are for guiding the eye. | |
These results indicate that large uncertainty can be observed for PNSDs measured using the original NanoScan SMPS as suggested by several other performance studies.16,21,31 By modifying the NanoScan SMPS, it can now provide more accurate PNSD results, with reduced size and maintenance needed as well.
3.2 Implication
Finding a balance between the accuracy of measured PNSDs and compactness along with easy maintenance is one of the main considerations when choosing the instrument for building dense monitoring networks. Ideal instruments would have high scores from both perspectives, for example, by being as small and easily maintained as hand-held devices and able to provide PNSDs as accurate as those measured using an SMPS (Fig. 5). Such instruments have not yet been developed to the best of our knowledge. We do, however, see potential in portable SMPS that have managed to reduce the size to a reasonable range, and can obtain quite reliable PNSD results (compared to handheld devices) despite the uncertainty caused by unipolar charging. We have shown in this study that by discarding the unipolar charger and measuring charged particles of both polarities, the modified NanoScan SMPS can be more compact and also obtain more accurate results. With one less part which required high voltage and clean sheath air to protect the charger needle, it also reduces the maintenance needed for such instruments. This can be further applied to other models of portable SMPSs, making this type of instrument closer to the ideal one for building dense networks. What's more, these modified portable SMPSs can also find applications in occupational hygiene, mobile exposure monitoring, and aircraft measurement.
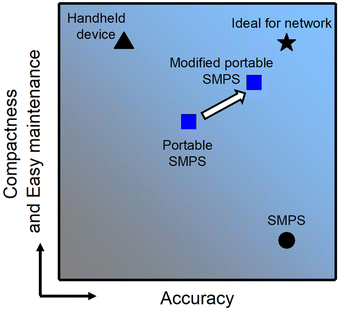 |
| Fig. 5 Conceptual image of different types of size distribution measurement instruments rated with accuracy (x-axis) and compactness along with easy maintenance (y-axis). | |
3.3 Limitation when measuring aerosols charged by other mechanisms
A prerequisite for a modified NanoScan SMPS to work properly is that the charge state of measured aerosols be conditioned by colliding with bipolar ions.22 When this assumption is not met, the calculated charge fractions can deviate, leading to deviations in the obtained PNSDs. For example, when measuring polydisperse NaCl aerosols that are charged by spray electrification as they are atomized29,32 (Fig. 2, neutralizer turned off), the modified NanoScan SMPS would fail to produce correct PNSD results (Fig. 6a). If we turn on the neutralizer so that the prerequisite is met, the PNSDs of polydisperse NaCl aerosols can be effectively measured using the modified NanoScan SMPS, even for concentrations as high as 1.8 × 105 cm−3 (Fig. 6b). These results indicate a limitation in extreme situations where a modified NanoScan SMPS is used to measure aerosols whose charge states are not conditioned by colliding with bipolar ions. Fortunately, this prerequisite can be met for indoor and outdoor environments where most of the dense network nodes would be placed.
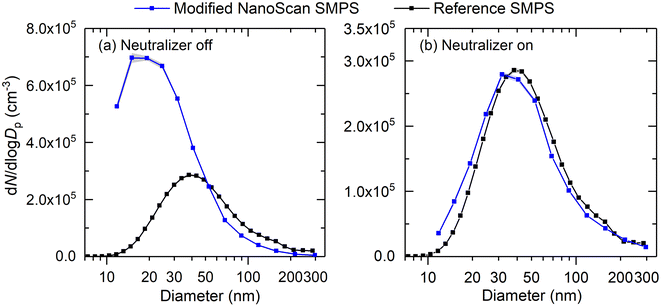 |
| Fig. 6 PNSDs measured using the reference SMPS and modified NanoScan SMPS of (a) polydisperse NaCl aerosols without pre-conditioning and (b) polydisperse NaCl aerosols pre-conditioned with a neutralizer. | |
4. Conclusion
Unipolar chargers are widely used for portable SMPS instruments and have caused uncertainty in the obtained size distributions due to their unstable charging performance. We demonstrate that it is feasible to discard the unipolar charger and obtain size distributions that are in better agreement with those obtained using a reference SMPS. This is achieved by measuring charged particles of both polarities and obtaining the actual charge distributions of aerosols conditioned by natural air ions. This improvement has proven to be a solution to the uncertainty brought about by unipolar chargers for both indoor and outdoor aerosols. By discarding the charger, it reduces the size and simplifies the maintenance needed for these instruments, making them much more suitable for applications in building networks in a variety of different environments.
Conflicts of interest
There are no conflicts of interest to declare.
Acknowledgements
Financial support from the National Key R&D Program of China (2018YFE0106800) and the National Natural Science Foundation of China (22188102) is acknowledged. We thank Prof. Guofeng Shen for generously sharing their instrument. Technical support from Qiang Zhang at Tsinghua is acknowledged.
References
- X. Yang, C. F. Zhao, L. J. Zhou, Y. Wang and X. H. Liu, Distinct impact of different types of aerosols on surface solar radiation in China, J. Geophys. Res. Atmos., 2016, 121, 6459–6471 CrossRef.
- C. F. Zhao, Y. K. Yang, H. Fan, J. P. Huang, Y. F. Fu, X. Y. Zhang, S. C. Kang, Z. Y. Cong, H. Letu and M. Menenti, Aerosol characteristics and impacts on weather and climate over the Tibetan Plateau, Natl. Sci. Rev., 2020, 7, 492–495 CrossRef CAS PubMed.
- X. C. Yang, Y. Wang, C. F. Zhao, H. Fan, Y. K. Yang, Y. L. Chi, L. X. Shen and X. Yan, Health risk and disease burden attributable to long-term global fine-mode particles, Chemosphere, 2022, 287 Search PubMed.
- J. R. Pierce and P. J. Adams, Efficiency of cloud condensation nuclei formation from ultrafine particles, Atmos. Chem. Phys., 2007, 7, 1367–1379 CrossRef CAS.
- S. C. Wang and R. C. Flagan, Scanning Electrical Mobility Spectrometer, Aerosol Sci. Technol., 1990, 13, 230–240 CrossRef CAS.
- A. Wiedensohler, W. Birmili, A. Nowak, A. Sonntag, K. Weinhold, M. Merkel, B. Wehner, T. Tuch, S. Pfeifer, M. Fiebig, A. M. Fjaraa, E. Asmi, K. Sellegri, R. Depuy, H. Venzac, P. Villani, P. Laj, P. Aalto, J. A. Ogren, E. Swietlicki, P. Williams, P. Roldin, P. Quincey, C. Huglin, R. Fierz-Schmidhauser, M. Gysel, E. Weingartner, F. Riccobono, S. Santos, C. Gruning, K. Faloon, D. Beddows, R. M. Harrison, C. Monahan, S. G. Jennings, C. D. O'Dowd, A. Marinoni, H. G. Horn, L. Keck, J. Jiang, J. Scheckman, P. H. McMurry, Z. Deng, C. S. Zhao, M. Moerman, B. Henzing, G. de Leeuw, G. Loschau and S. Bastian, Mobility particle size spectrometers: harmonization of technical standards and data structure to facilitate high quality long-term observations of atmospheric particle number size distributions, Atmos. Meas. Tech., 2012, 5, 657–685 CrossRef CAS.
- Q. L. Liu, D. Liu, X. T. Chen, Q. Zhang, J. Jiang and D. R. Chen, A Cost-effective, Miniature Electrical Ultrafine Particle Sizer (mini-eUPS) for Ultrafine Particle (UFP) Monitoring Network, Aerosol Air Qual. Res., 2020, 20, 231–241 CrossRef CAS.
- S. Ohlwein, R. Kappeler, M. K. Joss, N. Kunzli and B. Hoffmann, Health effects of ultrafine particles: a systematic literature review update of epidemiological evidence, Int J Public Health, 2019, 64, 547–559 CrossRef PubMed.
-
WMO/GAW Report No. 207, Recommendations for a Composite Surface-Based Aerosol Network, Geneva, Switzerland, 2012 Search PubMed.
- C. Brochot, F. Haghighat and A. Bahloul, Comparative Performance of the NanoScan and the Classic SMPS in Determining N95 Filtering Facepiece Efficiency Against Nanoparticles, Aerosol Sci. Eng., 2020, 4, 178–191 CrossRef.
- T. Tritscher, M. Beeston, A. F. Zerrath, S. Elzey, T. J. Krinke, E. Filimundi and O. F. Bischof, NanoScan SMPS - A Novel, Portable Nanoparticle Sizing and Counting Instrument, J. Phys.: Conf. Ser., 2013, 429 Search PubMed.
- P. Kulkarni, C. L. Qi and N. Fukushima, Development of portable aerosol mobility spectrometer for personal and mobile aerosol measurement, Aerosol Sci. Eng., 2016, 50, 1167–1179 CrossRef CAS PubMed.
- M. Fierz, C. Houle, P. Steigmeier and H. Burtscher, Design, Calibration, and Field Performance of a Miniature Diffusion Size Classifier, Aerosol Sci. Eng., 2011, 45, 1–10 CrossRef CAS.
- J. Marra, M. Voetz and H. J. Kiesling, Monitor for detecting and assessing exposure to airborne nanoparticles, J. Nanopart. Res., 2010, 12, 21–37 CrossRef CAS.
- C. Zhang, D. Wang, R. Zhu, W. Yang and P. Jiang, A Miniature Aerosol Sensor for Detecting Polydisperse Airborne Ultrafine Particles, Sensors, 2017, 17, 929 CrossRef PubMed.
- T. C. Hsiao, Y. C. Lee, K. C. Chen, W. C. Ye, K. Sopajaree and Y. I. Tsai, Experimental Comparison of Two Portable and Real-Time Size Distribution Analyzers for Nano/Submicron Aerosol Measurements, Aerosol Air Qual. Res., 2016, 16, 919–929 CrossRef CAS.
- C. L. Qi and P. Kulkarni, Miniature Dual-Corona Ionizer for Bipolar Charging of Aerosol, Aerosol Sci. Technol., 2013, 47, 81–92 CrossRef CAS PubMed.
- H. Kaminski, T. A. J. Kuhlbusch, S. Rath, U. Gotz, M. Sprenger, D. Wels, J. Polloczek, V. Bachmann, N. Dziurowitz, H. J. Kiesling, A. Schwiegelshohn, C. Monz, D. Dahmann and C. Asbach, Comparability of mobility particle sizers and diffusion chargers, J. Aerosol Sci., 2013, 57, 156–178 CrossRef CAS.
- A. Ahlawat, K. Weinhold, J. Marval, P. Tronville, A. Leskinen, M. Komppula, H. Gerwig, L. Gerling, S. Weber, R. B. Jørgensen, T. N. Jensen, M. Merizak, U. Vogt, C. Ribalta, M. Viana, A. Schmitz, M. Chiesa, G. Gerosa, L. Keck, M. Pesch, G. Steiner, T. Krinke, T. Tritscher, W. Birmili and A. Wiedensohler, Performance analysis of the NanoScan SMPS and the Mini WRAS Ultrafine Aerosol Particle Size Spectrometers, Atmos. Meas. Tech. Discuss., 2022, 2022, 1–26 Search PubMed.
- L. Stabile, E. Cauda, S. Marini and G. Buonanno, Metrological Assessment of a Portable Analyzer for Monitoring the Particle Size Distribution of Ultrafine Particles, Ann. Occup. Hyg., 2014, 58, 860–876 CAS.
- A. S. Fonseca, M. Viana, N. Perez, A. Alastuey, X. Querol, H. Kaminski, A. M. Todea, C. Monz and C. Asbach, Intercomparison of a portable and two stationary mobility particle sizers for nanoscale aerosol measurements, Aerosol Sci. Technol., 2016, 50, 653–668 CrossRef CAS.
- X. T. Chen and J. Jiang, Retrieving the ion mobility ratio and aerosol charge fractions for a neutralizer in real-world applications, Aerosol Sci. Technol., 2018, 52, 1145–1155 CrossRef CAS.
- R. G. Harrison and K. S. Carslaw, Ion-aerosol-cloud processes in the lower atmosphere, Rev. Geophys., 2003, 41, 26 CrossRef.
- Y. R. Li, X. T. Chen and J. Jiang, Measuring size distributions of atmospheric aerosols using natural air ions, Aerosol Sci. Technol., 2022, 56, 655–664 CrossRef CAS.
- A. Medved, F. Dorman, S. L. Kaufman and A. Pöcher, A new corona-based charger for aerosol particles, J. Aerosol Sci., 2000, 31, 616–617 CrossRef.
- S. H. Zhang, Y. Akutsu, L. M. Russell, R. C. Flagan and J. H. Seinfeld, Radial Differential Mobility Analyzer, Aerosol Sci. Technol., 1995, 23, 357–372 CrossRef CAS.
- H. Fissan, A. Pocher, S. Neumann, D. Boulaud and M. Pourprix, Analytical and empirical transfer functions of a simplified spectrometre de mobilite electrique circulaire (SMEC) for nano particles, J. Aerosol Sci., 1998, 29, 289–293 CrossRef CAS.
- Z. J. An, X. Li, Y. Yuan, F. K. Duan and J. Jiang, Large contribution of non-priority PAHs in atmospheric fine particles: Insights from time-resolved measurement and nontarget analysis, Environ. Int., 2022, 163 Search PubMed.
- B. Forsyth, B. Y. H. Liu and F. J. Romay, Particle charge distribution measurement for commonly generated laboratory aerosols, Aerosol Sci. Technol., 1998, 28, 489–501 CrossRef CAS.
- D. E. Hagen and D. J. Alofs, Linear Inversion Method to Obtain Aerosol Size Distributions from Measurements with a Differential Mobility Analyzer, Aerosol Sci. Technol., 1983, 2, 465–475 CrossRef.
- M. Yamada, M. Takaya and I. Ogura, Performance evaluation of newly developed portable aerosol sizers used for nanomaterial aerosol measurements, Ind. Health, 2015, 53, 511–516 CrossRef CAS PubMed.
- T. S. Chadha, S. Chattopadhyay, C. Venkataraman and P. Biswas, Study of the Charge Distribution on Liposome Particles Aerosolized by Air-Jet Atomization, J. Aerosol Med. Pulm. Drug Delivery, 2012, 25, 355–364 CrossRef CAS PubMed.
|
This journal is © The Royal Society of Chemistry 2023 |