DOI:
10.1039/D2QI01007K
(Research Article)
Inorg. Chem. Front., 2022,
9, 5616-5623
Assembly of cyclic ferrocene-sensitized titanium-oxo clusters with excellent photoelectrochemical activity†
Received
8th May 2022
, Accepted 31st August 2022
First published on 1st September 2022
Abstract
The development of crystalline titanium-oxo clusters has made great progress in recent years. However, the geometric assembly of titanium-oxo clusters is still very challenging. Herein, we report the assembly of two cyclic titanium-oxo clusters with ferrocene connectors, formulated as Ti12(μ3-O)4(Fcdc)4(OiPr)32·0.5CH2Cl2 (Fcdc = 1,1′-ferrocenedicarboxylic acid; Ti12Fcdc4) and Ti18(μ2-O)6(μ3-O)6(Fcdc)2(L1)2(L2)4(OiPr)34·2HOiPr (L1 = isonicotinic acid; L2 = salicylhydroxamic acid; Ti18Fcdc2). Single-crystal X-ray diffraction studies demonstrate that ferrocene with two carboxylic arms can adhere to the surface of {Ti6} and/or {Ti3} units, resulting in fascinating cyclic structures of Ti12Fcdc4 and Ti18Fcdc2. Notably, ferrocene ligands can not only serve as connectors to induce the geometric assembly of Ti-oxo clusters, but can also act as photosensitizers to greatly improve the light absorption behavior of the resulting compounds. Furthermore, the DFT calculation results suggest that the HOMO → LUMO transition mainly involves ferrocenyl group → Ti-oxo core charge transfer. Using the two ferrocene-containing clusters as electrode precursors, both electrodes exhibited excellent photocurrent responses. Our work opens a new way for the geometric assembly of titanium-oxo clusters and also contributes to the deep understanding of the structure–property relationships of sensitized Ti-based materials.
Introduction
Recently, crystalline titanium-oxo cluster materials have attracted a significant amount of attention.1–8 The main motivation not only comes from their potential applications in photocatalysis and photoelectric conversion, but also lies in their accurate atomic structure which is beneficial for theoretical calculations and mechanism studies.9–14 In the past decade, crystalline titanium-oxo clusters have achieved rapid growth in their structural library.15–25 Although some attractive titanium-oxo cluster architectures were assembly synthesized and characterized by X-ray diffraction,26–35 such as cages, prisms, and cyclic structures, the rational design and geometric assembly of titanium-oxo clusters is still very challenging. Various synthesis parameters, including temperature, reactant ratio, solvent, and coordinating ligands, will directly affect the assembly of titanium-oxo clusters.36–40 Among them, the coordinating ligands as one of the most important raw materials play crucial roles in the assembly of titanium-oxo clusters.41–49
The metallocene-based molecule 1,1′-ferrocenedicarboxylic acid (FcdcH2) is a potential coordinating ligand. The FcdcH2 connector can display multiple conformations formed by the different angles between the carboxylate groups, which can be helpful for the assembly of complexes with fascinating geometries.50–52 Additionally, ferrocene-containing complexes, which are a class of potential excellent photoelectric materials, usually show narrow band gaps and excellent photoelectric responses.53–55 However, as far as we know, only a few titanium-oxo clusters with FcdcH2 ligands have been reported to date, and they also show broad light absorption and efficient charge transfer.56–58 Based on the above considerations, we hope to effectively regulate the structural geometry and light absorption of titanium-oxo clusters by employing FcdcH2 as a bridging ligand, thereby realizing the controllable assembly and photoelectric applications of titanium-oxo clusters containing ferrocene connectors.
Herein, we successfully synthesized two cyclic titanium-oxo clusters with FcdcH2 connectors, formulated as Ti12(μ3-O)4(Fcdc)4(OiPr)32·0.5CH2Cl2 (Fcdc = 1,1′-ferrocenedicarboxylic acid; Ti12Fcdc4) and Ti18(μ2-O)6(μ3-O)6(Fcdc)2(L1)2(L2)4(OiPr)34·2HOiPr (L1 = isonicotinic acid; L2 = salicylhydroxamic acid; Ti18Fcdc2). Single-crystal X-ray diffraction studies demonstrate that the {Ti6} and/or {Ti3} units are connected by bridging FcdcH2 connectors to generate fascinating cyclic structures of Ti12Fcdc4 and Ti18Fcdc2. We found that ferrocene ligands can not only serve as connectors to induce the assembly of titanium-oxo clusters, but can also act as photosensitizers to greatly improve the light absorption behavior of the resulting compounds. The DFT calculation results suggest that the HOMO → LUMO transition mainly involves ferrocenyl group → Ti-oxo core charge transfer. Using the two compounds as electrode precursors, both electrodes exhibited excellent photocurrent responses.
Experimental
Synthesis of Ti12(μ3-O)4(Fcdc)4(OiPr)32·0.5CH2Cl2 (1, Ti12Fcdc4)
To a 23 mL Teflon-lined autoclave were added 55 mg of FcdcH2 (0.2 mmol) and 3 mL of CH2Cl2, and then 100 μL of Ti(OiPr)4 (0.3 mmol) was added. After stirring for 10 min, the mixture was transferred to an oven at 100 °C for 72 h. After cooling, red crystals of Ti12Fcdc4 were obtained (76% yield based on Ti(OiPr)4). IR data (cm−1): 1633 (m), 1491 (s), 1394 (s), 1358 (m), 1194 (m), 1029 (w), 924 (w), 787 (m), 618 (w), 576 (w), 521 (w).
Synthesis of Ti18(μ2-O)6(μ3-O)6(Fcdc)2(L1)2(L2)4(OiPr)34·2HOiPr (2, Ti18Fcdc2)
To a 23 mL Teflon-lined autoclave were added 55 mg of FcdcH2 (0.2 mmol), 77 mg of salicylhydroxamic acid (0.5 mmol), 62 mg of isonicotinic acid (0.5 mmol), and 5 mL of isopropanol, and then 100 μL of Ti(OiPr)4 (0.3 mmol) was added. After stirring for 10 min, the mixture was transferred to an oven at 100 °C for 72 h. After cooling, yellow crystals of Ti18Fcdc2 were obtained (68% yield based on Ti(OiPr)4). IR data (cm−1): 1600 (s), 1577 (m), 1509 (s), 1399 (s), 1358 (w), 1312 (w), 1267 (m), 1249 (w), 1194 (w), 1157 (w), 1102 (w), 1033 (m), 920 (w), 763 (m), 677 (m), 613 (m), 521 (w).
Results and discussion
Syntheses
Crystals of Ti12Fcdc4 and Ti18Fcdc2 were obtained by a solvothermal reaction. Fig. 1 displays the crystal pictures of Ti12Fcdc4 and Ti18Fcdc2. During synthesis, we found that the solvent has an important effect on the assembly synthesis of Ti12Fcdc4. When dichloromethane was replaced by isopropanol, only a microcrystalline sample could be obtained. As for the synthesis of Ti18Fcdc2, salicylhydroxamic acid as an auxiliary ligand plays a significant role in the reaction system. If there is no salicylhydroxamic acid, only a solution sample could be obtained. For the discussion of the molecular structures, intrinsic characteristics, and photoelectric behaviors of these two Ti-oxo clusters containing ferrocene connectors, see the following sections.
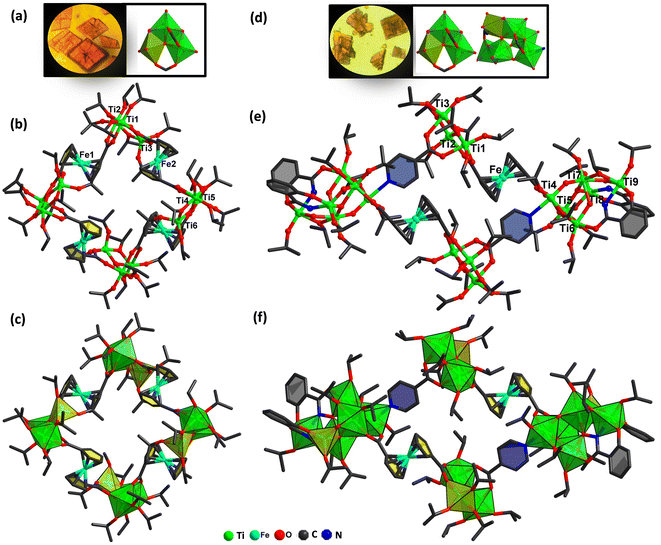 |
| Fig. 1 (a) Crystal photograph of Ti12Fcdc4; inset is the {Ti3} unit of Ti12Fcdc4. (b) Ball–stick view of Ti12Fcdc4. (c) Polyhedral view of Ti12Fcdc4. (d) Crystal photograph of Ti18Fcdc2; insets are {Ti3} and {Ti6} units of Ti18Fcdc4. (e) Ball–stick view of Ti18Fcdc2. (f) Polyhedral view of Ti18Fcdc2. | |
Structures
X-ray single crystal diffraction analysis reveals that Ti12Fcdc4 crystallizes in the orthorhombic system, space group Pbcn, and the structure of Ti12Fcdc4 consists of twelve Ti4+ ions, four Fcdc2− connectors, four μ3-O2−, thirty-two –OiPr− ions, and half a free CH2Cl2 molecule. In the structure of Ti12Fcdc4, three Ti atoms are linked by one μ3-O atom to form a planar triangle [Ti3(μ3-O)]10+ unit, and four [Ti3(μ3-O)]10+ units are connected by four bridging Fcdc2− linkers to form a closed-loop structure of Ti12Fcdc4 (Fig. 1b and S1†). Notably, the two carboxylate groups of each Fcdc2− connector present an angle of 142.76°, and each carboxylate group was coordinated to two Ti atoms in the [Ti3(μ3-O)]10+ unit. Except for the Fcdc2− groups, the outer surface of each [Ti3(μ3-O)]10+ unit is surrounded by two bridging isopropyl groups and six terminal isopropyl groups. In the [Ti3(μ3-O)]10+ unit, Ti1 and Ti2 (Ti4 and Ti5) atoms are six-coordinated in an octahedral environment, while the Ti3 (Ti6) atom is five-coordinated in a hexahedral environment (Fig. 1c). Furthermore, the space-filling structure and packing structure of Ti12Fcdc4 are shown in Fig. 2a and b. The distance between the two adjacent [Ti3(μ3-O)]10+ units in Ti12Fcdc4 is about 4.98 Å.
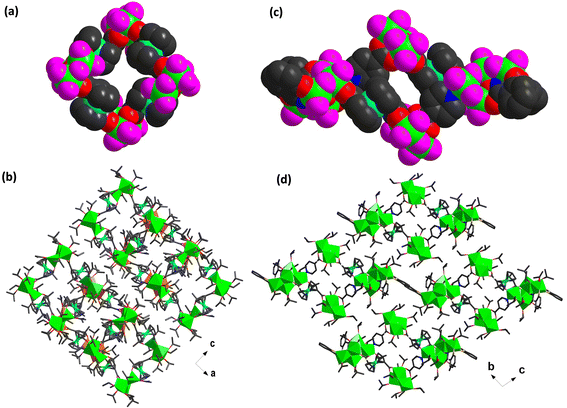 |
| Fig. 2 Space-filling structure and packing structure of Ti12Fcdc4 (a and b) and Ti18Fcdc2 (c and d). | |
X-ray single crystal diffraction analysis revealed that Ti18Fcdc2 crystallizes in the triclinic system, space group P
. In the structure of Ti18Fcdc2, two [Ti3(μ3-O)]10+ units and two [Ti6(μ2-O)3(μ3-O)2]14+ units are connected by two Fcdc2− and two isonicotinic acid connectors, resulting in the rectangular structure of Ti18Fcdc2 (Fig. 1e and S2†). The two carboxylate groups of each Fcdc2− connector present an angle of 135.47°, and four O atoms of the Fcdc2− linker were coordinated with Ti1, Ti2 in the [Ti3(μ3-O)]10+ unit and Ti4, Ti5 in the [Ti6(μ2-O)3(μ3-O)2]14+ unit, respectively. One N atom and two O atoms of isonicotinic acid were coordinated to Ti4 in the [Ti6(μ2-O)3(μ3-O)2]14+ unit and Ti2, Ti3 in the [Ti3(μ3-O)]10+ unit, respectively. The two salicylhydroxamic acid ligands are attached to each [Ti6(μ2-O)3(μ3-O)2]14+ unit. Moreover, the outer surface of each [Ti6(μ2-O)3(μ3-O)2]14+ unit is surrounded by nine isopropyl groups, involving three bridging isopropyl groups and six terminal groups. In the [Ti6(μ2-O)3(μ3-O)2]14+ unit, all the Ti atoms except for the Ti8 atom show octahedral TiO6 coordination environments, while the Ti8 atom is five-coordinated in a hexahedral environment (Fig. 1f). Also, the space-filling structure and packing structure of Ti18Fcdc2 are shown in Fig. 2c and d. The length and width of the rectangles in Ti18Fcdc2 are about 4.96 and 4.29 Å, respectively.
The X-ray diffraction (XRD) patterns of Ti12Fcdc4 and Ti18Fcdc2 are in good agreement with those simulated from the crystallographic data (Fig. S5 and S6†), indicating the phase purity of the samples. Thermogravimetric analysis (TGA) showed that Ti12Fcdc4 and Ti18Fcdc2 have similar thermal behaviour, and the two cluster frameworks remain stable up to approximately 200 °C (Fig. S7 and S8†). The infrared spectroscopy (IR) spectra of Ti12Fcdc4 and Ti18Fcdc2 are shown in Fig. S9 and S10.† The vibrational bands in the range of 600–700 cm−1 can be attributed to Ti–O–Ti vibrations, while the vibrational bands in the range of 1000–1100 cm−1 correspond to Ti–O–C vibrations. The vibration observed at around 1500 cm−1 belongs to the characteristic vibrations of the ferrocene groups. To identify the chemical state of the elements in Ti12Fcdc4 and Ti18Fcdc2, X-ray photoelectron spectroscopy (XPS) analysis was carried out. Fig. S11† shows the Ti 2p and Fe 2p regions of the high resolution XPS spectra. There were two signal peaks located at 458.8 and 464.6 eV, corresponding to Ti 2p3/2 and Ti 2p1/2, respectively, which confirms the existence of a Ti4+ cation. The pair of binding energies existing at 708.1 and 720.7 eV correspond to Fe 2p3/2 and Fe 2p1/2, indicating the presence of an Fe2+ cation.
Light absorption behaviors
To evaluate the light absorption of these compounds, UV-vis absorption spectrum analysis was carried out. As shown in Fig. 3a, Ti12Fcdc4 and Ti18Fcdc2 display broad absorption bands with an absorption edge at about 600 nm, which is similar to previously reported ferrocene-sensitized Ti-oxo clusters.55,57,58 The ultraviolet absorption is mainly ascribed to the O2p → Ti3d charge transfer transitions in titanium-oxo clusters.2,4 The remarkable visible absorption bands of Ti12Fcdc4 and Ti18Fcdc2 can be mainly attributed to the charge transfer transition from the ferrocenyl groups to the Ti-oxo cores. The band gaps of Ti12Fcdc4 and Ti18Fcdc2 were calculated to be about 2.05 and 2.11 eV, respectively, from the absorption band edge (Fig. 3b). Actually, both Ti12Fcdc4 and Ti18Fcdc2 display lower band gaps than pure Ti-oxo clusters. The narrow band gap may be explained by the contribution from charge transfer between the Fcdc ligands and the Ti-oxo cores.
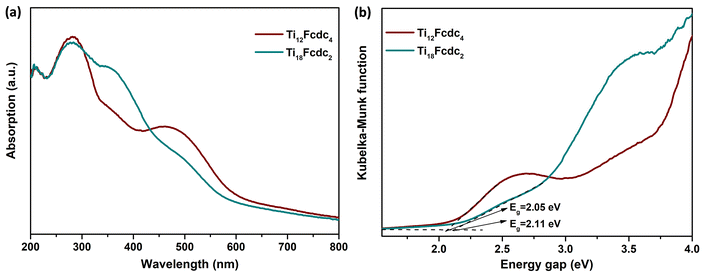 |
| Fig. 3 (a) Solid-state UV/vis absorption spectra and (b) the band gaps of Ti12Fcdc4 and Ti18Fcdc2. | |
Density functional theory (DFT) calculations
Theoretical DFT calculations based on the crystal data were performed to further investigate the charge transfer transition process of Ti12Fcdc4 and Ti18Fcdc2. For Ti12Fcdc4, the electron densities of the highest occupied molecular orbitals (HOMO to HOMO−3) are mainly assigned to the Fe 3d orbitals of the ferrocenyl groups, and the lowest unoccupied molecular orbitals (LUMO to LUMO+3) are predominantly dominated by contributions from the Ti 3d orbitals of the Ti-oxo core (Fig. 4a). The DFT calculation results suggest that the HOMO → LUMO transition mainly involves the charge transfer from the ferrocenyl groups to the Ti-oxo cores. For Ti18Fcdc2, most coefficients of the HOMO, HOMO−2 and HOMO−3 are located on the salicylhydroxamic acid and FcdcH2 ligands, and the LUMO, LUMO+2 and LUMO+3 are mainly located on the Ti-oxo core and isonicotinic acid (Fig. 4b). This result suggests that for Ti18Fcdc2, the HOMO → LUMO electron transition can mainly correspond to the charge transfer from the salicylhydroxamic acid and Fcdc ligands to the Ti-oxo cores.
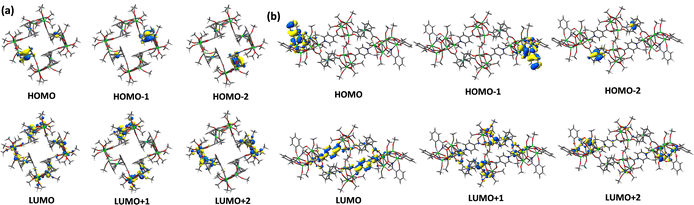 |
| Fig. 4 Some related frontier molecular orbitals of Ti12Fcdc4 (a) and Ti18Fcdc2 (b). | |
Photoelectrochemical (PEC) measurements
To investigate the carrier charge transfer efficiency, we measured transient photocurrent responses of these Ti-oxo clusters containing ferrocene connectors. As shown in Fig. 5a, Ti12Fcdc4 and Ti18Fcdc2 as electrode precursors exhibit clear photocurrent responses upon on–off cycling irradiation. This rapid rise and fall in photocurrent density indicates that the carriers transport in these cluster-based materials proceeds quickly. The incorporation of FcdcH2 ligands into Ti-oxo structures may effectively promote the charge transfer transition between the ferrocenyl groups and Ti-oxo cores and greatly extend the visible light absorption band, resulting in the excellent photoelectric activity of these ferrocene-sensitized titanium-oxo clusters. The photocurrent densities are 0.53 and 0.46 μA cm−2 for Ti12Fcdc4 and Ti18Fcdc2, respectively. The enhanced photoelectric activity of Ti12Fcdc4 can be mainly attributed to the broader light absorption and narrower band gap in comparison with that of Ti18Fcdc2. Moreover, the intrinsic structure and coordination environment of Ti-oxo clusters also directly affect their photoelectric responses. The XRD patterns and IR spectra of the samples after photocurrent measurements were basically identical to those of the original compounds, indicating that these compounds are stable during the photoelectric experiments (Fig. S5 and S6†).
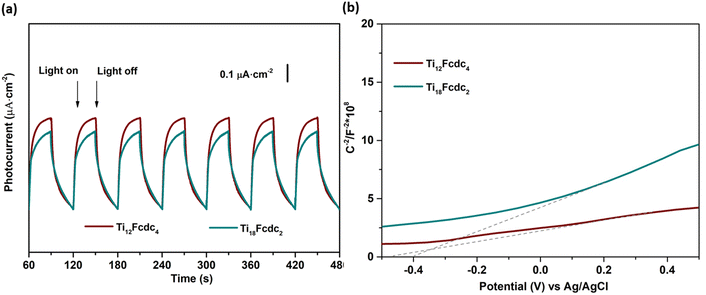 |
| Fig. 5 (a) Photocurrent responses of Ti12Fcdc4 and Ti18Fcdc2. (b) Mott–Schottky plots of Ti12Fcdc4 and Ti18Fcdc2. | |
Fig. 5b displays the Mott–Schottky plot. The slopes of the plots for these photoelectrodes were positive which demonstrated that Ti12Fcdc4 and Ti18Fcdc2 exhibit typical characteristics of n-type semiconductors. A lower slope was observed for Ti12Fcdc4 compared to that of Ti12Fcdc4, suggesting a higher carrier density. The corresponding flat band potential can be obtained from the interception of the plots with the potential axis. They are −0.26 V (vs. NHE) for Ti12Fcdc4 and −0.20 V (vs. NHE) for Ti18Fcdc2. For an n-type semiconductor, the flat band potential is about 0.1 eV positive than the conduction band potential. Based on the band gap, the corresponding valence band potentials are calculated to be about 1.69 and 1.81 V (vs. NHE) for Ti12Fcdc4 and Ti18Fcdc2, respectively. Additionally, the Nyquist plots of electrochemical impedance spectroscopy (EIS) could illustrate the interfacial charge transfer process (Fig. S12†). The radius of the arc is related to the charge transfer resistance occurring on the surface of the electrode, and the smaller radius means the higher charge transfer efficiency. Furthermore, the size of the semicircle for the Ti12Fcdc4 electrode is smaller than that of the Ti18Fcdc2 electrode, suggesting the greatly improved charge transfer efficiency. These findings are also consistent with the results of the photocurrent measurements.
Conclusions
In summary, two Ti-oxo clusters containing ferrocene connectors were successfully synthesized using {Ti6} and/or {Ti3} units as building blocks and FcdcH2 ligands as bridging connectors. The introduction of ferrocene ligands has positive effects on the light absorption behaviors and carrier charge transfer of the resulting clusters. Moreover, these two clusters as electrode precursors showed excellent photocurrent responses under visible light irradiation, suggesting that they may have promising applications in fields such as photocurrent sensors and dye-sensitized solar cells. Further studies on the assembly engineering and photoelectric behaviors of Ti-oxo clusters containing ferrocene ligands as photosensitizers are underway.
Conflicts of interest
There are no conflicts to declare.
Acknowledgements
C. Wang acknowledges the support from the Qilu University of Technology Science Research Initial Fund.
References
- W. H. Fang, L. Zhang and J. Zhang, Synthetic strategies, diverse structures and tuneable properties of polyoxo-titanium clusters, Chem. Soc. Rev., 2018, 47, 404–421 RSC.
- P. Coppens, Y. Chen and E. Trzop, Crystallography and properties of polyoxotitanate nanoclusters, Chem. Rev., 2014, 114, 9645–9661 CrossRef CAS.
- L. Rozes and C. Sanchez, Titanium-oxo-clusters: precursors for a Lego-like construction of nanostructured hybrid materials, Chem. Soc. Rev., 2011, 40, 1006–1030 RSC.
- N. Li, P. D. Matthews, H. K. Luo and D. S. Wright, Novel properties and potential applications of functional ligand-modified polyoxotitanate cages, Chem. Commun., 2016, 52, 11180–11190 RSC.
- P. D. Matthews, T. C. King and D. S. Wright, Structure, photochemistry and applications of metal doped polyoxotitanium alkoxide cages, Chem. Commun., 2014, 50, 12815–12823 RSC.
- Y. J. Liu, W. H. Fang, L. Zhang and J. Zhang, Recent advances in heterometallic polyoxotitanium clusters, Coord. Chem. Rev., 2020, 404, 213099 CrossRef CAS.
- Q. Y. Zhu and J. Dai, Titanium oxo/alkoxyl clusters anchored with photoactive ligands, Coord. Chem. Rev., 2021, 430, 213664 CrossRef CAS.
- U. Schubert, Titanium-Oxo Clusters with Bi- and Tridentate Organic Ligands: Gradual Evolution of the Structures from Small to Big, Chem. – Eur. J., 2021, 27, 11239–11256 CrossRef CAS PubMed.
- C. Y. Liu and Y. F. Wang, Supramolecular Chemistry of Titanium Oxide Clusters, Chem. – Eur. J., 2021, 27, 4270–4282 CrossRef CAS PubMed.
- M. Y. Fu, H. Y. Wang, H. L. Zhai, Q. Y. Zhu and J. Dai, Assembly of a Titanium-Oxo Cluster and a Bismuth Iodide Cluster, a Single-Source Precursor of a p–n-Type Photocatalyst, Inorg. Chem., 2021, 60, 9589–9597 CrossRef CAS PubMed.
- C. Zhao, Y. Z. Han, S. Dai, X. Chen, J. Yan, W. Zhang, H. Su, S. Lin, Z. Tang, B. K. Teo and N. F. Zheng, Microporous cyclic titanium-oxo clusters with labile surface ligands, Angew. Chem., Int. Ed., 2017, 56, 16252–16256 CrossRef CAS PubMed.
- Y. R. Zhao, H. Zheng, L. Q. Chen, H. J. Chen, X. J. Kong, L. S. Long and L. S. Zheng, The effect on the luminescent properties in lanthanide-titanium-oxo clusters, Inorg. Chem., 2019, 58, 10078–10083 CrossRef CAS PubMed.
- N. Li, D. Pranantyo, E. T. Kang, D. S. Wright and H. K. Luo, A simple drop-and-dry approach to grass-like multifunctional nano-coating on flexible cotton fabrics using in situ generated coating solution comprising titanium-oxo custers and silver nanoparticles, ACS Appl. Mater. Interfaces, 2020, 12, 12093–12100 CrossRef CAS PubMed.
- N. Li, D. Pranantyo, E. T. Kang, D. S. Wright and H. K. Luo, In situ self-assembled polyoxotitanate cages on flexible cellulosic substrates: multifunctional coating for hydrophobic, antibacterial, and UV-blocking applications, Adv. Funct. Mater., 2018, 28, 1800345 CrossRef.
- P. Yang, Z. W. Zhang, G. D. Zou, Y. Huang, N. Li and Y. Fan, Template Thermolysis to Create a Carbon Dots-Embedded Mesoporous Titanium-Oxo Sulfate Framework for Visible-Light Photocatalytic Applications, Inorg. Chem., 2020, 59, 2062–2069 CrossRef CAS PubMed.
- X. Fan, J. H. Wang, K. F. Wu, L. Zhang and J. Zhang, Isomerism in titanium-oxo clusters: molecular anatase model with atomic structure and improved photocatalytic activity, Angew. Chem., Int. Ed., 2019, 58, 1320–1323 CrossRef CAS.
- B. C. Zhu, Q. L. Hong, X. F. Yi, J. Zhang and L. Zhang, Supra-molecular co-assembly of the Ti8L12 cube with [Ti(DMF)6] species and Ti12-oxo cluster, Inorg. Chem., 2020, 59, 8291–8297 CrossRef CAS.
- W. H. Fang, L. Zhang and J. Zhang, A 3.6 nm Ti52-oxo nanocluster with precise atomic structure, J. Am. Chem. Soc., 2016, 138, 7480–7483 CrossRef CAS.
- M. Y. Gao, F. Wang, Z. G. Gu, D. X. Zhang, L. Zhang and J. Zhang, Fullerene like polyoxotitanium cage with high solution stability, J. Am. Chem. Soc., 2016, 138, 2556–2559 CrossRef CAS PubMed.
- M. Czakler, C. Artner and U. Schubert, Two new hexanuclear titanium-oxo cluster types and their structural connection to known clusters, New J. Chem., 2018, 42, 12098–12103 RSC.
- G. Zhang, C. Liu, D. L. Long, L. Cronin, C. H. Tung and Y. F. Wang, Water-soluble pentagonal-prismatic titanium-oxo clusters, J. Am. Chem. Soc., 2016, 138, 11097–11100 CrossRef CAS PubMed.
- Y. Z. Yu, Y. Guo, Y. R. Zhang, M. M. Liu, Y. R. Feng, C. H. Geng and X. M. Zhang, A series of silver doped butterfly-like Ti8Ag2 clusters with two Ag ions panelled on a Ti8 surface, Dalton Trans., 2019, 48, 13423–13429 RSC.
- Y. P. He, G. H. Chen, D. J. Li, Q. H. Li, L. Zhang and J. Zhang, Combining Titanium-Organic Cage and Hydrogen-Bonded Organic Cage for Highly Effective Third-Order Nonlinear Optics, Angew. Chem., 2021, 133, 2956–2959 CrossRef.
- X. Fan, F. R. Yuan, D. J. Li, S. Chen, Z. B. Cheng, Z. J. Zhang, S. J. Xiang, S. Q. Zang, J. Zhang and L. Zhang, Threefold Collaborative Stabilization of Ag14-Nanorods by Hydrophobic Ti16-Oxo Clusters and Alkynes: Designable Assembly and Solid-State Optical-Limiting Application, Angew. Chem., Int. Ed., 2021, 60, 12949–12954 CrossRef CAS PubMed.
- Y. Y. Sun, D. F. Lu, Y. Z. Sun, Q. Wei, M. Y. Gao, N. Zheng, C. Gu, F. Wang and J. Zhang, Large Titanium-oxo Clusters as Precursors to Synthesize the Single Crystals of Ti-MOFs, ACS Mater. Lett., 2021, 3, 64–68 CrossRef CAS.
- Y. P. He, L. B. Yuan, G. H. Chen, Q. P. Lin, Q. P. Wang, L. Zhang and J. Zhang, Water Soluble and Ultra-Stable Ti4L6 Tetrahedron with Coordination Assembly Function, J. Am. Chem. Soc., 2017, 139, 16845–16851 CrossRef CAS.
- M. Y. Fu, H. Y. Wang, H. L. Zhai, Q. Y. Zhu and J. Dai, A Convenient Procedure for Preparing BiOX–TiO2 Photoelectrocatalytic Electrodes from a Titanium-Oxo Compound-Modified Carbon Fiber Cloth, Inorg. Chem., 2022, 61, 4024–4032 CrossRef CAS PubMed.
- C. Y. Ge, J. L. Ho, Z. Y. Zhou, Q. Y. Zhu and J. Dai, A Cyclic Titanium-Oxo Cluster with a Tetrathiafulvalene Connector as a Precursor for Highly Efficient Adsorbent of Cationic Dyes, Inorg. Chem., 2022, 61, 486–495 CrossRef CAS PubMed.
- X. Fan, L. B. Yuan, J. Zhang and L. Zhang, Phenol-triggered supramolecular transformation of titanium–oxo cluster based coordination capsules, Chin. Chem. Lett., 2021, 32, 2415–2418 CrossRef CAS.
- Y. P. He, G. H. Chen, D. J. Li, Q. H. Li, L. Zhang and J. Zhang, Combining Titanium-Organic Cage and Hydrogen-Bonded Organic Cage for Highly Effective Third-Order Nonlinear Optics, Angew. Chem., Int. Ed., 2021, 60, 2920–2923 CrossRef CAS PubMed.
- M. Y. Gao, X. Fan, L. Zhang and J. Zhang, Dicarboxylate Ligands Oriented Assembly of {Ti3(μ3-O)} Units: From Dimer to Coordination Triangles and Rectangles, Inorg. Chem., 2018, 57, 5642–5647 CrossRef CAS PubMed.
- Q. R. Ding, G. L. Xu, L. Zhang and J. Zhang, Ligand-directed assembly engineering of trapezoidal {Ti5} building blocks stabilized by dimethylglyoxime, Dalton Trans., 2019, 48, 9916–9919 RSC.
- X. X. Liu, G. H. Chen, J. Tao, J. Zhang and L. Zhang, Synthesis, Structure, and Light Absorption Behaviors of Prismatic Titanium-Oxo Clusters Containing Lacunary Lindqvist-like Species, Inorg. Chem., 2022, 61, 1385–1390 CrossRef CAS PubMed.
- M. Y. Gao, L. Zhang and J. Zhang, Acid-Controlled Synthesis of Carboxylate-Stabilized Ti44-Oxo Clusters: Scaling up Preparation, Exchangeable Protecting Ligands, and Photophysical Properties, Chem. – Eur. J., 2019, 25, 10450–10455 CrossRef CAS PubMed.
- H. Fu, S. Y. Zhou, X. Fan, L. Zhang and J. Zhang, Assembly and packing models of [Ti6Co12] ring based on the titanium-capped cobaltclathrochelates, Chin. Chem. Lett., 2021, 32, 923–925 CrossRef CAS.
- G. H. Chen, Y. P. He, F. P. Liang, L. Zhang and J. Zhang, A green separation process of Ag via a Ti4(embonate)6 cage, Dalton Trans., 2021, 49, 17194–17199 RSC.
- R. Y. Chen, G. H. Chen, Y. P. He, H. Xu and J. Zhang, Synthesis and Third-Order Nonlinear Optical Properties of Metal-Organic Zeolites Built from Ti4(embonate)6 Tetrahedra, Cryst. Growth Des., 2022, 22, 66–73 CrossRef CAS.
- C. Y. Liu, C. Gao, A. Said, H. H. Niu, D. X. Wang, C. H. Tung and Y. F. Wang, Assembly of Interlocked Superstructures with a Titanium Oxide Molecular Ring in Water, Inorg. Chem., 2021, 60, 14520–14524 CrossRef CAS.
- N. Li, J. Liu, J. J. Liu, L. Z. Dong, S. L. Li, B. X. Dong, Y. H. Kan and Y. Q. Lan, Self-assembly of a phosphate-centered polyoxo-titanium cluster: discovery of the heteroatom keggin family, Angew. Chem., Int. Ed., 2019, 58, 17260–17264 CrossRef CAS.
- H. Zheng, M. H. Du, S. C. Lin, Z. C. Tang, X. J. Kong, L. S. Long and L. S. Zheng, Assembly of a wheel like Eu24Ti8 cluster under the guidance of high resolution electrospray ionization mass Spectrometry, Angew. Chem., Int. Ed., 2018, 57, 10976–10979 CrossRef CAS PubMed.
- C. Y. Liu, J. Y. Hu, W. M. Liu, F. Zhu, G. Wang, C. H. Tung and Y. F. Wang, Binding Modes of Salicylic Acids to Titanium Oxide Molecular Surfaces, Chem. – Eur. J., 2020, 26, 2666–2674 CrossRef CAS PubMed.
- C. Y. Liu, J. Y. Hu, F. Zhu, J. H. Zhan, L. Du, C. H. Tung and Y. F. Wang, Functionalization of Titanium Oxide Cluster Ti17O24(OiC3H7)20 with Catechols: Structures and Ligand-Exchange Reactivities, Chem. – Eur. J., 2019, 25, 14843–14849 CrossRef CAS.
- D. H. Zou, L. N. Cui, P. Y. Liu, S. Yang, Q. Y. Zhu and J. Dai, Molecular model of dye sensitized titanium oxides based on aryl-amine dye anchored titanium-oxo clusters, Inorg. Chem., 2019, 58, 9246–9252 CrossRef CAS PubMed.
- J. L. Hou, Y. G. Weng, P. Y. Liu, L. N. Cui, Q. Y. Zhu and J. Dai, Effects of the ligand structures on the photoelectric activities, a model study based on titanium-oxo clusters anchored with S-heterocyclic ligands, Inorg. Chem., 2019, 58, 2736–2743 CrossRef CAS PubMed.
- L. N. Cui, P. Y. Liu, L. Yang, X. P. Shu, Q. Y. Zhu and J. Dai, A series of Ti6-oxo clusters anchored with arylamine dyes: effect of dye structures on photocurrent responses, Chem. – Asian J., 2019, 14, 3198–3204 CrossRef CAS PubMed.
- Q. R. Ding, Y. H. Yu, C. S. Cao, J. Zhang and L. Zhang, Stepwise assembly and reversible structural transformation of ligated titanium coated bismuthoxo cores: shell morphology engineering for enhanced chemical fixation of CO2, Chem. Sci., 2022, 13, 3395–3401 RSC.
- Y. H. Guo, Y. Z. Yu, Y. H. Shen, L. G. Yang, N. N. Liu, Z. Y. Zhou and Y. S. Niu, “Three-in-One” Structural-Building-Mode-Based Ti16-Type Titanium Oxo Cluster Entirely Protected by the Ligands Benzoate and Salicylhydroxamate, Inorg. Chem., 2022, 61, 8685–8693 CrossRef CAS PubMed.
- Q. R. Ding, Y. H. Yu, C. S. Cao, J. Zhang and L. Zhang, Stepwise assembly and reversible structural transformation of ligated titanium coated bismuthoxo cores: shell morphology engineering for enhanced chemical fixation of CO2, Chem. Sci., 2022, 13, 3395–3401 RSC.
- H. Y. Wang, M. Y. Fu, H. L. Zhai, Q. Y. Zhu and J. Dai, Mono- and Bismetalphenanthroline-Substituted Ti12 Clusters: Structural Variance and the Effect on Electronic State and Photocurrent Property, Inorg. Chem., 2021, 60, 12255–12262 CrossRef CAS PubMed.
- R. Gao, S. M. Chen, F. Wang and J. Zhang, Single-Crystal Syntheses and Properties of Indium-Organic Frameworks Based on 1,1′-Ferrocenedicarboxylic Acid, Inorg. Chem., 2021, 60, 239–245 CrossRef CAS PubMed.
- J. Benecke, E. S. Grape, A. Fuß, S. Wöhlbrandt, T. A. Engesser, A. K. Inge, N. Stock and H. Reinsch, Polymorphous Indium Metal-Organic Frameworks Based on a Ferrocene Linker: Redox Activity, Porosity, and Structural Diversity, Inorg. Chem., 2020, 59, 9969–9978 CrossRef CAS PubMed.
- Q. X. Wang, Z. C. Guo, Y. Qin, X. Wang and G. Li, High Proton Conduction in Three Highly Water-Stable Hydrogen-Bonded Ferrocene-Based Phenyl Carboxylate Frameworks, Inorg. Chem., 2021, 60, 19278–19286 CrossRef CAS PubMed.
- Z. K. Huang, H. J. Yu, L. Wang, X. W. Liu, T. F. Lin, F. Haq, S. Z. Vatsadze and D. A. Lemenovskiy, Ferrocene-contained metal organic frameworks: From synthesis to applications, Coord. Chem. Rev., 2021, 430, 213737 CrossRef CAS.
- J. L. Hou, W. Luo, Y. Guo, P. Zhang, S. Yang, Q. Y. Zhu and J. Dai, Titanium Oxo Cluster with Six Peripheral Ferrocene Units and Its Photocurrent Response Properties for Saccharides, Inorg. Chem., 2017, 56, 6451–6458 CrossRef CAS PubMed.
- Y. Fan, H. M. Li, R. H. Duan, H. T. Lu, J. T. Cao, G. D. Zou and Q. S. Jing, Phosphonate-Stabilized Titanium-Oxo Clusters with Ferrocene Photosensitizer: Structures, Photophysical and Photoelectrochemical Properties, and DFT/TDDFT Calculations, Inorg. Chem., 2017, 56, 12775–12782 CrossRef CAS PubMed.
- Z. C. Liu, J. Y. Lei, M. Frasconi, X. H. Li, D. Cao, Z. X. Zhu, S. T. Schneebeli, G. C. Schatz and J. F. Stoddart, A Square-Planar Tetracoordinate Oxygen-Containing Ti4O17 Cluster Stabilized by Two 1,1′-Ferrocenedicarboxylato Ligands, Angew. Chem., Int. Ed., 2014, 53, 9193–9197 CrossRef CAS.
- J. J. Liu, N. Li, J. W. Sun, J. Liu, L. Z. Dong, S. J. Yao, L. Zhang, Z. F. Xin, J. W. Shi, J. X. Wang, S. L. Li and Y. Q. Lan, Ferrocene-Functionalized Polyoxo-Titanium Cluster for CO2 Photo-reduction, ACS Catal., 2021, 11, 4510–4519 CrossRef CAS.
- E. M. Han, W. D. Yu, L. J. Li, X. Y. Yi, J. Yan and C. Liu, Accurate assembly of ferrocene-functionalized {Ti22Fc4} clusters with photocatalytic amine oxidation activity, Chem. Commun., 2021, 57, 2792–2795 RSC.
|
This journal is © the Partner Organisations 2022 |
Click here to see how this site uses Cookies. View our privacy policy here.