The potential contribution of urine source separation to the SDG agenda – a review of the progress so far and future development options†
Received
3rd December 2020
, Accepted 22nd April 2021
First published on 6th May 2021
Abstract
Sanitation and wastewater management are highly relevant for reaching a number of interconnected sustainable development goals (SDGs), especially SDG 6, the provision of safe drinking water and adequate sanitation for all as well as protection of water resources against pollution, and SDG 14.1, reducing nutrient emissions to the marine environment. Recent evidence increasingly shows that conventional sewer-based wastewater management will not be able to reach these targets. Rather than further optimizing and diffusing this ageing infrastructure paradigm, radical innovations like urine source separation technologies could help to leapfrog towards faster achievement of the SDGs. The technology would simplify on-site sanitation and develop a closed-loop nutrient cycle, thereby allowing for exceptionally high nutrient removal from wastewater and direct reuse in agriculture from the first day of implementation. Radical innovations, however, need decades to materialize. Based on a review of relevant academic and grey literature, we show how the past three decades of development of urine source separation have brought breakthroughs in toilet design and treatment processes, enabling the technology's value chain to reach the brink of maturity. In a short outlook, we discuss how the technology may reach global diffusion over the next decade, with the main remaining challenges relating to the creation of mass-markets for urine-diverting toilets, automation and mass-production of treatment systems, and the legitimation of fertilizer produced from urine in the agricultural sector.
Water impact
Transforming sanitation has the potential to contribute to achieving several water-related SDGs. We review how the radical innovation of urine separation can help achieve key goals, and how local and global actors supported implementation in different cultural contexts. By embedding the case of urine separation in a suitable innovation framework, we stimulate the transfer to other SDG-related radical innovations.
|
1. Introduction
Reaching the sustainable development goals (SDGs) is presently one of the most challenging goals of humanity. Innovation in the water and sanitation sector has a major role to play, but in order to realize this potential, the solutions offered by the scientific community have to integrate environmental, social and economic perspectives.1 For achieving sustainable urban wastewater management on a global scale, Hoffmann et al.2 suggest that non-grid alternatives and source separation of wastewater fractions will play a major role. In this frontier review, we will focus on urine source separation, which is one specific eco-innovation in this broad field. We will show how the interplay between researchers of different disciplines and practice partners have driven this radical innovation from a “hopeful monstrosity”3 in the 1990s towards a plausible alternative for actual implementation in the 2020s.
The review has three main parts. In section 2, we will review the role of urban water management for the SDGs. In section 3, we will sketch out why urine source separation – if applied at scale – could make a real change to the environment and help reach some of the SDGs on water and sustainable cities. In section 4, we will review the past evolution of this radical innovation, based on academic and grey literature. In the forward-looking section 5, we discuss key bottlenecks for further diffusion and provide an outlook on where this approach might scale up and thereby start to unfold its full contribution to an imminent sustainability transition in the water and sanitation field.
2. Urban water management and the SDGs
The SDGs for 2030 were adopted in 2015 in order to approach the grand challenges of the 21st century, including a number of goals to end the inequality between countries with respect to urban water management.4 SDG 6 requires safe drinking water and adequate sanitation for all as well as protection of water resources against pollution. This goal includes halving the proportion of untreated wastewater and substantially increasing recycling and safe reuse globally. SDG 14.1 demands reduction of marine pollution, explicitly mentioning nutrient pollution. SDG 2 demands zero hunger, and more recycling of water and nutrients from wastewater may have a part to play in fulfilling this goal. Many other SDGs depend on improvements in the water and sanitation sector, especially SDG 3 to ensure healthy lives, SDG 5 on gender equality, and SDG 11 on sustainable cities. During the last 40 years, the international community has set up a number of plans to improve world-wide sanitation, but none of them reached their own targets.5 The present SDG 6 has the most ambitious goals ever, presenting an enormous challenge: By 2030, 1.3 billion people should abandon open defecation and 5.6 billion people should reach adequate sanitation including safely managed excreta, securing hygiene as well as treatment either on-site or off-site.5
We will not speculate on the possible achievement of the specific SDGs until 2030, but rather review the general development of urban wastewater management in the light of the SDGs. Some of the papers cited in this review base their prognoses on scenarios, building on the shared socioeconomic pathways (SSPs). Originally introduced for the development of climate scenarios, SSP1–SSP5 describe five different plausible directions of global socio-economic development, with different degrees of challenges for mitigation of and adaptation to climate change.6,7 SSP1 is the most optimistic scenario with low population growth, low energy intensity, and low carbon intensity, whereas SSP3 generally describes the opposite situation and the three other SSPs provide some intermediate pathways.8 In a rare analysis of the relevance of these pathways for the SDGs, Liu et al.9 conclude that a development corresponding to SSP1 will also lead to improvements on all SDGs, independently of the climate actions taken.
Urban water management at the global scale
In 2007, readers of the British Medical Journal voted the “sanitary revolution to be the greatest medical advance since 1840”, in front of antibiotics and anesthesia.10 Indeed, the provision of piped water to households, the introduction of the flush toilet, and the construction of sewers to carry away the combined wastewater led to the separation of drinking water and sewage, an issue which is thought to be important for increasing life expectancy in cities of the late 19th century.11 In England, for instance, life expectancy increased from an average of 41 years in 1870 to 50 years around 1910, and although there were many reasons for this, public hygiene most probably played a large role.12 The flush toilet is the visible symbol of the sanitary revolution, but also led to a loss of fertilizer and severe pollution of surface waters.13 It is common knowledge that the 20th century brought fossil and synthetic fertilizers to replace the missing nutrients from the human metabolism in agriculture and that wastewater treatment plants (WWTPs) can reduce the pollution of surface waters that naturally follows water-borne sanitation. Historically, cities introduced wastewater treatment stepwise,13 starting with primary treatment, i.e. sedimentation to remove particulate matter, followed by secondary treatment consisting of biological treatment to remove most of the remaining organic matter and oxidize ammonia to nitrate, and finally introducing tertiary treatment to remove nitrogen and phosphorus, the first one biologically and the latter either chemically or biologically.
The centralized, grid-based wastewater management system has become the aspirational model for societies worldwide, although it still serves a minority of the global population. Merely around a third of the global population has access to sewers, and in Sub-Saharan Africa, the connection rate is only about 5%.14 Non-grid wastewater management exists, but grid-based centralized system are generally considered the natural endpoint of infrastructure development at least for cities.15 The historic concept of stepwise improvement is still applied: of those with access to sewers, i.e. one third of the world population, 21% have no wastewater treatment at all, 35% primary, 23% secondary, and only 21% tertiary treatment.16 Modern WWTPs with tertiary treatment, i.e. nutrient removal, thus serve less than 10% of the total world population, primarily in North America, Western Europe, Japan and Oceania.16
Environmental concerns of conventional urban wastewater management
Historically, centralized urban wastewater management always resulted in an initial conflict between human welfare – piped water and sanitation based on the flush toilet – and the aquatic environment that received the resulting wastewater.13 Solving this conflict was possible, but with time lags between the single steps of wastewater management, from the initial introduction of sewers only, followed eventually by simple treatment technologies before finally reaching tertiary treatment,13 and always based on tax money for water-related infrastructure.17 In the slowly growing cities of Europe, this seemed acceptable at the time, although in reality, eutrophication may have led to irreversible loss of biodiversity in some aquatic ecosystems.18 Today, the situation is very different. With unprecedented population growth and urbanization on a global scale, environmental concerns have become global as well. About a decade ago, Rockstrom et al.19 drew attention to the global negative effects of nutrient emissions, arguing that the biogeochemical flows of nitrogen and phosphorus may already have transgressed the planetary boundaries for a safe operating space for humanity, primarily due to eutrophication of coastal areas and the atmospheric nitrogen pollution. For nitrogen, the major threat to coastal areas, the authors set up tentative boundaries of 35 Tg per year for industrial and intentional biological fixation of nitrogen from dinitrogen gas, as compared to today's 130 Tg per year. For simplicity, we will omit a discussion on phosphorus, where the main effects are on local freshwater bodies.20
With the inherent inefficiency of nitrogen uptake in agriculture and the inevitable losses in the food chain,21 there is an obvious conflict between this tight boundary for nitrogen fixation and SDG 2, demanding zero hunger. Just to feed the present global population of close to 8 billion people, we need around 30 Tg per year of nitrogen in the form of protein, with about the same amount being excreted from the human metabolism.22 Based on a detailed study by De Vries et al.23 that took into account the environmental concerns as well as the requirement for global food security, Steffen et al.24 adopted a new boundary for intentional nitrogen fixation of 62 Tg per year. Building on three of the shared socioeconomic pathways (SSP1–SSP3), Bodirsky et al.25 set up global scenarios to evaluate realistic mitigation actions to reach the new, more realistic nitrogen boundary. Based on the “middle of the road” pathway SSP2, the authors concluded that even with ambitious global mitigation actions for all main sectors (including agriculture, responsible for the largest emissions, and waste management), it is unclear whether these actions can reduce the water and atmospheric N pollution below the critical thresholds formulated for 2050. An important mitigation measure suggested by these authors is to recycle 40–60% of the nitrogen from the human metabolism to agriculture by 2050, as compared to an assumed 0% recycling today. Equally, the United Nations Environment Program (UNEP) recently adopted a declaration on sustainable nitrogen management, with “an ambition to halve nitrogen waste by 2030” and an urge to “promote innovation on anthropogenic nitrogen use and recycling”.26 In this paper, we review one possibility of promoting radical innovation on anthropogenic recycling of wastewater nitrogen. In agriculture, equally radical measures have been suggested, e.g. by Pikaar et al.,27 who advocate reactor-based protein production as an alternative to conventional agriculture.
The limits of conventional urban water management in reaching the SDGs
On a global scale, it is an open question whether conventional urban water management can at the same time solve the SDGs' welfare challenge of urban hygiene, i.e. safely managed excreta as required by SDG6, and the environmental challenge of reducing water pollution, especially nutrient pollution as required by SDG14.1.
Based on the SSPs, Van Puijenbroek et al.16 set up prognoses for the global development of conventional urban wastewater infrastructure and nutrient emissions to the aquatic environment. Depending on the actual pathway, the authors predict that nitrogen emissions from wastewater will increase by 29–71% from 2010 to 2050, and not decrease as suggested by Bodirsky et al.25 The best results are obtained for SSP5, a pathway with a focus on high economic growth, new technology, high energy-demand and a continued dependence on fossil fuels.6,16 The technical assumptions for this urban wastewater management scenario are optimistic, especially for nitrogen elimination. Nearly a third of all treatment plants would achieve quaternary treatment, defined as 95% nitrogen elimination, as compared to the 50–75% nitrogen elimination obtained in tertiary treatment.28 At present, quaternary treatment is hardly implemented because it relies on an external carbon source like methanol leading to high costs and high energy demand.29 In absolute numbers, this would correspond to seven times more people with advanced nutrient elimination, i.e. tertiary or quaternary treatment, than the number with tertiary treatment today. For the overall more desirable SSP1, the authors predict a 50% increase in nitrogen emissions, partially due to the lower percentage of quaternary treatment. Even with highly optimistic technical scenarios under the assumption that the world will continue to rely heavily on fossil fuels until 2050, Van Puijenbroek et al.16 were not able to model any net contribution of conventional wastewater management to reaching SDG 14.1 with respect to nitrogen.
As discussed above, achieving SDG6 is most challenging in low and middle-income countries, where we also see the most rapid population growth. Due to the long planning and construction periods of large centralized infrastructures, such rapid population growth is not favorable for conventional grid-based wastewater management.30 Based on an analysis of the 60 fastest growing cities in the world, Öberg et al.31 calculated that the implementation rate of sewers and basic WWTPs should proceed at a rate 10–50 times faster than the typical rate for World Bank projects in order to keep up with population growth. The same authors found that Lagos, Nigeria, would have to invest 14–37% of the state budget every year in order to provide the growing population with such an infrastructure. For the costs of wastewater treatment, anaerobic lagoons served as model.31,32 Öberg et al.31 concluded that due to high costs and long planning periods, conventional grid-based wastewater management is presently not able to secure adequate sanitation including safe disposal of excreta as required by SDG 6.
3. Urine source-separation as an enabling technology to reach the water-related SDGs
In the previous section, we have identified two major SDG-related challenges for conventional grid-based wastewater management. First, even with highly optimistic assumptions, there is a high probability that further development of sewers and wastewater treatment will not result in a reduction of aquatic nitrogen pollution as required by SDG14.1.16 Second, in the large, rapidly growing cities of low and middle-income countries, it is not plausible that conventional grid-based wastewater management will be able to reach the goal of adequate sanitation, including safe disposal of excreta as required by SDG6.31
A number of authors have argued that urine source separation can generally act as an enabling technology to improve the performance of existing sanitation options. This role is based on the high nutrient content of urine: 80% of the nitrogen and 50% of the phosphorus ending up in domestic wastewater stem from this single source.33 Separating most of the urine in the toilet thus strongly reduces the nutrient pollution potential of wastewater and at the same time gives rise to new recycling options, supporting the circular economy.34 In this section, we will review the case for urine source separation as an enabling technology to reach the SDGs.
According to Van Puijenbroek et al.,16 the number of people with access to sewers will be a factor of 1.9–2.7 higher in 2050 than in 2010, resulting in an increase in nitrogen emissions because it is not plausible that the construction of tertiary and quaternary wastewater treatment plants will keep up with this development. Wilsenach and van Loosdrecht35 showed that combining very simple wastewater treatment with urine source separation would in fact reach the same effluent nitrogen concentration as quaternary treatment. Where suitable, it would be highly beneficial to introduce urine source separation as a complement to simple wastewater treatment.
For the many areas where conventional grid-based wastewater management is not possible, on-site technologies are an alternative, e.g., in India, where around 60% of the urban population rely on onsite sanitation, e.g. septic tanks.36 However, in urban areas with a high population density, these conventional on-site technologies do not produce an effluent with sufficiently high quality to protect the receiving waters, oftentimes groundwater.22,31,36 For the urban on-site systems in India, Dasgupta et al.36 suggest replacing the prevailing septic tanks with small-scale packet plants. Nitrogen removal in addition to removal of organic matter is certainly possible in such plants. Mažeikienė and Grubliauskas37 showed that in a lab setting, a packet plant can remove about 60% of the total nitrogen, albeit at the cost of larger and more complex plants. In an LCA, Kulak et al.38 compared four different technologies for the Indian situation: centralized wastewater treatment, twin-pit latrines, and latrines with urine source separation, with and without biogas production from feces. The combination of urine source separation and biogas was the most favorable combination, with high recycling of nitrogen from urine to combat the fertilizer deficit in India and a positive effect on greenhouse gas emissions. The latter is due to the pour flush latrines typical for an Indian context, leading to anaerobic pit latrines with high production of the potent greenhouse gas methane. Likewise, Yiougo et al.39 did a mass flux analysis for different sanitation solutions for Pouytenga, a city in Burkina Faso, with 72
000 inhabitants. At present, more than 300 tons of nitrogen annually pollutes the drinking water reservoir and the groundwater. With the introduction of urine source separation combined with composting of solid waste, the city could redirect around 200 tons of nitrogen to beneficial use in agriculture.
For urban slums, presently inhabited by more than a billion people, nearly one quarter of the world's urban population,40 the social enterprise Sanergy has developed container-based sanitation (CBS) as a sustainable alternative, with the double purpose of providing safe sanitation and valuable nutrients for local farmers.41 The CBS toilets collect excreta on-site, followed by frequent transport to a semi-centralized facility for fertilizer production. Recently, Sanergy42,43 proposed to upgrade the system with urine source separation, in order to facilitate treatment and recycling of organic matter (from feces) and nutrients (from urine). Schmitt et al.44 set up a method for organizing collection and transport of the separate fractions, a crucial service provision to ensure customer's satisfaction with the sanitation solution.41
From the examples in the previous paragraphs, we observe that resource recovery is a strong driver for the introduction of urine source separation. This also makes sense from an environmental point of view. Whereas a number of life cycle assessments have consistently shown that urine source separation is environmentally beneficial,45–49 this is strongly dependent on the ability to off-set synthetic fertilizer45 and the ability to produce a fertilizer product with low nitrogen emissions during spreading.50
We thus observe three positive aspects of urine source separation with respect to the SDGs. Reducing nitrogen pollution from on-site and centralized wastewater treatment plants strongly supports SDG 14.1, nutrient recycling in areas with a lack of fertilizers supports SDG 2, and improving on-site sanitation in urban slums helps achieve SDG 6 and 11.
The socio-technical challenges of urine source separation
Although the concept of urine source separation is simple and the potential benefits obvious, implementation is complex. Instead of simply connecting a conventional toilet to the general building wastewater pipe infrastructure, it takes a new type of toilet (at the “front-end”), separate piping and storage for urine (at the “middle-end”), and a new type of treatment technology (at the “back-end”). In order to obtain nutrient recycling, farmers and consumers must accept a new type of fertilizer.
At the front-end, urine-diverting dehydration toilets, UDDTs, and urine-diverting flush toilets exist (see Fig. 1 for a typical example of each type of toilet).
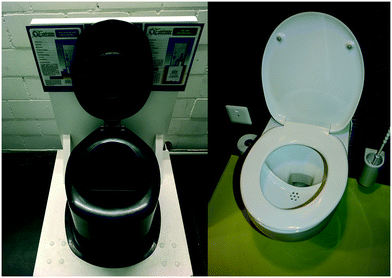 |
| Fig. 1 The Envirosan urine-diverting dehydration toilet (UDDT; left) and the Roediger urine-diverting flush toilet (right). Copyright Eawag. | |
Urine-diverting flush toilets are employed in areas with sewers, primarily in Europe,51 whereas UDDTs have gained some popularity as an alternative to conventional dry toilets, especially because they give rise to less odor.52 In Durban, South Africa, a new law obliged the municipality to provide sanitation to all from 2001, resulting in the installation of double vault UDDTs to serve the areas without sewers.53 With urine infiltrating the ground and alternate use of the feces-containing vaults, it was possible to evacuate the vaults in a relatively safe way to gain organic matter for soil improvement. However, whereas Europeans are largely in favor of urine-separating flush toilets, albeit quite critical with respect to the toilet technology available,51 the UDDTs are not at all popular in Durban.54 With the flush toilet being the standard solution in the affluent parts of Durban, the affected population considers dry sanitation an unattractive and unjust solution for the poor.55
At the middle-end, blockage of pipes due to precipitation of phosphate compounds is the main plumbing problem.56 In some full-scale installations, this problem was solved by regular addition of 10% citric acid during toilet cleaning, combined with large-diameter pipes installed with a slope instead of the typical horizontal pipes.57 Boyer and Saetta58 reviewed a number of methodologies for inhibiting urea hydrolysis in the first place or, alternatively, inducing precipitation close to the toilet in order to protect the pipes. One of those technologies, real-time controlled continuous addition of acetic acid to avoid urea hydrolysis, has been successfully demonstrated at a university campus equipped with waterless urinals.59
At the back-end, Maurer et al.60 defined seven process-engineering objectives for reaching the double goal of water pollution control and safe nutrient recycling. Besides the production of a safe fertilizer, i.e. without organic micropollutants and pathogens, volume reduction for facilitating transport of nutrients to agriculture or further processing is of high practical importance for the development of the field.61 A detailed review of the large number of technologies presently under development for urine treatment is found elsewhere.61 At this place, we will only present two technologies for volume reduction at two different scales, which have reached the stage of a pilot plant. For the apartment building scale or above, Fumasoli et al.62 described a biological nitrification pilot plant serving the stabilization of volatile free ammonia as ammonium nitrate followed by distillation. Introducing a treatment with activated carbon between biological treatment and distillation resulted in a safe fertilizer product with a sufficiently low content of organic micropollutants to obtain an unlimited fertilizer allowance from the Swiss authorities, without co-removal of beneficial nutrients.63 At the bathroom scale, Simha et al.64 tested alkaline dehydration at pilot-scale for the production of a volume-reduced dry, transportable fertilizer. With this technology, fresh urine is dehydrated by a forced air stream within an alkaline medium. The high alkalinity prevents enzymatic hydrolysis of urea, which would lead to loss of free ammonia. While a bathroom-scale treatment unit provides the unique advantage of implementing urine treatment directly under the toilet, omitting the complexity of additional plumbing at the mid-end, until now we have seen no proposal of a mechanism for removal of organic micropollutants in such a system.
Nutrient recycling, the last step of the complex chain of urine source separation, depends on farmer's and consumer acceptance of a urine-based fertilizer. In a large international survey, Simha et al.65 showed that on average, 60% of the population had a favorable attitude towards food fertilized by urine. The authors draw up a number of aspects of importance for acceptance, but generally, they conclude that consumer acceptance will not be the key limitation for nutrient recycling from urine. Similar results exist for farmer's potential uptake of urine-based fertilizer in Switzerland.66
4. The past innovation journey of urine source separation technologies
Given the potential transformative effects of urine source separation on the sanitation sector's environmental impacts, the essential question is why this idea has so far not scaled beyond niche applications. In the remainder, we will review recent academic and grey literature that has analyzed the evolution of this innovation from the early days until today and identify key bottlenecks for its future development. We follow other reviews in the field by conceptualizing urine source separation as a systemic innovation challenge, where successful diffusion depends on the creation of a supportive innovation system that encourages innovation in all parts of a complex value chain reaching from the toilet interface to reuse in agriculture. We will argue that as in many other SDG-related sustainability challenges, diffusing this innovation needs a full systems view that many governments and other key stakeholders in the sanitation field are still lacking.2
A first key insight from academic literature on urine source separation is that mainstreaming this innovation is of formidable complexity, not only from a technical, but also from a social point of view.67–69 What is at stake here is not a conventional product innovation (as e.g. a new smartphone model that can be sold in global, pre-existing mass markets), but a disruptive technical approach that contradicts the taken-for-granted state of the art – or ‘socio-technical regime’ in its field.2,70 The creation of two waste streams (instead of one) inside buildings, which need to be separately managed, challenges taken-for-granted core technologies, regulations and policies in the sanitation sector. This creates various frictions with incumbent actors, professional standards and user routines,2,58,68,70,71 and implies that urine source-separating technologies cannot diffuse in a linear commercialization pattern. Instead, development depends on repeated experiments with social and technical elements before first ‘configurations that work’ emerge, which can fundamentally challenge the existing regime.69,71,72 Innovation on the technology side thus have to closely co-evolve with social innovations like changing effluent quality standards, adapting the curricula for architects and plumbers, teaching users about new ways of using and cleaning toilets, etc.
To complicate matters further, the success of urine source separation also depends on innovation in various interconnected parts of a complex value chain.68,69,73 For the whole system to achieve the environmental benefits discussed in prior sections, innovation at the front-end (user interface or toilet), has to be matched with innovation at the mid-end (piping, storage, transport, logistics), and the back-end (treatment systems creating marketable end-products). Urine-based fertilizer products will then have to find broad uptake in agriculture. Creating a complete value chain furthermore depends on innovation in complementary sectors like design, advertising, finance, or legal services. Streamlining all these elements to each other takes a long-term socio-technical experimentation process, which depends on iterative cycles of learning from failures and typically takes several decades to succeed.71,74,75
We have found particularly well suited frameworks for conceptualizing the past innovation journey of urine source separation in the academic literature on technological innovation systems and socio-technical transitions (e.g. ref. 68, 69 and 76). Bergek et al.77 suggest that a systemic innovation like urine source separation depends on a network of supportive actors and institutions – i.e. a ‘technological innovation system’, TIS, which forms around the core technology and helps develop and diffuse the new approach.77,78 TIS often start off in protected spaces and then scale up and out into new contexts, becoming more and more elaborate and powerful in challenging a sector's taken-for-granted regime.71,77 Four key resources have to be created in the system to enable successful diffusion: knowledge, markets, financial investment, and legitimacy.79 If any of these elements is missing, the technology will either not diffuse or be confronted with major development barriers. In such cases, entrepreneurs will have to find ways to overcome the system weaknesses and adapt actor networks and institutions in a way that they become supportive of the innovation.68,80
In the remainder, we will review the history of urine source-separating technologies in light of these four key system resources and a full value chain perspective to assess how the innovation has developed in the past, where current bottlenecks for development are, and which next steps are necessary to further scale up the idea. Several review articles on local developments have recently been published.67–69 We complement these excellent studies by applying a global perspective on the evolution of the urine source separation idea and explicitly relating it back to the UN's SDG agenda.
Phase 1: local innovation in eco-villages and research projects (1990–2000)
The idea of source separation originates from local experiments in Sweden, mostly based on small research projects and early implementations in niche markets.67,68,81 Knowledge on source separation started developing from the early 90s on. At this most initial stage, conceptual work at universities33 evolved in parallel with local bottom-up, low-tech innovation processes in Sweden, with strong interactions between academia and practitioners.82,83 Swedish entrepreneurs were the first to develop urine-separating toilet designs and directly implement them in summerhouses and eco-villages.68 The Swedish context constituted first niche markets for experimenting with the technology. Especially eco-villages provided protected (and subsidized) spaces to test various toilet designs and to gain scientific knowledge on user acceptability and market potentials.51,68 Before 2006, more than 3000 urine-separating toilets had been installed in these experimental settings and 135
000 source-separating toilets in Sweden as a whole.51,68 Some authors even estimate that by 2009, 2% of all households in Sweden were using source separation technologies, most of which were composting or dry toilets.84 This phase resulted in extensive experience and user feedback,51 but no scaling beyond early niche contexts happened due to various unresolved technical and institutional issues discussed below.68,85
Financial investment in the local innovation phase mostly came from research projects, the Swedish government, local municipalities the dwellers of eco-villages, as well as environmental idealists in green movements.68 Two small, pioneering Swedish manufacturers that started investing in the design and distribution of urine-separating toilets in 1991 are still active in relevant niche markets today.86,87 Legitimacy of the innovation in this local innovation phase remained largely confined to specific rural communities. It varied strongly between different communities and academic expert circles and the broader public were not especially affected by (or interested in) the innovation.68,69 Initially, supportive narratives built strongly on the opportunity to close the nutrient cycle, especially for the limited resource phosphorus.83,88,89 The business model for reuse of urine in agriculture was simple: urine was stored for long enough to obtain a sanitized product, which was subsequently collected by local farmers90 and there were no attempts to produce a volume-reduced fertilizer suited for transport.68 Furthermore, many of the toilets did not work properly, did not look nice, and had problems with smell, urine precipitation, and clogging of valves.68,69 Finally, a stable reuse market for the stored urine was lacking in many communities.51,68
The relevant TIS structures in this first phase thus remained limited to small niches, where first knowledge about the technology developed. Financial investment, market formation and legitimacy remained limited and a functional front-, mid- and backend was largely missing. It took about ten years until some better designed and functional systems appeared that moved the idea to a next development stage.
Phase 2: local validation in demonstration projects in different countries (2000–2010)
In the early 2000s, urine source separation reached a first milestone with the setup of large research projects and pilot and demonstration (PD) projects in various parts of the world.51,57,91 In Europe alone, 38 PD projects with urine-separating toilets were realized in that phase.51 Some projects like the Eawag main building in Switzerland,67 the headquarters of GIZ in Germany,92 or Solarcity, a settlement in Linz, Austria,93 created first socio-technical configurations that worked (at least for some time), thus promoting the technology at the scale of an urban building. In this context, the German company Roediger developed a first mass-producible urine diverting flush toilet, which was applied in the above PD projects.57 The toilet design however retained some fundamental design problems related to urine precipitation, clogging of valves and users complaining about the need to sit on the toilet in order to make the separation system work.92 The idea did consequently not scale beyond local PD projects.
In this second phase, knowledge development intensified in dedicated research projects,91 which increasingly profited from practical knowledge accumulating in Sweden and from the field applications of the new toilet in PD projects. A key result from these initiatives was the high acceptance of the concept by end users, but the slow uptake of the actual urine-separating toilets on the market.51,81 Additional issues arose, especially with the servicing of the toilets in large office buildings,58,67,68 and the lack of volume-reduced fertilizer production, which would enable transport from dense cities to agricultural areas.60
Markets in this phase further differentiated, with challenges arising both around introducing urine diverting toilets and treatment systems in buildings, as well as creating marketable fertilizer products for agriculture. The application of urine diverting systems remained largely restricted to PD and niche projects, which now however expanded beyond the Swedish context, to other European countries,51 Africa,52 and Asia,52,53,94–96 albeit with different toilet technologies in the different socio-economic and cultural contexts. As mentioned before, the municipality of Durban in South Africa was the first to implement urine source separation at scale, yet, with rather mixed results.53 Regarding agricultural markets, a survey conducted by Pahl-Wostl et al.66 amongst Swiss farmers showed that acceptance of a urine-based fertilizer would be high, if the fertilizer was easy to apply, hygienically safe and devoid of pharmaceuticals excreted in urine, thus pointing to a large future market potential.
Financial investment was mostly mobilized by research projects, but also increasingly through third-party contributions to PD projects, R&D departments in large firms and some additional small donors and SMEs.68 Roediger invested substantial resources into developing the new toilet design, but eventually abandoned the project, because sales volumes remained below expectations. Legitimacy for urine source separation remained mixed in this phase. The technology had been successfully demonstrated in some local PD projects, but it also remained strongly challenged by many experts in the sanitation sector.69,93 Key problems persisted with the user interface, i.e. existing toilet designs were not suitable for children using the toilet, men standing, women not sitting etc.67,68,95,97 Combined with failures in some high-profile demonstration projects (e.g. in Ordos City, China97), urine source separation approaches kept struggling with persistent legitimacy issues. In particular, it became obvious that the front-end (toilet interface) and back-end (treatment technologies), as well as the interface with agricultural reuse needed further refinement.
Phase 3: global diffusion is taking off (2010–today)
Due to the mixed experiences from many PD projects in developed economies, urine source separation from 2010 on started diffusing mostly into emerging and developing countries, where needs for novel sanitation approaches were most pressing and opportunities for further innovation high.54,67,96 In parallel, new actors like The Bill & Melinda Gates Foundation (BMGF), designers (EOOS), and additional toilet manufacturers entered the field and started supporting the innovation system with new ideas, additional investment, know-how and access to international networks and markets.
In terms of knowledge creation, innovative activities in the front-end initially largely focused on a high-tech approach.67 A new manufacturer developed pilot-type toilets including complex sensors that could distinguish urine from water.98 This toilet however never reached market maturity. In parallel, major design improvements happened in the field of container-based solutions. Especially a novel squatting urine-diverting dry toilet by Sanergy enjoys high user acceptance.99 Research on various treatment processes and potential uses in agriculture also intensified,100 and a large research initiative was founded in France, which strategically researched the potential for introducing a full urine source separation full value chain (including agricultural reuse), in the larger Paris metropolitan area.101,102 Finally, also the development of urine treatment technology for the back-end considerably intensified in this phase, with more conventional treatment methods advancing towards the pilot scale,62,64,103,104 and with a boost of new high-tech experimental electrochemical processes.105 In addition, a large number of articles discussed the quality of a urine-based fertilizer with respect to organic micropollutants, e.g. ref. 106 and 107. For an overview of the general surge in articles on urine treatment, see ref. 61.
The TIS also strongly profited from the Bill and Melinda Gates Foundation's move into the sanitation field. In 2010–11, the foundation first financed a large project on urine source separation in Durban,108 and then initiated a major research program (Re-invent The Toilet Challenge, RTTC). This challenge sponsored research teams for inventing high-tech sanitation systems that could work in informal settlements without any access to water networks and sewers at a price of less than US$ 0.05 per person and day.15 Some of those projects involved urine source separation (e.g. ref. 109 and 110), and in 2017, the development of alternative source-separating toilet interfaces became an explicit strategy of the foundation. A grant given to EOOS111 spurred a major breakthrough in toilet design, with the invention of the ‘urine trap’ in 2017. The urine trap is based on the teapot effect, a well-known hydrological phenomenon,112 illustrated in Fig. 2. This new design enables the implementation of urine source-separating technology without any needs for behavior change and it allows manufacturers to produce toilets without additional valves or sensors, which are difficult to implement in conventional production schemes for ceramic or plastic toilets.112
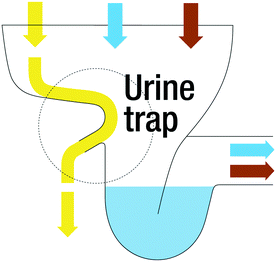 |
| Fig. 2 Basic principle of urine-separating flush toilets using the teapot effect. Copyright EOOS. | |
Markets.
In this phase, the literature reports increasing diffusion of the idea into growing niche markets around the world. Promising market segments reported in the literature comprise: (1) large events, sports campuses and mountain huts;113 (2) informal settlements in low-income countries;110,114,115 (3) new city districts, university campuses and parks in South Africa, China and Paris;102,116 or (4) applications in cooperative and temporary housing projects in Geneva or Paris.117,118 First business models now also emerged around the production and distribution of urine-based fertilizer, which got an allowance also for all edible crops from the Swiss authorities in 2018.63,119
Financial investment also started to flow from a broader variety of sources, including BMGF, a number of national research funders (i.e. in Sweden, the USA, China, etc.) and some development NGOs or social enterprises like Sanergy in Kenya.42,99 In combination with the increase in knowledge and the invention of the urine trap (Fig. 2), it became possible to convince a multinational toilet manufacturer (Laufen) to enter the innovation system and invest in developing an aesthetically pleasing toilet design, which targeted a high-end, environmentally-friendly customer segment (Fig. 3). At a high-profile toilet fair in 2018, the World Bank and BMGF committed themselves to unlock at least 1bn. US$ of investment for innovative sanitation technologies, including urine source separation.120,121 At about the same time, the greater Paris region, L'Agence de l' Eau Seine-Normandie instituted the first large-scale subsidy for installation of urine source-separating technologies in new residential districts.122
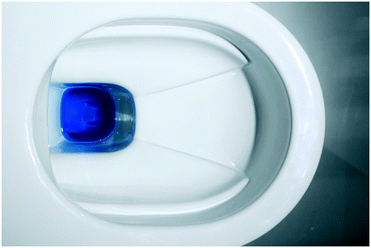 |
| Fig. 3 The urine-separating toilet safe! from LAUFEN, implementing the urine trap. The special structures at the front-end of the toilet direct the urine towards the (nearly invisible) urine trap.112 Copyright LAUFEN.123 | |
For the innovation system as a whole, especially the engagement of BMGF was an additional boost in legitimacy. BMGF supported the idea both directly through Bill Gate's own media appearances and indirectly by pulling new actors like design firms (EOOS), toilet manufacturers (Laufen, various firms in emerging economies) or large investors (the World Bank) into the field.121,124 In parallel, actors in the expanding innovation system jointly worked on re-instating legitimacy by developing narratives that connected urine source separation to popular cultural frames like the circular economy, sustainability, but also to design, beauty and poetry.125–127 In Europe and the USA, supportive stories were framed around baking bread or brewing beer from crops fertilized with urine and widely taken up in public media.128–130 In addition, NGOs like the Rich Earth Institute and research programs like OCAPI have recently invested heavily into creating research, public outreach and education campaigns, as well as establishing a regulatory pathway for agricultural urine reuse in the US and France.102,130 Last but not least, a number of prestigious prizes, e.g. the wallpaper ‘life enhancer of the year’ award127 and “black bee” for an installation on urine source separation at the 2019 Triennale “Broken Nature” in Milano,131 helped reframe the concept as a beautiful idea to pursue for creating a more sustainable world.
5. Outlook: current state of the art, key bottlenecks and potential application contexts for the scaling up of urine source separation technologies
The past innovation journey summarized above exemplifies how long it may take until a systemic innovation like urine source separation is taken up in practice, even if it promises clear benefits in achieving environmental and social benefits as outlined in the SDGs. In 2021, after a 30 year long journey, urine source separation is still not a taken-for-granted alternative to the conventional regime solution in the water sector. This notwithstanding, some key innovation bottlenecks have been successfully mitigated in the process. Especially knowledge development and financial investment have seen considerable progress over the past three decades and the actor structure has diversified both in breadth and scope. Large donors and multinational firms, plus various startups and research institutes have improved the overall innovation system structure, which has now entered a phase of international diffusion, even though the technology is still struggling with pushback from incumbents in most contexts.
Our review has shown that one key breakthrough arguably happened at the front end. Until the mid-2010s, an important obstacle for successful technology diffusion was the lack of attractive urine-separating toilets. This caused a hen-and-egg problem that is typical for systemic innovation: without suitable toilets for pilot projects, no market and legitimacy could arise; and without market and legitimacy, no producer had an interest in investing in developing toilets or suitable mid- and back-end solutions. The new toilet design in combination with innovative treatment technologies constitute a potential milestone in further diffusing the idea, because they enable the planning of new major demonstration and lighthouse projects, and eventually the implementation at the level of whole city districts. Table S1† outlines some demonstration projects that are currently under development. We expect that a further surge of development in the TIS for urine source separating technologies will result from these projects.
A second key breakthrough is required for the back-end. While piloting has already been successful for several treatment technologies, only industrialization, automation and mass production will lead to low-cost competitive solutions. At present, two spin-off companies pursue the commercialization of urine treatment technology. Sanitation 360, a spin-off from the Agricultural University in Uppsala, Sweden, recently won the Skapa prize, the largest Swedish innovation prize in memory of Alfred Nobel, for the alkaline dehydration technology suited for bathroom installation.132 Vuna, a spin-off of Eawag, Switzerland, offers solutions for larger buildings to produce the concentrated fertilizer Aurin.113 Both firms have not yet reached full automation and mass-manufacturing capacities, which we consider crucial for the further diffusion of urine source-separating technologies.
Industrializing small-scale urine treatment technology is a different task from involving the sanitary industry in producing a new toilet. When we build conventional WWTPs, we do this according to what we may call the “civil engineering logic”. Every treatment plant is a prototype, built for purpose and cast in concrete like any other building, with a number of specialized industries providing the standardized electro-mechanical parts like pumps, aeration, and process control systems. For small-scale urine treatment systems, an “industrial logic” applies, where one company mass-produces standardized treatment units.133,134 Such companies exist for the production of simple small-scale treatment systems treating combined wastewater, e.g. in rural areas, but until now, no large industrial actor exists, which can drive the mass production of the urine treatment technologies discussed above.114 Treatment technologies, however, do not have the same cultural interpretation as toilets, so the chances may be larger that markets for treatment technology grow faster and more globally than for toilets.
Another key bottleneck for future diffusion is the lack of streamlined norms and standards for urine source separating technologies. At the front-end, more design norms exist globally for toilets than for electricity plugs, and no norms at all exist for source-separating toilets. Many toilet manufacturers have thus shied away from investing in novel, non-standard toilet designs. In view of this situation, BMGF, EOOS NEXT and various non-profit actors currently pursue a strategy to provide open design templates and technical support for urine-separating toilets based on the urine trap for fast commercialization in low and middle-income countries (Table S2† and Fig. 4). In addition, a new global industry standard for ‘non-sewered sanitation systems’ (ISO 30500) has recently been developed,135 with the intention to smoothen the playing field for commercial actors. The practical impacts of this highly aspirational standard are still somewhat hard to predict.136
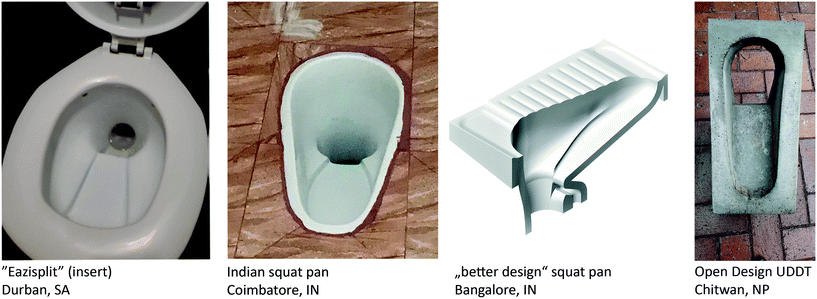 |
| Fig. 4 Examples of the urine trap integration for low-income countries, developed by EOOS NEXT in cooperation with local and global partners. Copyright EOOS NEXT. More information in ESI,† Table S2. | |
A number of challenges also remain at the mid-end, mostly relating to a lack of knowledge and capacity among architects and plumbers on the potential benefits and concrete implementation of urine source-separating technologies in buildings. Several of the studies reviewed above cite persistent problems with the planning, installation and proper maintenance of urine source-separating systems in buildings.57,58,67–69 Introducing urine source-separating technologies into the standard curriculum of architects, plumbers and environmental engineers, as well as developing solid O&M models for on-site urine treatment system should thus be a future focus of development. Increased cooperation between firms, research institutes, authorities and artisans will be needed to develop and quickly diffuse this type of supporting social innovation in the TIS.
A final key bottleneck is the lacking market for urine-based fertilizer products in agriculture. As discussed above, this is the crucial step for realizing the technology's full contribution to the SDG agenda, which rests on the idea of a circular economy model for the nutrients available in urine. One problem is the lack of government certification for urine-based fertilizer in many countries, combined with farmer's and consumer's possible skepticism toward this new type of fertilizer.66,137–139 A second barrier is that nutrients derived from urine still have a relatively low economic value, especially when compared with conventional, synthetic fertilizers.69 Potential ways to overcome these bottlenecks would be pushing for certification of urine-based fertilizer products with national and supra-national authorities (i.e. the EU), as well as incentivizing the uptake of urine-based fertilizers through climate legislation that applies tariffs and taxes to more polluting, conventional fertilizer products (i.e. taxes on energy-intensive processes like N-fixation).69
Last, but not least, we would like to shortly discuss three ideal-type application contexts, in which urine source separation could arguably most directly reap the SDG-related benefits brought up in prior sections. A first promising context are informal settlements in developing countries, which combine strong sanitation problems with a lack of conventional, centralized infrastructure. In some cases, these are currently served by social enterprises like Sanergy, which supply bottom of the pyramid markets with container-based sanitation solutions.110,114 Sanergy already successfully applies urine-separating toilets, but still searches for the technology to produce a urine-based fertilizer instead of infiltrating urine.43 Adding urine source separation to their activities while scaling up their business model would not only protect the environment against nitrogen and slum dwellers against water-borne diseases, but also provide a much-needed additional income to expand the social enterprise's operation and help overcome local fertilizer shortages. In this extremely resource-limited environment, urine source separation can thus concomitantly help achieve SDGs 2, 6, 11 and 14.1.
Second, the Rich Earth Institute, based in Vermont, US, is an example of a non-profit organization running a urine source-separation program that targets communities with pre-existing on-site sanitation systems (mostly septic tanks).106,130,140 In this case, the main driver is the double benefit of local water pollution control and resource recovery outlined in section 3, thus targeting SDGs 2, 6 and 14.1. While this program is close to the early programs in Sweden, it goes much further by taking up regulatory aspects like fertilizer permits, and addresses a number of important stakeholders like plumbers in the innovation activities. Rich Earth Institute also produces a fertilizer based on acidification, pasteurization and membrane filtration.130 It is interesting to observe that the demand for this group's urine-based fertilizer currently exceeds local supplies.130
Finally, in Europe, a movement for urine source separation has built up in the greater Paris area in France around the university-based program OCAPI.101,118 In this case, urine source separation is envisioned as an add-on to existing centralized sewer systems in the high-density urban environment of high-income countries. Similar to the cases above, SDG-related benefits revolve around protecting aquatic ecosystems (SDG 14.1), while promoting sustainable agriculture (SDG 2) and creating more resilient cities (SDG 11). The main narratives accordingly emphasize circular economy thinking102 and the protection of the river Seine that leads into a highly eutrophic coastal area.141 Noticeable, important stakeholders in the region are increasingly interested in urine source separation, e.g. the water utility SIAPP, responsible for wastewater treatment in the greater Paris area,142 and l'Agence de l'eau Seine-Normandie,122 a French authority recently implementing high subsidies for the installation of urine-separating technology in this part of France.
6. Conclusions
With the example of urine diversion, we have shown how long it may take before a systemic and radical innovation reaches the point where global implementation becomes a plausible option. This is critical for the SDGs, where in many cases existing mainstream technologies prevail even though they have clearly shown their inability to solve essential social and environmental problems. Urban water management is one of those hugely important fields, where most people assume that suitable technologies exist and just need implementation. Drawing on the literature from a diversity of fields, we have shown that this is not the case. For instance, the sanitation problem of rapidly growing cities in low-income countries (SDG 6) cannot be solved with highly planning and capital-intensive centralized technologies. The typical stepwise implementation of treatment technology in the centralized sanitation regime will furthermore fail to reduce nutrient emissions as required by SDG 14.1, even if we extend the time line to 2050.
Further developing and diffusing urine source separation technologies is thus arguably one of the scarce leverage points that help addressing several SDGs in parallel and (re-)establishing a circular economy model around urban sanitation and food production. Our review has shown that this systemic innovation has come a long way in developing a full value chain and supportive innovation system. Public perception is increasingly favorable and the technology has made important steps toward wider legitimation. The innovation now stands in the midst of a (global) diffusion phase, with key bottlenecks revolving around the standardization of key components, challenges in the mid-end, as well as mass-production of treatment technologies and lacking reuse markets in agriculture. We encourage future work to focus on these issues, in order to enable urine source separation to fulfill its role in achieving the SDGs.
Conflicts of interest
Harald Gruendl is managing director of the social enterprise EOOS NEXT (http://www.eoosnext.com), which works under an industrialization grant of the Bill & Melinda Gates Foundation to find industrial partners for the “Urine Trap” technology to serve people in need in the target countries South Africa, India and China. For the projects in Table S2† by EOOS NEXT all activities are for non-profit. The “Urine Trap” technology IP is co-owned by the Bill & Melinda Gates Foundation and EOOS.
Acknowledgements
The Bill & Melinda Gates Foundation (BMGF) financed the work of EOOS and EOOS NEXT presented in this paper with two grants: Grant OPP1149059 for EOOS and Grant OPP1192614 for EOOS NEXT. The authors thank Lotte Kristoferitsch, EOOS NEXT, for the careful preparation of the figures and the provision of technical information.
References
- I. N. Daliakopoulos and S. Keesstra, TERRAenVISION: Science for Society, Environmental issues today, Sci. Total Environ., 2020, 704, 135238 CrossRef CAS PubMed.
- S. Hoffmann, U. Feldmann, P. M. Bach, C. Binz, M. Farrelly, N. Frantzeskaki, H. Hiessl, J. Inauen, T. A. Larsen, J. Lienert, J. Londong, C. Lüthi, M. Maurer, C. Mitchell, E. Morgenroth, K. L. Nelson, L. Scholten, B. Truffer and K. M. Udert, A Research Agenda for the Future of Urban Water Management: Exploring the Potential of Nongrid, Small-Grid, and Hybrid Solutions, Environ. Sci. Technol., 2020, 54(9), 5312–5322 CrossRef CAS PubMed.
-
J. Mokyr, The Lever of Riches: Technological Creativity and Economic Progress, Oxford University Press, USA, 1990 Search PubMed.
-
UN General Assembly, Transforming our world: the 2030 Agenda for Sustainable Development, https://www.refworld.org/docid/57b6e3e44.html, [accessed 30.11.2020].
- D. Mara and B. Evans, The sanitation and hygiene targets of the sustainable development goals: Scope and challenges, J. Water, Sanit. Hyg. Dev., 2018, 8(1), 1–16 CrossRef.
- B. C. O'Neill, E. Kriegler, K. Riahi, K. L. Ebi, S. Hallegatte, T. R. Carter, R. Mathur and D. P. van Vuuren, A new scenario framework for climate change research: The concept of shared socioeconomic pathways, Clim. Change, 2014, 122(3), 387–400 CrossRef.
- D. P. van Vuuren, E. Kriegler, B. C. O'Neill, K. L. Ebi, K. Riahi, T. R. Carter, J. Edmonds, S. Hallegatte, T. Kram, R. Mathur and H. Winkler, A new scenario framework for Climate Change Research: Scenario matrix architecture, Clim. Change, 2014, 122(3), 373–386 CrossRef.
- V. J. Schweizer and B. C. O'Neill, Systematic construction of global socioeconomic pathways using internally consistent element combinations, Clim. Change, 2014, 122(3), 431–445 CrossRef.
- J. Y. Liu, S. Fujimori, K. Takahashi, T. Hasegawa, W. Wu, Y. Geng, J. Takakura and T. Masui, The importance of socioeconomic conditions in mitigating climate change impacts and achieving Sustainable Development Goals, Environ. Res. Lett., 2021, 16(1), 014010 CrossRef.
- A. Ferriman, BMJ readers choose the “sanitary revolution” as greatest medical advance since 1840, Br. Med. J., 2007, 334(7585), 111 CrossRef.
- C. Hamlin and P. Sidley, Revolutions in public health: 1848, and 1998?, Br. Med. J., 1998, 317, 587 CrossRef CAS PubMed.
-
D. M. Cutler, A. S. Deaton and A. Lleras-Muney, The determinants of mortality, Working Paper 11963, National Bureau of Economic Research, Cambridge, MA 02138, 2006 Search PubMed.
- P. Naden, V. Bell, E. Carnell, S. Tomlinson, U. Dragosits, J. Chaplow, L. May and E. Tipping, Nutrient fluxes from domestic wastewater: A national-scale historical perspective for the UK 1800–2010, Sci. Total Environ., 2016, 572, 1471–1484 CrossRef CAS PubMed.
-
WHO and UNICEF, Progress on Drinking Water, Sanitation and Hygiene: 2017 Update and SDG Baselines, World Health Organization (WHO) and the United Nations Children's Fund (UNICEF), Geneva, Switzerland, 2017, assessed 07.05.2021, available at: http://apps.who.int/iris/bitstream/handle/10665/258617/9789241512893-eng.pdf;jsessionid=0A90368E83B640AF9FB95D985E0C7218?sequence=1 Search PubMed.
- J. N. Bhagwan, S. Pillay and D. Koné, Sanitation game changing: Paradigm shift from end-of-pipe to off-grid solutions, Water Pract. Tech., 2019, 14(3), 497–506 CrossRef.
- P. J. T. M. van Puijenbroek, A. H. W. Beusen and A. F. Bouwman, Global nitrogen and phosphorus in urban waste water based on the Shared Socio-economic pathways, J. Environ. Manage., 2019, 231, 446–456 CrossRef CAS PubMed.
- K. F. Bartz, Cost oriented prices and pricing policy of public enterprises under the aspect of different funding
models for investment in the field of water supply and sewage treatment and disposal, Water Supply, 1997, 15(3), 39–49 Search PubMed.
- T. J. Alexander, P. Vonlanthen and O. Seehausen, Does eutrophication-driven evolution change aquatic ecosystems?, Philos. Trans. R. Soc., B, 2017, 372(1712), 20160041 CrossRef.
- J. Rockstrom, W. Steffen, K. Noone, A. Persson, F. S. Chapin, III, E. F. Lambin, T. M. Lenton, M. Scheffer, C. Folke, H. J. Schellnhuber, B. Nykvist, C. A. de Wit, T. Hughes, S. van der Leeuw, H. Rodhe, S. Sorlin, P. K. Snyder, R. Costanza, U. Svedin, M. Falkenmark, L. Karlberg, R. W. Corell, V. J. Fabry, J. Hansen, B. Walker, D. Liverman, K. Richardson, P. Crutzen and J. A. Foley, A safe operating space for humanity, Nature, 2009, 461(7263), 472–475 CrossRef PubMed.
- S. Poikane, M. G. Kelly, F. Salas Herrero, J. A. Pitt, H. P. Jarvie, U. Claussen, W. Leujak, A. Lyche Solheim, H. Teixeira and G. Phillips, Nutrient criteria for surface waters under the European Water Framework Directive: Current state-of-the-art, challenges and future outlook, Sci. Total Environ., 2019, 695, 133888 CrossRef CAS PubMed.
- J. G. Conijn, P. S. Bindraban, J. J. Schröder and R. E. E. Jongschaap, Can our global food system meet food demand within planetary boundaries?, Agric., Ecosyst. Environ., 2018, 251, 244–256 CrossRef CAS.
- T. A. Larsen, S. Hoffmann, C. Löthi, B. Truffer and M. Maurer, Emerging solutions to the water challenges of an urbanizing world, Science, 2016, 352(6288), 928–933 CrossRef CAS PubMed.
- W. De Vries, J. Kros, C. Kroeze and S. P. Seitzinger, Assessing planetary and regional nitrogen boundaries related to food security and adverse environmental impacts, Curr. Opin. Environ. Sustain., 2013, 5(3–4), 392–402 CrossRef.
- W. Steffen, K. Richardson, J. Rockström, S. E. Cornell, I. Fetzer, E. M. Bennett, R. Biggs, S. R. Carpenter, W. De Vries, C. A. De Wit, C. Folke, D. Gerten, J. Heinke, G. M. Mace, L. M. Persson, V. Ramanathan, B. Reyers and S. Sörlin, Planetary boundaries: Guiding human development on a changing planet, Science, 2015, 347(6223), 1259855 CrossRef PubMed.
- B. L. Bodirsky, A. Popp, H. Lotze-Campen, J. P. Dietrich, S. Rolinski, I. Weindl, C. Schmitz, C. Müller, M. Bonsch, F. Humpenüder, A. Biewald and M. Stevanovic, Reactive nitrogen requirements to feed the world in 2050 and potential to mitigate nitrogen pollution, Nat. Commun., 2014, 5, 3858 CrossRef CAS PubMed.
-
UNEP, Colombo Declaration on Sustainable Nitrogen Management, https://papersmart.unon.org/resolution/uploads/colombo_declaration_final_24_oct_2019.pdf, [accessed 30.11.2020].
- I. Pikaar, S. Matassa, K. Rabaey, B. L. Bodirsky, A. Popp, M. Herrero and W. Verstraete, Microbes and the Next Nitrogen Revolution, Environ. Sci. Technol., 2017, 51(13), 7297–7303 CrossRef CAS.
- T. A. Larsen, M. Maurer, K. M. Udert and J. Lienert, Nutrient cycles and resource management: Implications for the choice of wastewater treatment technology, Water Sci. Technol., 2007, 56(5), 229–237 CrossRef CAS PubMed.
- P. L. McCarty, What is the Best Biological Process for Nitrogen Removal: When and Why?, Environ. Sci. Technol., 2018, 52(7), 3835–3841 CrossRef CAS PubMed.
-
M. Maurer, Full costs, (dis-)economies of scale and the price of uncertainty, in Source Separation and Decentralization for Wastewater Management, ed. T. A. Larsen, J. Lienert and K. M. Udert, IWA Publishing, London, New York, 2013, pp. 85–100 Search PubMed.
- G. Öberg, G. S. Metson, Y. Kuwayama and S. A. Conrad, Conventional sewer systems are too time-consuming, costly and inflexible to meet the challenges of the 21st century, Sustainability, 2020, 12(16), 6518 CrossRef.
-
C. Platzer, H. Hoffmann and E. Ticona, Alternatives to Waterborne Sanitation – a Comparative Study – Limits and Potentials, Proceedings of the IRC Symposium: Sanitation for the Urban Poor, Delft, The Neatherlands, 2008 Search PubMed.
- T. A. Larsen and W. Gujer, Separate management of anthropogenic nutrient solutions (human urine), Water Sci. Technol., 1996, 34(3–4), 87–94 CrossRef CAS.
- D. Hellström and E. Kärrman, Exergy analysis and nutrient flows of various sewerage systems, Water Sci. Technol., 1997, 35(9), 135–144 CrossRef.
- J. A. Wilsenach and M. C. M. van Loosdrecht, Integration of processes to treat wastewater and source-separated urine, J. Environ. Eng., 2006, 132(3), 331–341 CrossRef CAS.
- S. Dasgupta, N. Agarwal and A. Mukherjee, Moving up the On-Site Sanitation ladder in urban India through better systems and standards, J. Environ. Manage., 2021, 280, 111656 CrossRef PubMed.
- A. Mažeikienė and R. Grubliauskas, Biotechnological wastewater treatment in small-scale wastewater treatment plants, J. Cleaner Prod., 2021, 279, 123750 CrossRef.
- M. Kulak, N. Shah, N. Sawant, N. Unger and H. King, Technology choices in scaling up sanitation can significantly affect greenhouse gas emissions and the fertiliser gap in India, J. Water, Sanit. Hyg. Dev., 2017, 7(3), 466–476 CrossRef.
- L. S. A. Yiougo, H. Koanda, J. Wethe, C. Luthi, O. Yapo and E. D. Dapola, The method of material flow analysis, a tool for selecting sustainable sanitation technology options: The case of Pouytenga (Burkina Faso), WIT Trans. Ecol. Environ., 2011, 145, 671–680 Search PubMed.
-
U. Nations, SDG 11: Make cities and human settlements inclusive, safe, resilient and sustainable, https://unstats.un.org/sdgs/report/2019/goal-11/, [accessed 02.03.2021].
- L. Couder and S. Kibuthu, Unlocking the circular economy potential to tackle the sanitation challenge, Field Actions Sci. Rep., 2020, 2020(Special Issue 22), 72–77 Search PubMed.
-
Sanergy, Introducing VUNA – recovering nutrients from urine to promote sustainable sanitation, http://www.sanergy.com/2016/08/31/introducing-vuna-recovering-nutrients-from-urine-to-promote-sanitation/, [accessed 30.11.2020].
- K. C. Russel, K. Hughes, M. Roach, D. Auerbach, A. Foote, S. Kramer and R. Briceño, Taking Container-Based Sanitation to Scale: Opportunities and Challenges, Front. Environ. Sci. Eng., 2019, 7, 190 CrossRef.
- R. J. P. Schmitt, E. Morgenroth and T. A. Larsen, Robust planning of sanitation services in urban informal settlements: An analytical framework, Water Res., 2017, 110, 297–312 CrossRef CAS PubMed.
- S. P. Hilton, G. A. Keoleian, G. T. Daigger, B. Zhou and N. G. Love, Life Cycle Assessment of Urine Diversion and Conversion to Fertilizer Products at the City Scale, Environ. Sci. Technol., 2021, 55(1), 593–603 CrossRef CAS PubMed.
- H. Kjerstadius, A. Bernstad Saraiva, J. Spångberg and Å. Davidsson, Carbon footprint of urban source separation for nutrient recovery, J. Environ. Manage., 2017, 197, 250–257 CrossRef CAS PubMed.
- R. Malila, S. Lehtoranta and E. L. Viskari, The role of source separation in nutrient recovery – Comparison of alternative wastewater treatment systems, J. Cleaner Prod., 2019, 219, 350–358 CrossRef CAS.
- D. L. Medeiros, L. M. Queiroz, E. Cohim, J. A. D. Almeida-Neto and A. Kiperstok, Human urine fertiliser in the Brazilian semi-arid: Environmental assessment and water-energy-nutrient nexus, Sci. Total Environ., 2020, 713, 136145 CrossRef CAS PubMed.
- C. M. J. Pausta, L. F. Razon, M. A. B. Promentilla and D. P. Saroj, Life cycle assessment of a retrofit wastewater nutrient recovery system in metro Manila, Chem. Eng. Trans., 2018, 70, 337–342 Search PubMed.
- J. Spångberg, P. Tidåker and H. Jönsson, Environmental impact of recycling nutrients in human excreta to agriculture compared with enhanced wastewater treatment, Sci. Total Environ., 2014, 493, 209–219 CrossRef PubMed.
- J. Lienert and T. A. Larsen, High acceptance of urine source separation in seven European countries: A review, Environ. Sci. Technol., 2010, 44(2), 556–566 CrossRef CAS.
- Y. Abarghaz, M. Mahi, N. Bendaou, M. Fekhaoui and C. Werner, Lessons learnt on Ecosan in Morocco: Case of the urine-diversion dehydration toilets, J. Water Reuse Desalin., 2013, 3(1), 55–68 CrossRef.
-
T. Gounden, B. Pfaff, N. Macleod and C. Buckley, Provision of free sustainable basic sanitation: The durban experience, Sustainable Development of Water Resources, Water Supply and Environmental Sanitation: Proceedings of the 32nd WEDC International Conference, 2007.
- E. Roma, K. Philp, C. Buckley, S. Xulu and D. Scott, User perceptions of urine diversion dehydration toilets: Experiences from a cross-sectional study in eThekwini Municipality, Water SA, 2013, 39(2), 305–312 Search PubMed.
- P. Bond, Tokenistic water and neoliberal sanitation in post-apartheid Durban, J. Contemp. Afr. Stud., 2019, 37(4), 275–293 CrossRef.
- K. M. Udert, T. A. Larsen, M. Biebow and W. Gujer, Urea hydrolysis and precipitation dynamics in a urine-collecting system, Water Res., 2003, 37(11), 2571–2582 CrossRef CAS PubMed.
- J. Lienert and T. A. Larsen, Pilot projects in bathrooms: A new challenge for wastewater professionals, Water Pract. Tech., 2007, 2(3) DOI:10.2166/WPT.2007057.
- T. H. Boyer and D. Saetta, Opportunities for Building-Scale Urine Diversion and Challenges for Implementation, Acc. Chem. Res., 2019, 52(4), 886–895 CrossRef CAS PubMed.
- D. Saetta, A. Padda, X. Li, C. Leyva, P. B. Mirchandani, D. Boscovic and T. H. Boyer, Water and Wastewater Building CPS: Creation of Cyber-Physical Wastewater Collection System Centered on Urine Diversion, IEEE Access, 2019, 7, 182477–182488 Search PubMed.
- M. Maurer, W. Pronk and T. A. Larsen, Treatment processes for source-separated urine, Water Res., 2006, 40(17), 3151–3166 CrossRef CAS PubMed.
- T. A. Larsen, M. E. Riechmann and K. M. Udert, State of the art of urine treatment technologies: A critical review, Water Res.: X Search PubMed submitted.
- A. Fumasoli, B. Etter, B. Sterkele, E. Morgenroth and K. M. Udert, Operating a pilot-scale nitrification/distillation plant for complete nutrient recovery from urine, Water Sci. Technol., 2016, 73(1), 215–222 CrossRef CAS PubMed.
- B. D. Özel Duygan, K. M. Udert, A. Remmele and C. S. McArdell, Removal of pharmaceuticals from human urine during storage, aerobic biological treatment, and activated carbon adsorption to produce a safe fertilizer, Resour., Conserv. Recycl., 2021, 166, 105341 CrossRef.
- P. Simha, C. Karlsson, E. L. Viskari, R. Malila and B. Vinnerås, Field Testing a Pilot-Scale System for Alkaline Dehydration of Source-Separated Human Urine: A Case Study in Finland, Front. Environ. Sci. Eng., 2020, 8, 570637 CrossRef.
- P. Simha, M. A. Barton, L. F. Perez-Mercado, J. R. McConville, C. Lalander, M. E. Magri, S. Dutta, H. Kabir, A. Selvakumar, X. Zhou, T. Martin, T. Kizos, R. Kataki, Y. Gerchman, R. Herscu-Kluska, D. Alrousan, E. G. Goh, D. Elenciuc, A. Głowacka, L. Korculanin, R. V. Tzeng, S. S. Ray, C. Niwagaba, C. Prouty, J. R. Mihelcic and B. Vinnerås, Willingness among food consumers to recycle human urine as crop fertiliser: Evidence from a multinational survey, Sci. Total Environ., 2021, 765, 144438 CrossRef CAS PubMed.
- C. Pahl-Wostl, A. Schönborn, N. Willi, J. Muncke and T. A. Larsen, Investigating consumer attitudes towards the new technology of urine separation, Water Sci. Technol., 2003, 48(1), 57–65 CrossRef CAS.
-
L. Keogh, Flows of Science, Source Separation Technology at the Swiss Federal Institute of Aquatic Science and Technology, 2018. Report available from Eawag, Dübendorf, Switzerland, DOI:10.5281/zenodo.1283188.
- J. R. McConville, E. Kvarnström, H. Jönsson, E. Kärrman and M. Johansson, Source separation: Challenges & opportunities for transition in the swedish wastewater sector, Resour., Conserv. Recycl., 2017, 120, 144–156 CrossRef.
- J. R. McConville, E. Kvarnström, H. Jönsson, E. Kärrman and M. Johansson, Is the Swedish wastewater sector ready for a transition to source separation?, Desalin. Water Treat., 2017, 91, 320–328 CrossRef.
- F. Geels, Co-evolution of technology and society: The transition in water supply and personal hygiene in the Netherlands (1850-1930) - A case study in multi-level perspective, Technol. Soc., 2005, 27(3), 363–397 CrossRef.
- R. Kemp, J. Schot and R. Hoogma, Regime Shifts to Sustainability Through Processes of Niche Formation: The Approach of Strategic Niche Management, Technol. Anal. Strateg. Manag., 1998, 10(2), 175–195 CrossRef.
- D. Loorbach, N. Frantzeskaki and F. Avelino, Sustainability Transitions Research: Transforming Science and Practice for Societal Change, Annu. Rev. Environ. Resour., 2016, 42(1), 599–626 CrossRef.
- E.-L. Viskari, S. Lehtoranta and R. Malila, Urine: The Potential, Value Chain and Its Sustainable Management, Sanitation Value Chain, 2021, 5(1), 10–12 Search PubMed.
- F. W. Geels, The hygienic transition from cesspools to sewer systems (1840-1930): The dynamics of regime transformation, Res. Policy, 2006, 35(7), 1069–1082 CrossRef.
- J. Markard, R. Raven and B. Truffer, Sustainability transitions: An emerging field of research and its prospects, Res. Policy, 2012, 41(6), 955–967 CrossRef.
- S. Harris-Lovett, C. Binz, D. Sedlak, M. Kiparsky and B. Truffer, Beyond User Acceptance: A Legitimacy Framework for Potable Water Reuse in California, Environ. Sci. Technol., 2015, 49(13), 7552–7561 CrossRef CAS PubMed.
- A. Bergek, S. Jacobsson, B. Carlsson, S. Lindmark and A. Rickne, Analyzing the functional dynamics of technological innovation systems: A scheme of analysis, Res. Policy, 2008, 37(3), 407–429 CrossRef.
- M. Hekkert, R. Suurs, S. Negro, S. Kuhlmann and R. Smits, Functions of innovation systems: A new approach for analysing technological change, Technol. Forecast. Soc. Change, 2007, 74(4), 413–432 CrossRef.
- C. Binz, B. Truffer and L. Coenen, Path creation as a process of resource alignment and anchoring – Industry formation for on-site water recycling in Beijing, Econ. Geogr., 2016, 92(2), 172–200 CrossRef.
- K. M. Weber and H. Rohracher, Legitimizing research, technology and innovation policies for transformative change: Combining insights from innovation systems and multi-level perspective in a comprehensive ‘failures’ framework, Res. Policy, 2012, 41(6), 1037–1047 CrossRef.
-
B. Vinnerås and H. Jönsson, The Swedish experience with source separation, in Source Separation and Decentralization for Wastewater Management, ed. T. A. Larsen, K. M. Udert and J. Lienert, IWA Publishing, London, UK, 2013, pp. 415–422 Search PubMed.
- H. Jönsson, T. A. Stenström, J. Svensson and A. Sundin, Source separated urine-nutrient and heavy metal content, water saving and faecal contamination, Water Sci. Technol., 1997, 35(9), 145–152 CrossRef.
- H. Kirchmann and S. Pettersson, Human urine - Chemical composition and fertilizer use efficiency, Fert. Res., 1994, 40(2), 149–154 CrossRef.
-
M. Ek, C. Junestedt, C. Larsson, M. Olshammar and M. Ericsson, Teknikenkät - enskilda avlopp 2009, SMED Report nr 44, Sveriges Meteorologiska och Hydrologiska Institut, 2011. Assessed 07.05.2021, available at: http://www.smed.se/vatten/1155 Search PubMed.
-
E. Kvarnström, K. Emilsson, A. Richert Stintzing, M. Johansson, H. Jönsson, E. af Petersens, C. Schönning, J. Christensen, D. Hellström, L. Qvarnström, P. Ridderstolpe and J. O. Drangert, Urine diversion: One step towards sustainable sanitation, EcoSanRes Publications Series, Report, 2006-1, 2006, [accessed 30.11.2020] available at: http://www.ecosanres.org/news-publications.htm.
-
Dubbletten, http://www.dubbletten.nu/, [accessed 30.11.2020].
-
Wostman Ecology, https://www.wostman.se, [accessed 30.11.2020].
- J. Hanæus, D. Hellström and E. Johansson, A study of a urine separation system in an ecological village in northern Sweden, Water Sci. Technol., 1997, 35(9), 153–160 CrossRef.
- H. Jönsson, B. Vinnerås, C. Höglund and T. A. Stenström, Source separation of urine, Wasser und Boden, 1999, 51(11), 21–25 Search PubMed.
- C. Höglund, T. A. Stenström, H. Jönsson and A. Sundin, Evaluation of faecal contamination and microbial die-off in urine separating sewage systems, Water Sci. Technol., 1998, 38(6), 17–25 CrossRef.
-
Novaquatis, https://www.novaquatis.eawag.ch/index_EN.html, [accessed 30.11.2020].
- S. Blume and M. Winker, Three years of operation of the urine diversion system at GTZ headquarters in Germany: User opinions and maintenance challenges, Water Sci. Technol., 2011, 64(3), 579–586 CrossRef CAS PubMed.
- M. Starkl, M. Ornetzeder, E. Binner, P. Holubar, M. Pollak, M. Dorninger, F. Mascher, M. Fuerhacker and R. Haberl, An integrated assessment of options for rural wastewater management in Austria, Water Sci. Technol., 2007, 56(5), 105–113 CrossRef CAS PubMed.
- E. Medilanski, L. Chuan, H. Mosler, R. Schertenleib and T. A. Larsen, Wastewater management in Kunming, China: a stakeholder perspective on measures at the source, Environ. Urban., 2006, 18, 353–368 CrossRef.
- D.-B. Huang, H.-P. Bader, R. Scheidegger, R. Schertenleib and W. Gujer, Confronting limitations: New solutions required for urban water management in Kunming City, J. Environ. Manage., 2007, 84(1), 49–61 CrossRef CAS PubMed.
- B. Etter, E. Tilley, R. Khadka and K. M. Udert, Low-cost struvite production using source-separated urine in Nepal, Water Res., 2011, 45(2), 852–862 CrossRef CAS PubMed.
-
A. Rosemarin, J. McConville, A. Flores and Z. Qiang, The challenges of urban ecological sanitation: lessons from the Erdos eco-town project, Stylus Publishing, 2012 Search PubMed.
-
Eawag, NEST: developing the toilet of the future, https://www.eawag.ch/en/news-agenda/news-portal/news-detail/ein-nest-fuer-die-toilette-der-zukunft/?tx_news_pi1[controller]=News&tx_news_pi1[action]=detail&cHash=99e5297e7f89b52facd1e112dd2eaaa0, [accessed 30.11.2020].
- M. O'Keefe, C. Lüthi, I. K. Tumwebaze and R. Tobias, Opportunities and limits to market-driven sanitation services: evidence from urban informal settlements in East Africa, Environ. Urban., 2015, 27(2), 421–440 CrossRef.
- R. Harder, R. Wielemaker, T. A. Larsen, G. Zeeman and G. Öberg, Recycling nutrients contained in human excreta to agriculture: Pathways, processes, and products, Crit. Rev. Environ. Sci. Technol., 2019, 49(8), 695–743 CrossRef.
-
Ocapi, https://www.leesu.fr/ocapi/presentation/ocapi-program/, [accessed 30.11.2020].
- F. Esculier, J. Le Noë, S. Barles, G. Billen, B. Créno, J. Garnier, J. Lesavre, L. Petit and J. P. Tabuchi, The biogeochemical imprint of human metabolism in Paris Megacity: A regionalized analysis of a water-agro-food system, J. Hydrol., 2019, 573, 1028–1045 CrossRef CAS.
- W. A. Tarpeh, I. Wald, M. O. Omollo, T. Egan and K. L. Nelson, Evaluating ion exchange for nitrogen recovery from source-separated urine in Nairobi, Kenya, Dev. Eng., 2018, 3, 188–195 CrossRef.
- S. P. Wei, F. van Rossum, G. J. van de Pol and M.-K. H. Winkler, Recovery of phosphorus and nitrogen from human urine by struvite precipitation, air stripping and acid scrubbing: A pilot study, Chemosphere, 2018, 212, 1030–1037 CrossRef CAS PubMed.
- P. Ledezma, P. Kuntke, C. J. Buisman, J. Keller and S. Freguia, Source-separated urine opens golden opportunities for microbial electrochemical technologies, Trends Biotechnol., 2015, 33(4), 214–220 CrossRef CAS PubMed.
-
A. Noe-Hays, K. Nace, N. Patel, R. Lahr, H. Goetsch, R. Mullen, N. Love, D. Aga, C. Bott, B. Foxman, J. Jimenez, T. Luo, K. Ramadugu and K. Wigginton, Urine diversion for nutrient recovery and micropollutant management: Results from a regional urine recycling program, 88th Annual Water Environment Federation Technical Exhibition and Conference, WEFTEC 2015, 2015, vol. 1, pp. 3993–4002, DOI:10.2175/193864715819538921.
- A. S. Cohen, N. G. Love and J. Árvai, Communicating the risks and benefits of human urine-derived fertilizer, Sustainability, 2020, 12(23), 1–14 Search PubMed.
- K. M. Udert, C. A. Buckley, M. Wähter, C. S. McArdell, T. Kohn, L. Strande, H. Zöllig, A. Fumasoli, A. Oberson and B. Etter, Technologies for the treatment of source-separated urine in the eThekwini Municipality, Water SA, 2015, 41(2), 212–221 CrossRef CAS.
-
Z. Fishman, Y. M. T. Jung, P. Pironi, M. Krajcovic, S. Melamed, M. Webb, J. L. Torero, J. I. Gerhard, L. L. Diosady, Y. Lawryshyn, E. Edwards and M. T. Kortschot, A unit operations approach for rapid disinfection of human waste based on drying/smoldering of solid and sand filtration/UV disinfection of liquid waste, presented at the International faecal sludge management conference, Durban, South Africa, 2012.
- T. A. Larsen, H. Gebauer, H. Gründl, R. Künzle, C. Lüthi, U. Messmer, E. Morgenroth, C. B. Niwagaba and B. Ranner, Blue diversion: A new approach to sanitation in informal settlements, J. Water, Sanit. Hyg. Dev., 2015, 5(1), 64–71 CrossRef.
- The Bill & Melinda Gates Foundation, Grant to support development of an improved source separating dry toilet user interface for sitting and squatting toilets for family use in developing countries, https://www.gatesfoundation.org/How-We-Work/Quick-Links/Grants-Database/Grants/2017/04/OPP1149059, [accessed 30.11.2020].
- J. Gundlach, M. Bryla, T. A. Larsen, L. Kristoferitsch, H. Gründl and M. Holzner, Novel NoMix toilet concept for efficient separation of urine and feces and its design optimization using computational fluid mechanics, J. Build. Eng., 2021, 33, 101500 CrossRef.
-
Vuna, https://vuna.ch/en/, [accessed 30.11.2020].
- H. Gebauer, M. Haldimann and C. J. Saul, Business model innovations for overcoming barriers in the base-of-the-pyramid market, Ind. Innov., 2017, 24(5), 543–568 CrossRef.
- N. Mkhize, M. Taylor, K. M. Udert, T. G. Gounden and C. A. Buckley, Urine diversion dry toilets in eThekwini Municipality, South Africa: acceptance, use and maintenance through users' eyes, J. Water, Sanit. Hyg. Dev., 2017, 7(1), 111–120 CrossRef PubMed.
-
S. Gao and J. Zhang, Technical Options for Source-Separated Collection of Municipal Wastewater, Water Infrastructure for Sustainable Communities, 2010, p. 253 Search PubMed.
-
Kompotoi, Report on SRF 1 about compost toilets in apartment buildings, https://www.kompotoi.ch/2018/07/01/bericht-auf-srf-1-%C3%BCber-komposttoiletten-im-mehrfamilienhaus/, [accessed 30.11.2020].
-
M. Legrand, A. Jovéniaux, A. Arbarotti, B. de Gouvello, F. Esculier and J.-P. Tabuchi, The Emergence of Systems for the Source Separation and Valorization of Human Waste in Greater Paris: From Necessity to Implementation. Assessed 07.05.2021. Available at: https://www.leesu.fr/ocapi/wp-content/uploads/2020/12/Legrand-al_EauMega-<?pdb_no 2020?>2020<?pdb<?db_id PDB?> END?>_VF.pdf Search PubMed.
-
Vuna, Aurin recycled fertiliser, https://vuna.ch/en/aurin-recycling-dunger/, [accessed 30.11.2020].
-
The World Bank, World Bank Group and Bill & Melinda Gates Foundation Commit to Innovation to Speed Up Sanitation for All, [accessed 10.03.2021], available at: https://www.worldbank.org/en/news/press-release/2018/11/06/world-bank-group-and-bill-melinda-gates-foundation-commit-to-innovation-to-speed-up-sanitation-for-all.
- A. Schrecongost, D. Pedi, J. W. Rosenboom, R. Shrestha and R. Ban, Citywide inclusive sanitation: a public service approach for reaching the urban sanitation SDGs, Front. Environ. Sci. Eng., 2020, 8(19), 1–8 Search PubMed.
-
Agence de l'eau Seine-Normandie, 11e Programme d'intervention de l'agence de l'eau Seine-Normandie 2019–2024 (in French), Comité de bassin et le conseil d'administration, 2018. Assessed 07.05.2021, available at: http://www.eau-seine-normandie.fr/programme_eau_climat_seine_normandie.
-
LAUFEN, https://www.laufen.com/news-stories/save-smart-sanitation-2, [accessed 30.11.2020].
-
S.-L. Wee, In China, Bill Gates Encourages the World to Build a Better Toilet, The New York Times, 2018, Newspaper article (6.11.2018) available at: https://www.nytimes.com/2018/11/06/business/bill-gates-reinvented-toilet.html, accessed on 04.03.2021 Search PubMed.
-
P. K. El Hadj, Comment l'urine humaine pourrait dynamiser l'agriculture du futur, Le Monde, 2020, Newspaper article (6.7.2020) available at: https://www.lemonde.fr/videos/article/2020/07/06/comment-l-urine-humaine-pourrait-dynamiser-l-agriculture-du-futur_6045316_1669088.html, Assessed 12.03.2021 Search PubMed.
-
K. Lee, Pee fanatics want to feed the world with your liquid gold, Wired, 2020, Newspaper Article (07.10.2020) available at: https://www.wired.co.uk/article/urine-fertiliser-growing-plants, accessed on 09.03.2021 Search PubMed.
-
Wallpaper, ‘Save’ separation toilet by Laufen wins Wallpaper* Design Award for Life-Enhancer of the Year, https://www.wallpaper.com/lifestyle/design-awards-2020-life-enhance-of-the-year-shortlist, [accessed 16.03.2021].
-
L. I. P. Region, Note rapide Environnement, n° 858. Valoriser l'azote et le phosphore des urines pour une meilleure sécurité écologique et alimentaire (in French), https://www.institutparisregion.fr/nos-travaux/publications/valoriser-lazote-et-le-phosphore-des-urines-pour-une-meilleure-securite-ecologi.html, [accessed 23.10.2020].
-
BBC Newsbeat, There's a new beer made with human urine from a Danish music festival http://www.bbc.co.uk/newsbeat/article/39817245/theres-a-new-beer-made-with-human-urine-from-a-danish-music-festival, [accessed 23.10.2020].
-
J. Atlee, A. Noe-Hays, K. Nace, T. Schreiber, A. Davis, C. Lally and S. Dotson, Guide to Starting a Community-scale Urine Diversion Program, Rich Earth Institute Brattleboro, Brattleboro, VT, 1.1 edn, 2019. Available at: http://richearthinstitute.org/wp-content/uploads/2019/05/RichEarth_Urine_Diversion_Guide_01_1.pdf Search PubMed.
-
XXII Triennale Milano, 2019, Broken Nature, http://www.brokennature.org/exhibition/international-participations/, [accessed 30.11.2020] Search PubMed.
-
Kretsloppsteknik, Sanitation360 wins the Skapapriset 2020 for Gotland County, https://blogg.slu.se/kretsloppsteknik/2020/06/25/sanitation360-wins-the-skapapriset-in-gotland-county-2020/, [accessed 23.10.2020].
- H. Gebauer, B. Truffer, C. Binz and E. Störmer, Capability perspective on business network formation: Empirical evidence from the wastewater treatment industry, European Business Review, 2012, 24(2), 169–190 CrossRef.
-
B. Truffer, C. Binz, H. Gebauer and E. Störmer, Market success of on-site treatment: a systemic innovation problem, in Wastewater Treatment: Source Separation and Decentralisation, ed. T. Larsen, K. Udert and J. Lienert, IWA Publishing, London, 2012, pp. 209–223 Search PubMed.
- ISO 30500, https://www.iso.org/standard/72523.html, [accessed 27.10.2020].
-
J. Miörner and C. Binz, Toward a multi-scalar
perspective of transition trajectories, Papers in Innovation Studies, 2020, vol. 2020/10.
-
F. Brun, Freins et leviers a l'emploi de fertilisants à base d'urine humaine en agriculture en Ile-de-France [Incentives and obstacles for the use of human urine based fertilizers in agriculture in Île-de-France] (in French), Ecole Nationale des Ponts et Chaussees, LEESU, 2018. Assessed 07.05.2021. Available at: https://hal.archives-ouvertes.fr/hal-01914242v2/document.
- T. M. P. Martin, F. Esculier, F. Levavasseur and S. Houot, Human urine-based fertilizers: A review, Crit. Rev. Environ. Sci. Technol., 2020 DOI:10.1080/10643389.2020.1838214.
- A. Segrè Cohen, N. G. Love, K. K. Nace and J. Árvai, Consumers' Acceptance of Agricultural Fertilizers Derived from Diverted and Recycled Human Urine, Environ. Sci. Technol., 2020, 54(8), 5297–5305 CrossRef PubMed.
- J. Jimenez, C. Bott, N. Love and J. Bratby, Source Separation of Urine as an Alternative Solution to Nutrient Management in Biological Nutrient Removal Treatment Plants, Water Environ. Res., 2015, 87(12), 2120–2129 CrossRef CAS.
- P. Passy, R. Le Gendre, J. Garnier, P. Cugier, J. Callens, F. Paris, G. Billen, P. Riou and E. Romero, Eutrophication modelling chain for improved management strategies to prevent algal blooms in the Bay of Seine, Mar. Ecol.: Prog. Ser., 2016, 543, 107–125 CrossRef CAS.
-
A. Caby, Quel intérêt et quelle opportunité de mettre en place une collecte sélective des urines en milieu urbain dense? Etude sur le territoire du SIAAP, Ecole des Ponts ParisTech and AgroParisTech-Engref, Paris, 2013. Assessed 07.05.2021. Available at: https://www.leesu.fr/ocapi/wp-content/uploads/2019/01/CABY_Collecte-s%C3%A9lective-urines-SIAAP_2013.pdf.
Footnote |
† Electronic supplementary information (ESI) available. See DOI: 10.1039/d0ew01064b |
|
This journal is © The Royal Society of Chemistry 2021 |