DOI:
10.1039/D0GC01135E
(Paper)
Green Chem., 2020,
22, 3539-3545
Photochemical synthesis of acetals utilizing Schreiner's thiourea as the catalyst†
Received
30th March 2020
, Accepted 6th May 2020
First published on 6th May 2020
Abstract
Acetalization of aldehydes is an area of great importance in Organic Chemistry for both synthetic and biological puproses. Herein, we report a mild, inexpensive and green photochemical protocol, where Schreiner's thiourea (N,N′-bis[3,5-bis(trifluoromethyl)-phenyl]-thiourea) is utilized as the catalyst and cheap household lamps as the light source. A variety of aromatic and aliphatic aldehydes were converted into acetals in good to high yields (23 examples, 36–96% yield) and an example of the synthesis of a cyclic acetal is provided. The reaction mechanism was also studied.
Introduction
Compounds bearing the carbonyl moiety, such as aldehydes appear to have great synthetic interest and are classified among the most widely used functional groups in Organic Synthesis.1 In some cases, its high reactivity towards nucleophilic, metal–hydride, alkyl and aryl reagents, constitutes an inhibiting factor that has to be confronted, in order to achieve the desired synthetic transformations. The protection of the carbonyl moieties into acetals with alcohols or diols is a widely employed method in pharmaceutical industry, such as drug design and also in other sectors.2 The importance of acetals as carbonyl protecting groups lies in their stability in neutral and strongly basic conditions, which allows a wide spectrum of reactions to occur in their presence. Acetals, apart from their role as a protection group, can also behave as excellent intermediates in many synthetic transformations.3 Expanding their use, acetals are also considered as flavoring additives and aroma enhancers in cosmetic and food products4 or as anti-freezing additives in biodiesel fuels.5 Since aldehydes are one of the most frequently modifiable functional groups, a great variety of approaches have been reported for their conversion into acetals. The currently used methodologies include the use of strong mineral acids, such as HCl,6 solid acids,7 acidic polymers,8 metal catalysts,9 Lewis acids or organic acids10,11 (Scheme 1A). The reported synthetic strategies, where either the use of metal catalysts or stoichiometric amounts of acids are employed, raise many disadvantages and potential environmental problems, such as corrosive conditions, long reaction times and usually poor selectivity.12 In an effort to address these problems and comply with the principles of green and sustainable chemistry,13 alternative methods for the synthesis of acetals have been developed. Acetalization of carbonyl compounds can be also achieved utilizing basic14 or other non-acidic conditions employing hydroxylamine,15 iodine,16 trialkyl orthoformates,17 halohydrins18 or even catalytic amount of anionic metal–oxides as polyoxometalates (POMs)19 (Scheme 1B). Recently, a totally alternative approach for the synthesis of acetals, in line with the principles of photochemistry20 was introduced in literature21 (Scheme 1C). The authors utilized Eosyn Y as the photocatalyst and green LED irradiation and succeeded in the acetalization of aldehydes in excellent yields. However, they did not clarify whether the acidic groups that Eosyn Y bear or the heat source that green LED emit, can be complementary or even the main factor in the completion of the reaction.
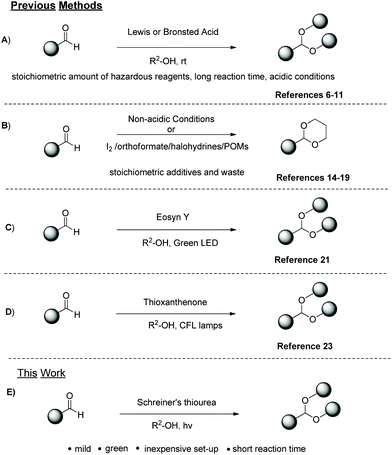 |
| Scheme 1 Common synthetic pathways for the acetalization of aldehydes. | |
Being active for many years in the field of Photochemistry,22 our laboratory had successfully synthesized many different categories of compounds, utilizing a great variety of photocatalysts and studying simultaneously the mechanism of the reaction. Specifically, in 2019, our research group proposed a photochemical synthetic pathway for the acetalization of aldehydes with alcohols or diols, utilizing thioxanthenone as the catalyst and common household lamps as the light source.23 The synthesis of both aromatic and aliphatic acetals was achieved in satisfactory to high yields via this protocol (Scheme 1D). The only drawback of the method appeared to be the purification step, since thioxanthenone (the catalyst) had a similar Rf with the product and a copious column chromatography was necessary. Every other attempt to purify the product by alternative means failed. Herein, exploiting the existing knowledge, we present a novel, mild, green, and fast photochemical synthesis of acetals from aldehydes, utilizing Schreiner's thiourea as the catalyst and cheap, commercially-available household lamps as the irradiation source (Scheme 1E). The driving idea in this work was initially the use of low-cost and environmentally friendly reagents, avoiding the production of hazardous by-products and time-consuming reactions. Working on this logic, we designed a photochemical protocol, where Schreiner's thiourea, an organic compound with a variety of properties (for example, hydrogen bonding with various classes of substrates),24 is used as the catalyst. Its major feature is its ability to activate substrates via hydrogen bonding, a field that presents increasing interest in organic chemistry over the last two decades. Moreover, the cheap household lamps set-up or sunlight, which can be also employed, guarantee that no special apparatus or inert atmosphere are required. The reaction time varies from 3 to 18 h, depending on the substrate, and the product can be easily obtained via aqueous base extraction or evaporation, rendering this method intertwining with the principles of green chemistry.
Results and discussion
Bearing in mind that thioureas via their hydrogen bonding activation can behave similarly to Lewis acids,24b we began our investigations with the evaluation of (thio)urea derivatives as the catalyst (Table 1).25 A range of urea and thiourea catalysts were investigated, concluding that N,N′-bis[3,5-bis(trifluoromethyl)phenyl]-thiourea (3a) afforded higher yields versus simple urea and thiourea derivatives (Table 1, entry 1 vs. entries 5–7), confirming our initial hypothesis that the presence of the trifluoromethyl groups on the thiourea play a crucial role and ortho-CH is also important for the interactions in the catalyst–substrate complex. It has to be pinpointed that the product of the reaction is pure enough and purification can be achieved by just simple evaporation of the excess of methanol and aqueous base wash, without the need for column chromatography.25 Decreasing the catalyst loading to 10 mol%, the yield of the desired acetal 2a dropped to 59% (Table 1, entry 2). When the reaction was kept in dark, acetal 2a was not formed (hydrogen bonding activation by itself is not enough for the catalysis, Table 1, entry 3). When the reaction was performed at 50 °C, the desired acetal was formed in 13% yield, verifying that light irradiation is the main factor for the reaction outcome and the thermal pathway is minor (Table 1, entry 4). Furthermore, the irradiated reaction mixture in the absence of 3a led to 9% yield.25
Table 1 Photochemical synthesis of acetal 2a from aldehyde 1a
a
In an effort to further reduce the amount of the alcohol employed, we studied the behavior of the reaction in a 1
:
1 mixture of methanol and other solvents (Table 2, entries 1–7). Unfortunately, only in few occasions the yield of the desired product was good (Table 2, entries 2, 4 and 5), but still lower than when methanol was used alone. Attempts to alter the light source (blue and green LEDs) led to lower yields (Table 2, entries 8 and 9). Finally, the reaction time could be decreased to 3 h (Table 2, entry 10). Sunlight irradiation could efficiently replace household lamp irradiation.
Table 2 Methanol-solvent screening for the synthesis of acetal 2a from aldehyde 1a
a
Once the optimum reaction conditions were found, we diverted our attention into exploring the substrate scope (Schemes 2 and 3). Utilizing 3-phenyl-propanal (1a) as the starting material, a variety of alcohols were tested, providing the desired acetals in good to excellent yields (Scheme 2). Primary alcohols, alcohols bearing functional groups, such as –Cl or –CF3 groups, or diols were utilized successfully, while secondary alcohols led to moderate yields, probably due to steric reasons, affording acetals 2a–2l. Time reaction varied from 3 to 18 h, depending on substrate.
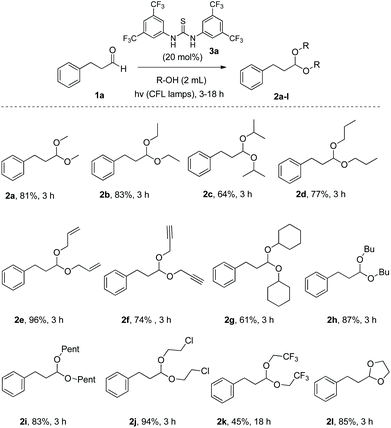 |
| Scheme 2 Substrate scope – alcohols. | |
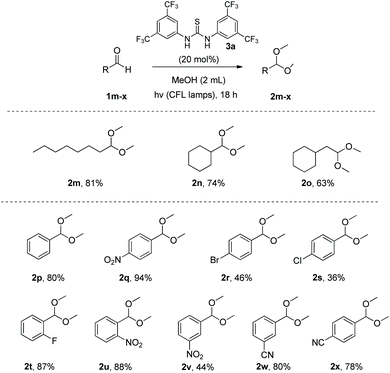 |
| Scheme 3 Substrate scope – aldehydes. | |
Subsequently, we explored the scope of the aldehydes with methanol (Scheme 3). Initially, other aliphatic aldehydes were tested. The desired products 2m–o were obtained in good to excellent yields, even in the case of α,α-disubstituted aldehydes (like 1n). In an effort to expand our method's application, we applied this method in a variety of aromatic aldehydes, bearing many different functional groups (2p–x) (Scheme 3). Aromatic substitution did not pose any problem for this methodology, since para-, meta- and ortho-substituted aromatic aldehydes afforded yields that varied from 36% to 94%. The crucial point is that substrates, that were problematic in previous studies (like products 2q and 2u–x) that bear groups, like –CN or –NO2, behaved excellently, providing a strong generality to our method.
Next, the reaction mechanism was studied. For better understanding of the hydrogen bonding activation that 3a is providing, and having literature as a strong inspiration,26,27 we performed 1H-NMR and 19F-NMR mechanistic experiments (Fig. 1).25 Initially, the 1H-NMR (in CDCl3) of Schreiner's thiourea 3a was recorded (Fig. 1A). Two sets of aromatic signals were recorded at 7.92 ppm and 7.81 ppm, while the NH appeared at 7.29 ppm. 3-Phenyl-propanal's (1a) 1H-NMR spectrum is presented in Fig. 1B. The addition of 3-phenyl-propanal (1a) to a solution of thiourea 3a in CDCl3, led to remarkable changes in 1H-NMR chemical shifts of the catalyst, as expected (Fig. 1C). The peak at 7.92 ppm shifted downfield at 7.96 ppm, the peak 7.81 ppm shifted upfield at 7.78 ppm, while the NH protons shifted downfield from 7.29 ppm to 8.31 ppm. These results confirm the presence of hydrogen bonds between the substrate and the thiourea, supporting the formation of an aldehyde-catalyst complex. After irradiation, similar observations can be drawn (Fig. 1D).
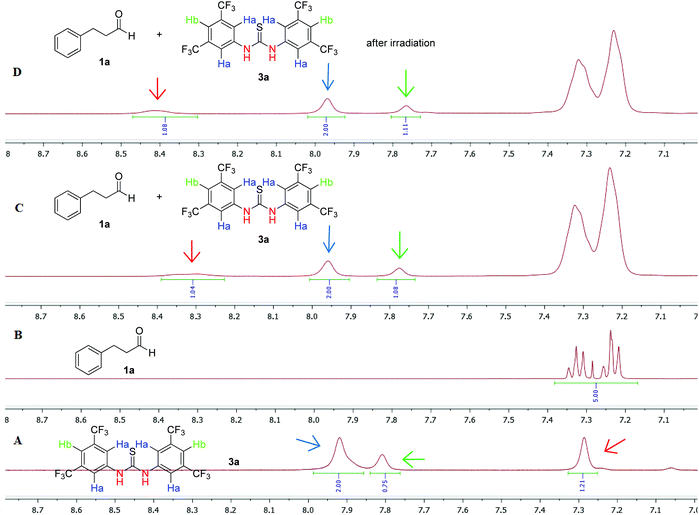 |
| Fig. 1 Zoom in (8.70–7.00 ppm) of 1H-NMR (in CDCl3) of (A) Schreiner's thiourea 3a, (B) 3-phenyl propanal (1a), (C) reaction mixture before irradiation and (D) reaction mixture after irradiation. | |
Furthermore, the calculation of the quantum yield (Φ) of the reaction was performed. According to Yoon and Cismenia,28 the value of the quantum yield is critical for the reaction mechanism. The quantum yield (Φ) of the photochemical reaction was measured: [Φ = 46 (Φ > 1)], indicating that a radical propagation mechanism is taking place.
Finally, UV-Vis absorbance studies were carried out (Fig. 2).25 In literature, the presence of an electron donor–acceptor (EDA) complex has been postulated as key mechanistic point in selected photochemical reactions.29,30 The formation of an EDA complex is sometimes identified by the bathochromic shift in the UV-Vis absorbance spectrum, after mixing of the two reagents. Based on the UV-Vis absorbance spectra presented in Fig. 2, the absorption of Schreiner's thiourea 3a, after the addition of aldehyde 1a did not show any change (Fig. 2). Thus, an EDA-complex is not formed. The reaction was also performed open air and under argon atmosphere, without showing any significant differentiation, reinforcing the fact that oxygen does not participate in the reaction mechanism.
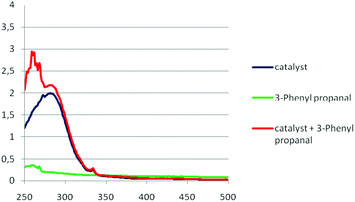 |
| Fig. 2 UV-Vis absorbance of Schreiner's thiourea 3a (6 × 10−5 M) in MeOH, 3-phenyl-propanal (1a) (1 × 10−3 M) in MeOH, and the reaction mixture (3-phenyl-propanal 1 × 10−3 M and Schreiner's thiourea 6 × 10−5 M) in MeOH. | |
Collecting all these data, a possible mechanism can be proposed (Scheme 4). Initially, Schreiner's thiourea 3a interacts with the aldehyde and via hydrogen bonding leads to aldehyde-catalyst complex 4. Upon irradiation, 4 is excited to 5. Herein, two possible pathways can be envisaged. Following literature precedent,24e,31 where a phenol or a thiourea can behave as a photoacid, complex 5 can lead to an in situ-generated acidic species, which leads to intermediates 6 and 7 (pathway A). Protonated intermediate 6 is intercepted by methanol, which after proton transfer, loss of water and another methanol addition leads to product 2 and regenerates the photogenerated acid. Alternatively (pathway B), one can assume a direct nucleophilic addition of methanol to 5, leading to 8, with or without a “loose” interaction with the thiourea moiety. Another molecule of aldehyde is activated by abstracting the proton from 8 leading to 6 and 9, which afford the final product and ensure the propagation similarly to pathway A. A photo induced excited state proton transfer coupled electron transfer mechanism can not been completely excluded at the moment.
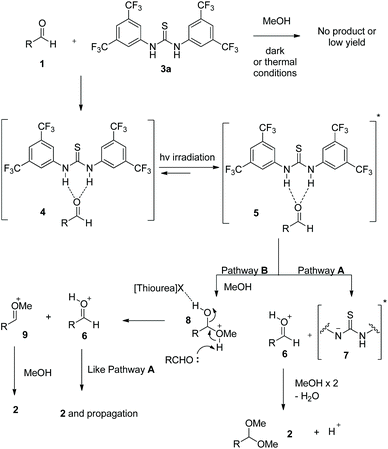 |
| Scheme 4 Proposed reaction mechanism. | |
Conclusions
In conclusion, a green, inexpensive, mild, environmentally–friendly and fast photochemical synthetic protocol for the synthesis of acetals from aldehydes and alcohols was developed. Utilizing commercially available household lamps as the irradiation source and a simple catalyst, Schreiner's thiourea 3a, which acts like hydrogen-bond donor, a variety of aromatic and aliphatic acetals were isolated in good to excellent yields, either by solvent evaporation and aqueous basic extractions or column chromatography in some cases. Based on extensive mechanistic investigation, a proposed mechanistic pathway is presented.
Experimental
General procedure for the synthesis of acetals
In a glass vial with a screw cap containing N,N′-bis[3,5-bis(trifluoromethyl) phenyl]-thiourea (3a) (Schreiner's thiourea) (50 mg, 0.10 mmol) in alcohol (2 mL), aldehyde (0.50 mmol) was added. The vial was sealed with a screw cap and left stirring under household bulb irradiation (2 × 80 W household lamps) for 3–18 h. The desired product was isolated after base aqueous wash (when full consumption of aldehyde took place) or purification by column chromatography.
Conflicts of interest
There are no conflicts to declare.
Acknowledgements
The authors gratefully acknowledge the Hellenic Foundation for Research and Innovation (HFRI) for financial support through a grant, which is financed by 1st Call for H.F.R.I. Research Projects to Support Faculty Members & Researchers and the procurement of high-cost research equipment grant (grant number 655). N. S. would like to thank the Hellenic Foundation for Research and Innovation (HFRI) for financial support through a doctoral by the Hellenic Foundation for Research and Innovation (HFRI) under PhD Fellowship grant (Fellowship Number: 721). N. F. N. would like to thank the State Scholarships Foundation (IKY) for financial support through a doctoral fellowship, which is co-financed by Greece and the European Union (European Social Fund-ESF) through the Operational Programme “Human Resources Development, Education and Lifelong Learning” in the context of the project “Strengthening Human Resources Research Potential via Doctorate Research” (MIS-5000432), implemented by the State Scholarships Foundation (IKY)”. The authors would like to thank Costas Constantinou and Marios Constantinou (DIMCO) for access to the equipment for the measurement of the emission spectrum of the lamps. Also, COST Action C–H Activation in Organic Synthesis (CHAOS) CA15106 is acknowledged for helpful discussions.
Notes and references
-
(a) P. S. Baran and J. M. Richter, J. Am. Chem. Soc., 2004, 126, 7450–7451 CrossRef CAS PubMed
;
(b) S. Krautwald, D. Sarlah, M. A. Schafroth and E. M. Carreira, Science, 2013, 340, 1065–1068 CrossRef CAS PubMed
;
(c) T. Sandmeier, S. Krautwald, H. F. Zipfel and E. M. Carreira, Angew. Chem., Int. Ed., 2015, 54, 14363–14367 CrossRef CAS PubMed
;
(d) L. R. Malins, J. N. deGruyter, K. J. Robbins, P. M. Scola, M. D. Eastgate, M. R. Ghadiri and P. S. Baran, J. Am. Chem. Soc., 2017, 139, 5233–5241 CrossRef CAS PubMed
.
-
(a) D. M. Clode, Chem. Rev., 1979, 79, 491–513 CrossRef CAS
;
(b)
K. Bauer, D. Garbe and H. Surburg, Common Fragrances and Flavor Materials: Preparation and Uses, 4th edn, 2001, pp. 103–110 CrossRef
;
(c)
P. G. M. Wuts and T. W. Greene, Greene's Protective Groups in Organic Synthesis, 4th edn, 2007, pp. 424–428 Search PubMed
.
- For selected examples, see:
(a) P. A. Bartlett, W. S. Johnson and J. D. Elliott, J. Am. Chem. Soc., 1983, 105, 2088–2089 CrossRef CAS
;
(b) A. Mori and H. Yamamoto, J. Org. Chem., 1985, 50, 5444–5446 CrossRef CAS
;
(c) T. Xu, Z. Yu and L. Wang, Org. Lett., 2009, 11, 2113–2116 CrossRef CAS PubMed
;
(d) Z.-B. Zhu, Y. Wei and M. Shi, Chem. – Eur. J., 2009, 15, 7543–7548 CrossRef CAS PubMed
;
(e) I. Suzuki, M. Yasuda and A. Baba, Chem. Commun., 2013, 49, 11620–11622 RSC
;
(f) Q. Yang, T. Xu and Z. Yu, Org. Lett., 2014, 16, 6310–6313 CrossRef CAS PubMed
.
-
H. Maarse, Volatile Compounds I Foods and Beverages, Marcel Dekker, Inc., New York, 1991 Search PubMed
.
- P. H. R. Silva, V. L. C. Gonçalves and C. J. A. Mota, Bioresour. Technol., 2010, 101, 6225–6229 CrossRef CAS PubMed
.
-
(a) T. H. Fife and L. K. Jao, J. Org. Chem., 1965, 30, 1492–1495 CrossRef CAS
;
(b) S. Sugai, T. Kodama, S. Akaboshi and S. Ikegami, Chem. Pharm. Bull., 1984, 32, 99–105 CrossRef CAS
;
(c) R. A. Ugarte and T. W. Hudnall, Green Chem., 2017, 19, 1990–1998 RSC
.
- S. A. Patwardhan and S. Der, Synthesis, 1974, 348–349 CrossRef CAS
.
-
(a) L. Myles, R. Gore, M. Špulák, N. Gathergood and S. J. Connnon, Green Chem., 2010, 12, 1157–1162 RSC
;
(b) M. X. Tan, L. Gu, N. Li, J. Y. Ying and Y. Zhang, Green Chem., 2013, 15, 1127–1132 RSC
.
-
(a) D. B. G. Williams and M. C. Lawton, Green Chem., 2008, 10, 914–917 RSC
;
(b) Z. Miao, L. Xu, H. Song, H. Zhao and L. Chou, Catal. Sci. Technol., 2013, 3, 1942–1954 RSC
;
(c) N. M. Leonard, M. C. Oswald, D. A. Freiberg, B. A. Nattier, R. C. Smith and R. S. Mohan, J. Org. Chem., 2002, 67, 5202–5207 CrossRef CAS PubMed
;
(d) S. M. Patel, U. V. Chudasama and P. A. Ganeshpure, J. Mol. Catal. A: Chem., 2003, 194, 267–271 CrossRef CAS
;
(e) J.-Y. Qi, J.-X. Ji, C.-H. Yueng, H.-L. Kwong and A. S. C. Chan, Tetrahedron Lett., 2004, 45, 7719–7721 CrossRef CAS
;
(f) R. Kumar and A. K. Chakraborti, Tetrahedron Lett., 2005, 46, 8319–8323 CrossRef CAS
;
(g) H. Mansilla and M. M. Afonso, Synth. Commun., 2008, 38, 2607–2618 CrossRef CAS
;
(h) A. D. Chowdhury and G. K. Lahiri, Chem. Commun., 2012, 48, 3448–3450 RSC
.
- A. Thurkauf, A. E. Jacobson and K. C. Riee, Synthesis, 1988, 233–234 CrossRef CAS
.
- B. Procuranti and S. J. Connon, Org. Lett., 2008, 10, 4935–4938 CrossRef CAS PubMed
.
- A. J. Showler and P. A. Darley, Chem. Rev., 1967, 67, 427–440 CrossRef CAS PubMed
.
-
P. T. Anastas and J. C. Warner, Green Chemistry: Theory and Practice, University Press, Oxford, New York, 1998 Search PubMed
.
- J. Grabowski, J. M. Granda and J. Jurczak, Org. Biomol. Chem., 2018, 16, 3114–3120 RSC
.
- K. J. Mickelsen, C. M. Tajc, K. R. Greenwood and C. C. Browder, Synth. Commun., 2012, 42, 186–194 CrossRef CAS
.
-
(a) M. K. Basu, S. Samajdar, F. F. Becker and B. K. Banik, Synlett, 2002, 319–321 CrossRef CAS
;
(b) B. Karimi and B. Golshani, Synthesis, 2002, 784–788 CrossRef CAS
.
- K. Kumamoto, Y. Ichikawa and H. Kotsuki, Synlett, 2005, 2254–2256 CAS
.
- M. Barbasiewicz and M. Mąkosza, Org. Lett., 2006, 8, 3745–3748 CrossRef CAS PubMed
.
- S. Zhao, Y. Jia and Y. F. Song, Catal. Sci. Technol., 2014, 4, 2618–2625 RSC
.
- For selected reviews, see:
(a) C. K. Prier, D. A. Rankic and D. W. C. MacMillan, Chem. Rev., 2013, 113, 5322–5363 CrossRef CAS PubMed
;
(b) D. Ravelli, S. Protti and M. Fagnoni, Acc. Chem. Res., 2016, 49, 2232–2242 CrossRef CAS PubMed
;
(c) M. D. Kärkäs, J. A. Porco Jr. and C. R. J. Stephenson, Chem. Rev., 2016, 116, 9683–9747 CrossRef PubMed
;
(d) K. L. Scubi, T. R. Blum and T. P. Yoon, Chem. Rev., 2016, 116, 10035–10074 CrossRef PubMed
;
(e) N. A. Romero and D. A. Nicewicz, Chem. Rev., 2016, 116, 10075–10166 CrossRef CAS PubMed
;
(f) D. Cambie, C. Bottecchia, N. J. W. Straathof, V. Hessel and T. Noel, Chem. Rev., 2016, 116, 10276–10341 CrossRef CAS PubMed
;
(g) J. Schwarz and B. Konig, Green Chem., 2018, 20, 323–361 RSC
;
(h) I. K. Sideri, E. Voutyritsa and C. G. Kokotos, Org. Biomol. Chem., 2018, 16, 4596–4614 RSC
.
- H. Yi, L. Niu, S. Wang, T. Liu, A. K. Singh and A. Lei, Org. Lett., 2017, 19, 122–125 CrossRef CAS PubMed
.
-
(a) G. N. Papadopoulos, D. Limnios and C. G. Kokotos, Chem. – Eur. J., 2014, 20, 13811–13814 CrossRef CAS PubMed
;
(b) G. N. Papadopoulos and C. G. Kokotos, Chem. – Eur. J., 2016, 22, 6964–6967 CrossRef CAS PubMed
;
(c) G. N. Papadopoulos and C. G. Kokotos, J. Org. Chem., 2016, 81, 7023–7038 CrossRef CAS PubMed
;
(d) N. Kaplaneris, A. Bisticha, G. N. Papadopoulos, D. Limnios and C. G. Kokotos, Green Chem., 2017, 19, 4451–4456 RSC
;
(e) D. Limnios and C. G. Kokotos, Adv. Synth. Catal., 2017, 359, 323–328 CrossRef CAS
;
(f) G. S. Koutoulogenis, M. G. Kokotou, E. Voutyritsa, D. Limnios and C. G. Kokotos, Org. Lett., 2017, 19, 1760–1763 CrossRef CAS PubMed
;
(g) I. Triandafillidi, M. G. Kokotou and C. G. Kokotos, Org. Lett., 2018, 20, 36–39 CrossRef CAS PubMed
;
(h) G. N. Papadopoulos, E. Voutyritsa, N. Kaplaneris and C. G. Kokotos, Chem. – Eur. J., 2018, 24, 1726–1731 CrossRef CAS PubMed
;
(i) I. K. Sideri, E. Voutyritsa and C. G. Kokotos, ChemSusChem, 2019, 12, 4194–4201 CrossRef CAS PubMed
;
(j) N. F. Nikitas, D. I. Tzaras, I. Triandafillidi and C. G. Kokotos, Green Chem., 2020, 22, 471–477 RSC
;
(k) E. Voutyritsa and C. G. Kokotos, Angew. Chem., Int. Ed., 2020, 59, 1735–1741 CrossRef CAS PubMed
.
- N. F. Nikitas, I. Triandafillidi and C. G. Kokotos, Green Chem., 2019, 21, 669–674 RSC
.
-
(a) Z. Zhang and P. R. Schreiner, Synlett, 2007, 1455–1457 CAS
;
(b) P. R. Schreiner and A. Wittkopp, Org. Lett., 2002, 4, 217–220 CrossRef CAS PubMed
;
(c) K. M. Lippert, K. Hof, D. Gerbig, D. Ley, H. Hausmann, S. Guenther and P. R. Schreiner, Eur. J. Org. Chem., 2012, 5919–5927 CrossRef CAS
;
(d) Z. Zhang, Z. Bao and H. Xing, Org. Biomol. Chem., 2014, 12, 3151–3162 RSC
;
(e) Z. M. Salem, J. Saway and J. J. Badillo, Org. Lett., 2019, 21, 8528–8532 CrossRef CAS PubMed
.
- For more details, see ESI.†.
- M. Blain, H. Yau, L. Jean-Gerard, R. Auvergne, D. Benazet, P. R. Schreiner, S. Caillol and B. Andrioletti, ChemSusChem, 2016, 9, 2269–2272 CrossRef CAS PubMed
.
- S. Zhuo, T. Zhu, L. Zhou, C. Mou, H. Chai, Y. Lu, L. Pan, Z. Jin and Y. R. Chi, Angew. Chem., Int. Ed., 2019, 58, 1784–1788 CrossRef CAS PubMed
.
- M. A. Cismesia and T. P. Yoon, Chem. Sci., 2015, 6, 5426–5434 RSC
.
-
(a) R. S. Mulliken, J. Phys. Chem., 1952, 801–822 CrossRef CAS
;
(b) I. R. Gould and S. Farid, Acc. Chem. Res., 1996, 522–528 CrossRef CAS
;
(c) S. Farid, J. P. Dinnocenzo, P. B. Merkel, R. H. Young and D. Shukla, J. Am. Chem. Soc., 2011, 133, 4791–4801 CrossRef CAS PubMed
;
(d) S. Farid, J. P. Dinnocenzo, P. B. Merkel, R. H. Young, D. Shukla and G. Guirado, J. Am. Chem. Soc., 2011, 133, 11580–11587 CrossRef CAS PubMed
;
(e) M. Koch, G. Licari and E. Vaunthey, J. Phys. Chem. B, 2015, 119, 11846–11857 CrossRef CAS PubMed
.
-
(a) E. Arceo, I. D. Junberg, A. Alvarez and P. Melchiorre, Nat. Chem., 2013, 5, 750–756 CrossRef CAS PubMed
;
(b) L. Wozniak, J. J. Murphy and P. Melchiorre, J. Am. Chem. Soc., 2015, 137, 5678–5681 CrossRef CAS PubMed
;
(c) M. Silvi, E. Arceo, I. D. Jurberg, C. Cassini and P. Melchiorre, J. Am. Chem. Soc., 2015, 137, 6120–6123 CrossRef CAS PubMed
;
(d) J. J. Murphy, D. Bastida, S. Paria, M. Fagnoni and P. Melchiorre, Nature, 2016, 532, 218–222 CrossRef CAS PubMed
;
(e) A. Bahamonde and P. Melchiorre, J. Am. Chem. Soc., 2016, 138, 8019–8030 CrossRef CAS PubMed
;
(f) M. L. Spell, K. Devenaux, C. G. Bresnahan, B. L. Bernard, W. Sheffield, R. Kumar and J. R. Ragains, Angew. Chem., Int. Ed., 2016, 55, 6515–6519 CrossRef CAS PubMed
;
(g) R. Wang, L. Wang, Q. Xu, B. Y. Ren and F. Liang, Org. Lett., 2019, 21, 3072–3076 CrossRef CAS PubMed
.
-
(a) R. Iwata, K. Uda, D. Takahashi and K. Toshima, Chem. Commun., 2014, 50, 10695–10698 RSC
;
(b) N. Vallavoju, S. Selvakumar, S. Jockusch, M. P. Sibi and J. Sivaguru, Angew. Chem., Int. Ed., 2014, 53, 5604–5608 CrossRef CAS PubMed
;
(c) T. Kimura, T. Eto, D. Takahashi and K. Toshima, Org. Lett., 2016, 18, 3190–3193 CrossRef CAS PubMed
;
(d) C. Chen, L. Zhu, M. S. Baranov, L. Tang, N. S. Baleeva, A. Y. Smirnov, I. V. Yampolsky, K. M. Solntsev and C. Fang, J. Phys. Chem. B, 2019, 123, 3804–3821 CrossRef CAS PubMed
.
Footnote |
† Electronic supplementary information (ESI) available: Experimental data, 1H, 13C and 19F NMR data, UV-Vis and mechanistic studies. See DOI: 10.1039/d0gc01135e |
|
This journal is © The Royal Society of Chemistry 2020 |