DOI:
10.1039/C8SC03730B
(Edge Article)
Chem. Sci., 2018,
9, 8836-8841
Silver-mediated oxidative functionalization of alkylsilanes†
Received
21st August 2018
, Accepted 22nd September 2018
First published on 24th September 2018
Abstract
A general approach to the functionalization of aliphatic C–Si bonds in the presence of silver salts and oxidants has been reported. This strategy encompasses a range of valuable C–Si transformations, including the direct conversions of a C–Si bond to C–OCF3, C–OBz, C–OCOCF3, C–SCF3, C–SCN, and C–N3 bonds. Among them, trifluoromethoxylation of alkylsilanes is reported for the first time. In addition, mechanistic studies indicate that this reaction may proceed through a radical mechanism.
Organosilicon compounds have a multitude of applications in basic science, medicine, and industry due to their stability and non-toxicity, and natural abundance of silicon.1 For example, organosilicon compounds have received much attention in the cross-coupling reaction.2 However, the strategic functionalization of aliphatic carbon–silicon bonds is limited,3 for example, the trifluoromethoxy (OCF3) group and trifluoromethylthio (SCF3) group are becoming increasingly important in medicinal, agrochemical and materials science due to their strong electron-withdrawing effect and high lipophilicity.4 Thus, the development of efficient methods for the synthesis of OCF3 and SCF3 compounds is of great importance.5,6 However, the trifluoromethoxylation and trifluoromethylthiolation of organosilanes are extremely underdeveloped.7 To the best of our knowledge, no trifluoromethoxylation of alkylsilanes has been reported to date. Herein, we sought a strategy that would facilitate the direct conversion of aliphatic C–Si bonds into a variety of functional groups, including the trifluoromethoxylation and trifluoromethylthiolation of alkylsilanes (Scheme 1).
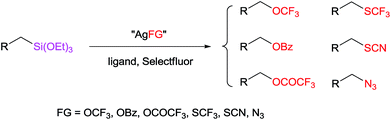 |
| Scheme 1 Silver-mediated oxidative functionalization of alkylsilanes. | |
Methods for aliphatic C–Si oxidation,8 halogenation,9 and azidation10 have been reported, but new reaction systems are typically required to promote each transformation. In addition, methods for trifluoromethoxylation of organosilanes are rare, and only two examples were reported. In 2008, using trifluoromethyl triflate as the trifluoromethoxylation reagent, the Kolomeitsev group reported the trifluoromethoxylation of arynes from o-trimethylsilylphenyl triflate.7a In 2018, trifluoromethyl benzoate (TFBz) was reported as a new trifluoromethoxylation reagent by the Hu group and was used to prepare trifluoromethyl ether from trifluoromethoxylation–halogenation of arynes, which was in situ generated from o-trimethylsilylphenyl triflate.7g Despite the advances in these methods, trifluoromethoxylation of alkylsilanes has not been reported to date, so the development of a general approach for functionalization including trifluoromethoxylation of alkylsilanes is highly desirable.
Inspired by our previous work of a hypervalent iodine-mediated fluorination of alkylsilanes using fluoride ions as the fluorinating agent,9e we became interested in the possibility of functionalization of alkylsilanes using other nucleophiles such as −OCF3. Recently, trifluoromethyl arylsulfonate (TFMS) as a new trifluoromethoxylation reagent was disclosed by our group, which was used to in situ generate AgOCF3 in the presence of silver salts and fluoride ions.11 Thus, we envisioned whether the oxidative trifluoromethoxylation of alkylsilanes could be achieved with −OCF3 which was in situ generated in the presence of fluoride ions and TFMS. Initial investigations focused on the reaction of alkylsilane 1 with various fluorine sources in the presence of trifluoromethyl 4-methylbenzenesulfonate (TFMS, 2) (Table 1, see more details in the ESI†). No desired product 3a was observed when Et3N·3HF, CsF, TBAF or FeF3 was used as the fluoride ion source (Table 1, entries 1–4). We were delighted to find that 61% yield of the desired product 3a was observed in the presence of AgF (Table 1, entry 5). Different ligands were evaluated, and 3,4,7,8-tetramethyl-1,10-phenanthroline gave the highest yield (Table 1, entries 5–10). Switching to other oxidants such as N-fluorobenzenesulfonimide (NFSI), PhIO, PhI(OAc)2, and K2S2O8 could not generate 3a (Table 1, entries 11–14). No desired products were detected in control experiments with AgF or the oxidant omitted. After extensive screening of various solvents, different substitutions on TFMS, and temperatures (see more details in the ESI†), the ideal conditions of 4.0 equiv. of AgF, 0.3 equiv. of 3,4,7,8-tetramethyl-1,10-phenanthroline, 3.0 equiv. of Selectfluor, and 5.0 equiv. of TFMS (2) in 7
:
2 (v
:
v) MeCN/DCM under a N2 atmosphere at 25 °C were found to produce the highest yields. Encouraged by these results, we successfully extended the present system to a trifluoromethylthiolation of alkylsilanes by changing the ligand and silver salt. After optimization of the reaction conditions, the desired trifluoromethylthiolated product (3b) was obtained in 80% yield using 4.0 equiv. of AgSCF3, 0.4 equiv. of 4,4′-di-tert-butyl-2,2′-bipyridine, 3.0 equiv. of Selectfluor, and 4.0 equiv. of CsF in 1
:
1 (v
:
v) MeCN/dioxane under a N2 atmosphere at 50 °C (Table 1, entry 15, see more details in the ESI†).
Table 1 Optimized reaction conditionsa

|
Entry |
−OCF3 or −SCF3 |
Ligand |
Oxidant |
Yield (%)c |
General conditions: 1 (1.0 equiv.), silver salt (4.0 equiv.), TFMS (2) (5.0 equiv.), ligand (0.3 equiv.), oxidant (3.0 equiv.), MeCN/DCM (v/v 7 : 2), 25 °C, N2.
AgSCF3 (4.0 equiv.), CsF (4.0 equiv.), dtbpy (0.4 equiv.), Selectfluor (3.0 equiv.), MeCN/dioxane (v/v 1 : 1), 50 °C, N2.
Yields were determined by 19F NMR with benzotrifluoride as a standard.
|
1 |
Et3N·3HF, TFMS (2) |
3,4,7,8-Me4-Phen |
Selectfluor |
3a, 0 |
2 |
CsF, TFMS (2) |
3,4,7,8-Me4-Phen |
Selectfluor |
3a, 0 |
3 |
TBAF, TFMS (2) |
3,4,7,8-Me4-Phen |
Selectfluor |
3a, 0 |
4 |
FeF3, TFMS (2) |
3,4,7,8-Me4-Phen |
Selectfluor |
3a, 0 |
5 |
AgF, TFMS (2) |
3,4,7,8-Me4-Phen |
Selectfluor |
3a, 61 |
6 |
AgF, TFMS (2) |
Phen |
Selectfluor |
3a, 45 |
7 |
AgF, TFMS (2) |
Neocuproine |
Selectfluor |
3a, 8 |
8 |
AgF, TFMS (2) |
4,7-Ph2-Phen |
Selectfluor |
3a, 52 |
9 |
AgF, TFMS (2) |
5,6-Dione-Phen |
Selectfluor |
3a, 2 |
10 |
AgF, TFMS (2) |
dtbpy |
Selectfluor |
3a, 56 |
11 |
AgF, TFMS (2) |
3,4,7,8-Me4-Phen |
NFSI |
3a, 0 |
12 |
AgF, TFMS (2) |
3,4,7,8-Me4-Phen |
PhIO |
3a, 0 |
13 |
AgF, TFMS (2) |
3,4,7,8-Me4-Phen |
PhI(OAc)2 |
3a, 0 |
14 |
AgF, TFMS (2) |
3,4,7,8-Me4-Phen |
K2S2O8 |
3a, 0 |
15b |
AgSCF3 |
dtbpy |
Selectfluor |
3b, 80 |
Having established optimized reaction conditions, we then explored the scope of trifluoromethoxylation and trifluoromethylthiolation with structurally diverse alkylsilanes. As displayed in Table 2, a wide range of primary alkylsilanes bearing electron-donating and electron-withdrawing substituents on aryl rings were successfully converted into the desired trifluoromethoxylated and trifluoromethylthiolated products with good isolated yields (4 to 16). Notably, heteroaromatic substrates, such as pyridine, indole, and thiophene, were also successfully employed to provide the corresponding trifluoromethoxylated and trifluoromethylthiolated products (17 to 21). A good range of functional groups including ester, ether, ketone, nitrile, nitro, amide, chloride, bromide, and even iodide were well tolerated under the mild reaction conditions. Moreover, the trifluoromethylthiolation of an alkylsilane with tertiary alcohol also proceeded smoothly (36). These results encouraged the application of this method to more complex small molecules, which gave the corresponding trifluoromethoxylated and trifluoromethylthiolated products in moderate yields (39, 40 and 41), for example, the alkylsilane derived from celecoxib, which is a nonsteroidal anti-inflammatory drug, was converted to the tri-fluoromethoxylated product (40a) in 31% yield or trifluoromethylthiolated product (40b) in 57% yield. In addition, we prepared compounds 5a and 5b at the gram scale under the standard reaction conditions in 44% and 69% isolated yields, respectively, which demonstrates the scalability of this method. However, trifluoromethoxylation and trifluoromethylthiolation of secondary alkylsilanes were observed with low yields (37 and 38), and alkenes were the major byproducts.
Table 2 Substrate scope for silver-mediated oxidative trifluoromethoxylation and trifluoromethylthiolation of alkylsilanesa
Conditions A: alkylsilanes (1.0 equiv.), AgF (4.0 equiv.), 3,4,7,8-tetramethyl-1,10-phenanthroline (0.3 equiv.), Selectfluor (3.0 equiv.), TFMS (2) (5.0 equiv.), MeCN/DCM (v/v 7 : 2), N2 atmosphere, 25 °C. Conditions B: alkylsilanes (1.0 equiv.), AgSCF3 (4.0 equiv.), 4,4′-di-tert-butyl-2,2′-bipyridine (0.4 equiv.), Selectfluor (3.0 equiv.), CsF (4.0 equiv.), MeCN/dioxane (v/v 1 : 1), N2 atmosphere, 50 °C. Yields of isolated products are given.
25 °C was used.
MeCN/DCM (v/v 1 : 1) was used.
Yield was determined by 19F NMR with benzotrifluoride as a standard.
|
|
Encouraged by our success with trifluoromethoxylation and trifluoromethylthiolation of alkylsilanes, we investigated the use of other silver salts to develop the functionalization of alkylsilanes (Table 3). When AgOBz, AgOCOCF3, or AgSCN was used, the corresponding products (42, 43, and 44) were obtained in moderate yields. Installation of an azide group has proven to be useful in chemical biology, medicinal chemistry, and materials science.12 The use of AgF and TsN3 enabled azidation of alkylsilane 1 to prepare the desired product 45 in 63% isolated yield. We note that in each system, both the silver salt and oxidant were necessary for productive reactivity. Although these reactions are not fully optimized, they provide a general strategy for the functionalization of alkylsilanes.
Table 3 Substrate scope for silver-mediated oxidative functionalization of alkylsilanes

|
Entry |
AgFG |
Ligand |
Yield |
1 (1.0 equiv.), AgOBz (4.0 equiv.), CsF (4.0 equiv.), ligand (0.4 equiv.), Selectfluor (3.0 equiv.), MeCN/DCE (v/v 1 : 1), 50 °C, N2.
1 (1.0 equiv.), AgOCOCF3 (4.0 equiv.), CsF (4.0 equiv.), ligand (0.4 equiv.), Selectfluor (3.0 equiv.), DMC, 50 °C, N2.
1 (1.0 equiv.), AgSCN (4.0 equiv.), CsF (4.0 equiv.), ligand (0.4 equiv.), Selectfluor (3.0 equiv.), MeCN/DCE (v/v 1 : 1), 50 °C, N2.
1 (1.0 equiv.), AgF (4.0 equiv.), TsN3 (4.0 equiv.), ligand (0.4 equiv.), Selectfluor (II) (3.0 equiv.), MeCN/DCE/EtOH (v/v/v 5 : 5 : 1), 25 °C, N2.
|
1 |
AgOBz |
3,4,7,8-Me4-Phen |
|
2 |
AgOCOCF3 |
4,7-MeO2-Phen |
|
3 |
AgSCN |
4,4′-MeO2-bby |
|
4 |
AgF + TsN3 |
4,7-MeO2-Phen |
|
To gain some insight into the reaction mechanism, we performed some preliminary studies (Scheme 2a). A less than 10% trifluoromethoxylated or trifluoromethylthiolated product was formed when a radical inhibitor 2,6-di-tert-butyl-4-methylphenol (BHT) (8 equiv.) was added. In addition, when 4 equiv. of 2,2,6,6-tetramethyl-1-piperidinyloxy (TEMPO) was added, the TEMPO adduct 46 or 47 was obtained in 25% and 38% isolated yield, respectively. Furthermore, the reaction of alkanesilanes under the standard reaction conditions (conditions B) gave the trifluoromethylthiolated product 48 (37%) along with the 5-exo-cyclization trifluoromethylthiolated product 48′ in 12% yield. Together, these observations strongly suggested that a radical-chain mechanism or single-electron transfer (SET) was involved in the reactions. In addition, a 28% trifluoromethoxylated product was obtained when AgF2 was used in the absence of AgF and Selectfluor, which indicated that Ag(II) species could be involved in the reaction (see more details in the ESI†). Finally, a silver mirror was observed in the reaction, which suggested that Ag(0) was generated.
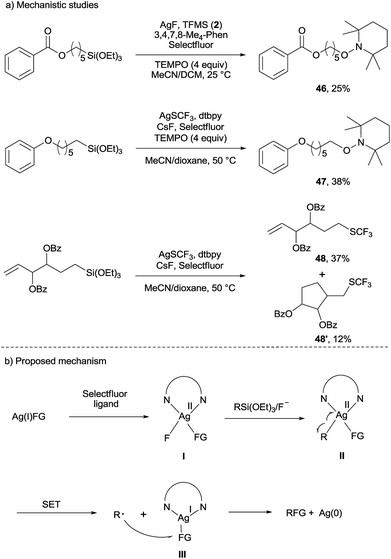 |
| Scheme 2 (a)Mechanistic studies and (b) proposed mechanism. | |
On the basis of these mechanistic investigations, we proposed the mechanism depicted in Scheme 2b. In the presence of ligand and Selectfluor, AgFG (FG = OCF3, OBz, OCOCF3, SCF3, SCN, N3) is oxidized to produce Ag(II) intermediate I;11d then R group transmetalation from silicon to Ag(II) intermediate I can afford alkylsilver species II. The subsequent single-electron transfer between Ag(II) and the R group in intermediate II leads to the generation of the R radical and Ag(I) species III. Finally, the FG group transfer from the intermediate III to the R radical generates RFG and Ag(0). At present, we cannot rule out the possibility of an alternative mechanism in which the R radical intermediate is further oxidized to generate an R carbocation intermediate, which is trapped by the FG anion to form the desired product.
Conclusions
In conclusion, we have developed a silver-mediated oxidative functionalization of alkylsilanes. This strategy enables accessing a range of functionalized products directly, thus obviating the need to develop a new methodology for each specific C–Si transformation. Furthermore, the first example of silver-mediated trifluoromethoxylation of alkylsilanes was developed using trifluoromethyl arylsulfonate (TFMS) as the trifluoromethoxylation reagent. Additionally, preliminary mechanistic studies suggested that this reaction may proceed through a radical mechanism.
Conflicts of interest
There are no conflicts to declare.
Acknowledgements
We gratefully acknowledge the State Key Laboratory of Elemento-Organic Chemistry for generous start-up financial support. This work was supported by the National Key Research and Development Program of China (2016YFA0602900) and the National Natural Science Foundation of China (21522205 and 21672110) and the Fundamental Research Funds for the Central Universities.
Notes and references
-
(a)
A. Bassindale and P. P. Gaspar, Frontiers of Organosilicon Chemistry, Royal Society of Chemistry, 1991 Search PubMed;
(b)
G. L. Larson, Advances in Silicon Chemistry, JAI Press, 1996 Search PubMed;
(c)
N. Auner and J. Weis, Organosilicon Chemistry V, Wiley-VCH Verlag GmbH, 2003 CrossRef.
-
(a)
T. Hiyama, Metal-catalyzed Cross-Coupling Reactions, Wiley-VCH, Weinheim, 1998, ch. 4 Search PubMed;
(b)
M. A. Brook, Silicon in Organic, Organometallic, and Polymer Chemistry, Wiley, New York, 2000 Search PubMed;
(c) S. E. Denmark and J. H. Liu, Angew. Chem., Int. Ed., 2010, 49, 2978 CrossRef CAS PubMed;
(d) Y. Nakao and T. Hiyama, Chem. Soc. Rev., 2011, 40, 4893 RSC;
(e) H. F. Sore, W. R. J. D. Galloway and D. R. Spring, Chem. Soc. Rev., 2012, 41, 1845 RSC;
(f) H. Luo, Z. Zhang, H. Liu and H. Liu, Chin. J. Org. Chem., 2015, 35, 802 CrossRef CAS;
(g) S. E. Denmark and A. Ambrosi, Org. Process Res. Dev., 2015, 19, 982 CrossRef CAS PubMed;
(h) H. Luo, H. Liu, X. Chen, K. Wang, X. Luo and K. Wang, Chem. Commun., 2017, 53, 956 RSC.
-
(a) L. Li, Y. Zhang, L. Gao and Z. Song, Tetrahedron Lett., 2015, 56, 1466 CrossRef CAS;
(b) T. Komiyama, Y. Minami and T. Hiyama, ACS Catal., 2017, 7, 631 CrossRef CAS.
-
(a) A. Leo, C. Hansch and D. Elkins, Chem. Rev., 1971, 71, 525 CrossRef CAS;
(b) F. Leroux, P. Jeschke and M. Schlosser, Chem. Rev., 2005, 105, 827 CrossRef CAS PubMed;
(c) M. Shimizu and T. Hiyama, Angew. Chem., Int. Ed., 2005, 44, 214 CrossRef CAS PubMed;
(d) B. Menteau, S. Pazenok, J. P. Vors and F. R. Leroux, J. Fluorine Chem., 2010, 131, 140 CrossRef;
(e) G. Landelle, A. Panossian and F. R. Leroux, Curr. Top. Med. Chem., 2014, 14, 941 CrossRef CAS PubMed.
- For recent reviews on trifluoromethoxylation reactions, see
(a) A. Tlili, F. Toulgoat and T. Billard, Angew. Chem., Int. Ed., 2016, 55, 11726 CrossRef CAS PubMed;
(b) T. Besset, P. Jubault, X. Pannecoucke and T. Poisson, Org. Chem. Front., 2016, 3, 1004 RSC. For selected examples of the synthesis of trifluoromethoxylated compounds, see
(c) K. Stanek, R. Koller and A. Togni, J. Org. Chem., 2008, 73, 7678 CrossRef CAS PubMed;
(d) R. Koller, K. Stanek, D. Stolz, R. Aardoom, K. Niedermann and A. Togni, Angew. Chem., Int. Ed., 2009, 48, 4332 CrossRef CAS PubMed;
(e) O. Marrec, T. Billard, J. Vors, S. Pazenok and B. R. Langlois, Adv. Synth. Catal., 2010, 352, 2831 CrossRef CAS;
(f) O. Marrec, T. Billard, J. Vors, S. Pazenok and B. R. Langlois, J. Fluorine Chem., 2010, 131, 200 CrossRef CAS;
(g) C. Huang, T. Liang, S. Harada, E. Lee and T. Ritter, J. Am. Chem. Soc., 2011, 133, 13308 CrossRef CAS PubMed;
(h) C. Zhang and D. A. Vicic, Organometallics, 2012, 31, 7812 CrossRef CAS;
(i) K. N. Hojczyk, P. Feng, C. Zhan and M. Y. Ngai, Angew. Chem., Int. Ed., 2014, 53, 14559 CrossRef CAS PubMed;
(j) J. Liu, C. Chen, L. Chu, Z. Chen, X. Xu and F. Qing, Angew. Chem., Int. Ed., 2015, 54, 11839 CrossRef CAS PubMed;
(k) J. Liu, X. Xu and F. Qing, Org. Lett., 2015, 17, 5048 CrossRef CAS PubMed;
(l) C. Chen, P. Chen and G. Liu, J. Am. Chem. Soc., 2015, 137, 15648 CrossRef CAS PubMed;
(m) S. Chen, Y. Huang, X. Fang, H. Li, Z. Zhang, T. S. Andy Hor and Z. Weng, Dalton Trans., 2015, 44, 19682 RSC;
(n) A. Liang, S. Han, Z. Liu, L. Wang, J. Li, D. Zou, Y. Wu and Y. Wu, Chem.–Eur. J., 2016, 22, 5102 CrossRef CAS PubMed;
(o) P. Feng, K. N. Lee, J. W. Lee, C. Zhan and M. Y. Ngai, Chem. Sci., 2016, 7, 424 RSC;
(p) G. Zha, J. Han, X. Hu, H. Qin, W. Fang and C. Zhang, Chem. Commun., 2016, 52, 7458 RSC;
(q) M. Zhou, C. Ni, Z. He and J. Hu, Org. Lett., 2016, 18, 3754 CrossRef CAS PubMed;
(r) C. Chatalova-Sazepin, M. Binayeva, M. Epifanov, W. Zhang, P. Foth, C. Amador, M. Jagdeo, B. R. Boswell and G. M. Sammis, Org. Lett., 2016, 18, 4570 CrossRef CAS PubMed;
(s) Q. Zhang, A. T. Brusoe, V. Mascitti, K. D. Hesp, D. C. Blakemore, J. T. Kohrt and J. F. Hartwig, Angew. Chem., Int. Ed., 2016, 55, 9758 CrossRef CAS PubMed;
(t) J. N. Brantley, A. V. Samant and F. D. Toste, ACS Cent. Sci., 2016, 2, 341 CrossRef CAS PubMed;
(u) S. Krishanmoorthy, S. D. Schnell, H. Dang, F. Fu and G. K. S. Prakash, J. Fluorine Chem., 2017, 203, 130 CrossRef CAS;
(v) W. Huang, X. Wan and Q. Shen, Angew. Chem., Int. Ed., 2017, 56, 11986 CrossRef CAS PubMed;
(w) E. Schmitt, S. Bouvet, B. Pégot, A. Panossian, J. Vors, S. Pazenok, E. Magnier and F. R. Leroux, Org. Lett., 2017, 19, 4960 CrossRef CAS PubMed;
(x) J. W. Lee, D. N. Spiegowski and M. Y. Ngai, Chem. Sci., 2017, 8, 6066 RSC;
(y) C. Chen, Y. Luo, L. Fu, P. Chen, Y. Lan and G. Liu, J. Am. Chem. Soc., 2018, 140, 1207 CrossRef CAS PubMed;
(z) M. Zhou, C. Ni, Y. Zeng and J. Hu, J. Am. Chem. Soc., 2018, 140, 6801 CrossRef CAS PubMed;
(a
a) W. Zheng, C. A. Morales-Rivera, J. W. Lee, P. Liu and M. Ngai, Angew. Chem., Int. Ed., 2018, 57, 9645 CrossRef CAS PubMed;
(a
b) B. J. Jelier, P. F. Tripet, E. Pietrasiak, I. Franzoni, G. Jeschke and A. Togni, Angew. Chem., Int. Ed., 2018 DOI:10.1002/anie.201806296.
- For recent reviews on trifluoromethylthiolation reactions, see
(a) X. Shao, C. Xu, L. Lu and Q. Shen, Acc. Chem. Res., 2015, 48, 1227 CrossRef CAS PubMed;
(b) X. Xu, K. Matsuzaki and N. Shibata, Chem. Rev., 2015, 115, 731 CrossRef CAS PubMed;
(c) H. Chachignon and D. Cahard, Chin. J. Chem., 2016, 34, 445 CrossRef CAS . For selected examples of the synthesis of trifluoromethylthiolated compounds, see: ;
(d) A. Ferry, T. Billard, B. R. Langlois and E. Bacqué, Angew. Chem., Int. Ed., 2009, 48, 8551 CrossRef CAS PubMed;
(e) G. Teverovskiy, D. S. Surry and S. L. Buchwald, Angew. Chem., Int. Ed., 2011, 50, 7312 CrossRef CAS PubMed;
(f) C. Zhang and D. A. Vicic, J. Am. Chem. Soc., 2012, 134, 183 CrossRef CAS PubMed;
(g) L. D. Tran, I. Popov and O. Daugulis, J. Am. Chem. Soc., 2012, 134, 18237 CrossRef CAS PubMed;
(h) X. Shao, X. Wang, T. Yang, L. Lu and Q. Shen, Angew. Chem., Int. Ed., 2013, 52, 3457 CrossRef CAS PubMed;
(i) T. Bootwicha, X. Liu, R. Pluta, I. Atodiresei and M. Rueping, Angew. Chem., Int. Ed., 2013, 52, 12856 CrossRef CAS PubMed;
(j) Y. Yang, A. Azuma, E. Tokunaga, M. Yamasaki, M. Shiro and N. Shibata, J. Am. Chem. Soc., 2013, 135, 8782 CrossRef CAS PubMed;
(k) E. V. Vinogradova, P. Müller and S. L. Buchwald, Angew. Chem., Int. Ed., 2014, 53, 3125 CrossRef CAS PubMed;
(l) G. Danoun, B. Bayarmagnai, M. F. Gruenberg and L. J. Goossen, Chem. Sci., 2014, 5, 1312 RSC;
(m) F. Yin and X. Wang, Org. Lett., 2014, 16, 1128 CrossRef CAS PubMed;
(n) J. Liu, X. Xu, Z. Chen and F. Qing, Angew. Chem., Int. Ed., 2015, 54, 897 CrossRef CAS PubMed;
(o) G. Yin, I. Kalvet, U. Englert and F. Schoenebeck, J. Am. Chem. Soc., 2015, 137, 4164 CrossRef CAS PubMed;
(p) S. Guo, X. Zhang and P. Tang, Angew. Chem., Int. Ed., 2015, 54, 4065 CrossRef CAS PubMed;
(q) H. Wu, Z. Xiao, J. Wu, Y. Guo, J. Xiao, C. Liu and Q. Chen, Angew. Chem., Int. Ed., 2015, 54, 4070 CrossRef CAS PubMed;
(r) X. Liu, R. An, X. Zhang, J. Luo and X. Zhao, Angew. Chem., Int. Ed., 2016, 54, 5846 CrossRef PubMed;
(s) S. Mukherjee, B. Maji, A. Tlahuext-Aca and F. Glorius, J. Am. Chem. Soc., 2016, 138, 16200 CrossRef CAS PubMed;
(t) L. J. C. B. Milandou, H. Carreyre, S. Alazet, G. Greco, A. Martin-Mingot, C. N. Loumpangou, J. Ouamba, F. Bouazza, T. Billard and S. Thibaudeau, Angew. Chem., Int. Ed., 2017, 56, 169 CrossRef PubMed;
(u) Z. Zhang, Z. Sheng, W. Yu, G. Wu, R. Zhang, W. Chu, Y. Zhang and J. Wang, Nat. Chem., 2017, 9, 970 CrossRef CAS PubMed.
-
(a) A. A. Kolomeitsev, M. Vorobyev and H. Gillandt, Tetrahedron Lett., 2008, 49, 449 CrossRef CAS;
(b) J. Liu, L. Chu and F. Qing, Org. Lett., 2013, 15, 894 CrossRef CAS PubMed;
(c) J. Sheng and J. Wu, Org. Biomol. Chem., 2014, 12, 7629 RSC;
(d) S. Alazet, L. Zimmer and T. Billard, Chem.–Eur. J., 2014, 20, 8589 CrossRef CAS PubMed;
(e) S. Arimori, M. Takada and N. Shibata, Org. Lett., 2015, 17, 1063 CrossRef CAS PubMed;
(f) Y. Zeng and J. Hu, Org. Lett., 2016, 18, 856 CrossRef CAS PubMed;
(g) M. Zhou, C. Ni, Y. Zeng and J. Hu, J. Am. Chem. Soc., 2018, 140, 6801 CrossRef CAS PubMed.
-
(a) A. G. Brook, J. Am. Chem. Soc., 1958, 80, 1886 CrossRef CAS;
(b) A. G. Brook, Acc. Chem. Res., 1974, 7, 77 CrossRef CAS;
(c) A. Riahi, J. Cossy, J. Muzart and J. P. Pete, Tetrahedron Lett., 1985, 26, 839 CrossRef CAS;
(d) A. S. Guram, Synlett, 1993, 4, 259 CrossRef;
(e) C. Kaimakliotis and A. J. Fry, J. Org. Chem., 2003, 68, 9893 CrossRef CAS PubMed;
(f) A. B. Smith III and C. M. Adams, Acc. Chem. Res., 2004, 37, 365 CrossRef PubMed;
(g) Y. Deng, Q. Liu and A. B. Smith III, J. Am. Chem. Soc., 2017, 139, 9487 CrossRef CAS PubMed.
-
(a) K. Tamao, J. Yoshida, M. Takahashi, H. Yamanoto, T. Kakui, H. Matsumoto, A. Kurita and M. Kumada, J. Am. Chem. Soc., 1978, 100, 290 CrossRef CAS;
(b) K. Tamao, T. Kakui and M. Kumada, J. Am. Chem. Soc., 1978, 100, 2268 CrossRef CAS;
(c) J. Yoshida, K. Tamao and M. Kumada, J. Am. Chem. Soc., 1980, 102, 3269 CrossRef CAS;
(d) K. Tamao, J. Yoshida, H. Yamamoto, T. Kakui, H. Matsumoto, M. Takahashi, A. Kurita, M. Murata and M. Kumada, Organometallics, 1982, 1, 355 CrossRef CAS;
(e) P. Xu, F. Wang, G. Fan, X. Xu and P. Tang, Angew. Chem., Int. Ed., 2017, 56, 1101 CrossRef CAS PubMed.
- M. Arimoto, H. Yamaguchi, E. Fujita, M. Ochiai and Y. Nagao, Tetrahedron Lett., 1987, 28, 6289 CrossRef CAS.
-
(a) S. Guo, F. Cong, R. Guo, L. Wang and P. Tang, Nat. Chem., 2017, 9, 546 CrossRef CAS PubMed;
(b) X. Jiang, Z. Deng and P. Tang, Angew. Chem., Int. Ed., 2018, 57, 292 CrossRef CAS PubMed;
(c) F. Cong, Y. Wei and P. Tang, Chem. Commun., 2018, 54, 4473 RSC;
(d) H. Yang, F. Wang, X. Jiang, Y. Zhou, X. Xu and P. Tang, Angew. Chem., Int. Ed., 2018 DOI:10.1002/anie.201807144.
- For selected reviews, see:
(a)
S. Bräse and K. Banert, Organic Azides: Synthesis and Applications, Wiley-VCH, Weinheim, 2010 Search PubMed;
(b) S. Bräse, C. Gil, K. Knepper and V. Zimmermann, Angew. Chem., Int. Ed., 2005, 44, 5188 CrossRef PubMed.
Footnotes |
† Electronic Supplementary Information (ESI) available: Data for new compounds and experimental procedures. See DOI: 10.1039/c000000x/. |
‡ These authors contributed equally to this work. |
|
This journal is © The Royal Society of Chemistry 2018 |