DOI:
10.1039/C4QO00308J
(Research Article)
Org. Chem. Front., 2015,
2, 133-140
Binary reducing agents containing dichloroindium hydride for the selective, partial, or tandem reductions of bifunctional compounds consisting of halo-nitriles, halo-esters and halo-carboxylic acids†‡
Received
21st November 2014
, Accepted 27th December 2014
First published on 30th December 2014
Abstract
The selective, partial, or tandem reductions of bifunctional compounds containing primary alkyl or benzyl bromides can generate a variety of different products using a mixture of dichloroindium hydride (HInCl2) and an additional hydride, such as borane–tetrahydrofuran (BH3
:
THF) or diisobutylaluminum hydride (DIBAL-H). Binary metal hydride systems, containing HInCl2 and BH3
:
THF, are readily generated from anhydrous indium trichloride (InCl3) and sodium borohydride (NaBH4) in THF. Dichloroindium hydride can reduce primary halides and in the presence of another hydride, either generated in situ or added to the single-pot reaction, can perform tandem reductions of a variety of bifunctional bromides. Together, the hydrides reduce carbon–halogen bonds as well as an electrophilic group, such as a nitrile, an ester, or a carboxylic acid. The reduction of 4-(bromomethyl)benzonitrile using the HInCl2 and BH3
:
THF binary metal hydride system affords 4-methylbenzylamine in an excellent yield under ambient conditions and short reaction times. By using the binary metal hydride system consisting of HInCl2 and DIBAL-H, the tandem reduction of 4-(bromomethyl)benzonitrile was achieved affording para-tolualdehyde in an excellent yield, also under ambient conditions. The reduction of 4-(bromomethyl)phenyl acetic acid by HInCl2 selectively reduced the carbon–halogen bond generating para-tolylacetic acid. When using either of the binary hydride systems, both functional groups were reduced generating 4-methylphenethyl alcohol. Methyl 4-(bromomethyl) benzoate underwent a selective or tandem reduction to generate [4-(bromomethyl)phenyl]methanol, methyl 4-methyl benzoate or para-tolylmethanol depending on the hydride system used. Consequently, a single bifunctional compound was transformed into a variety of different compounds by the simple manipulation of the binary hydride system used without the need for protecting groups, and in most cases, by a one-pot procedure.
1. Introduction
Chemoselective reduction is an important tool in synthetic organic chemistry. The development of partial, selective, and tandem reaction cascades helps reduce or eliminate the need for protecting groups, which can help improve the yield while decreasing waste generation.1a There are a variety of reducing agents that vary in strength for the reduction of many different electrophilic functional groups.1b Traditional and common metal hydride reducing agents, such as sodium borohydride (NaBH4)2a–c or lithium aluminum hydride (LiAlH4),3a–c have been used and studied extensively. The ability of LiAlH4 to reduce most functional groups limits its use in multifunctional compounds when selective reduction is desired.3a Conversely, NaBH4 is a mild reducing agent with limited abilities and does not reduce electrophiles, such as nitriles, carboxylic acids, or esters.2a Sodium borohydride is known to reduce aldehydes or ketones in the presence of other functional groups.2d New reducing agents, such as dichloroindium hydride (HInCl2), have been developed for the selective reduction of an alkyl halide bond in multifunctional compounds.4a–d Indium hydride reagents such as LiInH4, LiPhInH3 and LiPh2InH2 were first prepared from InCl3 and LiH by Wiberg and Schmidt.5 Subsequently, Butsugan and coworkers suggested that these metals hydrides reduce aldehydes and ketones, but esters were not reduced using LiInH4.6
There are many methods to generate dichloroindium hydride (Fig. 1). Baba and coworkers synthesized HInCl2 from InCl3 and tributyltin hydride (eqn (1)) and used it to reduce a variety of functional groups, such as aldehydes, ketones, and alkyl halides.4d,7a,b However other methods of generating HInCl2 have been developed to avoid using toxic tributyltin hydride. Baba and coworkers also showed that HInCl2 can be prepared from InCl3 using less toxic triethylsilane (Et3SiH)4b,8 (eqn (2)), which can undergo a 1,4-addition to enones9 in addition to reducing carbon–halogen bonds. Additionally, we had previously explored the preparation of HInCl2 from InCl3 and lithium aminoborohydride (LAB) but this system required a careful control of the stoichiometry of the reactants (eqn (3)).10–13 Baba and Oshima have shown that HInCl2 can also be generated from InCl3 and diisobutylaluminum hydride (DIBAL-H) at 0 °C (eqn (4)).14–16 Oshima and coworkers have demonstrated that HInCl2 and catalytic amounts of Et3B selectively reduced alkyl halides in the presence of aromatic esters and aromatic ketones. Finally, HInCl2 can also be synthesized by the reduction of InCl3 with NaBH4 in acetonitrile (eqn (5)) or THF (eqn (6)).12
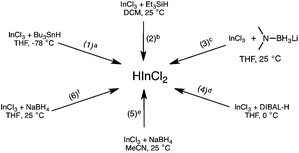 |
| Fig. 1 Different methods for generating HInCl2. aThe side product was tributyltin chloride. bChlorotriethylsilane was generated, but does not interfere with the reduction. c3 equiv. of anhydrous InCl3 and 1 equiv. of MeLAB were needed to generate HInCl2. Excess LAB reagent would over-reduce HInCl2 to In(0). N,N-dimethylaminoborane and LiCl were generated, but do not hinder the reduction. d Diisobutylaluminum chloride was generated. e1 equiv. of anhydrous InCl3 and 3 equiv. of NaBH4 were necessary to generate HInCl2. Acetonitrile trapped the in situ formation of borane, creating a single hydride reducing system consisting of only HInCl2. f1 equiv. of anhydrous InCl3 and 3 equiv. of NaBH4 generated HInCl2. BH3 : THF was generated in situ. | |
A closer examination of the InCl3/NaBH4 system by 11B NMR spectroscopy revealed a unique solvent effect.12 When the synthesis of HInCl2 from InCl3 and NaBH4 was carried out in THF, 11B NMR analysis showed the formation of an equimolar amount of BH3
:
THF.12 When the same reaction was performed in acetonitrile (MeCN), the 11B NMR spectrum showed the formation of an “N-BH2” species, arising from in situ trapping of borane generated by the solvent.12 Additionally, we have recently demonstrated that the binary reducing system, prepared from InCl3/NaBH4 in THF, readily reduced a variety of aliphatic and aromatic nitriles to the corresponding primary amine in excellent yields.12 Subsequently, we became interested in exploring the use of this binary hydride system to reduce a variety of other bifunctional compounds that contained a primary alkyl or benzyl bromide and an additional electrophilic functionality in a selective, partial, or tandem fashion. We anticipated that HInCl2 present in the binary hydride mixture would reduce the primary alkyl or benzyl bromides to the corresponding hydrocarbon, while BH3
:
THF would reduce the nitrile group of a halonitrile to the corresponding primary amine and carboxylic acids to the alcohol. We also envisioned that a binary reducing system containing HInCl2 and DIBAL-H can be exploited to reductively manipulate halonitriles, haloesters, and haloacids in a selective, partial, or tandem fashion. Herein, we report an operationally simple, selective, partial, or tandem reduction of bifunctional compounds containing alkyl and benzyl halides by binary hydrides often capable of being carried out in a one-pot reaction.
2. Results and discussion
Our goal was to develop the chemoselective reduction of bifunctional compounds using a single or binary hydride system. Developing a new methodology for the selective reduction of multi-functional compounds would help eliminate the use of protecting groups in synthetic organic chemistry, reduce the number of steps in a reaction and ultimately increase the overall yield while reducing waste. Accordingly, bifunctional primary or benzylic halides containing a carboxylic acid, ester, or nitrile were selected in order to further explore the various hydride systems. We used BH3
:
THF-HInCl2 and DIBAL-H-HInCl2 as our binary hydride reducing systems. We compared our results with those obtained from reducing the representative bifunctional compounds with single hydride reducing agents, such as BH3
:
THF, HInCl2, and DIBAL-H. All the reductions were carried out at 25 °C and the products were isolated by standard aqueous acid–base workup procedures and identified by NMR spectral analyses.
2.1 Reduction of 6-bromohexanoic acid
We selected 6-bromohexanoic acid as the representative for the family of aliphatic halo-acids. There are three different outcomes possible in the selective or tandem reduction of 6-bromohexanoic acid, which include 6-bromohexanol, 1-hexanol, or hexanoic acid. The selective or tandem reduction of halogenated carboxylic acids was achieved using a variety of different hydrides and the results are summarized in Scheme 1. The selective reduction of the carboxylic acid gave 6-bromohexanol and can be accomplished using DIBAL-H or BH3
:
THF with yields of 94 and 98% respectively (Scheme 1, b or c). Both reactions were carried out under ambient conditions. For complete reduction, three equivalents of DIBAL-H were needed, whereas only one equivalent of BH3
:
THF was needed for the quantitative production of 6-bromohexanol. Neither BH3
:
THF nor DIBAL-H reduced the carbon–halogen bond. Using BH3
:
THF was more attractive because of the stoichiometry, facile work-up procedure and a higher yield of 6-bromohexanol. However, commercial samples of BH3
:
THF are often compromised during the shipping and handling process.17 Consequently, it is advisable to check the purity of BH3
:
THF by 11B NMR or hydride analysis as some of the commercial samples contain only tributoxyborate due to shipping and/or handling. The selective reduction of the carbon–halogen bond afforded hexanoic acid, and was achieved using two equivalents of HInCl2 synthesized from InCl3 and DIBAL-H in THF. This pathway was the only method that selectively cleaved the carbon–halogen bond while leaving the unprotected carboxylic acid intact and required no radical initiator, such as Et3B.4a,14,18a,b A stepwise reduction of 6-bromohexanoic acid to hexanol was achieved by reducing the acid first using BH3
:
THF or DIBAL-H followed by the reduction of the crude 6-bromohexanol by HInCl2 generated in a separate flask from InCl3 and DIBAL-H. Unfortunately, the InCl3/NaBH4 in the THF system did not afford the tandem reduction of the carbon–halogen and the carboxylic acid group, but instead generated 6-bromohexanol. This is because the reduction of the carbon–bromide bond by HInCl2 was inhibited by BH3
:
THF.12,16 The reduction of 6-bromohexaonic acid with InCl3 and NaBH4 in MeCN gave a complex mixture of products.
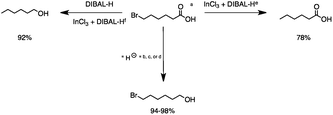 |
| Scheme 1 The selective or tandem reduction of 6-bromohexanoic acid with single or binary hydrides. aAll reactions were run on a 3 mmol substrate scale at 25 °C for 4 h under Ar. b3 equivalents of DIBAL-H is required for the complete reduction of the carboxylic acid group (94%). c1 equivalent of BH3 : THF is required for complete reduction of the carboxylic acid group (98%). d1 equivalent of InCl3 and 3 equivalents of NaBH4 in THF were used for the reduction of the carboxylic acid group (94%). e2 equivalents of HInCl2 (from InCl3 and DIBAL-H) are required for complete reduction of the C–Br bond. The first for deprotonation of the acidic proton and the second for the cleavage of the C–Br bond (78%). fHInCl2 generated in a separate flask from InCl3 and DIBAL-H and added to 6-bromohexanol (92%). | |
2.2 Reduction of ethyl 6-bromohexanoate
Ethyl 6-bromohexanoate was selected as the representative example to probe the possibility of a tandem and selective reduction of bromo esters using binary reducing agents. Three different products were feasible via the selective or tandem reduction (Table 1). The selective reduction of the ester carbonyl group, which gave the bromo alcohol, was accomplished by using two equivalents of DIBAL-H under ambient conditions (Table 1, entry 1). Brown had shown that pure BH3
:
THF does not reduce esters. However, commercial BH3
:
THF, containing NaBH4 as a stabilizer, afforded some amount of bromo alcohol arising from the reduction of the ester group (Table 1, entry 2). We achieved a selective reduction of the carbon–bromide bond using two equivalents of HInCl2 generated from InCl3 and DIBAL-H to afford ethyl hexanoate, again without the use of a protecting group, a radical initiator, or column purification (Table 1, entry 3).4 In our hands, the use of 1.3 equiv. of HInCl2 gave incomplete reduction and, in order to achieve a full reduction of the carbon–bromide bond, we used one equivalent excess hydride rather than using the pyrophoric radical initiator triethyl borane.18a,b The stepwise reduction of the carbon–bromide bond and the ester carbonyl group was carried out using a two-pot procedure. The ester was reduced using DIBAL-H, which gave the halo-alcohol. The isolated halo-alcohol was then reduced using HInCl2, which gave the corresponding aliphatic alcohol (Table 1, entry 4). Indium trichloride (InCl3) and NaBH4 in the THF system gave the selective reduction of the ester group and formed the bromo alcohol. Since BH3
:
THF alone did not reduce the ester, the excess NaBH4 present must have catalyzed the reduction of the ester and allowed for the formation of 6-bromohexanol albeit in a lower isolated yield. As expected, switching the solvent from THF to acetonitrile (MeCN) resulted in the selective reduction of the carbon–bromide bond (Table 1, entry 6). When one equivalent of HInCl2 was used, only the starting material was recovered. However when we increased HInCl2 to two equivalents, ethyl hexanoate was afforded in a 92% isolated yield.
Table 1 The selective and tandem reductions of aliphatic halo-esters
2.3 Reduction of methyl octanoate
Methyl octanoate was used as a representative example for the family of aliphatic esters in our study. We were interested in comparing the reductive reactivity of aliphatic esters with the results obtained with aliphatic bromo esters using binary reducing agents (Table 2). In the reduction of an aliphatic ester, two products were possible: partial reduction to give an aldehyde or complete reduction leading to a primary alcohol. At ambient temperature, complete reduction of the ester group was achieved using two equivalents of DIBAL-H (Table 2, entry 1). It should be noted that at −78 °C DIBAL-H is capable of reducing esters to the corresponding aldehyde with very little over reduction arising from β-hydride elimination.19,20 As with the bromo ester, commercial BH3
:
THF produced a mixture of octanol and an unreacted starting material via an incomplete NaBH4 catalyzed ester reduction (Table 2, entry 2). Dichloroindium hydride, generated from InCl3/DIBAL-H in THF or InCl3/NaBH4 in MeCN, did not reduce the aliphatic ester group (Table 2, entries 3 and 6). Dichloroindium hydride, as a single hydride reagent, did not reduce the ester (Table 2, entries 3 and 6). However, excess DIBAL-H proceeded to give octanol (Table 2, entry 4). InCl3 and NaBH4 in THF generated the binary reducing system containing both HInCl2 and BH3
:
THF.12 This mixture of hydrides reduced methyl octanoate to 1-octanol in a quantitative yield (Table 2, entry 5). Even though both HInCl2 and BH3
:
THF were not capable of reducing the ester group on their own, in the binary reducing system the two hydrides acted synergistically and reduced the carbonyl group. Dichloroindium hydride potentially acts as a Lewis acid to activate the ester group and facilitates hydride transfer from BH3
:
THF. We have previously observed similar synergistic effects in the reduction of aryl cyanides using a binary reducing system containing both HInCl2 and BH3
:
THF.12
Table 2 Reduction of aliphatic esters with mono- or binary hydrides
2.4 Reduction of benzylic halides containing an aromatic ester
For the carboalkoxy benzylic halide family of compounds, methyl 4-(bromomethyl) benzoate was selected. Three different reduction products can arise from methyl 4-(bromomethyl) benzoate, either in a selective or tandem reduction. Diisobutylaluminum hydride selectively reduced the ester group to the corresponding alcohol in an excellent yield and was the only method that selectively reduced the ester functionality in the presence of a benzylic bromide (Table 3, entry 1). The stoichiometry of DIBAL-H was important in this reduction. When 3 equivalents of hydride were used, we observed the reduction of the ester group as well as a significant reduction of the carbon–bromide bond. By decreasing the DIBAL-H to 2.2 equivalents, only the ester group was reduced affording isolated [4-(bromomethyl)phenyl]methanol in 90% yield. Conversely, pure or commercial BH3
:
THF alone does not reduce aromatic esters and only the starting material was recovered (Table 3, entry 2). The selective reduction of the carbon–bromide bond can be achieved in a variety of ways. Dichloroindium hydride (HInCl2) generated from InCl3 and DIBAL-H selectively reduced the carbon–halogen bond (Table 3, entry 3) in 78% yield. Indium trichloride and the NaBH4 system in THF or MeCN (Table 3, entries 5 and 6) gave methyl p-toluate in 99 and 84% yield, respectively. Although InCl3 and NaBH4 in THF generated both HInCl2 and BH3
:
THF, only the carbon–halogen bond was cleaved because neither BH3
:
THF nor HInCl2 can reduce aromatic esters. The highest yield of methyl p-toluate was achieved using the InCl3/NaBH4 reducing system in THF and involved a straightforward work-up procedure. Unfortunately, the tandem reduction of the carbon–halogen bond and the aromatic ester group was not achieved using HInCl2 and excess DIBAL-H in a sequential addition one-pot procedure. The aluminum alkoxide formed in the ester reduction was likely insoluble, making the initial product of the tandem reduction unavailable for the second stage of the reduction. para-(Bromomethyl) benzyl alcohol was isolated from the first step and the crude compound then underwent reduction with HInCl2 to give para-methylbenzyl alcohol.
Table 3 Selective and tandem reductions of aromatic halo-esters
Because we sought to accomplish the tandem reduction in a one-pot procedure, we turned our attention to another reducing agent. Lithium dimethylaminoborohydride (methyl-LAB) is not only known to reduce ester groups, but LAB and InCl3 can also generate HInCl2. As previously mentioned, the stoichiometry of methyl-LAB to InCl3 plays a significant role in the formation of HInCl2.12 In order to successfully generate HInCl2 from InCl3 and methyl-LAB, 3 equivalents of InCl3 were needed for 1 equivalent of the LAB reagent, otherwise HInCl2 gets over-reduced to a metal nugget.12 In order to eliminate the use of an excess anhydrous InCl3, we decided to generate HInCl2 from DIBAL-H since this reaction only required 1 equivalent of InCl3 and 1 equivalent of DIBAL-H. With HInCl2 in hand, methyl 4-(bromomethyl) benzoate was added, and the reduction of the benzyl bromide proceeded smoothly. Methyl-LAB was then added resulting in the reduction of the ester group (Table 3, entry 4). Immediately upon the addition of the LAB reagent, a solid precipitate aggregated to form a metal nugget in the reaction flask. Excess LAB reagent was then added in order to reduce the ester functional group. We speculated that this metallic nugget was indium(0), because we have previously observed the same nugget formation when generating HInCl2 from InCl3 and LAB.12 Powder X-ray diffraction (PXRD) was used to elucidate the composition of the metal nugget. Analysis of the PXRD data showed that the sample was a highly crystalline indium metal with no impurities or amorphous phases (Fig. 2).
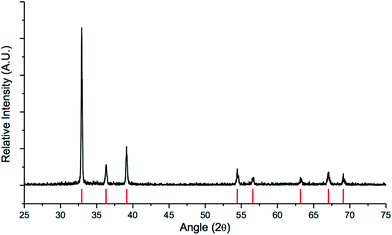 |
| Fig. 2 PXRD spectrum of the indium metal nugget. Red bars at the bottom indicate the theoretical peak positions of indium (PDF card #01-075-3850). | |
2.5 Reduction of 4-(bromomethyl)phenyl acetic acid
4-Bromomethy-l-phenyl acetic acid served as a representative example for the family of halomethyl-aryl acids. Three different products are possible via either a selective or tandem reduction (Scheme 2). Both DIBAL-H and BH3
:
THF achieved the selective reduction of the carboxylic acid group in the presence of the benzyl bromide functionality in 85% and 94% isolated yields, respectively (Scheme 2, b and c). It should be pointed out that only one equivalent of BH3
:
THF21 was required for the selective reduction of carboxylic acid, while three equivalents of DIBAL-H were required for the complete reduction of the carboxylic acid group at 25 °C.22 The selective reduction of the carbon–halogen bond was successfully achieved using HInCl2 generated from InCl3 and DIBAL-H and gave para-tolylacetic acid in a 90% yield without the need for a protecting group or a radical initiator (Scheme 2, d). A one-pot tandem reduction of 4-(bromomethyl)phenylacetic acid was achieved using a variety of different binary metal hydride systems. Examples include HInCl2 generated from InCl3 and an excess of DIBAL-H, as well as HInCl2 from InCl3 and NaBH4 in THF or MeCN. For the tandem reduction, HInCl2 from InCl3 and DIBAL-H followed by 3 equivalents of DIBAL-H gave 4-methylphenethyl alcohol in a 70% isolated yield (Scheme 2, e). The same product was accessed through InCl3 and NaBH4 in THF or MeCN with isolated yields of 94 and 59% respectively (Scheme 2, f and g). The binary reducing system containing HInCl2 and BH3
:
THF gave the highest isolated yield of 4-methylphenethyl alcohol and involved a simple work-up procedure.
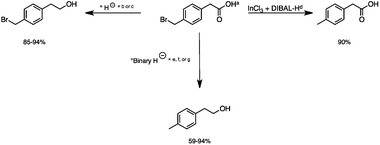 |
| Scheme 2 The selective and tandem reduction of aromatic halo-carboxylic acids. aThe reaction runs on a 3 mmol scale at 25 °C. b3 equiv. of DIBAL-H is required for the complete reduction of carboxylic acid (85%). c1 equiv. of BH3 : THF is required for the complete reduction of carboxylic acid (94%). d6 mmol HInCl2 generated from InCl3 and DIBAL-H and allowed to stir for 1 hour. 3 mmol of the substrate was added to HInCl2 and allowed to stir for 4 hours (90%). eA binary hydride system was used for the tandem reduction of 4-(bromomethyl)phenyl acetic acid. 6 mmol HInCl2 was generated from InCl3 and DIBAL-H and allowed to stir for 1 hour. 3 mmol of the substrate was added to HInCl2 and allowed to stir for 4 hours followed by the addition of 9 mmol of DIBAL-H for the reduction of the carboxylic acid (70%). fThe binary hydride system HInCl2 and BH3 : THF was generated from InCl3 and NaBH4 in THF and allowed to mix for 1 hour, followed by the addition of 4-(bromomethyl)phenyl acetic acid and stirred for 4 hours (94%). gHInCl2 was generated from InCl3 and NaBH4 in MeCN. Excess NaBH4 reduced the carboxylic acid group. The reaction was allowed to stir for 1 hour, followed by the addition of 4-(bromomethyl)phenyl acetic acid and stirred for 4 more hours (59%). | |
2.6 Reduction of 4-(halomethyl)benzonitrile
4-(Bromomethyl)benzonitrile was selected as a representative member of the family of aromatic halo-nitriles. The most interesting results of the selective, partial and tandem reductions were seen with halogenated substituted aromatic nitriles. Binary reducing agents led to five different useful compounds. Partial reduction of the nitrile group in both 4-(bromomethyl)benzonitrile and 4-(chloromethyl)benzonitrile was carried out at 25 °C and gave the corresponding halomethyl substituted benzaldehyde in isolated yields of 85% and 80%, respectively (Table 4, entry 1). The selective reduction of 4-(bromomethyl)benzonitrile can be achieved using BH3
:
THF under THF reflux conditions for 12 h to afford 4-(bromomethyl)-phenyl)methanamine (Table 4, entry 2).21 In our study, this was the only pathway available to selectively convert a nitrile to the corresponding primary amine in the presence of a primary or benzylic halide. 4-(Bromomethyl)benzonitrile was reduced, by the sequential addition of DIBAL-H followed by HInCl2, to 4-methylbenzaldehyde through the intermediate formation of the corresponding imine derivative (Table 4, entry 4). When the tandem reduction was carried out under ambient conditions using InCl3 and NaBH4 in THF, HInCl2 reduced the carbon–halogen bond and BH3
:
THF reduced the nitrile and gave para-tolymethanamine (Table 4, entry 5).12 For the selective reduction of the carbon–halogen bond we used InCl3 and NaBH4 in the MeCN system, where borane generated in situ was trapped by MeCN and rendered the borane unavailable for reduction. Consequently, InCl3/NaBH4 in MeCN caused the selective reduction of the carbon–halogen bond to afford para-methylbenzonitrile (Table 4, entry 6).12 This selective reduction was also achieved using HInCl2 prepared from InCl3 and DIBAL-H (Table 4, entry 3).
Table 4 Selective, partial, and tandem reduction of aromatic halo-nitriles
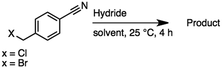
|
Entrya |
Hydrideb |
Product |
Yieldc |
All reactions were run on a 3 mmol substrate scale at 25 °C for 4 h under Ar.
The reaction was run in a two-pot procedure. DIBAL-H was added to 4-(bromomethyl)benzonitrile and allowed to stir for 4 hours. In a separate pot, HInCl2 was generated and then added to the first reaction mixture.
Isolated yield.
Ref. 21.
Ref. 12.
|
1 |
DIBAL-H |
|
X = Cl, 80% |
X = Br, 85% |
2 |
BH3 : THF |
|
Ref. 21
|
3 |
InCl3 + DIBAL-H |
|
X = Cl, 74% |
X = Br, 85% |
4 |
DIBAL-Hb |
|
X = Cl,63% |
InCl3 + DIBAL-H |
X = Br, 67% |
5 |
InCl3 + NaBH4 |
|
X = Cl, 65% |
THF |
X = Br, 61%e |
6 |
InCl3 + NaBH4 |
|
X = Cl, 68% |
MeCN |
X = Br, 65%e |
3. Conclusions
Dichloroindium hydride containing either BH3
:
THF or DIBAL-H has shown promising results as a binary hydride reducing system to achieve the selective, partial, and tandem reductions of bifunctional benzylic halides. Primary halides and benzylic halides containing a carboxylic acid, an ester, or a nitrile functional group were selected for further study. Our goal was to develop chemoselective reductions of these bifunctional compounds using a single or binary hydride system. By developing a new methodology for the partial, selective, or tandem reduction of bifunctional compounds it was demonstrated that the synthesis of a variety of different products can be achieved from a single starting material without the use of protecting groups or pyrophoric radical initiators. With many ways of generating HInCl2, the binary hydride reducing system can be utilized in a deliberate way for the chemoselective reduction of a targeted functional group. For example, 4-(bromomethyl)benzonitrile produces five different products, based on the binary hydride system used. Diisobutylaluminum hydride generated 4-(bromomethyl)benzaldehyde in an excellent yield, while BH3
:
THF gave 4-(bromomethyl)benzylamine. When 4-(bromomethyl)benzonitrile was sequentially reduced with HInCl2 and DIBAL-H, para-tolualdehyde was obtained as the product. When the binary reducing system of HInCl2 and BH3
:
THF, generated in situ from InCl3/NaBH4 in THF was used, 4-methylbenzylamine was isolated as the tandem reduction product. Meanwhile, when using HInCl2 as a single hydride, whether generated from InCl3via DIBAL-H or NaBH4 in MeCN, only the carbon–halogen bond was cleaved and the nitrile was left intact. This method also works for other benzyl halides containing electrophilic groups, such as carboalkoxy benzylic bromide which gave three possible different products. This methodology was expanded to both aliphatic and aromatic esters containing a primary or benzylic bromide generating four and three possible different products, respectively. We also included the reductions of monosubstituted compounds for comparison. The results are summarized in Table 5.
Table 5 Summary of selective, partial, or tandem reductions of mono- and bi-substituted compounds with different hydrides
Hydride |
DIBAL-H |
BH3 : THF |
HInCl2 (from InCl3 + DIBAL-H) |
HInCl2 (from InCl3 + DiBAlH) + DIBAL-H |
HInCl2 + BH3 : THF (InCl3 + NaBH4 in THF) |
HInCl2 + “BH2Z” (InCl3 + NaBH4 in MeCN) |
Substrate |
NR = no reaction.
Ref. 22.
Ref. 21.
Ref. 4a.
Ref. 12.
Ref. 16.
Ref. 7a.
Reduction of the functional group occurs from DIBAL-H or BH3 : THF. HInCl2 alone does not reduce carboxylic acids.
Ref. 6.
Mixture of products.
|
n-C11H23CH2Br |
NRa,b |
NRa,c |
n-C11H23CH3 d |
n-C11H23CH2 d |
Incomplete reductione,f |
n-C11H23CH3 e,f |
|
NRa,b |
NRa,c |
|
|
Incomplete reductione,f |
|
|
|
|
NRa |
|
|
NRa |
|
|
|
NRa |
|
|
NRa |
|
|
|
|
|
|
|
|
|
|
|
|
|
|
|
|
|
NRa |
|
|
NRa |
|
|
|
NRa |
— |
|
— |
|
|
|
NRa |
— |
|
— |
|
|
|
|
|
|
|
|
|
|
|
|
|
|
|
|
|
|
|
|
|
|
|
NRa |
|
|
|
|
|
|
|
|
|
|
|
|
|
|
|
|
|
|
The method described herein has shown promising results in the partial, selective, and tandem reductions of compounds containing multiple-functional groups without the need for protecting groups. In most cases a one-pot procedure was used along with simple work-up procedures that resulted in good to excellent isolated yields. All the reductions reported in this manuscript were carried out under ambient conditions and with short reaction times. A simple manipulation of the binary reducing system containing HInCl2 can potentially generate a wide variety of different compounds starting from bifunctional primary or benzylic halides.
Notes and references
-
(a) R. A. Shenvi, D. P. O'Malley and P. S. Baran, Acc. Chem. Res., 2009, 42, 530 CrossRef CAS PubMed;
(b) E. R. Walker, Chem. Soc. Rev., 1976, 5, 23 RSC.
-
(a) H. C. Brown, H. I. Schlesinger and D. M. Ritter, J. Am. Chem. Soc., 1953, 75, 192 CrossRef CAS;
(b) S. Chandrasekhar and A. Shrinidhi, Synth. Commun., 2014, 44, 2051 CrossRef CAS;
(c) K. Gilmore, S. Vukelić, D. T. McQuade, B. Koksch and P. Seeberger, Org. Process Res. Dev., 2014, 18, 1771 CrossRef CAS;
(d) D. E. Ward and C. K. Rhee, Can. J. Chem., 1989, 67, 1206 CrossRef CAS.
-
(a) A. E. Finholt, A. C. Bond and H. I. Schlesinger, J. Am. Chem. Soc., 1947, 69, 1199 CrossRef CAS;
(b) B. Thiedemann, C. M. L. Schmitz and A. Staubitz, J. Org. Chem., 2014, 79, 10284 CrossRef CAS PubMed;
(c) B. Ravinder, S. R. Reddy, P. A. Reddy and R. Bandichhor, Tetrahedron Lett., 2013, 54, 4908 CrossRef CAS PubMed.
-
(a) K. Takami, S. Mikami, H. Yorimitsu, H. Shinolubo and K. Oshima, Tetrahedron, 2003, 59, 6627 CrossRef CAS;
(b) L. Benati, G. Bencivenni, R. Leardini, D. Nanni, M. Minozzi, P. Spagnolo, R. Scialpi and G. Zanardi, Org. Lett., 2006, 8, 2499 CrossRef CAS PubMed;
(c) B. C. Ranu, A. Das and A. Hajra, Synthesis, 2003, 7, 1012 CrossRef PubMed;
(d) N. Hayashi, H. Honda, M. Yasuda, I. Shibata and A. Baba, Org. Lett., 2006, 8, 4553 CrossRef CAS PubMed.
- E. Wiberg, O. Dittman and M. Schmidt, Z. Naturforsch., 1957, 12, 56 Search PubMed.
- M. Yamada, K. Tanaka, S. Araki and Y. Butsugan, Tetrahedron Lett., 1995, 36, 3169 CrossRef CAS.
-
(a) T. Miyai, K. Inoup, M. Yasida, I. Shibata and A. Baba, Tetrahedron Lett., 1998, 39, 1929 CrossRef CAS;
(b) K. Inoue, T. Ishida, I. Shibata and A. Baba, Adv. Synth. Catal., 2002, 344, 283 CrossRef CAS.
- I. Shibata and A. Baba, Org. Lett., 2005, 7, 3093 CrossRef PubMed.
- I. Shibata, H. Kato, T. Ishida, M. Yasuda and A. Baba, Angew. Chem., Int. Ed., 2004, 43, 711 CrossRef CAS PubMed.
-
(a) G. B. Fischer, J. Harrison, J. C. Fuller, C. T. Goralski and B. Singaram, Tetrahedron Lett., 1992, 33, 4533 CrossRef;
(b) L. Pasumansky, C. Goralski and B. Singaram, Org. Process Res. Dev., 2014, 10, 2006 Search PubMed;
(c) S. Thomas, C. J. Collins, J. R. Cuzens, D. Spiciarich, C. T. Goralksi and B. Singaram, J. Org. Chem., 2001, 66, 1999 CrossRef CAS PubMed.
-
(a) G. B. Fisher, J. C. Fuller, J. Harrison, S. G. Alvarez, E. R. Burkhardt, C. T. Goralski and B. Singaram, J. Org. Chem., 1994, 59, 6378 CrossRef CAS;
(b) L. Pasumansky, D. Haddenham, J. W. Clary, G. B. Fisher, C. T. Goralski and B. Singaram, J. Org. Chem., 2008, 73, 1898 CrossRef CAS PubMed;
(c) D. Haddenham, L. Pasumansky, J. DeSoto, S. Eagon and B. Singaram, J. Org. Chem., 2009, 74, 1964 CrossRef CAS PubMed.
- J. Z. Saavedra, A. Resendez, A. Rovira, S. Eagon, D. Haddenham and B. Singaram, J. Org. Chem., 2012, 77, 221 CrossRef CAS PubMed.
- J. Z. Saavedra, P. Bayrasy, A. Resendez, R. Snelling, M. H. Anderson and B. Singaram, ARKIVOC, 2012, 167 CrossRef CAS.
- K. Takimi, H. Yorimitsu and K. Oshima, Org. Lett., 2002, 4, 2993 CrossRef PubMed.
- T. Miyai, K. Inoue, M. Yasuda, I. Shibata and A. Baba, Tetrahedron Lett., 1998, 39, 1929 CrossRef CAS.
- K. Inoue, A. Sawada, I. Shibata and A. Baba, J. Am. Chem. Soc., 2002, 124, 906 CrossRef CAS PubMed.
- M. Potyen, K. V. B. Josyula, M. Schuck, S. Lu, P. Gao and C. Hewitt, Org. Process Res. Dev., 2007, 11, 210 CrossRef CAS.
-
(a) K. Takami, S. Usugi, H. Yorimitsu and K. Oshima, Synthesis, 2005, 0824 CAS;
(b) K. Takami, H. Yorimitsu and K. Oshima, Org. Lett., 2004, 6, 4555 CrossRef CAS PubMedS. Usugi, H. Yorimitsu and K. Oshima, Tetrahedron Lett., 2001, 42, 4535 CrossRef CAS.
- I. M. Khorlina and L. I. Zakharkin, Tetrahedron Lett., 1962, 14, 619 Search PubMed.
- C. Szántay, L. Töke and P. Kolonits, J. Org. Chem., 1966, 31, 1447 CrossRef.
- N. M. Yoon, P. Heim and H. C. Brown, J. Am. Chem. Soc., 1970, 92, 1637 CrossRef.
- N. M. Yoon and Y. S. Gyoung, J. Org. Chem., 1985, 50, 2443 CrossRef CAS.
Footnotes |
† Dedicated to Prof. Ei-ichi Negishi on the occasion of his 80th birthday. |
‡ Electronic supplementary information (ESI) available: Experimental procedures and the characterization data of the isolated compounds. See DOI: 10.1039/c4qo00308j |
|
This journal is © the Partner Organisations 2015 |
Click here to see how this site uses Cookies. View our privacy policy here.