DOI:
10.1039/C4QO00292J
(Research Article)
Org. Chem. Front., 2015,
2, 127-132
A chiral bicyclo[2.2.2]octa-2,5-diene ligand substituted with the ferrocenyl group and its use for rhodium-catalyzed asymmetric 1,4-addition reactions†
Received
7th November 2014
, Accepted 22nd December 2014
First published on 23rd December 2014
Abstract
A chiral diene, (R,R)-Fc,Ph-bod, which bears the ferrocenyl (Fc) group and the phenyl (Ph) group on the bicyclo[2.2.2]octa-2,5-diene skeleton, has been synthesized, and its rhodium complex was examined as a catalyst for the asymmetric 1,4-addition of arylboronic acids to α,β-unsaturated ketones. Its enantioselectivity was generally higher than that observed with (R,R)-Ph-bod, which is C2 symmetric with two phenyl groups.
Introduction
Chiral dienes have attracted considerable attention as chiral ligands for transition metal-catalyzed asymmetric reactions,1 and a structurally diverse array of chiral dienes have been developed for various types of catalytic asymmetric reactions.1 In the first report published in 2003, we have synthesized a C2 symmetric chiral diene whose basic skeleton is bicyclo[2.2.1]hepta-2,5-diene and we used it successfully for rhodium-catalyzed asymmetric conjugate addition reactions.2 Carreira reported C1 symmetric chiral diene ligands which are based on the bicyclo[2.2.2]octa-2,5-diene skeleton.3 Subsequently, there appeared chiral dienes based on bicyclo[3.3.1]nona-2,6-diene4 and bicyclo[3.3.0]octa-2,5-diene.5Fig. 1 illustrates some of the bicyclo[2.2.2]octa-2,5-diene (bod) that have been most commonly used.6–10 Some of them are C2 symmetric and some others are not.
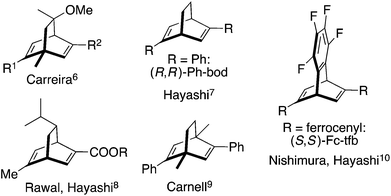 |
| Fig. 1 Some of the chiral diene ligands based on the bicyclo[2.2.2]octa-2,5-diene skeleton. | |
One remarkable feature of the bod-type ligands is that Fc-tfb, which bears two ferrocenyl (Fc) groups on the tetrafluorobenzobarrelene (tfb) skeleton,10a has shown higher catalytic activity and higher enantioselectivity than other ligands in several types of rhodium-catalyzed asymmetric reactions.10–15 Typically, they are asymmetric additions of organoboron reagents to unsaturated compounds, such as aldehydes,10a imines,11 β-alkoxyacrylates,12 α,β-unsaturated sulfonyl compounds,13 and enynamides.14
On the other hand, Abele and coworkers recently reported16 that enantiomerically pure 5-phenylbicyclo[2.2.2]oct-5-en-2-one 1 is readily available on a large scale through an organocatalytic one-pot Michael addition–aldol reaction and the ketone 1 is a suitable substrate for the synthesis of 2,5-diphenylbicyclo[2.2.2]octa-2,5-diene (Ph-bod) and its C1-symmetric analogs substituted with aryl and alkyl groups (Scheme 1). These chiral dienes have been examined as chiral ligands for the rhodium-catalyzed asymmetric addition of arylboronic acids to enones and N-sulfonylimines.17
 |
| Scheme 1 Synthesis of chiral bicyclo[2.2.2]octa-2,5-diene ligands by Abele. | |
In this article, we report on the synthesis of a novel diene obtained by introducing the ferrocenyl group onto the bod skeleton based on Abele's procedure,16 and the diene ligand (Fc,Ph-bod) thus obtained which is substituted with ferrocenyl and phenyl groups is examined for its enantioselectivity in the rhodium-catalyzed asymmetric 1,4-addition of aryl- and alkenylboronic acids to α,β-unsaturated ketones.18 The enantioselectivity is compared with that observed with Ph-bod and Fc-tfb to see the effects of the ferrocenyl group.
Results and discussion
Synthesis of 2-ferrocenyl-5-phenylbicyclo[2.2.2]octa-2,5-diene (Fc,Ph-bod)
According to the procedures reported by Abele,16 the ketone 1 was converted into alkenyl triflate 2, and it was subjected to the palladium-catalyzed cross-coupling with the ferrocenylzinc reagent7 (Scheme 2). An excess amount of FcZnCl, which is generated by the reaction of ferrocene with t-BuLi followed by treatment of the resulting FcLi with ZnCl2, was allowed to react with triflate 2 in the presence of 10 mol% Pd(PPh3)4 in refluxing THF for 16 h. Aqueous work-up followed by silica gel column chromatography gave the diene, (R,R)-Fc,Ph-bod, in 72% yield. This diene was used for the preparation of the rhodium complex, [RhCl((R,R)-Fc,Ph-bod)]2, by the ligand exchange reaction with [RhCl(coe)2]2 in dichloromethane.
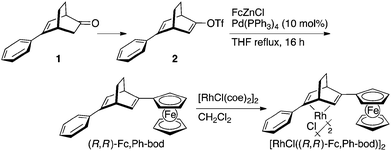 |
| Scheme 2 Synthesis of 2-ferrocenyl-5-phenylbicyclo[2.2.2]octa-2,5-diene (Fc,Ph-bod). | |
Asymmetric 1,4-addition catalyzed by rhodium complexes coordinated with (R,R)-Fc,Ph-bod and its related chiral dienes
Table 1 summarizes the results of the asymmetric 1,4-addition of organoboronic acids to α,β-unsaturated cyclic ketones in the presence of a rhodium catalyst coordinated with (R,R)-Fc,Ph-bod obtained above. For comparison, this table also contains the results of reactions in the presence of other related chiral diene/rhodium complexes, which have been previously reported as effective catalysts for the asymmetric 1,4-addition. First, (R,R)-Fc,Ph-bod was examined for the addition of phenylboronic acid to 2-cyclohexenone, which is one of the benchmark reactions to demonstrate the high efficiency of newly developed chiral rhodium catalysts.1 Thus, the reaction of 2-cyclohexenone 3a with phenylboronic acid 4m (2 equiv.) was carried out in the presence of [RhCl((R,R)-Fc,Ph-bod)]2 (3 mol% Rh) and KOH (50 mol%) in dioxane/H2O (10/1) at 30 °C for 1 h to give a high yield (99%) of (R)-3-phenylcyclohexanone 5am with 98% ee (entry 1). In situ generation of the chiral diene/rhodium catalyst from [RhCl(coe)2]2 and (R,R)-Fc,Ph-bod gave the addition product 5am of the same 98% ee (entry 2). This enantioselectivity is slightly higher than that observed with (R,R)-Ph-bod under the same conditions (entries 3 and 4). The higher enantioselectivity of (R,R)-Fc,Ph-bod (95% ee) than (R,R)-Ph-bod (93% ee) was also observed in the addition of alkenylboronic acid 4n to 3a (entries 5 and 6). In comparison, (R,R)-Ph-tfb and (S,S)-Fc-tfb gave the product 5an with 93% and 98% ee, respectively (entries 7 and 8). In the addition of phenylboronic acid 4m to acyclic enone, (E)-non-3-en-2-one 3b, which is known to be a difficult substrate to achieve high enantioselectivity in the rhodium-catalyzed conjugate addition, Fc,Ph-bod gave the product 3bm of 90% ee (entry 9). Although this enantioselectivity is lower than that obtained with Fc-tfb (98% ee, entry 12), it is higher than that obtained with Ph-bod (83% ee, entry 10) and Ph-tfb (85% ee, entry 11). The higher enantioselectivity of Fc,Ph-bod than Ph-bod was also observed in the reaction of enone 3b with phenylboronic acids substituted with 4-methoxy 4o, 4-chloro 4p, and 4-methoxycarbonyl 4q groups (entries 13–18). Similarly, the reaction of another acyclic enone, (E)-5-methylhex-3-en-2-one (3c), with arylboronic acids, 4m, 4o, 4p, and 4q, proceeded with higher enantioselectivity in the presence of Fc,Ph-bod than Ph-bod (entries 19–26).
Table 1 Rhodium-catalyzed asymmetric 1,4-addition of organoboronic acids to α,β-unsaturated ketonesa
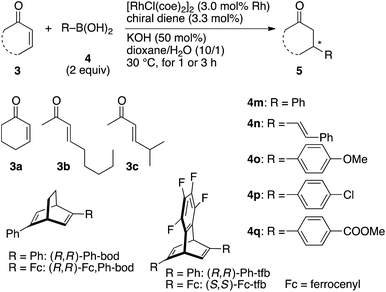
|
Entry |
3
|
4
|
Time (h) |
Ligand |
Product 5 |
Yieldb (%) |
eec (%) |
Enone 3 (0.30 mmol), boronic acid 4 (0.60 mmol), [RhCl(coe)2]2 (9.0 μmol of Rh), diene ligand (9.9 μmol), and KOH (0.15 mmol) in dioxane–H2O (1.0/0.1 mL).
Isolated yield.
The % ee was determined by chiral HPLC. The absolute configurations of known compounds were determined by comparison of their optical rotations with those reported, and the absolute configurations of new compounds, 5bq, 5cp, and 5cq, are estimated by similarity of the stereochemical pathway.
Isolated rhodium complex [RhCl(diene)]2 was used in place of in situ generation from [RhCl(coe)2]2 and the diene ligand.
In the experiment of entries 7 and 11, the (S,S) isomer of the Ph-tfb ligand was used. For easier understanding of the stereochemical outcome, it is shown as if the (R,R) isomer is used. In entry 12, (R,R)-Fc-tfb was used in the experiment.
|
1d |
3a
|
4m
|
1 |
(R,R)-Fc,Ph-bod |
5am
|
99 |
98 (R) |
2 |
|
|
1 |
(R,R)-Fc,Ph-bod |
5am
|
93 |
98 (R) |
3d |
|
|
1 |
(R,R)-Ph-bod |
5am
|
93 |
97 (R) |
4 |
|
|
1 |
(R,R)-Ph-bod |
5am
|
95 |
97 (R) |
5 |
3a
|
4n
|
3 |
(R,R)-Fc,Ph-bod |
5an
|
89 |
95 (R) |
6 |
|
|
3 |
(R,R)-Ph-bod |
5an
|
90 |
93 (R) |
7d |
|
|
3 |
(R,R)-Ph-tfbe |
5an
|
87 |
93 (R) |
8d |
|
|
3 |
(S,S)-Fc-tfb |
5an
|
99 |
98 (R) |
9 |
3b
|
4m
|
1 |
(R,R)-Fc,Ph-bod |
5bm
|
90 |
90 (S) |
10 |
|
|
1 |
(R,R)-Ph-bod |
5bm
|
97 |
83 (S) |
11d |
|
|
1 |
(R,R)-Ph-tfbe |
5bm
|
85 |
85 (S) |
12d |
|
|
1 |
(S,S)-Fc-tfbe |
5bm
|
92 |
98 (S) |
13 |
3b
|
4o
|
1 |
(R,R)-Fc,Ph-bod |
5bo
|
95 |
95 (S) |
14 |
|
|
1 |
(R,R)-Ph-bod |
5bo
|
96 |
89 (S) |
15 |
3b
|
4p
|
2 |
(R,R)-Fc,Ph-bod |
5bp
|
81 |
92 (S) |
16 |
|
|
2 |
(R,R)-Ph-bod |
5bp
|
76 |
89 (S) |
17 |
3b
|
4q
|
2 |
(R,R)-Fc,Ph-bod |
5bq
|
99 |
93 (S) |
18 |
|
|
2 |
(R,R)-Ph-bod |
5bq
|
96 |
87 (S) |
19 |
3c
|
4m
|
1 |
(R,R)-Fc,Ph-bod |
5cm
|
91 |
88 (R) |
20 |
|
|
1 |
(R,R)-Ph-bod |
5cm
|
84 |
84 (R) |
21 |
3c
|
4o
|
1 |
(R,R)-Fc,Ph-bod |
5co
|
96 |
92 (R) |
22 |
|
|
1 |
(R,R)-Ph-bod |
5co
|
98 |
89 (R) |
23 |
3c
|
4p
|
1 |
(R,R)-Fc,Ph-bod |
5cp
|
88 |
94 (R) |
24 |
|
|
1 |
(R,R)-Ph-bod |
5cp
|
93 |
92 (R) |
25 |
3c
|
4o
|
1 |
(R,R)-Fc,Ph-bod |
5co
|
99 |
92 (R) |
26 |
|
|
1 |
(R,R)-Ph-bod |
5co
|
99 |
90 (R) |
In all the reactions shown in Table 1, the Fc,Ph-bod ligand showed higher enantioselectivity than Ph-bod and Ph-tfb, but it was less enantioselective than Fc-tfb. It follows that the Fc (ferrocenyl) group brings about higher enantioselectivity than the Ph group, and two Fc groups are more enantioselective than one Fc group in the present 1,4-addition reactions (Fig. 2).
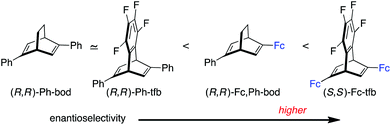 |
| Fig. 2 Higher enantioselectivity after introducing the Fc (ferrocenyl) group. | |
Conclusions
We have synthesized a chiral diene, (R,R)-Fc,Ph-bod, which is C1 symmetric substituted with the ferrocenyl group and the phenyl group. Its rhodium complex, [RhCl((R,R)-Fc,Ph-bod)]2, was used as a catalyst for the asymmetric 1,4-addition of arylboronic acids to α,β-unsaturated ketones to show higher enantioselectivity than the Ph-bod catalyst. Through these studies, it has been demonstrated that the ferrocenyl (Fc) group on the bod ligand contributes to the higher enantioselectivity.
Experimental
General
All air- and moisture-sensitive manipulations were carried out with standard Schlenk techniques under argon. The starting materials were obtained from commercial sources and were used without further purification. THF and dioxane were distilled over benzophenone ketyl under argon. CH2Cl2 was distilled over CaH2 under argon. [RhCl(coe)2]2 was prepared following the literature procedures.19
Preparation of (R,R)-Fc,Ph-bod and [RhCl((R,R)-Fc,Ph-bod)]2
The alkenyl triflate 2 was prepared from enantiomerically pure ketone, 5-phenylbicyclo[2.2.2]oct-5-en-2-one (1), according to the reported procedures.16
To a solution of ferrocene (259 mg, 1.39 mmol) in THF (2.5 mL), t-BuLi (1.5 M in pentane, 0.85 mL, 1.27 mmol) was slowly added at −78 °C, and the mixture was allowed to warm to room temperature and stirred for 2 h. ZnCl2 (173 mg, 1.27 mmol) in THF (1.5 mL) was added and the mixture was stirred at room temperature for 0.5 h to form an FcZnCl solution. The FcZnCl solution was added dropwise to a solution of 2 (100 mg, 0.30 mmol) and Pd(PPh3)4 (35 mg, 0.030 mmol) in THF (1.0 mL), and the mixture was refluxed for 16 h. Water was added to quench the reaction, and the mixture was extracted with Et2O. The organic layer was washed with brine, dried over Na2SO4, and evaporated under vacuum. The residue was subjected to column chromatography on silica gel with hexane–ethyl acetate (49/1) to give the orange solid (R,R)-Fc,Ph-bod (79.5 mg, 0.22 mmol, 72% yield). The diene ligand was allowed to react with [RhCl(coe)2]2 (95 mg, 0.26 mmol) in CH2Cl2 (5 mL) at room temperature for 2 h to give a dark-red solid [RhCl((R,R)-Fc,Ph-bod)]2 (100 mg, 0.20 mmol, 91% yield). The NMR spectrum of [RhCl((R,R)-Fc,Ph-bod)]2 is difficult to be analyzed due to the stereoisomers.
(R,R)-Fc,Ph-bod.
[α]22D +71 (c 0.99, CHCl3). 1H NMR (CDCl3): δ 7.46 (dd, J = 8.4, 1.3 Hz, 2H), 7.34 (t, J = 7.5 Hz, 2H), 7.23 (tt, J = 7.4, 1.1 Hz, 1H), 6.67 (dd, J = 6.4, 2.0 Hz, 1H), 6.30 (dd, J = 6.4, 2.0 Hz, 1H), 4.36–4.37 (m, 1H), 4.34–4.35 (m, 1H), 4.20–4.22 (m, 2H), 4.13 (s, 5H), 4.08–4.10 (m, 1H), 3.93–3.94 (m, 1H), 1.52–1.56 (m, 4H). 13C NMR (CDCl3): δ 146.8, 144.6, 138.3, 129.1, 128.4, 126.7, 125.1, 124.7, 83.7, 68.6, 68.5, 68.4, 65.5, 64.5, 40.8, 39.6, 26.4, 25.8. HRMS (ESI) m/z calcd for C24H22Fe M+ 366.1065, found 366.1060.
General procedure for asymmetric 1,4-addition catalyzed by a rhodium complex in situ generated from [RhCl(coe)2]2 and the chiral diene
A solution of [RhCl(coe)2]2 (9.0 μmol Rh), chiral diene ligand (9.9 μmol), and boronic acid 4 (0.60 mmol) in 1.0 mL of dioxane was stirred at room temperature for 5 min. To this mixture was added aqueous KOH (0.10 mL, 1.5 M, 0.15 mmol), and the mixture was stirred at room temperature for another 5 min. Enone 3 (0.30 mmol) was added, and the mixture was stirred at 30 °C for 1 h or 3 h. The mixture was passed through a short silica gel column (eluent: diethyl ether) and the solvent was removed under vacuum. The residue was subjected to column chromatography on silica gel with EtOAc/hexane to give the 1,4-addition product.
General procedure for asymmetric 1,4-addition catalyzed by an isolated rhodium complex [RhCl(diene)]2
To a solution of [RhCl(diene)]2 complex (9.0 μmol Rh) and boronic acid 4 (0.60 mmol) in 1.0 mL of dioxane was added aqueous KOH (0.10 mL, 1.5 M, 0.15 mmol), and the mixture was stirred at room temperature for 5 min. Enone 3 (0.30 mmol) was added, and the mixture was stirred at 30 °C for 1 h or 3 h. The same work-up as that above gave the 1,4-addition product.
Characterization data for the addition products
(R)-3-Phenylcyclohexanone (5am).
(CAS 34993-51-6) Colorless oil. 99% yield. The ee was determined on a Daicel Chiralpak AD-H column with hexane–2-propanol = 100/1, flow = 1.0 mL min−1. Retention times: 10.0 min [minor enantiomer], 12.3 min [major enantiomer]. 98% ee, [α]25D +20 (c 1.00, CHCl3), R configuration. 1H NMR (500 MHz, CDCl3): δ 7.33 (t, J = 7.5 Hz, 2H), 7.25–7.21 (m, 3H), 3.01 (tt, J = 11.9 and 3.9 Hz, 1H), 2.60 (ddt, J = 14.0, 4.4, and 1.9 Hz, 1H), 2.53 (ddd, J = 13.8, 12.5, and 0.9 Hz, 1H), 2.50–2.43 (m, 1H), 2.38 (dddd, J = 14.3, 12.6, 6.1, and 0.9 Hz, 1H), 2.15 (ddt, J = 13.0, 6.6, and 3.2 Hz, 1H), 2.12–2.06 (m, 1H), 1.91–1.73 (m, 2H).
(R)-3-((E)-2-Phenylethenyl)cyclohexanone (5an).
(CAS 1063949-45-0) Colorless oil. 89% yield. The ee was determined on a Daicel Chiralcel OD-H column with hexane–2-propanol = 100/2, flow = 1.0 mL min−1. Retention times: 15.1 min [minor enantiomer], 17.2 min [major enantiomer]. 95% ee, [α]25D −8.9 (c 1.01, CHCl3), R configuration. 1H NMR (500 MHz, CDCl3): δ 7.35 (d, J = 7.2 Hz, 2H), 7.30 (t, J = 7.6 Hz, 2H), 7.22 (t, J = 7.2 Hz, 1H), 6.39 (d, J = 15.9 Hz, 1H), 6.16 (dd, J = 15.9 and 6.9 Hz, 1H), 2.73–2.62 (m, 1H), 2.57–2.48 (m, 1H), 2.44–2.37 (m, 1H), 2.36–2.27 (m, 2H), 2.15–2.06 (m, 1H), 2.05–1.98 (m, 1H), 1.81–1.69 (m, 1H), 1.67–1.57 (m, 1H).
(S)-4-Phenyl-2-nonanone (5bm).
(CAS 501919-45-5) Colorless oil. 90% yield. The ee was determined on a Daicel Chiralcel OB-H column with hexane–2-propanol = 100/1, flow = 0.5 mL min−1. Retention times: 12.0 min [major enantiomer], 15.8 min [minor enantiomer]. 90% ee, [α]25D +18 (c 1.00, CHCl3), S configuration. 1H NMR (500 MHz, CDCl3): δ 7.28 (t, J = 7.5 Hz, 2H), 7.21–7.15 (m, 3H), 3.15–3.06 (m, 1H), 2.73 (dd, J = 16.1, and 7.4 Hz, 1H), 2.69 (dd, J = 16.1, and 7.0 Hz, 1H), 2.00 (s, 3H), 1.65–1.50 (m, 2H), 1.30–1.06 (m, 6H), 0.82 (t, J = 6.9 Hz, 3H).
(S)-4-(4-Methoxyphenyl)nonan-2-one (5bo).
(CAS 850409-87-9) Colorless oil. 95% yield. The ee was determined on two Daicel Chiralcel OJ-H columns with hexane–2-propanol = 100/1, flow = 0.5 mL min−1. Retention times: 36.2 min [major enantiomer], 39.9 min [minor enantiomer]. 95% ee, [α]25D +18 (c 1.02, CHCl3), S configuration. 1H NMR (500 MHz, CDCl3): δ 7.08 (d, J = 8.6 Hz, 2H), 6.82 (d, J = 8.6 Hz, 2H), 3.78 (s, 3H), 3.10–2.99 (m, 1H), 2.71–2.63 (m, 2H), 2.00 (s, 3H), 1.62–1.45 (m, 2H), 1.29–1.05 (m, 6H), 0.82 (t, J = 6.9 Hz, 3H).
(S)-4-(4-Chlorophenyl)nonan-2-one (5bp).
(CAS 1346758-81-3) Colorless oil. 81% yield. The ee was determined on two Daicel Chiralcel OJ-H columns with hexane–2-propanol = 100/1, flow = 0.5 mL min−1. Retention times: 21.4 min [major enantiomer], 22.6 min [minor enantiomer]. 92% ee, [α]25D +13 (c 0.73, CHCl3), S configuration. 1H NMR (500 MHz, CDCl3): δ 7.25 (d, J = 8.3 Hz, 2H), 7.10 (d, J = 8.3 Hz, 2H), 3.14–3.05 (m, 1H), 2.68 (d, J = 7.2 Hz, 2H), 2.02 (s, 3H), 1.62–1.45 (m, 2H), 1.27–1.03 (m, 6H), 0.82 (t, J = 6.8 Hz, 3H).
(S)-Methyl 4-(2-oxononan-4-yl)benzoate (5bq).
Colorless oil. 99% yield. The ee was determined on two Daicel Chiralcel OJ-H columns with hexane–2-propanol = 100/2, flow = 1.0 mL min−1. Retention times: 23.2 min [major enantiomer], 24.8 min [minor enantiomer]. 93% ee, [α]25D +11 (c 0.87, CHCl3), S configuration. 1H NMR (500 MHz, CDCl3): δ 7.95 (d, J = 8.3 Hz, 2H), 7.24 (d, J = 8.3 Hz, 2H), 3.89 (s, 3H), 3.21–3.16 (m, 1H), 2.73 (d, J = 7.1 Hz, 2H), 2.02 (s, 3H), 1.65–1.50 (m, 2H), 1.27–1.01 (m, 6H), 0.82 (t, J = 6.9 Hz, 3H). 13C NMR (125 MHz, CDCl3): δ 207.3, 167.0, 150.2, 129.8, 128.3, 127.5, 52.0, 50.5, 41.1, 36.2, 31.6, 30.6, 26.9, 22.4, 14.0. HRMS (ESI) m/z calcd for C17H24O3Na (M + Na+) 299.1618, found 299.1628.
(R)-5-Methyl-4-phenylhexan-2-one (5cm).
(CAS 162239-77-2) Colorless oil. 91% yield. The ee was determined on Daicel Chiralcel OD-H + Daicel Chiralpak ID columns with hexane–2-propanol = 100/1, flow = 1.0 mL min−1. Retention times: 12.6 min [major enantiomer], 13.9 min [minor enantiomer]. 88% ee, [α]25D +23 (c 0.88, CHCl3), R configuration. 1H NMR (500 MHz, CDCl3): δ 7.27 (t, J = 7.5 Hz, 2H), 7.18 (t, J = 7.3 Hz, 1H), 7.14 (d, J = 7.4 Hz, 2H), 2.94–2.88 (m, 1H), 2.84–2.7 (m, 2H), 1.97 (s, 3H), 1.83 (octet, J = 6.8 Hz, 1H), 0.93 (d, J = 6.7 Hz, 3H), 0.74 (d, J = 6.7 Hz, 3H).
(R)-4-(4-Methoxyphenyl)-5-methylhexan-2-one (5co).
(CAS 1346758-78-8) Colorless oil. 96% yield. The ee was determined on a Daicel Chiralpak IF column with hexane–2-propanol = 100/1, flow = 1.0 mL min−1. Retention times: 10.2 min [major enantiomer], 11.1 min [minor enantiomer]. 92% ee, [α]25D +29 (c 1.00, CHCl3), R configuration. 1H NMR (500 MHz, CDCl3): δ 7.06 (d, J = 8.6 Hz, 2H), 6.81 (d, J = 8.5 Hz, 2H), 3.77 (s, 3H), 2.88–2.84 (m, 1H), 2.80–2.71 (m, 2H), 1.97 (s, 3H), 1.78 (octet, J = 6.8 Hz, 1H), 0.91 (d, J = 6.7 Hz, 3H), 0.72 (d, J = 6.7 Hz, 3H).
(R)-4-(4-Chlorophenyl)-5-methylhexan-2-one (5cp).
Colorless oil. 88% yield. The ee was determined on two Daicel Chiralcel OJ-H columns with hexane–2-propanol = 100/0.5, flow = 1.0 mL min−1. Retention times: 14.9 min [major enantiomer], 17.4 min [minor enantiomer]. 94% ee, [α]25D +23 (c 0.85, CHCl3), R configuration. 1H NMR (500 MHz, CDCl3): δ 7.24 (d, J = 8.6 Hz, 2H), 7.07 (d, J = 8.4 Hz, 2H), 2.93–2.88 (m, 1H), 2.80 (dd, J = 16.2 and 5.0 Hz, 1H), 2.74 (dd, J = 16.2 and 9.5 Hz, 1H), 1.99 (s, 3H), 1.84–1.74 (m, 1H), 0.92 (d, J = 6.7 Hz, 3H), 0.73 (d, J = 6.7 Hz, 3H). 13C NMR (125 MHz, CDCl3): δ 207.8, 141.8, 131.9, 129.6, 128.3, 47.5, 47.3, 33.2, 30.6, 20.6, 20.2. HRMS (ESI) m/z calcd for C13H16ClO (M − H+) 223.0895, found 223.0890.
(R)-Methyl 4-(2-methyl-5-oxohexan-3-yl)benzoate (5cq).
Pale yellow oil. 99% yield. The ee was determined on a Daicel Chiralpak IF column with hexane–2-propanol = 100/5, flow = 1.0 mL min−1. Retention times: 14.4 min [major enantiomer], 16.2 min [minor enantiomer]. 92% ee, [α]25D +21 (c 0.94, CHCl3), R configuration. 1H NMR (500 MHz, CDCl3): δ 7.94 (d, J = 8.3 Hz, 2H), 7.22 (d, J = 8.3 Hz, 2H), 3.89 (s, 3H), 3.02–2.97 (m, 1H), 2.86–2.77 (m, 2H), 1.99 (s, 3H), 1.89–1.79 (m, 1H), 0.93 (d, J = 6.7 Hz, 3H), 0.73 (d, J = 6.7 Hz, 3H). 13C NMR (125 MHz, CDCl3): δ 207.5, 167.0, 149.0, 129.5, 128.3, 128.3, 51.9, 47.8, 47.3, 33.1, 30.6, 20.6, 20.3. HRMS (ESI) m/z calcd for C15H20O3Na (M + Na+) 271.1305, found 271.1300.
Acknowledgements
We thank Dr Stefan Abele, Actelion Pharmaceuticals Ltd, for a generous gift of 100 g of enantiomerically pure 5-phenylbicyclo[2.2.2]oct-5-en-2-one. We also thank Ms Lidan Yu for her experiments at an early stage. We are grateful to the National University of Singapore and the Ministry of Education (MOE) of Singapore (R-143-000-362-112 and R-143-000-539-133) for financial support.
Notes and references
- For reviews on chiral diene ligands, see:
(a) C.-G. Feng, M.-H. Xu and G.-Q. Lin, Synlett, 2011, 1345 CAS;
(b) R. Shintani and T. Hayashi, Aldrichimica Acta, 2009, 42, 31 CAS;
(c) C. Defieber, H. Grützmacher and E. M. Carreira, Angew. Chem., Int. Ed., 2008, 47, 4482 CrossRef CAS PubMed;
(d) J. B. Johnson and T. Rovis, Angew. Chem., Int. Ed., 2008, 47, 840 CrossRef CAS PubMed.
- T. Hayashi, K. Ueyama, N. Tokunaga and K. Yoshida, J. Am. Chem. Soc., 2003, 125, 11508 CrossRef CAS PubMed.
- C. Fischer, C. Defieber, T. Suzuki and E. M. Carreira, J. Am. Chem. Soc., 2004, 126, 1628 CrossRef CAS PubMed.
-
(a) Y. Otomaru, N. Tokunaga, R. Shintani and T. Hayashi, Org. Lett., 2005, 7, 307 CrossRef CAS PubMed;
(b) Y. Otomaru, A. Kina, R. Shintani and T. Hayashi, Tetrahedron: Asymmetry, 2005, 16, 1673 CrossRef CAS PubMed.
-
(a) Z.-Q. Wang, C.-G. Feng, M.-H. Xu and G.-Q. Lin, J. Am. Chem. Soc., 2007, 129, 5336 CrossRef CAS PubMed;
(b) C.-G. Feng, Z.-Q. Wang, P. Tian, M.-H. Xu and G.-Q. Lin, Chem. – Asian J., 2008, 3, 1511 CrossRef CAS PubMed;
(c) S. Helbig, S. Sauer, N. Cramer, S. Laschat, A. Baro and W. Frey, Adv. Synth. Catal., 2007, 349, 2331 CrossRef CAS.
- C. Defieber, J.-F. Paquin, S. Serna and E. M. Carreira, Org. Lett., 2004, 6, 3873 CrossRef CAS PubMed.
-
(a) N. Tokunaga, Y. Otomaru, K. Okamoto, K. Ueyama, R. Shintani and T. Hayashi, J. Am. Chem. Soc., 2004, 126, 13584 CrossRef CAS PubMed;
(b) Y. Otomaru, K. Okamoto, R. Shintani and T. Hayashi, J. Org. Chem., 2005, 70, 2503 CrossRef CAS PubMed.
-
(a) K. Okamoto, T. Hayashi and V. Rawal, Chem. Commun., 2009, 4815 RSC;
(b) K. Okamoto, T. Hayashi and V. Rawal, Org. Lett., 2008, 10, 4387 CrossRef CAS PubMed.
- Y. Luo and A. J. Carnell, Angew. Chem., Int. Ed., 2010, 49, 2750 CrossRef CAS PubMed.
-
(a) T. Nishimura, H. Kumamoto, M. Nagaosa and T. Hayashi, Chem. Commun., 2009, 5713 RSC;
(b) T. Nishimura, M. Nagaosa and T. Hayashi, Chem. Lett., 2008, 37, 860 CrossRef CAS;
(c) T. Nishimura, Y. Yasuhara, M. Nagaosa and T. Hayashi, Tetrahedron: Asymmetry, 2008, 19, 1778 CrossRef CAS PubMed.
-
(a) T. Nishimura, Y. Ebe, H. Fujimoto and T. Hayashi, Chem. Commun., 2013, 49, 5504 RSC;
(b) T. Nishimura, A. Noishiki, Y. Ebe and T. Hayashi, Angew. Chem., Int. Ed., 2013, 52, 1777 CrossRef CAS PubMed;
(c) T. Nishimura, A. Ashouri, Y. Ebe, Y. Maeda and T. Hayashi, Tetrahedron: Asymmetry, 2012, 23, 655 CrossRef CAS PubMed.
- T. Nishimura, A. Kasai, M. Nagaosa and T. Hayashi, Chem. Commun., 2011, 47, 10488 RSC.
- T. Nishimura, Y. Takiguchi and T. Hayashi, J. Am. Chem. Soc., 2012, 134, 9086 CrossRef CAS PubMed.
- T. Nishimura, H. Makino, M. Nagaosa and T. Hayashi, J. Am. Chem. Soc., 2010, 132, 12865 CrossRef CAS PubMed.
-
(a) K. Sasaki and T. Hayashi, Tetrahedron: Asymmetry, 2012, 23, 373 CrossRef CAS PubMed;
(b) T. Nishimura, Y. Takiguchi, Y. Maeda and T. Hayashi, Adv. Synth. Catal., 2013, 355, 1374 CrossRef CAS.
- S. Abele, R. Inauen, D. Spielvogel and C. Moessner, J. Org. Chem., 2012, 77, 4765 CrossRef CAS PubMed.
- R. Brönnimann, S. Chun, R. Marti and S. Abele, Helv. Chim. Acta, 2012, 95, 1809 CrossRef.
- For reviews on rhodium-catalyzed asymmetric conjugate addition, see:
(a) P. Tian, H.-Q. Dong and G.-Q. Lin, ACS Catal., 2012, 2, 95 CrossRef CAS;
(b) D. V. Partyka, Chem. Rev., 2011, 111, 1529 CrossRef CAS PubMed;
(c)
G. Berthon and T. Hayashi, in Catalytic Asymmetric Conjugate Reactions, ed. A. Córdova, Wiley-VCH, Weinheim, Germany, 2010, Ch. 1, p 1 Search PubMed;
(d) H. J. Edwards, J. D. Hargrave, S. D. Penrose and C. G. Frost, Chem. Soc. Rev., 2010, 39, 2093 RSC;
(e) J. B. Johnson and T. Rovis, Angew. Chem., Int. Ed., 2008, 47, 840 CrossRef CAS PubMed;
(f) T. Hayashi, Pure Appl. Chem., 2004, 76, 465 CrossRef CAS;
(g) S. Darses and J.-P. Genet, Eur. J. Org. Chem., 2003, 4313 CrossRef CAS;
(h) T. Hayashi and K. Yamasaki, Chem. Rev., 2003, 103, 2829 CrossRef CAS PubMed;
(i) K. Fagnou and M. Lautens, Chem. Rev., 2003, 103, 169 CrossRef CAS PubMed;
(j) C. Bolm, J. P. Hildebrand, K. Muñiz and N. Hermanns, Angew. Chem., Int. Ed., 2001, 40, 3284 CrossRef CAS.
- A. van Der Ent and A. L. Onderdelinden, Inorg. Synth., 1990, 28, 90 CrossRef CAS.
Footnote |
† Electronic supplementary information (ESI) available: Details of HPLC analysis of the addition products with chiral stationary phase columns. See DOI: 10.1039/c4qo00292j |
|
This journal is © the Partner Organisations 2015 |