DOI:
10.1039/D2RA07863E
(Review Article)
RSC Adv., 2023,
13, 8606-8629
Cancer nanomedicine: a review of nano-therapeutics and challenges ahead
Received
9th December 2022
, Accepted 7th March 2023
First published on 14th March 2023
Abstract
Cancer is known as the most dangerous disease in the world in terms of mortality and lack of effective treatment. Research on cancer treatment is still active and of great social importance. Since 1930, chemotherapeutics have been used to treat cancer. However, such conventional treatments are associated with pain, side effects, and a lack of targeting. Nanomedicines are an emerging alternative due to their targeting, bioavailability, and low toxicity. Nanoparticles target cancer cells via active and passive mechanisms. Since FDA approval for Doxil®, several nano-therapeutics have been developed, and a few have received approval for use in cancer treatment. Along with liposomes, solid lipid nanoparticles, polymeric nanoparticles, and nanoemulsions, even newer techniques involving extracellular vesicles (EVs) and thermal nanomaterials are now being researched and implemented in practice. This review highlights the evolution and current status of cancer therapy, with a focus on clinical/pre-clinical nanomedicine cancer studies. Insight is also provided into the prospects in this regard.
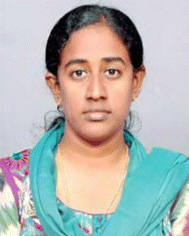 M. Joyce Nirmala | Dr M. Joyce Nirmala is the Principal Scientist in the Department of Chemical Engineering at IIT Madras. She did her Postdoctoral fellowship from the same institution. She obtained her Master's degree in Biotechnology and PhD in Nanobiotechnology (2014) from VIT University, Tamil Nadu, India. She did her Bachelor's degree from Christian Medical College and Hospital, Vellore, India. She was awarded as “Young Scientist” by DST-SERB, Government of India (2015–18). Her research is focused on developing cost-effective and safe nanomedicine for cancer therapy. |
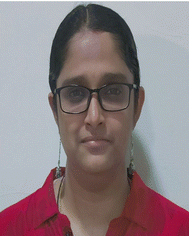 Uma Kizhuveetil | Dr Uma Kizhuveetil is a Postdoctoral research fellow at the Indian Institute of Technology Madras. She completed her PhD from the Department of Biotechnology, Indian Institute of Technology Madras. |
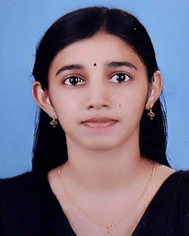 Athira Johnson | Dr Athira Johnson is currently working as Postdoctoral fellow at the Indian Institute of Technology Madras, Tamil Nadu, India. She received both her Bachelors and Master's degree from Mahatma Gandhi University, Kottayam, Kerala, India, and her PhD from National Taiwan Ocean University, Keelung, Taiwan in the field of Nanomedicine. Her current research topic includes formulation, characterization and in vitro drug release of drug delivery system for cancer therapy. |
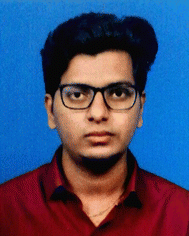 Balaji G | Mr Balaji is a Project Associate at the Department of Chemical Engineering, IIT Madras. He completed his Master's degree in Biotechnology from Bharathidasan University, Tiruchirapalli, Tamil Nadu, India. His current research focuses on the microemulsions. His research interests are Aquatic toxicology and Nano-biotechnology. |
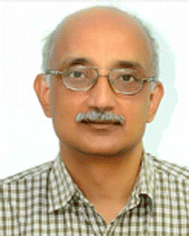 Ramamurthy Nagarajan | Dr Nagarajan is Alumni Community Chair Professor in the Department of Chemical Engineering at IIT Madras. He obtained his PhD from Yale University in 1986. From 1986–1988, he served as Research Faculty in the Department of Mechanical and Aerospace Engineering at West Virginia University, Morgantown, WV. From 1988–2003, he was a Senior Technical Staff Member with IBM Storage Systems' Development Laboratory in San Jose, CA. He took up his position as Professor at IIT Madras in February 2004. His teaching and research endeavors are focused on cleanroom processes, nano-technology and ultrasonic process-intensification. |
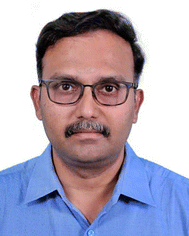 Vignesh Muthuvijayan | Dr Vignesh Muthuvijayan is a Professor in the Department of Biotechnology, Bhupat and Jyoti Mehta School of Biosciences, Indian Institute of Technology Madras. He graduated with a PhD in Chemical Engineering from Oklahoma State University (2009). His lab focuses on developing multifunctional bioactive materials for tissue engineering, designing self-assembling systems for drug delivery, and engineering polymer surfaces for improving long-term patency of implants. |
1. Introduction
Cancer is a major health concern even today, with the millions of deaths it accounts for every year.1 The term “cancer” was first proposed by the Greek physician Hippocrates due to the crab-like appearance of the finger-like projections generated from it.2 Another Greek physician, Galen used the term ‘oncos’, which means swelling, to describe tumors.3 Cancer has the hallmarks of uncontrolled proliferation, lack of differentiation into mature cells, cell death, altered redox, stress, and metabolic regulation as well as the ability to spread to other parts of the body (metastasis).4 These hallmarks of cancer alter the microenvironment of the tumor site, and actively route the energy and nutrient supply for its growth, rather than for bodily functions.5 This also results in heterogeneity and mixed populations of cells, each of which has a different response to various therapeutic approaches.6
Many therapeutic methods have been tested against cancer with varying levels of success,7 the most common ones being chemotherapy, radiotherapy, and surgery.8 Chemotherapy started in the early 1930s with the use of chemicals against tumor cells, prominently with the use of nitrogen mustard against leukemia,9 and alkylating drugs such aschlorambucil.10 However, the cure for cancer is still evasive, probably owing to the diverse population of cells present in the tumor environment, and the inability of the above-mentioned methods to effectively regulate the tumor microenvironment and the various hallmarks of cancer.11 Nowadays, different types of cancer are observed among the population, indicating a high death rate and increasing live cases among children. The mortality rate of several cancers and extrapolated future projections are shown in Fig. 1. According to World Health Organization (WHO), there were 10 million deaths attributable to cancer in 2020, and each year approximately 400
000 children develop cancer. It is expected that by 2040, new cases will rise to 29.5 million with 16.4 million cancer-related deaths.12
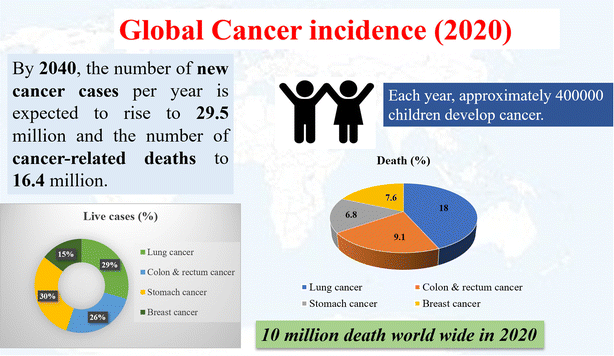 |
| Fig. 1 The global incidence of cancer in 2020. Source: World Health Organization. | |
Nano-oncology is an emerging strategy in cancer therapy that involves the use of nano-dimensional therapeutic materials as anticancer agents, and it has generated promising results in research and clinical trials.13 This is a field that has grown tremendously in the past two decades with the development of new approaches not only for cancer treatment but also for diagnosis and prevention.14 Nanotechnology has become an innovative method to treat various diseases, owing to its high potential and treatment efficacy in different cancer types. Cancer nanomedicine has wide applications in effective tumor therapy, based on targeting, imaging, viral nanoparticles, and enhanced delivery.15
In this review, we present the current status of the research in nano-oncology. Also, a review of promising nano-oncological products in various stages of clinical and preclinical trials is provided.
2. Origin and history of cancer nanomedicine
The early history of nanomedicine may be dated back to ancient times when colloidal gold particles were used for medicinal purposes.16 Ancient medical literature has a record of drug preparation methods that use pulverization of medicines to obtain required consistencies, a process later shown to be a nano-scale preparation technique.17 Nanomedicine in its current form has been considered a possibility ever since the concept of nanotechnology was first introduced in 1959 by Richard Feynman in his Caltech talk, “There is plenty of room at the bottom”. He mentioned that it would be possible to arrange the atoms as desired. Nanomedicine can be defined as nanotechnology, which deals with the size range of 1 to 100 nm, applied to health and medicine.18 In 1999, Robert A Freitas introduced the term “nanomedicine”, and it has been used extensively in technical literature since then. Nanomedicine has been sought due to the deficiencies that exist in treating cancer with drugs of low specificity, rapid drug clearance, biodegradation, and limited targeting.19 Nanocarriers were proposed as a superior alternative with targeted drug delivery to the tumor tissue and controlled release of the intended drug.20 Nanoparticles or nanomaterials which are innovated and modified are shown over a timeline in Fig. 2. Cancer therapy based on nanoparticles began with the development of doxorubicin-loaded liposomes for treating breast cancer. Later, polymers and dendrimers came into use. Between 2000 to 2015, siRNA molecules with different nanoparticles and solid lipid nanoparticles were developed for targeted therapy and treatment efficacy, respectively.21 Then, gold nanoparticles and quantum dots were deployed in cancer therapy, especially for bio-imaging. In the future, it is expected that better treatment can be achieved by using Nanobots.22,23
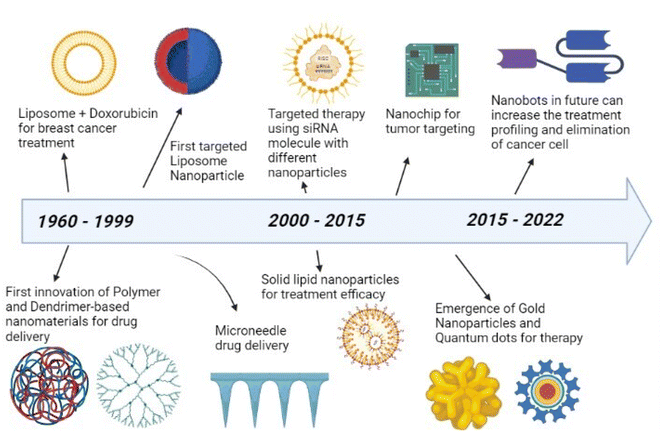 |
| Fig. 2 Timeline of cancer nanomedicine. | |
Nanotechnology has enabled applications in a wide range of fields such as Chemistry, Biology, Physics, Engineering, and Medicine. In the clinical industry, it has facilitated the development of delivery systems of drugs for complicated diseases.24–26 Nanomedicine is a pioneer field of nanotechnology that deals with the development of powerful techniques for treating diseases and for delivering certain biological compounds for treatment. An injection is the only method of administration for biologics such as peptides, therapeutic proteins, and antibodies (with a few exceptions). Nano-drug delivery has intensified efforts for the development of painless injections, targeted treatment, and an increased ability of the drug to penetrate the BBB (Blood Brain Barrier).27 In this century, researchers have advanced the uses of nanotechnology in medicine, with the usage of liposome-mediated drug delivery options, and the encapsulation of drugs such as Doxorubicin and Cabilivi™ as nano-sized drugs. Lipid nanoparticles show promising results in mediating synthetic compounds into nano-drugs.28 Loading mRNA and therapeutic proteins as a nano-drug enhances the clinical applications of nanomedicine. Recently, the application of nanomedicine in immunomodulation and immunotherapy has enabled the use of nanoparticles such as acid-base nano-constructs, extracellular vesicles, and virus-modified exosomes.29 There are safety concerns with attempts to use nano-medical research in clinical settings, necessitating a better understanding of the crucial characteristics that influence how nanomaterials interact with tissues and organs.30
When compared to conventional anticancer therapies, targeted nano-therapy has proved to be a more effective approach with minimum toxicity, enhanced permeability, and retention. It increases the plasma half-life of the nano-size drugs and alters biodistribution, resulting in differential accumulation of nanoparticles in the tumor tissues.31 The increased plasma half-life of nanoparticles happens when their size exceeds the limit of the renal excretion threshold and limits their clearance. The differential accumulation of nanoparticles in tumor tissues results in a higher concentration of the nano-sized drug in these tumor tissues than in the plasma or other organs; the accumulation is time-dependent and can be reproduced in tumors of different sizes.32 This phenomenon results in prolonged therapeutic effects in addition to targeting when the pharmacological effects and plasma concentration are synergized.33
Interest in the application of nanotechnology has increased in cancer therapy because of its advantages in drug delivery, diagnosis, and imaging.34 Several therapeutic nanoparticle platforms, such as albumin NPs, liposomes, and polymeric micelles, have been approved for cancer treatment.35 Each has its specific characteristics which make them advantageous for certain uses even while posing limitations in certain others.36,37
3. Mechanism of targeting by nano drug vehicles
A very important criterion for the selection of a nanomedicine formulation for cancer therapy would be its efficiency in targeting the cancer tissue in a specific manner and having minimal side effects on the normal tissue. The various nano-formulations used to deliver anticancer drugs to tumor sites use varying targeting mechanisms for this purpose. The mechanism of drug delivery and the advantages of nanocarriers will vary by carrier. Nanocarriers directly deliver therapeutic agents to the bloodstream and reach the targeted area. They then induce DNA damage by reactive oxygen species (ROS) overproduction. This may finally lead to apoptosis and cell death.38,39
Two major types of targeting methods are used for nano-based drug delivery: passive and active. In passive method (Fig. 3), the properties of the tumor site are used to concentrate the nano-vehicles to the tumor site. The major factors used for this are Enhanced Permeability and Retention (EPR) and Tumor Micro Environment (TME) properties. Unlike normal cells, tumor cells induce neovascularisation due to high proliferation and large pores in the vascular walls that favor passive targeting.40 Due to imperfect angiogenesis, particles can reach the tumor site and accumulate. Poor lymphatic drainage also increases particle retention resulting in EPR on tumors.41 However, the high interstitial fluid pressure inside the tumor microenvironment reduces the uptake and homogeneous distribution of nanoparticles.42
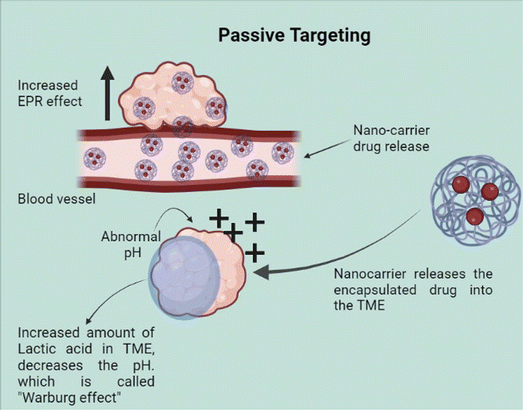 |
| Fig. 3 Passive targeting. | |
Although the enhanced permeability and retention effect of tumor tissue causes nanoparticles to preferentially accumulate there to a greater extent than in normal tissue,43 the abnormal and dysfunctional tumor microenvironment frequently leads to the heterogeneous distribution of nanoparticles44 which primarily reside in the perivascular area and tumor periphery.45 Therefore, many nanocarriers also utilize the TME properties such as acidic pH, higher redox potential, and differential secretion of lytic enzymes for uniform drug delivery throughout the tumor. Active targeting (Fig. 4) also utilizes the properties of the tumor cells such as the cell surface receptors expressed by the cancer cells. However, the targeting is achieved by the use of various molecules hybridized along with the carrier to specifically target these. Here, we look into the different modes of targeting used by the various nano-formulations and some of their advantages as well as disadvantages.
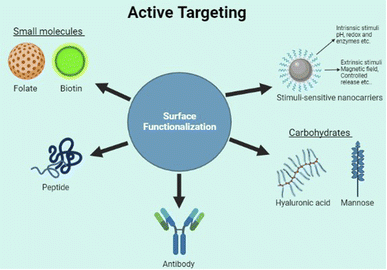 |
| Fig. 4 Active targeting. | |
In general, passive targeting is based on the diffusion mechanism and it is affected by various factors such as size, shape, and surface properties. It is noted that high bioavailability and reduced renal clearance can be achieved with 40 to 400 nm by increasing the circulation time. Likewise, maintaining the particle size between 50 to 200 and with a rigid and spherical appearance improves the circulation time and also reduces kidney clearance.46 Tumor cells were characterized by irregular neovascularization, higher expression of inflammatory factors, and lack of an efficient lymphatic drainage system.47 Because of the leaky vasculature structure and poor lymphatic drainage, nanoparticles will eventually enter into tumor tissues and retain them in the tumor bed due to long circulation time. In tumor cells, EPR effect helps to improve drug accumulation, however, in normal tissues, nanoparticles will be cleared by the mononuclear phagocyte system (MPS) or by glomerular filtration of the kidney. Certain barriers such as abnormal tumor vasculature, growth-induced solid stress, and solid stress from the abnormal stromal matrix will hinder the delivery of nanosized drugs.48 Gradually, acidic and hypoxic conditions in the tumor cells, heterogeneous perfusion, and elevated interstitial fluid pressure prevent nanoparticle penetration.49 These issues can be solved by improving drug delivery by taking the advantage of EPR effect and TME properties.
Maintaining the size of the nanoparticle is better to improve the EPR effect. In addition, the neutral or negative charge of the particles increases the circulation time and drug accumulation by improving plasma half-lives. Applying some adjuvants like nitric oxide donors to improve the EPR effects also can be done.47 EPR effect is affected by certain factors such as extravasation, diffusion, and convection in the interstitium, tumor vasculature, and biology, tumor extravascular environment, and physiochemical factors. Also, EPR is heterogenous, in terms of tumor blood flow, hypoxic areas, vascular permeability, extravasation, and penetration.46 When the nanoparticles enter the body, it passes through different stages including circulation, endocytosis, accumulation, etc. Besides, these particles are highly prone to the opsonization process and because of it; a protein corona will form over the nanoparticles depending on the nanoparticle's characteristics. To avoid this, hydrophilic polymers (PEGylation) with stealth properties can be used to reduce the absorption of opsonins to the nanoparticles. Another strategy is the silencing or depleting of Kupffer cells to escape from the RES system. These cells are specialized macrophages that facilitate the uptake of foreign materials.50 Recently, a reduction in tumor hypoxia and modulation of polyethyleneimine cytotoxicity by using PEGylated Prussian blue nanoparticles were reported. It is a dual-enhanced photodynamic therapy with an oxygen self-supply property. Besides, better therapeutic efficacy was observed in breast cancer cells and tumor-bearing mice after laser irradiation.51 In another study, evident cell apoptosis and necrosis were observed in PEGylated nanographene oxide-treated cancer cells and this study is an example of combination therapy.52 A recently published paper examined an iron-dependent regulated cell death process (ferroptosis) by liposomes embedded with PEG-coated 3 nm γ-Fe2O3 nanoparticles in the bilayer. These particles promote hydroxyl radical generation and cause efficient lipid peroxidation. The addition of doxorubicin improves the chemotherapeutic effect and traceable magnetic resonance imaging and pH/ROS dual-responsive drug delivery were also visible during the study.53
In detail, tumor development is affected by genetic/epigenetic and tumor microenvironment changes (TME). TME consists of tumor cells, stromal fibroblasts, endothelial cells, immune cells, and extra cellular matrix. Disruption of the interaction between stromal cells and tumors is one of the ways to combat tumor progression. Several strategies such as carcinoma-associated fibroblast (CAF) depletion, ECM targeting, anti-angiogenic therapy, exosome/circulating tumor cells (CTCs) targeting, and immune modulation/reprogramming can be done for this purpose.54 Designing a pH-responsive drug delivery system is also one of the ways to target tumor cells. Because of the Warburg effect, a large amount of lactic acid will release and it will help the cells to grow in low oxygen and acidic environment. Designing a pH-responsive nanoparticle, which is stable at physiological pH and degradable at tumor pH is the right track to target tumor cells.55 Along with this, light-sensitive drug delivery system design is also applicable to cancer treatment. Over expression of matrix metalloproteinase (MMP) is seen in most cancer cells and it can be used as a tumor-specific trigger to tune the size of nanomedicines to enhance tumor penetration. Another possible way is degrading the dense ECM to enhance drug penetration.56 Recently, researchers developed gelatin/nanochitosan/doxorubicin nanoparticles for cancer therapy. While reaching the tumor site, MMP-2 degrades gelatin from 178 nm GND to release smaller 4 nm nanochitosan/doxorubicin (ND) nanoparticles for deep tumor penetration and efficient tumor cell endocytosis. Finally, doxorubicin is released due to low pH and MMP-2 activity. In this study, the biocompatibility of the drug was also reported in a mouse tumor bearing model.57 An aptamer-decorated hypoxia-responsive nanoparticles (DGL)n@Apt co-loading with gemcitabine monophosphate and STAT3 inhibitor HJC0152 was developed to evaluate its tumor penetration in pancreatic ductal adenocarcinoma cells. This particle can reverse its charge in TME and reduce the size triggered by hypoxia.58
Immunotherapy is also considered as a promising technology to treat cancer. This technique involves the modulation of cell-specific immune responses toward the tumor. TME contains immunosuppressive cells like tumor associated macrophages (TAMs). So, cancer therapy has been improved by targeting TAMs.59 Solid tumors contain up to 50% of macrophages and it produces cytokines while recruiting to tumor site by the microenvironment. This process may depend on lactic acid level, inflammation, and local anoxia.60 M1 (proinflammatory and antitumor) and M2 (anti-inflammatory and pro-tumor) are the two subsets of TAMs61 It is noted that the interaction of immune cells with nanoparticles activates the immune system and thereby can improve therapeutic effects. Tumor growth can be reduced by depolarizing the M2 type to the M1 type and this TAM can serve as a drug depot for the accumulation of nanoparticles. So that, local delivery is also achievable.62 Recently, the anti-tumor activity of polyethylene glycol-conjugated gold nanoparticles was reported. In this study, nanoparticles suppressed TAMs M2 polarization via induction of lysosome dysfunction and autophagic flux inhibition in both in vitro and in vivo models.63 In another study, gene shifts in TAMs towards M1 type and also induced apoptosis in cancer associated fibroblasts were observed upon treating with a biodegradable nanoparticle called ONP-302 during in vivo analysis. These particles are negatively charged and were developed from poly(lactic-co-glycolic acid) (PLGA).64
3.1. Liposomes
Made from cholesterol and other natural or synthetic phospholipids,65 liposomes are one of the most commercially successful nano-drug delivery platforms.66 Both oral delivery and injection methods are applicable in the case of liposome-based drug delivery systems.67 FDA-approved intravenous injection is a primary route of administration for various drugs. Besides, subcutaneous, intradermal, intraperitoneal, and intramuscular administrations are also available. The mechanism of liposome drug delivery action relies on the accumulation, uptake, and release of drugs.68 The interactions with drug and tumor sites happen via passive and active targeting.69 Passive targeting mostly uses the enhanced permeability and retention effect observed in tumor microenvironments to improve the retention of liposomes at the tumor sites.70 However, active targeting by surface functionalization has improved the drug-targeting ability of liposomes.
Active targeting of liposomes for drug delivery to tumor sites uses surface functionalization of the lipids surface using various methods. The use of antibodies, small molecules, peptides, or carbohydrates has been tested for surface functionalization.71 Antibodies, with their specific targeting properties, can be used for targeting cancer-specific surface antigens such as Melanoma cell adhesion molecule (MCAM),72 Her 2 receptor, CD44, and growth factor receptors such as VEGFR and EGFR.71 Surface functionalization using small molecules such as folate, estrone, and anisamide are also effective in targeting tumor surface receptors for these molecules. Carbohydrates and proteins such as mannose and Beta FGF which can bind to specific cell surface receptors have also been investigated as potential surface active molecules.
Along with surface molecule based targeting, another method used to target liposomes is the stimuli-sensitive cleavage of coating polymers used to reduce the phagocytotic clearance of liposomes. Polymers such as PEG used for coating liposomes increase their circulation and may have reduced targeting properties. To improve the cancer site targeting by these coated liposomes, various stimuli based cleaving have been used. These methods also use the tumor microenvironment properties such as altered pH, redox potential, and secreted enzymes to induce cleavage of the surface coating, thereby inducing preferential drug release at the tumor site.71
3.2. Extracellular vesicles
Extracellular Vesicles (EVs), released by prokaryotic and eukaryotic cells into the extracellular environment, have recently gained prominence as cancer nano-drug vehicles. EVs may be actively produced by cells in a constitutive or inducible manner, and are naturally nano-scale to micron-sized membrane vesicles encased by phospholipid bilayers.73 EVs originate from the endosomal compartment. Their biochemical content consists of lipids, proteins, and microRNA, making them a promising candidate for targeted therapy.
EVs have favorable cellular uptake properties due to their biochemical composition and also can be targeted to tumor sites using surface functionalization.74,75 The inherent properties of EVs being able to cross the blood–brain barrier also make them promising drug delivery platforms for brain tumors. Also, engineered EV shows increased pharmacokinetic ability, drug load stability, and targeted therapy; for example, paclitaxel shows potent anticancer effects in a model of Murine Lewis lung carcinoma pulmonary metastases, and also accumulates in cancer cells upon systematic administration, thereby, improving therapeutic outcomes.76 However, the non-scalability of EV production is a major challenge to be overcome before it can become an attractive commercial nano-drug vehicle.
3.3. Nanoemulsions
Nanoemulsions are water-in-oil or oil-in-water emulsions of nano-dimensions that can be used for drug delivery to tumor sites.77 With the usage of generally recognized as safe (GRAS) oils as vehicles, nanoemulsions have the advantage of reduced side effects.78 Similar to liposomes, nanoemulsions also can utilize the EPR effect to passively target the tumor site. However, recently surface functionalization of the outer oil portion of the emulsions are being studied to improve the drug targeting to the specific tumor.79 Active targeting methods for nanoemulsions also use peptides such as RGD peptide and transferrin, small molecules such as biotin and folate, or certain antibodies specific to tumor surface antigens.80 Novel targets such as lysophosphatidic acid receptor is also being studied to improve the drug targeting to specific tumors.79
Advantages of nanoemulsions in their versatility for the drug load promoting combinatorial drug use, inherent ability to overcome multidrug resistance, and the potential ability to use the drug itself as the emulsion without the need for a carrier make them interesting choices for cancer therapy.
3.4. Dendrimers
Dendrimers are artificial nanocarriers made of radially symmetric arrangements of monomers81 with a tree or branch-like appearance.82 They have high surface functionalization and targeting properties owing to their versatility of design. As they can be designed specifically to suit each target, dendrimers are one of the nanocarriers with the easiest surface functionalization properties. The ability to precisely regulate the size of the dendrimers also makes it possible for them to be passively targeted to tumor sites via EPR. Dendrimers also can be hybridized into other types of nanocarriers, such as encapsulating them in other polymer shells83 making it a versatile drug delivery platform for cancer therapy.
Dendrimers have high carrier capacity for the drugs84 and can be specifically engineered for various drug release mechanisms. Some of the commonly used ones include variations in the number of terminal groups and the use of degradable spacers and pH-sensitive linkages.85 The surface functionalization methods using vitamins, antibodies, peptides, etc., have also been able to overcome the inherent drawbacks of dendrimer-mediated drug delivery such as rapid systemic elimination.
3.5. Nanoparticles from inorganic materials
Nanoparticles from inorganic materials are the other kind of drug delivery system widely used for cancer treatment. The commonly used materials for these nanoparticles include gold and silver nanoparticles, carbon quantum dots, carbon nanotubes, metal oxide particles, and mesoporous silica nanoparticles. They are well-known for their precise targeting, good pharmacokinetics profile, encapsulation of poorly soluble drugs, and diagnostic applications. The size and characteristics of nanoparticles are very important when designing drug delivery systems.86 Normally, nanoparticles act as a carrier system to incorporate active ingredients, and they release the active compound into the targeted site to induce cell death.
3.5.1. Gold/silver nanoparticles. Nanoparticles of gold and silver are capable of delivering small as well as large drug molecules to the tumor site. The major mode of drug targeting involves the use of EPR as well as tumor micro-environmental properties such as altered redox potential and pH.87 Being metallic, they can also be used for hyperthermic treatment using an external heat source such as microwave, post targeting to the tumor site. However, aggregation and higher cytotoxicity to healthy cells by these metal particles reduce their use unless hybridized with certain polymers such as PEG.88
3.5.2. Magnetic nanoparticles. Magnetic nanoparticles are used for cancer therapy, especially for hyperthermic treatment including metallic, or metallic shells and oxides. Usually, a magnetic nanoparticle is prepared from pure metal or in combination with polymers. Delivery of magnetic nanoparticles into the targeted site via injection using catheters or hypodermic needles has been demonstrated.89 A heat source such as a microwave can be used to induce hyperthermia at the specific target, thereby locally increasing the temperature to around 42 °C. Higher temperature leads to the death of cancerous cells due to their leaky vascular nature, without a significant effect on the normal cells. Apart from hyperthermia, magnetic nanoparticles have been used for theranostic, photodynamic therapy, photothermal ablation therapy, biosensors, drug delivery, and MRI imaging90 because of the super-paramagnetic feature of iron oxide nanoparticles.91
3.5.3. Carbon quantum dots. Carbon quantum dots (CDQs) are fluorescent carbon nanoparticles with imaging and drug delivery applications in cancer.92 They have high targeting capabilities due to their ease of surface functionalization, and therefore, can be used with multiple targeting molecules on the surface. The fluorescence properties of CDQs render them highly capable of bio-imaging, adding to their overall theranostic value.93 The low toxicity, high biocompatibility, ease of targeting, and added diagnostic functionalities of CDQs make them one of the most promising emerging nano-drug carrier platforms.
3.6. Solid lipid nanoparticles (SLN)
SLNs are a colloidal drug delivery system in which the liquid lipid is replaced by solid lipid.94 Generally, as the name indicates, solid lipid nanoparticles consist of a solid lipid, emulsifier, and water. Fatty acids, steroids, waxes, triglycerides, and partial glycerides are commonly used as lipids. SLN can be administered through various routes such as parenteral, oral, rectal, ophthalmic, and topical. Solid solution and core–shell models are the two types of SLNs models.95 It is observed that SLNs have higher drug release due to their high surface area, and the homogeneous distribution of the drugs will lead to slow release. In addition to this, the high mobility of the drug and crystallization behavior of lipid carriers also facilitates faster release.96 SLN is delivered into the targeted area by passive, active, and co-delivery mechanisms. Passive targeting is mainly achieved due to EPR effects, and active targeting is by recognition of receptors or transporters over-expressed on the surface of tumor cells. The co-delivery method is applied by delivering two compounds to the drug delivery system.97 Enhanced pharmacokinetics and efficacy against multidrug-resistant cancer cells were observed in cancer cell lines such as MCF-7, A549, and MDA-MB-231 when treated with vorinostat-loaded solid lipid nanoparticles.98
4. Status of approved drugs and those under clinical trials
Clinical trials are the last stage of drug development wherein the drug formulations are tested on humans to determine their actual efficacy and side effects, to obtain approval for commercial use of the drug formulation.99 There are various phases for the clinical trial of a drug and all of them have to be cleared sequentially for the drug to be approved for medical use against the disease. The duration of each phase, the conditions involved, and the number of people the drug is tested on at each phase is decided by the drug regulatory authority. However, there mostly involves four phases of clinical trials before a drug is approved for medical use.
Phase I of a clinical trial involves less than a hundred people and may include healthy people as control groups as this phase is to determine the safety and dosage of the drug. However, for cancer related drug trials, it is mandatory that the group includes people with that particular type of cancer.99 After clearing phase I, the drug can enter phase II clinical trials which is conducted on a few hundred people with a particular cancer. The objective of this phase of the clinical trials is to determine the efficacy and side effects. Therefore, it is common to have double blind studies with placebo control groups for this phase. The next phase also is aimed at determining the side effects. However, the focus is on long term and less common side effects and therefore include a larger study group of up to a few thousand people and can go on up to 3–4 years. Upon successful completion of phase III clinical trials, the drug formulation can be approved and may be marketed for medical use. However, the monitoring continues as phase IV wherein any and all adverse reactions reported are investigated for determining the overall safety and efficacy of the drug.
4.1. Approved nano-formulations for cancer therapy
Nano-formulations have been marketed for medical use against cancer since early 90's with the polymer–protein conjugate Zinostatin stimalamer100 being approved in Japan against hepatocellular carcinoma and the pegylated liposome Doxil® which was marketed as an anti-ovarian cancer drug formulation in the United States of America.101 With time, many other types of nano-formulations including liposomes, metal and metal oxide nanoparticles, polymeric micelles, and lipid nanoparticles have been developed and cleared for medical use by multiple agencies all over the world, with many more under various stages of clinical and preclinical trials. Table 1 gives a list of nano-formulations and the drugs used in them, which have already been approved for medical use.
Table 1 Approved nanomedicine drugs in the market for cancer treatment
S. No. |
Product |
Drug |
Nanotechnology platform |
Cancer type |
Approval |
Advantages |
Toxicity |
1 |
Zinostatin stimalamer |
Styrene maleic anhydride neocarzinostatin (SMANCS) |
Polymer protein conjugate |
Primary unresectable hepatocellular carcinoma |
1994 (Japan) |
Enhanced accumulation and EPR effect |
Slightly toxic (liver dysfunction) |
2 |
Doxil (Caelyx) |
Doxorubicin hydrochloride |
Pegylated liposome |
Ovarian cancer and AIDS-related Kaposi's sarcoma |
1995 (FDA) |
Prolonged drug circulation time, drug loading, tumor targeting |
Long-term use of Doxil® may predispose female patients to oral squamous cell carcinoma |
3 |
DaunoXome |
Daunorubicin |
Liposome |
HIV-related Kaposi sarcoma |
1996 (FDA) |
No polyethylene coating and slow release into circulation |
Adverse cardiac side effects |
4 |
Lipo-Dox |
Doxorubicin |
Liposome |
Kaposi's sarcoma, breast and ovarian cancer |
1998 (Taiwan) |
Longer half-life, lower clearance, extended blood circulation time, and better tolerance |
Mild myelosuppression and other nonhematological toxicities |
5 |
Myocet |
Doxorubicin |
Liposome |
Breast cancer |
2000 (EMA) |
Less cardiotoxicity and equal anticancer activity |
Relative instability |
6 |
Mepact |
Muramyl tripeptide phosphatidyl ethanolamine |
Liposome |
Non-metastatic osteosarcoma |
2009 (EMA) |
Longer half-life and less toxic |
Chills, fever, headache, myalgias, and fatigue |
7 |
Lipusu |
Paclitaxel |
Liposome |
Breast cancer, non-small-cell lung cancer |
2013 (EMA) |
Modulate paclitaxel toxicity without affecting antitumor activity |
Nausea, vomiting, dyspnea, peripheral neuritis |
8 |
NanoTherm |
Fe2O3 |
Nanoparticles of superparamagnetic iron oxide coated with amino silane |
Glioblastoma, prostate, and pancreatic cancers |
2013 (EMA) |
High blood circulation time and tumor uptake (EPR), heat production under stimulation with EMF, and teranostic properties |
Moderate adverse effect |
9 |
Ameluz |
5-Aminolevulinic acid |
Gel containing 5-aminolevulinic acid, E211, SoyPC, and PG |
Superficial and/or nodular basal cell carcinoma |
2011 (EMA) |
Sustained release and low toxicity |
Transient pain and erythema |
10 |
Depocyt |
Cytarabine |
Liposome |
Lymphomatous malignant meningitis |
1999 (FDA) |
Improve delivery and reduce toxicity |
Arachnoiditis and neurotoxicity |
11 |
Genexol-PM |
Paclitaxel |
Polymeric micelle |
Non-small cell lung cancer |
2006 (South Korea) |
Controlled drug release and targeted drug delivery |
Neuropathy, myalgia, and neutropenia |
12 |
Nanoxel |
Docetaxel |
Polymeric micelle |
Breast and ovarian cancers, NSCLC, and AIDS-related Kaposi's sarcoma |
2006 (India) |
Good efficacy and pharmacokinetic properties with less toxicity |
Myalgia, nausea, anemia, paresthesia, alopecia, diarrhea, and vomiting |
13 |
Marqibo |
Vincristine |
Liposome |
Leukemia |
2012 (FDA) |
No polyethylene coating and slow release into circulation |
Drug toxicity and adverse side effect |
14 |
Onivyde |
Irinotecan |
Liposome |
Pancreatic cancer |
2015 (FDA) |
Enhanced delivery and reduced systemic toxicity |
Diarrhea, nausea, vomiting, neutropenia, and febrile neutropenia |
15 |
Vyxeos |
Daunorubicin and cytarabine |
Liposome |
Acute myeloid leukemia (AML) |
2017 (EMA) |
Improved overall survival |
Febrile neutropenia, fatigue, pneumonia, hypoxia, hypertension, bacteremia, and sepsis |
16 |
Oncaspar |
L-Asparaginase |
PEGylated conjugate |
Acute lymphoblastic leukemia |
2006 (FDA) |
Improved stability of drug load |
Venous thromboembolism, pancreatitis, and hyperglycemia |
17 |
DPH107 |
Paclitaxel |
Lipid nanoparticles |
Advanced gastric cancer |
2016 (Korea) |
Absorption without the need for P-glycoprotein inhibitors or Cremophor EL |
Neutropenia and leukopenia |
18 |
NBTXR3 (Hensify) |
Hafnium oxide nanoparticles stimulated with external radiation to enhance tumor cell death via electron production |
Hafnium oxide nanoparticles |
Locally advanced squamous cell carcinoma |
2019 (CE Mark) |
Radiotherapy enhancer |
Injection site pain, hypotension, and radiation skin injury |
19 |
Apealea |
Paclitaxel |
Polymeric micelles |
Ovarian, peritoneal and fallopian tube cancer |
2018 (EMA) |
Improve progression-free survival in combination with carboplatin |
Neutropenia, diarrhea, nausea, vomiting, and peripheral neuropathy |
20 |
Ontak |
Denileukin diftitox |
Recombinant DNA derived cytotoxic protein |
Cutaneous T cell lymphoma |
1999 (FDA) |
Targeted delivery |
Acute hypersensitivity-type reactions, asthenia, nausea/vomiting (which led to dehydration in some patients), and infectious |
21 |
Eligard |
Leuprolide acetate |
Polymeric nanoparticles |
Advanced prostate cancer |
2002 (FDA) |
Controlled release and longer circulation time |
Hot flushes followed by malaise/fatigue testicular atrophy, dizziness, gynaecomastia and nausea |
22 |
Abraxane |
Paclitaxel |
Protein carrier |
Various cancers including metastatic and pancreatic cancers |
2005 (FDA) |
Overcomes very low solubility of paclitaxel |
Non-specific binding of paclitaxel to albumin |
23 |
Kadcyla |
DM1 |
Trastuzumab, covalently linked to DM1 via the stable thioether linker MCC |
HER2+ breast cancer |
2013 (FDA, EMA) |
High blood circulation time, tumor uptake (EPR), selectivity and less toxicity |
Nausea, fatigue, thrombocytopenia, headache, constipation, diarrhea, elevated liver enzymes, anorexia, and epistaxis |
24 |
Pazenir |
Paclitaxel |
Paclitaxel formulated as albumin bound nanoparticles. Powder for dispersion for infusion |
Metastatic breast cancer, metastatic adenocarcinoma of the pancreas, non-small cell lung cancer |
2019 (EMA) |
High solubility, blood circulation time, tumor uptake (EPR), and less toxicity |
Affects non-cancer cells such as blood and nerve cells |
Doxil® was the first liposome to get approval in the US in 1995101 for the treatment of ovarian cancer and AIDS-related Kaposi's sarcoma. After a year, NeXstar Pharmaceuticals developed daunorubicin-loaded NPs (DaunoXome®) to treat HIV-associated Kaposi sarcoma. In 2000, Myocet® is another formulation that contains doxorubicin
and cyclophosphamide and got EMEA approval for treating metastatic cancer. Later, Marqibo® got FDA approval for treating non-Hodgkin's lymphoma and leukemia. In 2013, Lipusu was developed by incorporating paclitaxel for treating gastric, ovarian, and lung cancers.101 It is understood that the addition of other compounds such as cholesterol and PEG will help attain the desired properties.102 Mifamurtide-loaded liposomes were developed by Takeda Pharmaceutical Limited to treat high-grade non-metastatic osteosarcoma. In 2017, Vyxeos got FDA approval. It is a liposomal formulation of daunorubicin and cytarabine. The investigators experimented on 309 patients with an average age range between 60–75 to treat acute myeloid leukemia (t-AML) or acute myeloid leukemia (AML) with myelodysplasia-related changes (AML-MRC).103 Along with it, Irinotecan-loaded PEGylated liposome (Onivyde) and cytarabine-loaded liposome (DepoCyt) also got approval for pancreatic adenocarcinoma and lymphomatous meningitis treatment respectively.104
Compared to liposomal preparations, other types of approved nano-formulations are lesser in number. However, the focus on the other types of nano-formulations have also been present and a few are approved for clinical use. Styrene maleic anhydride neocarzinostatin (SMANCS) loaded polymer protein conjugate got approval in Japan (1994) for treating renal carcinoma.105 Eligard® is a leuprolide acetate-loaded polymeric nanoparticle that got FDA approval in 2002 for prostate cancer.106 Another formulation is nanoxel® composed of N-isopropyl acrylamide and vinylpyrrolidone monomers and loaded with Doxetaxel. It got approval in India for the treatment of metastatic breast cancer, ovarian cancer, NSCLC, and AIDS-related Kaposi's sarcoma.107 Apealea is another drug that got approval from EMA for treating epithelial ovarian cancer, primary peritoneal cancer, and Fallopian tube cancer. It is a paclitaxel-containing polymeric micellar formulation.108 Ferucarbotran (carboxydextran coated) and Ferumoxide (dextran) coated were two iron oxide nanoparticles that got approval for cell labeling, especially in the USA.109 NanoTherm is an EMA-approved drug for treating glioblastoma, prostate, and pancreatic cancers. It is a nanoparticle of superparamagnetic iron oxide coated with aminosilane. Moderate adverse effects were identified with NanoTherm and it is good to increase blood circulation time and tumor uptake.110
4.2. Nano-formulations under clinical trials
The number of nano-formulations under clinical trials has been increasing in recent years with many trials in phase II and III at present. Even though liposomes and its hybrids still dominate the trials, there are many metal oxide nanoparticles, carbon quantum dots, polymeric micelles, nanoemulsions and other types of nano-formulations have also been gaining interest (Table 2).
Table 2 Nanomedicine drugs under clinical trial for cancer treatment
Name of drug |
Active ingredients/drugs used |
Nanocarrier/formulation type |
Cancer type |
Properties/objectives |
Status |
Reference |
Cetuximab nanoparticles |
Cetuximab and decorated with somatostatin analogue |
Polymeric nanoparticles |
Colorectal cancer |
Targeting somatostatin receptors |
Phase 1 |
NCT03774680 |
Nanoxel |
Paclitaxel |
Nanoparticle |
Advanced breast cancer |
Pharmacokinetic profile |
Phase 1 |
NCT00915369 |
Carbon nanoparticles |
Carbon nanoparticle |
Carbon nanoparticle |
Advanced gastric cancer |
Harvest lymph nodes after surgery |
Phase 3 |
NCT02123407 |
BIND-014 |
Docetaxel |
Nanoparticles |
KRAS positive or squamous cell non-small cell lung cancer |
Second-line therapy |
Phase 2 |
NCT02283320 |
Lurtotecan liposome |
Lurtotecan |
Liposome |
Advanced or recurrent ovarian epithelial cancer |
Chemotherapy |
Phase 2 (completed) |
NCT00010179 |
Nab-paclitaxel plus S-1 |
Nab-paclitaxel and S1 |
Nanoparticle |
Advanced pancreatic cancer |
Efficacy and safety first-line treatment |
Phase 2 |
NCT02124317 |
Fluorescent nanoparticles conjugated somatostatin analog |
Somatostatin analog |
CdS/ZnS core–shell type quantum dots with carboxylic acid-functionalized (QDs-COOH) |
Breast cancer |
Bioimaging |
Phase 1 |
NCT04138342 |
Magnetic nanoparticles |
Iron nanoparticle |
Nanoparticle |
Prostate cancer |
Magnetic thermoablation |
Early phase 1 (completed) |
NCT02033447 |
Lipodox® |
Doxorubicin hydrochloride |
Liposome |
Breast cancer and ovarian cancer |
Bioequivalence study |
Phase 1 |
NCT05273944 |
ABI-007 and gemcitabine |
Gemcitabine and paclitaxel protein-bound particles for injectable suspension (albumin-bound) |
Nanoparticle |
Metastatic breast cancer |
Combination chemotherapy |
Phase 2 |
NCT00110084 |
AGuIX gadolinium-based nanoparticles |
AGuIX |
Gadolinium based nanoparticle |
Centrally located lung tumors and pancreatic cancer |
Safety and efficacy, stereotactic magnetic resonance-guided adaptive radiation therapy |
Phase 1 & 2 |
NCT04789486 |
ABI-007 |
Paclitaxel albumin-stabilized nanoparticle formulation |
Nanoparticle |
Stage IV non-small cell lung cancer |
Safety and tolerance |
Phase 1& 2 |
NCT00077246 |
Paclitaxel albumin-stabilized nanoparticle formulation and carboplatin |
Carboplatin |
Nanoparticle |
Stage IIIB, stage IV, or recurrent non-small cell lung cancer |
Combinational chemotherapy |
Phase 2 (completed) |
NCT00729612 |
Nanotax |
Nanoparticulate paclitaxel |
Nanoparticles |
Peritoneal cancers |
Safety, pharmacokinetics, and preliminary efficacy |
Phase 1 (completed) |
NCT00666991 |
DOTAP:Chol-FUS1 |
fus1 gene |
Liposome complex |
Lung cancer |
Gene transfer therapy |
Phase 1 (completed) |
NCT00059605 |
Nano-QUT |
Quercetin |
PLGA-PEG nanoparticles |
Squamous cell carcinoma |
Therapeutic efficacy |
Phase 2 |
NCT05456022 |
ABI-007 plus capecitabine |
Nanoparticle albumin-bound paclitaxel |
Albumin-bound (Nab) paclitaxel nanoparticles |
Advanced gastric cancer patients |
Efficacy and safety |
Phase 2 |
NCT01641783 |
Granulocyte-macrophage colony-stimulating factor (GM-CSF) with weekly protein bound paclitaxel (Abraxane™) |
Paclitaxel albumin-stabilized nanoparticle formulation |
Paclitaxel albumin-stabilized nanoparticle formulation |
Advanced ovarian cancer, fallopian tube cancer, or primary peritoneal cancer |
Treating for cancers that did not respond to previous chemotherapy, chemoimmunotherapy |
Phase 2 (completed) |
NCT00466960 |
ABI-009 |
Sirolimus albumin-bound nanoparticles |
Sirolimus albumin-bound nanoparticles |
Advanced PEComa and patients with a malignancy with relevant genetic mutations or mTOR pathway activation |
Targeting multiple disease conditions |
Approved for marketing |
NCT03817515 |
MR-Linac-SPION |
Ferumoxytol |
Iron oxide nanoparticles (SPION) |
Primary & metastatic hepatic cancers |
Radiotherapy |
Not mentioned |
NCT04682847 |
CD24-gold nanocomposite |
CD24 primer and gold nanoparticle |
Gold nanoparticles |
Salivary gland tumors |
Diagnostic tool, biomarker |
Not mentioned |
NCT04907422 |
Liposomal daunorubicin |
Daunorubicin |
Liposome |
Metastatic breast cancer |
Chemotherapy |
Phase 1 |
NCT00004207 |
Albumin-bound paclitaxel (Abraxane) in combination with carboplatin and herceptin |
Abraxane |
Albumin-bound paclitaxel nanoparticle |
Advanced breast cancer |
Combination therapy |
Phase 2 (completed) |
NCT00093145 |
Abraxane® with or without Mifepristone |
Mifepristone |
Nab-paclitaxel |
Advanced, glucocorticoid receptor-positive, triple-negative breast cancer |
Pharmacokinetic studies |
Phase 2 |
NCT02788981 |
AA NABPLAGEM |
Ascorbic acid (AA),nanoparticle paclitaxel protein bound, cisplatin, gemcitabine |
Nanoparticle paclitaxel protein bound |
Metastatic pancreatic cancer |
Combination therapy |
Phase 1 and 2 (completed) |
NCT03410030 |
SPIO |
Ferumoxtran-10 (USPIO) |
Superparamagnetic nanoparticle |
Bladder cancer, genitourinary cancer and prostate cancer |
Detect cancer in the pelvic lymph nodes or malignant pelvic lymph nodes |
Not applicable |
NCT00147238 |
Nab-PTX plus S-1 and sintilimab |
Nab-PTX |
Nanoparticle albumin-bound-paclitaxel |
Stage IIIC gastric cancer |
Adjuvant therapy |
Phase 1 & 2 |
NCT04781413 |
EP0057 in combination with olaparib |
EP0057 and olaparib |
Investigational nanoparticle–drug conjugate with a camptothecin payload |
Advanced gastric cancer and small cell lung cancer |
Combination therapy |
Phase 2 |
NCT05411679 |
CRLX101(NLG207) |
CRLX101 |
Nanoparticle formulation of camptothecin |
Advanced non-small cell lung cancer |
Safety and activity |
Phase 2 |
NCT01380769 |
Combination of ABI-007 (Abraxane) and GW572016 (Lapatinib) |
Abraxane |
Paclitaxel albumin-stabilized nanoparticle formulation |
Stage I, stage II, or stage III breast cancer |
Combination therapy |
Early phase 1 |
NCT00331630 |
FOLFIRABRAX |
Paclitaxel albumin-stabilized nanoparticle formulation, fluorouracil, leucovorin calcium, and irinotecan hydrochloride |
Paclitaxel albumin-stabilized nanoparticle formulation |
Advanced gastrointestinal cancer |
Reduce spreading of cancer |
Phase 1 and 2 (completed) |
NCT02333188 |
TKM 080301 |
siRNA against the PLK1 gene product) |
Lipid nanoparticles |
Colorectal, pancreas, gastric, breast, ovarian, and esophageal cancers with hepatic metastases |
Safety and effectiveness |
Phase 1 (completed) |
NCT01437007 |
NK105 |
Paclitaxel |
Micellar nanoparticle |
Breast cancer |
Verify the non-inferiority |
Phase 3 (completed) |
NCT01644890 |
Magnetic particle-ICG |
Magnetic tracers (FerroTrace) and indocyanine green (ICG) |
Magnetic nanoparticles |
Colorectal cancer |
Feasibility of sentinel lymph node (SLN) mapping and safety |
Phase 1 & 2 |
NCT05092750 |
CPX-1 |
Irinotecan HCl:floxuridine |
Liposome |
Advanced colorectal cancer |
Combinational therapy |
Phase 2 (completed) |
NCT00361842 |
NC-6300 |
Epirubicin |
Nanoparticle |
Advanced solid tumors or soft tissue sarcoma |
Safety and tolerability |
Phase 1 & 2 |
NCT03168061 |
CPC634 (CriPec® Docetaxel) |
Docetaxel |
CriPec® nanoparticles |
Platinum resistant ovarian cancer (CINOVA) |
Efficacy over cancers that are resistant to prior platinum-based chemotherapy, safety, and tolerability |
Phase 2 (completed) |
NCT03742713 |
NanoTherm® |
Iron nanoparticles |
Iron nanoparticles |
Intermediate-risk prostate cancer |
NanoTherm ablation |
Not applicable |
NCT05010759 |
Doxorubicin loaded anti-EGFR immunoliposomes |
Anti-EGFR immunoliposomes and doxorubicin |
Liposomes |
Solid tumors |
Efficacy and safety |
Phase 1 completed) |
NCT01702129 |
Carbon nanoparticles |
Carbon nanoparticles and indocyanine green |
Carbon nanoparticles |
Colorectal cancer |
Lymph node tracers |
Phase 2 and 3 |
NCT04759820 |
NBTXR3 |
Hafnium oxide |
Nanoparticles |
Locally advanced or borderline-resectable pancreatic cancer |
Particle activation by radiation therapy |
Phase 1 |
NCT04484909 |
Liposomal paclitaxel |
Paclitaxel |
Liposome |
Cancer |
Pharmacokinetics |
Phase 4 |
NCT00606515 |
INT-1B3 |
microRNA (miR-193a-3p) |
Lipid nanoparticles |
Advanced solid tumors |
Safety, pharmacokinetics, pharmacodynamics, and preliminary efficacy |
Phase 1 |
NCT04675996 |
NanoPac |
Paclitaxel |
Sterile nanoparticulate paclitaxel |
Lung cancer |
Chemotherapy and safety |
Phase 2 |
NCT04314895 |
Docetaxel-PNP and taxotere |
Docetaxel |
Polymeric nanoparticle |
Advanced solid cancer |
Pharmacokinetic study |
Phase 1 (completed |
NCT02274610 |
Silicon incorporated with quaternary ammonium polyethylenimine nanoparticles |
Silicon |
Quaternary ammonium polyethylenimine nanoparticles |
Carcinoma of head and neck |
Antibacterial activity |
Phase 1 |
NCT01007240 |
E7389 liposomal formulation |
Eribulin |
Liposome |
Solid tumor |
Safety, pharmacokinetics (PK) and efficacy |
Phase 1 |
NCT03207672 |
OTX-2002 |
Biscistronic mRNA |
Lipid nanoparticles |
Hepatocellular carcinoma and other solid tumortypes known for association with the MYC oncogene |
Safety, tolerability, pharmacokinetics, pharmacodynamics, and preliminary antitumor activity |
Phase 1 & 2 |
NCT05497453 |
FF-10832 |
Gemcitabine |
Liposome |
Solid tumors |
Safety and tolerance |
Phase 1 |
NCT03440450 |
ELU001 |
Exatecans and folic acid analogs |
Silicon core/polyethylene glycol C'Dot nanoparticle |
Solid tumors that over express folate receptor alpha (FRα) |
Safety and efficacy |
Phase 1 & 2 |
NCT05001282 |
NBTXR3 |
Hafnium oxide |
Hafnium oxide-containing nanoparticles |
Esophageal cancer |
Radiation therapy with concurrent chemotherapy |
Phase 1 |
NCT04615013 |
Irinotecan liposomeand bevacizumab |
Irinotecan sucrosofate |
Liposome |
Platinum resistant ovarian, fallopian tube, or primary peritoneal cancer |
Efficacy and toxicity |
Phase 2 |
NCT04753216 |
mRNA-NP (nanoparticle) vaccine |
mRNA |
DOTAP liposome vaccine |
Early melanoma |
Toxicity and feasibility |
Phase 1 |
NCT05264974 |
Rexin-G |
Gene |
Nanoparticle |
Sarcoma |
Safety and efficacy |
Phase 1 & 2 (completed) |
NCT00505713 |
Silica nanoparticles |
Silica nanoparticles |
Silica nanoparticles |
Head and neck melanoma |
Bioimaging |
Phase 1 & 2 |
NCT02106598 |
NL CPT-11 |
CPT-11 |
Liposome |
Glioma |
Safety, tolerance and pharmacokinetics |
Phase 1 |
NCT00734682 |
Mitoxantrone hydrochloride liposome |
Mitoxantrone hydrochloride |
Liposome |
Platinum-resistant ovarian cancer |
Safety and efficacy |
Phase 1 |
NCT04718376 |
Bevacizumab + doxorubicin hydrochloride liposome |
Bevacizumab |
Doxorubicin hydrochloride liposome |
Breast cancer |
Combination therapy |
Phase 2 (completed) |
NCT00445406 |
Irinotecan liposome |
Irinotecan |
Liposome |
Advanced pancreatic cancer |
Safety and tolerability |
Phase 1 |
NCT04796948 |
LEP-ETU |
Paclitaxel |
Liposome |
Advanced cancer (neoplasm) |
Safety, pharmacokinetics, and anti-tumor effect |
Phase 1 (completed) |
NCT00080418 |
LE-DT |
Docetaxel |
Liposome |
Solid tumors |
Maximum tolerated dose and dose limiting toxicity, pharmacokinetics, and anti-tumor effect |
Phase 1 (completed) |
NCT01151384 |
Topotecan liposomes |
Topotecan |
Liposome |
Small cell lung cancer, ovarian cancer, solid tumors |
Safety and tolerability |
Phase 1 (completed) |
NCT00765973 |
Liposome doxorubicin |
Doxorubicin |
Liposome |
Desmoid tumor |
Efficacy and safety |
Phase 3 |
NCT05561036 |
FF-10850 |
Topotecan |
Liposome |
Merkel cell carcinoma |
Tolerance and safety |
Phase 1 |
NCT04047251 |
Pegylated liposomal doxorubicin |
Doxorubicin |
Pegylated liposome |
Endocrine-resistant breast cancer |
Chemo-immunotherapy |
Phase 1b |
NCT03591276 |
WGI-0301 |
Archexin® |
Lipid nanoparticle |
Advanced solid tumors |
Safety, tolerability, and pharmacokinetics |
Phase 1 |
NCT05267899 |
Several phase I trials using nano-platforms for use as drugs, as well as a combined theranostic platforms have reported positive results. Many of the nano-formulations under trial are for developing effective nanocarriers for established and approved anticancer drugs. More than one study is in phase I trial to test the efficacy of Topotecan-based liposomes for advanced solid tumors (NCT04047251),99 small cell lung carcinomas as well as ovarian carcinomas (NCT00765973).111 Even though the phase I trials have been completed, no results have been published. Another topoisomerase inhibitor undergoing clinical trials for a liposome mediated delivery is Irinotecan (NCT04796948).112 This study looks into the safety and tolerability of Irinotecan in combination with 5FU and oxaliplatin and is in the initial stages of phase I trials. Paclitaxel (NCT00080418)113 and Doxetaxel (NCT01151384)114 containing liposomes are also under phase I clinical trial as a part of various combinatorial therapy formulations. These drugs are being tested using various other nanocarrier platforms such as nanoparticulate paclitaxel (NCT00915369),115, (NCT00666991),116 albumin-stabilized paclitaxel (NCT00331630)117 and polymeric Doxetaxel nanoparticles (NCT02274610).118 Other drugs under PhaseI clinical trials as cancer nanomedicine include Cetuximab polymeric nanoparticles (NCT03774680),119 Doxorubicin liposome (NCT05273944),120 Daunorubicin liposomes (NCT00004207),121 doxorubicin PEGylated liposomes (NCT03591276)122 and lipid nanoparticles (NCT05267899).123 Another emerging field with many ongoing phase I clinical trials are nanomedicine platforms for imaging as well as for radiation therapy. CdS/ZnS core–shell type quantum dots with carboxylic acid-functionalized (QDs-COOH) are being studied for their bio-imaging capabilities as fluorescent nanoparticles conjugated somatostatin analogue (NCT04138342).124 Other metal/metal oxide nanoparticles studies include FerumoxytolIron oxide nanoparticles for hepatic cancers (NCT04682847),125 CD24 primer and gold nanoparticle being investigated as a diagnostic tool and biomarker (NCT04907422)126 for salivary gland tumors, Ferumoxtran-10 Superparamagnetic nanoparticle (NCT00147238)127 for detection of cancer in the pelvic lymph nodes or malignant pelvic lymph nodes and Hafnium Oxide Particle activation by radiation therapy against locally advanced or borderline-resectable pancreatic cancer (NCT04484909).128 Other studies with antibody based drugs, miRNAs, and targeted therapy against gene targets such as DOTAP: Chol-FUS1liposome complex gene transfer therapy (NCT00059605),129 TKM 080301siRNA lipid nanoparticles against the PLK1 Gene Product (NCT01437007)130 and INT-1B3 microRNA (miR-193a-3p) lipid nanoparticles are also in progress at phase I of clinical trials. Most of these studies have not had any reported results so far.
Recently, in phase II clinical trial (NCT02596373)131 mitoxantrone hydrochloride liposome (Lipo-MIT) was tested in patients with advanced breast cancer. Lipo-MIT was injected intravenously and the efficacy and safety in terms of objective response rate, disease control rate, and progression-free survival were investigated. An altered toxicity profile was observed with Lipo-MIT treatment with fewer cardiovascular events. But this study was limited due to its small sample size.132 In another study, the bioequivalence of a hybrid pegylated liposomal doxorubicin (PLD) hydrochloride injection with reference product Caelyx® was evident in patients with ovarian cancer.133 Another study reported promising efficacy and fewer toxic effects in platinum-resistant recurrent ovarian cancer patients treated with a formulation containing apatinib and pegylated liposomal doxorubicin. This was a comparative study conducted with and without the addition of apatinib in pegylated liposomal doxorubicin. However, some side effects such as hypertension and decreased neutrophil and white blood cell count were observed in patients who underwent this combinatorial therapy.134 Recently, a phase 1 study of Eribulin liposomal formulation (E7389-LF) was examined for breast cancer treatment. Patients received formulation every three weeks and tumor assessment was conducted once in six weeks. Tolerability and antitumor activities were evident in the investigation. However, adverse events such as neutropenia, leukopenia, and thrombocytopenia were observed in patients.135 Good efficacy of combinational drugs containing pembrolizumab, bevacizumab, and pegylated liposomal doxorubicin was observed in ovarian cancer patients. But four patients had palmar-plantar erythrodysesthesia.136 Liposomal Gemcitabine (FF-10832) is a stable liposome that was tested for antitumor activity in advanced solid tumor patients. Better efficacy and fewer adverse effects were reported in this study.137 ThermoDox® is a thermally sensitive liposome loaded with doxorubicin. This particle is triggered by mild hyperthermia and the quantification of accumulated drug concentration can be examined before and after ultrasound exposure. Results showed that this formulation is better for drug delivery and cell penetration.138
The percentage of studies which clear the phase II clinical trials to reach the phase III clinical trials are much lesser as compared to those which reach phase II form phase I. The major reason for this is the higher number of participants, thereby increasing the probability of determining the side effects.99 There have been a few studies that had to be dropped at phase II and III due to non-availability of participants as well. Phase III clinical trials have even more stringent requirements and are fewer in number. There are two carbon nanoparticle-based cancer nanomedicines currently under phase III trials (NCT04759820),139 NCT02123407).140 A paclitaxel micellar nanoparticle (NCT01644890)141 and a Doxorubicin liposome formulation (NCT05561036)142 are also undergoing phase III trials. Some of the formulations have also reached phase IV and have been approved for marketing (NCT00606515),143 NCT03817515).144 The numbers of effective and safe nano-formulations clearing clinical trials are still very few and there are still challenges ahead to be cleared before cancer nanomedicine can be utilized to its full potential.
5. Conclusion and future challenges
In the past years, nanotechnology has been widely applied in all aspects of science, engineering, and technology, and research & development in this discipline has been intense. Many cancer nanomedicine researchers have investigated largely consistent processes, which include formulation, characterization, in vitro proof of concept, and validation of anticancer activity in preclinical trials. Low throughput and weak predictive abilities plague validation efforts. This review has covered the evolution of cancer nanomedicine from its inception to its current state-of-the-art. In the beginning, we explained the concept of “nanotechnology” and its advancement during the next years, especially in the case of cancer nanomedicine. From 1959 to till now, advancement in nanotechnology is visible in each and every field, including clinical areas. Some of the formulations got approval and many in vitro, in vivo, and clinical studies are ongoing. Increased circulation time, suitable size range, less toxicity, and good drug accumulation made it superior to the conventional method. Nanoparticles can target a cancer cell via passive and active targeting. Due to EPR effect and small size, nanoparticles can easily enter into the tumor cells and retain them in the tumor bed due to long circulation time. Besides, targeting TME is also the best way to reduce tumor progression. Different types of nano-formulations such as liposomes, extracellular vesicles, nanoemulsions, gold/silver nanoparticles, magnetic nanoparticles, carbon dots, and solid lipid nanoparticles are investigated for tumor targeting, bio-imaging, and drug delivery. However, new methods/or technologies are always under consideration to overcome the current challenges explained below.
To develop nano-therapeutics of high efficacy with accumulation in the tumor site rather than the normal cells should be the main criteria focused by the researchers. Because of the composite structure of the nanoparticles, it is very difficult to identify their toxicity and there is no proper validated model for checking the nano-bio interactions, especially, nano-immuno interactions. Other two factors hindering the approval are reproducibility and transparency. Apart from that, the higher cost and complexity have added restriction to the translation success. The journey of a drug from its manufacturing stage to the approval stage is very complex and time-consuming. In addition, the advancement in the instrumentation and characterization methods are necessary for the accomplishment of a drug product.145 Moreover, choice on the drug selection with a combinatorial regimen for the specific disease over the targeted population should also be considered important. Most of the time biological barriers also cause an imbalance in terms of targeted delivery, permeability and penetration, endo/lysosomal escape, intracellular processing and trafficking, and metastasis.146
The lab-scale production of drugs are easier to achieve, but large-scale manufacturing seems to be a challenging task due to limitations in the advanced experimental set-up and non-availability of sufficient information on the scale-up technologies. Also, adverse effects are encountered due to the difficulties observed during the scale-up process and in reproducing the preparation process.147 The developed nanomedicines, are in general, screened by FDA and EMA, but the lack of standard operating procedure for the evaluation of nanomedicines makes the task tougher.148 Checking the drugs for any changes during every phase of the clinical trials seems crucial in terms of safety and biocompatibility. Moreover, well-defined characteristics and reproducibility of the drug are essential for initiating the clinical trials. Challenges faced during nanomedicine manufacturing include the presence of contaminants, poor quality control, insufficient batch-to-batch variability, chemical instability, biocompatibility, low production yield, scalability complexities, high cost, lack of infrastructure, government regulations and lack of funding.145,149
Patient stratification, drug selection, combination therapies and immunomodulation are some of the important parameters to overcome the challenges of nanoparticles150 and these details are illustrated in Fig. 5. Various approaches like CTC analysis, immunohistochemical assessment, and imaging of accumulation of nanomedicines are used for patient stratification.151,152 Liquid biomarkers, tissue biomarkers, and imaging biomarkers are currently available. While discussing drug selection, drug classes' modular design and library screening could be considered. Nowadays, combination therapies are widely used in cancer research to increase the efficacy. However, clinical trials for nanomedicines are mostly designed to evaluate monotherapy, which would affect the progress in clinical trials. Improving cancer therapy with the capability to target and modulate the component of the immune system is needed.146 A well-designed nanoparticle can be applied as a potential tool for bio-imaging and tumor detection. The high sensitivity of the nano-biosensors is due to the high surface area to volume ratio of the nanoparticle, most preferentially, the gold nanoparticles, quantum dots and polymer dots.153 Also, radio-labelled nanoparticles, uniquely suited for positron-emission tomography (PET) or single-photon emission computed tomography (SPECT) mapping of sentinel lymph node and T2-weighted magnetic resonance imaging (MRI) probes are mostly made from iron oxide nanoparticles.154 To improve the drug delivery, we have to consider every step ranging from internalization, circulation, penetration into the tumor microenvironment, binding to the target and the destruction of tumor cells. It is necessary to have a clear understanding of the relationship between the physicochemical properties of nanoparticles and their biological response. In recent years, researchers are also focusing on computer algorithms for achieving proper drug delivery.145
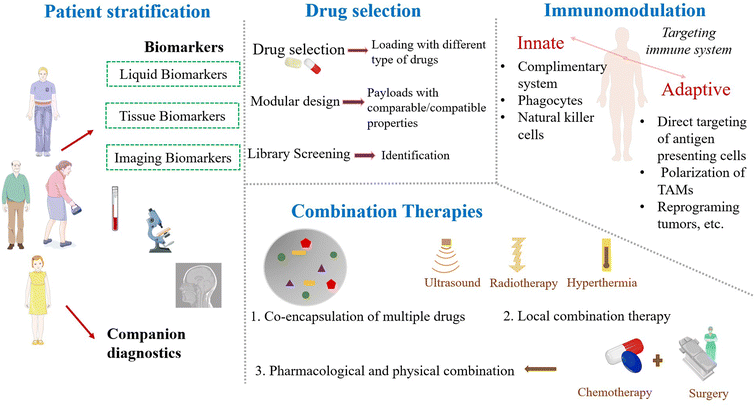 |
| Fig. 5 Modern approaches of nanomedicine in cancer therapy. TAMs: tumor associated macrophages. Parts of the figure were drawn using pictures from Servier Medical Art. Servier Medical Art by Servier is licensed under a Creative Commons Attribution 3.0 Unported License (https://creativecommons.org/licenses/by/3.0/). | |
Opportunities and challenges are two sides of a coin. More clinical trials are needed for the confirmation of preclinical and in vitro studies. These should help develop a deep understanding of the interaction of nanoparticles with cells and its after-effects. The main drug delivery routes are oral, intravenous, and subcutaneous for anticancer administration. Inhalation delivery, rectal delivery, and pulmonary delivery are newly proposed. However, these methods are limited due to the high toxicity caused by the combined action of drug deposition and high therapeutic potency. Besides, massive drug doses are needed due to the loss of drugs in the pulmonary tract. In rectal delivery, low absorption is also a major problem.155 Recently, mitochondria-based targeting has shown great promise in cancer therapy due to the involvement of mitochondria in tumor generation and progression. Difficulties in clinical trials and bio-safety concerns due to the positive charge on nanoparticles (toxic to normal cells) are the major challenges faced during mitochondrial-targeted therapy.156 Another study mentioned that liposomal formulations lose their activity overtime due to their exposure to blood proteins.157 The major drawback of carbon-based nanomaterials is the difficulty in standardization due to their non-uniform nature; the phototherapy method is associated with a lack of targeting. Besides, some tumors are difficult to identify due to their small size. Therefore, diagnosis techniques must be improved.158
Surely, all the challenges associated with cancer nanomedicine therapy will eventually disappear and, in the future, it will play a crucial role in all aspects of cancer therapy including diagnosis, bio-imaging, and drug delivery.
Conflicts of interest
The authors report no conflicts of interest in this work.
Acknowledgements
We gratefully acknowledge the support of Pratiksha Trust, Bangalore towards this research. The authors acknowledge the use of biorender platform for creating the Fig. 2–4.
References
- L. A. Torre, R. L. Siegel, E. M. Ward and A. Jemal, Global Cancer Incidence and Mortality Rates and Trends—An Update, Cancer Epidemiol. Biomark. Prev., 2016, 25(1), 16–27 CrossRef PubMed
. - G. B. Faguet, A brief history of cancer: age-old milestones underlying our current knowledge database: a brief history of cancer, Int. J. Cancer, 2015, 136(9), 2022–2036 CrossRef CAS PubMed
. - P. Stathopoulos, Galen's Contribution to Head and Neck Surgery, J. Oral Maxillofac. Surg., 2017, 75(6), 1095–1096 CrossRef PubMed
. - A. Emami Nejad, S. Najafgholian, A. Rostami, A. Sistani, S. Shojaeifar and M. Esparvarinha, et al., The role of hypoxia in the tumor microenvironment and development of cancer stem cell: a novel approach to developing treatment, Cancer Cell Int., 2021, 21(1), 62 CrossRef CAS PubMed
. - Y. A. Fouad and C. Aanei, Revisiting the hallmarks of cancer, Am. J. Cancer Res., 2017, 7(5), 1016–1036 CAS
. - I. Dagogo-Jack and A. T. Shaw, Tumour heterogeneity and resistance to cancer therapies, Nat. Rev. Clin. Oncol., 2018, 15(2), 81–94 CrossRef CAS PubMed
. - D. Rosenblum, N. Joshi, W. Tao, J. M. Karp and D. Peer, Progress and challenges towards targeted delivery of cancer therapeutics, Nat. Commun., 2018, 9(1), 1410 CrossRef PubMed
. - S. S. Joshi and B. D. Badgwell, Current treatment and recent progress in gastric cancer, Ca - Cancer J. Clin., 2021, 71(3), 264–279 CrossRef PubMed
. - L. Falzone, S. Salomone and M. Libra, Evolution of Cancer Pharmacological Treatments at the Turn of the Third Millennium, Front. Pharmacol., 2018, 9, 1300 CrossRef CAS PubMed
. - B. Saha, S. Bhattacharyya, S. Mete, A. Mukherjee and P. De, Redox-Driven Disassembly of Polymer–Chlorambucil Polyprodrug: Delivery of Anticancer Nitrogen Mustard and DNA Alkylation, ACS Appl. Polym. Mater., 2019, 1(9), 2503–2515 CrossRef CAS
. - A. I. Riggio, K. E. Varley and A. L. Welm, The lingering mysteries of metastatic recurrence in breast cancer, Br. J. Cancer, 2021, 124(1), 13–26 CrossRef PubMed
. - C. de Martel, D. Georges, F. Bray, J. Ferlay and G. M. Clifford, Global burden of cancer attributable to infections in 2018: a worldwide incidence analysis, Lancet Global Health, 2020, 8(2), e180–e190 CrossRef PubMed
. - Y. Sato, T. Nakamura, Y. Yamada and H. Harashima, The nanomedicine rush: new strategies for unmet medical needs based on innovative nano DDS, J. Controlled Release, 2021, 330, 305–316 CrossRef CAS PubMed
. - O. L. Gobbo, K. Sjaastad, M. W. Radomski, Y. Volkov and A. Prina-Mello, Magnetic Nanoparticles in Cancer Theranostics, Theranostics, 2015, 5(11), 1249–1263 CrossRef CAS PubMed
. - J. A. Kemp and Y. J. Kwon, Cancer nanotechnology: current status and perspectives, Nano Converg., 2021, 8(1), 34 CrossRef CAS PubMed
. - S. Mohan Bhagyaraj and O. S. Oluwafemi, Nanotechnology: The Science of the Invisible, in Synthesis of Inorganic Nanomaterials [Internet], Elsevier, 2018 [cited 2022 Oct 7], p. 1–18, available from: https://linkinghub.elsevier.com/retrieve/pii/B9780081019757000014 Search PubMed.
- D. Klemm, E. D. Cranston, D. Fischer, M. Gama, S. A. Kedzior and D. Kralisch, et al., Nanocellulose as a natural source for groundbreaking applications in materials science: today's state, Mater. Today, 2018, 21(7), 720–748 CrossRef CAS
. - O. P. Bodunde, O. M. Ikumapayi, E. T. Akinlabi, B. I. Oladapo, A. O. M. Adeoye and S. O. Fatoba, A futuristic insight into a “nano-doctor”: a clinical review on medical diagnosis and devices using nanotechnology, Mater. Today Proc., 2021, 44, 1144–1153 CrossRef CAS
. - G. Venkatraman, S. Ramya, G. Akila and S. Kumar, et al., Nanomedicine: towards development of patient-friendly drug-delivery systems for oncological applications, Int. J. Neurol., 2012, 1043 Search PubMed
. - M. Chamundeeswari, J. Jeslin and M. L. Verma, Nanocarriers for drug delivery applications, Environ. Chem. Lett., 2019, 17(2), 849–865 CrossRef CAS
. - S. Senapati, A. K. Mahanta, S. Kumar and P. Maiti, Controlled drug delivery vehicles for cancer treatment and their performance, Signal Transduction Targeted Ther., 2018, 3(1), 7 CrossRef PubMed
. - S. Quader and K. Kataoka, Nanomaterial-Enabled Cancer Therapy, Mol. Ther., 2017, 25(7), 1501–1513 CrossRef CAS PubMed
. - S. Alshehri, S. S. Imam, M. Rizwanullah, S. Akhter, W. Mahdi and M. Kazi, et al., Progress of Cancer Nanotechnology as Diagnostics, Therapeutics, and Theranostics Nanomedicine: Preclinical Promise and Translational Challenges, Pharmaceutics, 2020, 13(1), 24 CrossRef PubMed
. - M. Rai and A. Ingle, Role of nanotechnology in agriculture with special reference to management of insect pests, Appl. Microbiol. Biotechnol., 2012, 94(2), 287–293 CrossRef CAS PubMed
. - S. Dean, G. Mansoori and T. Fauzi Soelaiman, Nanotechnology — An Introduction for the Standards Community, J. ASTM Int., 2005, 2(6), 13110 CrossRef
. - Y. Dang, Nanoparticle-based drug delivery systems for cancer therapy, 2020, vol. 10 Search PubMed
. - J. W. Goodwin, J. Hearn, C. C. Ho and R. H. Ottewill, The preparation and characterisation of polymer latices formed in the absence of surface active agents, Br. Polym. J., 1973, 5(5), 347–362 CrossRef CAS
. - S. Bayda, M. Adeel, T. Tuccinardi, M. Cordani and F. Rizzolio, The History of Nanoscience and Nanotechnology: From Chemical–Physical Applications to Nanomedicine, Molecules, 2019, 25(1), 112 CrossRef PubMed
. - J. K. Patra, G. Das, L. F. Fraceto, E. V. R. Campos, M. del P. Rodriguez-Torres and L. S. Acosta-Torres, et al., Nano based drug delivery systems: recent developments and future prospects, J. Nanobiotechnol., 2018, 16(1), 71 CrossRef PubMed
. - D. C. Wimalachandra, Y. Li, J. Liu, S. Shikha, J. Zhang and Y. C. Lim, et al., Microfluidic-Based Immunomodulation of Immune Cells Using Upconversion Nanoparticles in Simulated Blood Vessel–Tumor System, ACS Appl. Mater. Interfaces, 2019, 11(41), 37513–37523 CrossRef CAS PubMed
. - D. Vllasaliu, M. Thanou, S. Stolnik and R. Fowler, Recent advances in oral delivery of biologics: nanomedicine and physical modes of delivery, Expet Opin. Drug Deliv., 2018, 15(8), 759–770 CrossRef CAS PubMed
. - S. Haque, S. Md, J. K. Sahni, J. Ali and S. Baboota, Development and evaluation of brain targeted intranasal alginate nanoparticles for treatment of depression, J. Psychiatr. Res., 2014, 48(1), 1–12 CrossRef PubMed
. - X. Tong, Z. Wang, X. Sun, J. Song, O. Jacobson and G. Niu, et al., Size Dependent Kinetics of Gold Nanorods in EPR Mediated Tumor Delivery, Theranostics, 2016, 6(12), 2039–2051 CrossRef CAS PubMed
. - M. S. Kim, M. J. Haney, Y. Zhao, D. Yuan, I. Deygen and N. L. Klyachko, et al., Engineering macrophage-derived exosomes for targeted paclitaxel delivery to pulmonary metastases: in vitro and in vivo evaluations, Nanomedicine, 2018, 14(1), 195–204 CrossRef CAS PubMed
. - X. Gao, N. Ran, X. Dong, B. Zuo, R. Yang and Q. Zhou, et al., Anchor peptide captures, targets, and loads exosomes of diverse origins for diagnostics and therapy, Sci. Transl. Med., 2018, 10(444), eaat0195 CrossRef PubMed
. - H. Kobayashi, K. Ebisawa, M. Kambe, T. Kasai, H. Suga, K. Nakamura, et al., Effects of exosomes derived from the induced pluripotent stem cells on skin wound healing [Internet], Nagoya University Graduate School of Medicine, School of Medicine, 2018 [cited 2022 Sep 21]. Available from: https://doi.org/10.18999/nagjms.80.2.141 Search PubMed.
- A. Kalani, P. Chaturvedi, P. K. Kamat, C. Maldonado, P. Bauer and I. G. Joshua, et al., Curcumin-loaded embryonic stem cell exosomes restored neurovascular unit following ischemia-reperfusion injury, Int. J. Biochem. Cell Biol., 2016, 79, 360–369 CrossRef CAS PubMed
. - Y. Du, W. He, Q. Xia, W. Zhou, C. Yao and X. Li, Thioether Phosphatidylcholine Liposomes: A Novel ROS-Responsive Platform for Drug Delivery, ACS Appl. Mater. Interfaces, 2019, 11(41), 37411–37420 CrossRef CAS PubMed
. - D. Mundekkad and W. C. Cho, Nanoparticles in Clinical Translation for Cancer Therapy, Int. J. Mol. Sci., 2022, 23(3), 1685 CrossRef CAS PubMed
. - M. Durymanov, T. Kamaletdinova, S. E. Lehmann and J. Reineke, Exploiting passive nanomedicine accumulation at sites of enhanced vascular permeability for non-cancerous applications, J. Controlled Release, 2017, 261, 10–22 CrossRef CAS PubMed
. - M. Estanqueiro, M. H. Amaral, J. Conceição and J. M. Sousa Lobo, Nanotechnological carriers for cancer chemotherapy: the state of the art, Colloids Surf. B Biointerfaces, 2015, 126, 631–648 CrossRef CAS PubMed
. - F. Danhier, N. Lecouturier, B. Vroman, C. Jérôme, J. Marchand-Brynaert and O. Feron, et al., Paclitaxel-loaded PEGylated PLGA-based nanoparticles: In vitro and in vivo evaluation, J. Controlled Release, 2009, 133(1), 11–17 CrossRef CAS PubMed
. - D. Kalyane, N. Raval, R. Maheshwari, V. Tambe, K. Kalia and R. K. Tekade, Employment of enhanced permeability and retention effect (EPR): nanoparticle-based precision tools for targeting of therapeutic and diagnostic agent in cancer, Mater. Sci. Eng. C, 2019, 98, 1252–1276 CrossRef CAS PubMed
. - I. A. Khawar, J. H. Kim and H. J. Kuh, Improving drug delivery to solid tumors: priming the tumor microenvironment, J. Controlled Release, 2015, 201, 78–89 CrossRef CAS PubMed
. - S. Brandenburg, A. Müller, K. Turkowski, Y. T. Radev, S. Rot and C. Schmidt, et al., Resident microglia rather than peripheral macrophages promote vascularization in brain tumors and are source of alternative pro-angiogenic factors, Acta Neuropathol., 2016, 131(3), 365–378 CrossRef CAS PubMed
. - M. A. Subhan, S. S. K. Yalamarty, N. Filipczak, F. Parveen and V. P. Torchilin, Recent Advances in Tumor Targeting via EPR Effect for Cancer Treatment, J. Personalized Med., 2021, 11(6), 571 CrossRef PubMed
. - J. Wu, The Enhanced Permeability and Retention (EPR) Effect: The Significance of the Concept and Methods to Enhance Its Application, J. Personalized Med., 2021, 11(8), 771 CrossRef PubMed
. - Y. Nakamura, A. Mochida, P. L. Choyke and H. Kobayashi, Nanodrug Delivery: Is the Enhanced Permeability and Retention Effect Sufficient for Curing Cancer?, Bioconjugate Chem., 2016, 27(10), 2225–2238 CrossRef CAS PubMed
. - E. Huynh and G. Zheng, Cancer nanomedicine: addressing the dark side of the enhanced permeability and retention effect, Nanomedicine, 2015, 10(13), 1993–1995 CrossRef CAS PubMed
. - V. Ejigah, O. Owoseni, P. Bataille-Backer, O. D. Ogundipe, F. A. Fisusi and S. K. Adesina, Approaches to Improve Macromolecule and Nanoparticle Accumulation in the Tumor Microenvironment by the Enhanced Permeability and Retention Effect, Polymers, 2022, 14(13), 2601 CrossRef CAS PubMed
. - H. Wang, R. Qu, Q. Chen, T. Zhang, X. Chen and B. Wu, et al., PEGylated Prussian blue nanoparticles for modulating polyethyleneimine cytotoxicity and attenuating tumor hypoxia for dual-enhanced photodynamic therapy, J. Mater. Chem. B, 2022, 10(28), 5410–5421 RSC
. - X. Pei, Z. Zhu, Z. Gan, J. Chen, X. Zhang and X. Cheng, et al., PEGylated nano-graphene oxide as a nanocarrier for delivering mixed anticancer drugs to improve anticancer activity, Sci. Rep., 2020, 10(1), 2717 CrossRef CAS PubMed
. - Y. Liu, X. Quan, J. Li, J. Huo, X. Li and Z. Zhao, et al., Liposomes embedded with PEGylated iron oxide nanoparticles enable ferroptosis and combination therapy in cancer, Natl. Sci. Rev., 2023, 10(1), nwac167 CrossRef PubMed
. - R. Baghban, L. Roshangar, R. Jahanban-Esfahlan, K. Seidi, A. Ebrahimi-Kalan and M. Jaymand, et al., Tumor microenvironment complexity and therapeutic implications at a glance, Cell Commun. Signal., 2020, 18(1), 59 CrossRef PubMed
. - M. Upreti, A. Jyoti and P. Sethi, Tumor microenvironment and nanotherapeutics, Transl. Cancer Res., 2013, 2(4), 309–319 CAS
. - Y. Zhang, R. Lin, H. Li, W. He, J. Du and J. Wang, Strategies to improve tumor penetration of nanomedicines through nanoparticle design, WIREs Nanomed Nanobiotechnol [Internet], 2019 [cited 2023 Feb 19];11(1). Available from: https://onlinelibrary.wiley.com/doi/10.1002/wnan.1519 Search PubMed.
- K. Li, D. Zhou, H. Cui, G. Mo, Y. Liu and K. Zheng, et al., Size-transformable gelatin/nanochitosan/doxorubicin nanoparticles with sequentially triggered drug release for anticancer therapy, Colloids Surf. B Biointerfaces, 2022, 220, 112927 CrossRef CAS PubMed
. - H. Chen, Q. Guo, Y. Chu, C. Li, Y. Zhang and P. Liu, et al., Smart hypoxia-responsive transformable and charge-reversible nanoparticles for the deep penetration and tumor microenvironment modulation of pancreatic cancer, Biomaterials, 2022, 287, 121599 CrossRef CAS PubMed
. - X. Wei, J. Wang, M. Liang and M. Song, Development of functional nanomedicines for tumor associated macrophages-focused cancer immunotherapy, Theranostics, 2022, 12(18), 7821–7852 CrossRef CAS PubMed
. - J. Zhou, Z. Tang, S. Gao, C. Li, Y. Feng and X. Zhou, Tumor-Associated Macrophages: Recent Insights and Therapies, Front. Oncol., 2020, 10, 188 CrossRef PubMed
. - Z. He and S. Zhang, Tumor-Associated Macrophages and Their Functional Transformation in the Hypoxic Tumor Microenvironment, Front. Immunol., 2021, 12, 741305 CrossRef CAS PubMed
. - N. Kumari and S. H. Choi, Tumor-associated macrophages in cancer: recent advancements in cancer nanoimmunotherapies, J. Exp. Clin. Cancer Res., 2022, 41(1), 68 CrossRef CAS PubMed
. - S. Zhang, F. Xie, K. Li, H. Zhang, Y. Yin and Y. Yu, et al., Gold nanoparticle-directed autophagy intervention for antitumor immunotherapy via inhibiting tumor-associated macrophage M2 polarization, Acta Pharm. Sin., 2022, 12(7), 3124–3138 CrossRef CAS PubMed
. - L. Donthireddy, P. Vonteddu, T. Murthy, T. Kwak, R. N. Eraslan and J. R. Podojil, et al., ONP-302 Nanoparticles Inhibit Tumor Growth By Altering Tumor-Associated Macrophages And Cancer-Associated Fibroblasts, J. Cancer., 2022, 13(6), 1933–1944 CrossRef CAS PubMed
. - P. Nakhaei, R. Margiana, D. O. Bokov, W. K. Abdelbasset, M. A. Jadidi Kouhbanani and R. S. Varma, et al., Liposomes: Structure, Biomedical Applications, and Stability Parameters With Emphasis on Cholesterol, Front. Bioeng. Biotechnol., 2021, 9, 705886 CrossRef PubMed
. - N. Marasini, K. A. Ghaffar, M. Skwarczynski and I. Toth, Liposomes as a Vaccine Delivery System, in Micro and Nanotechnology in Vaccine Development [Internet], Elsevier, 2017 [cited 2022 Nov 9], pp. 221–39, available from: https://linkinghub.elsevier.com/retrieve/pii/B9780323399814000129 Search PubMed.
- S. G. Antimisiaris, A. Marazioti, M. Kannavou, E. Natsaridis, F. Gkartziou and G. Kogkos, et al., Overcoming barriers by local drug delivery with liposomes, Adv. Drug Delivery Rev., 2021, 174, 53–86 CrossRef CAS PubMed
. - C. M. Dawidczyk, C. Kim, J. H. Park, L. M. Russell, K. H. Lee and M. G. Pomper, et al., State-of-the-art in design rules for drug delivery platforms: Lessons learned from FDA-approved nanomedicines, J. Controlled Release, 2014, 187, 133–144 CrossRef CAS PubMed
. - R. P. Das, V. V. Gandhi, B. G. Singh and A. Kunwar, Passive and Active Drug Targeting: Role of Nanocarriers in Rational Design of Anticancer Formulations, Clin. Prev. Dent., 2019, 25(28), 3034–3056 CAS
. - M. Riaz, M. Riaz, X. Zhang, C. Lin, K. Wong and X. Chen, et al., Surface Functionalization and Targeting Strategies of Liposomes in Solid Tumor Therapy: A Review, Int. J. Mol. Sci., 2018, 19(1), 195 CrossRef PubMed
. - C. H. Kim, S. G. Lee, M. J. Kang, S. Lee and Y. W. Choi, Surface modification of lipid-based nanocarriers for cancer cell-specific drug targeting, J. Pharm. Investig., 2017, 47(3), 203–227 CrossRef CAS
. - A. K. Iyer, Y. Su, J. Feng, X. Lan, X. Zhu and Y. Liu, et al., The effect of internalizing human single chain antibody fragment on liposome targeting to epithelioid and sarcomatoid mesothelioma, Biomaterials, 2011, 32(10), 2605–2613 CrossRef CAS PubMed
. - P. Wu, B. Zhang, D. K. W. Ocansey, W. Xu and H. Qian, Extracellular vesicles: A bright star of nanomedicine, Biomaterials, 2021, 269, 120467 CrossRef CAS PubMed
. - P. Vader, E. A. Mol, G. Pasterkamp and R. M. Schiffelers, Extracellular vesicles for drug delivery, Adv. Drug Delivery Rev., 2016, 106, 148–156 CrossRef CAS PubMed
. - T. J. Antes, R. C. Middleton, K. M. Luther, T. Ijichi, K. A. Peck and W. J. Liu, et al., Targeting extracellular vesicles to injured tissue using membrane cloaking and surface display, J. Nanobiotechnol., 2018, 16(1), 61 CrossRef PubMed
. - A. Pusuluri, D. Wu and S. Mitragotri, Immunological consequences of chemotherapy: Single drugs, combination therapies and nanoparticle-based treatments, J. Controlled Release, 2019, 305, 130–154 CrossRef CAS PubMed
. - F. Masood, P. Chen, T. Yasin, N. Fatima, F. Hasan and A. Hameed, Encapsulation of Ellipticine in poly-(3-hydroxybutyrate-co-3-hydroxyvalerate) based nanoparticles and its in vitro application, Mater. Sci. Eng. C, 2013, 33(3), 1054–1060 CrossRef CAS PubMed
. - S. Ganta, M. Talekar, A. Singh, T. P. Coleman and M. M. Amiji, Nanoemulsions in Translational Research—Opportunities and Challenges in Targeted Cancer Therapy, AAPS PharmSciTech, 2014, 15(3), 694–708 CrossRef CAS PubMed
. - B. Kim, C. D. Pena and D. T. Auguste, Targeted Lipid Nanoemulsions Encapsulating Epigenetic Drugs Exhibit Selective Cytotoxicity on CDH1–/FOXM1+ Triple Negative Breast Cancer Cells, Mol. Pharm., 2019, 16(5), 1813–1826 CrossRef CAS PubMed
. - R. Mahato, Nanoemulsion as Targeted Drug Delivery System for Cancer Therapeutics, J. Pharm. Sci. Pharmacol., 2017, 3(2), 83–97 CrossRef
. - J. Bugno, H. j. Hsu and S. Hong, Tweaking dendrimers and dendritic nanoparticles for controlled nano-bio interactions: potential nanocarriers for improved cancer targeting, J. Drug Targeting, 2015, 23(7–8), 642–650 CrossRef CAS PubMed
. - E. Abbasi, S. F. Aval, A. Akbarzadeh, M. Milani, H. T. Nasrabadi and S. W. Joo, et al., Dendrimers: synthesis, applications, and properties, Nanoscale Res. Lett., 2014, 9(1), 247 CrossRef PubMed
. - S. Sunoqrot, J. W. Bae, R. M. Pearson, K. Shyu, Y. Liu and D. H. Kim, et al., Temporal Control over Cellular Targeting through Hybridization of Folate-targeted Dendrimers and PEG-PLA Nanoparticles, Biomacromolecules, 2012, 13(4), 1223–1230 CrossRef CAS PubMed
. - A. A. Chis, C. Dobrea, C. Morgovan, A. M. Arseniu, L. L. Rus and A. Butuca, et al., Applications and Limitations of Dendrimers in Biomedicine, Molecules, 2020, 25(17), 3982 CrossRef CAS PubMed
. - S. H. Medina and M. E. H. El-Sayed, Dendrimers as Carriers for Delivery of Chemotherapeutic Agents, Chem. Rev., 2009, 109(7), 3141–3157 CrossRef CAS PubMed
. - S. Y. Fam, C. F. Chee, C. Y. Yong, K. L. Ho, A. R. Mariatulqabtiah and W. S. Tan, Stealth Coating of Nanoparticles in Drug-Delivery Systems, Nanomaterials, 2020, 10(4), 787 CrossRef CAS PubMed
. - P. Ghosh, G. Han, M. De, C. Kim and V. Rotello, Gold nanoparticles in delivery applications, Adv. Drug Delivery Rev., 2008, 60(11), 1307–1315 CrossRef CAS PubMed
. - S. Bisht, M. Mizuma, G. Feldmann, N. A. Ottenhof, S. M. Hong and D. Pramanik, et al., Systemic Administration of Polymeric Nanoparticle-Encapsulated Curcumin (NanoCurc) Blocks Tumor Growth and Metastases in Preclinical Models of Pancreatic Cancer, Mol. Cancer Ther., 2010, 9(8), 2255–2264 CrossRef CAS PubMed
. - A. Farzin, S. A. Etesami, J. Quint, A. Memic and A. Tamayol, Magnetic Nanoparticles in Cancer Therapy and Diagnosis, Adv. Healthc. Mater., 2020, 9(9), 1901058 CrossRef CAS PubMed
. - N. Ž. Knežević, I. Gadjanski and J. O. Durand, Magnetic nanoarchitectures for cancer sensing, imaging and therapy, J. Mater. Chem. B, 2019, 7(1), 9–23 RSC
. - P. B. Santhosh and N. P. Ulrih, Multifunctional superparamagnetic iron oxide nanoparticles: Promising tools in cancer theranostics, Cancer Lett., 2013, 336(1), 8–17 CrossRef CAS PubMed
. - S. Y. Lim, W. Shen and Z. Gao, Carbon quantum dots and their applications, Chem. Soc. Rev., 2015, 44(1), 362–381 RSC
. - L. J. Desmond, A. N. Phan and P. Gentile, Critical overview on the green synthesis of carbon quantum dots and their application for cancer therapy, Environ. Sci. Nano, 2021, 8(4), 848–862 RSC
. - Y. Duan, A. Dhar, C. Patel, M. Khimani, S. Neogi and P. Sharma, et al., A brief review on solid lipid nanoparticles: part and parcel of contemporary drug delivery systems, RSC Adv., 2020, 10(45), 26777–26791 RSC
. - R. Kumar, Lipid-Based Nanoparticles for Drug-Delivery Systems, in Nanocarriers for Drug Delivery [Internet], Elsevier, 2019, [cited 2022 Oct 9], pp. 249–284, available from: https://linkinghub.elsevier.com/retrieve/pii/B9780128140338000084 Search PubMed
. - W. Mehnert, Solid lipid nanoparticles Production, characterization and applications, Adv. Drug Delivery Rev., 2001, 47(2–3), 165–196 CrossRef CAS PubMed
. - M. Üner and G. Yener, Importance of solid lipid nanoparticles (SLN) in various administration routes and future perspectives, Int. J. Nanomed., 2007, 2(3), 289–300 Search PubMed
. - M. Akanda, G. Getti, U. Nandi, M. S. Mithu and D. Douroumis, Bioconjugated solid lipid nanoparticles (SLNs) for targeted prostate cancer therapy, Int. J. Pharm., 2021, 599, 120416 CrossRef CAS PubMed
. - A Study of FF-10850 Topotecan Liposome Injection in Advanced Solid Tumors RECRUITING Advanced Solid Tumors DRUG: FF-10850 Topotecan Liposome Injection Fujifilm Pharmaceuticals U.S.A., Inc. INTERVENTIONAL NCT04047251.
- U. Bulbake, S. Doppalapudi, N. Kommineni and W. Khan, Liposomal Formulations in Clinical Use: An Updated Review, Pharmaceutics, 2017, 9(4), 12 CrossRef PubMed
. - E. Beltrán-Gracia, A. López-Camacho, I. Higuera-Ciapara, J. B. Velázquez-Fernández and A. A. Vallejo-Cardona, Nanomedicine review: clinical developments in liposomal applications, Cancer Nanotechnol., 2019, 10(1), 11 CrossRef
. - F. Rommasi and N. Esfandiari, Liposomal Nanomedicine: Applications for Drug Delivery in Cancer Therapy, Nanoscale Res. Lett., 2021, 16(1), 95 CrossRef CAS PubMed
. - A. C. Krauss, X. Gao, L. Li, M. L. Manning, P. Patel and W. Fu, et al., FDA Approval Summary: (Daunorubicin and Cytarabine) Liposome for Injection for the Treatment of Adults with High-Risk Acute Myeloid Leukemia, Clin. Cancer Res., 2019, 25(9), 2685–2690 CrossRef CAS PubMed
. - I. Judson, J. A. Radford, M. Harris, J. Y. Blay, Q. van Hoesel and A. le Cesne, et al., Randomised phase II trial of pegylated liposomal doxorubicin (DOXIL®/CAELYX®) versus doxorubicin in the treatment of advanced or metastatic soft tissue sarcoma, Eur. J. Cancer, 2001, 37(7), 870–877 CrossRef CAS PubMed
. - S. Tran, P. DeGiovanni, B. Piel and P. Rai, Cancer nanomedicine: a review of recent success in drug delivery, Clinical and Translational Medicine [Internet], 2017 [cited 2023 Feb 25];6(1). Available from: https://onlinelibrary.wiley.com/doi/abs/10.1186/s40169-017-0175-0 Search PubMed.
- D. Bobo, K. J. Robinson, J. Islam, K. J. Thurecht and S. R. Corrie, Nanoparticle-Based Medicines: A Review of FDA-Approved Materials and Clinical Trials to Date, Pharm. Res., 2016, 33(10), 2373–2387 CrossRef CAS PubMed
. - P. Chowdhury, U. Ghosh, K. Samanta, M. Jaggi, S. C. Chauhan and M. M. Yallapu, Bioactive nanotherapeutic trends to combat triple negative breast cancer, Bioact. Mater., 2021, 6(10), 3269–3287 CrossRef CAS PubMed
. - A. Karabasz, M. Bzowska and K. Szczepanowicz, Biomedical Applications of Multifunctional Polymeric Nanocarriers: A Review of Current Literature, Int. J. Neurol., 2020, 15, 8673–8696 CAS
. - D. Alromi, S. Madani and A. Seifalian, Emerging Application of Magnetic Nanoparticles for Diagnosis and Treatment of Cancer, Polymers, 2021, 13(23), 4146 CrossRef CAS PubMed
. - F. Rodríguez, P. Caruana, N. De la Fuente, P. Español, M. Gámez and J. Balart, et al., Nano-Based Approved Pharmaceuticals for Cancer Treatment: Present and Future Challenges, Biomolecules, 2022, 12(6), 784 CrossRef PubMed
. - NCT00765973 Topotecan Liposomes Injection for Small Cell Lung Cancer (SCLC), Ovarian Cancer and Other Advanced Solid Tumors COMPLETED Small Cell Lung Cancer|Ovarian Cancer|Solid Tumors DRUG: Topotecan Liposomes Injection (TLI)|DRUG: Topotecan Liposomes Injection (TLI) Spectrum Pharmaceuticals, Inc INTERVENTIONAL.
- NCT04796948 A Study of Irinotecan Liposome in Advanced Pancreatic Cancer ENROLLING_BY_INVITATION Advanced Pancreatic Cancer DRUG: Irinotecan liposome oxaliplatin 5-FU(Fluorouracil Injection) LV(Calcium Folinate Injection) Jiangsu HengRui Medicine Co., Ltd. INTERVENTIONAL.
- NCT00080418 Liposome Entrapped Paclitaxel Easy to Use (LEP-ETU) in Patients With Advanced Cancer COMPLETED Neoplasm DRUG: Liposome Entrapped Paclitaxel Easy to Use INSYS Therapeutics Inc INTERVENTIONAL.
- NCT01151384 Liposome Encapsulated Docetaxel (LE-DT) in Patients With Solid Tumors COMPLETED Solid Tumors DRUG: LE-DT INSYS Therapeutics Inc INTERVENTIONAL.
- NCT00915369 A Clinical Trial to Study the Effects of Nanoparticle Based Paclitaxel Drug, Which Does Not Contain the Solvent Cremophor, in Advanced Breast Cancer UNKNOWN Advanced Breast Cancer DRUG: Nanoxel (Paclitaxel Nanoparticle formulation) Fresenius Kabi Oncology Ltd. INTERVENTIONAL.
- NCT00666991 Pharmacokinetic, Safety and Efficacy Study of Nanoparticle Paclitaxel in Patients With Peritoneal Cancers COMPLETED Peritoneal Neoplasms DRUG: nanoparticulate paclitaxel CritiTech, Inc. University of Kansas Medical Center|Beckloff Associates, Inc. INTERVENTIONAL.
- NCT00331630 Abraxane and Lapatinib in Treating Patients With Stage I, Stage II, or Stage III Breast Cancer COMPLETED Breast Cancer DRUG: lapatinib ditosylate|DRUG: paclitaxel albumin-stabilized nanoparticle formulation Northwestern University GlaxoSmithKline|Celgene Corporation INTERVENTIONAL.
- NCT02274610 Pharmacokinetic Study of Docetaxel-PNP and Taxotere to Treat Patient With Advanced Solid Cancer COMPLETED Solid Tumor DRUG: Docetaxel-PNP|DRUG: Taxotere Samyang Biopharmaceuticals Corporation INTERVENTIONAL.
- NCT03774680 Targeted Polymeric Nanoparticles Loaded With Cetuximab and Decorated With Somatostatin Analogue to Colon Cancer UNKNOWN Colon Cancer|Colo-rectal Cancer DRUG: Cetuximab nanoparticles|DRUG: oral approved anticancer drug Ahmed A. H. Abdellatif INTERVENTIONAL.
- NCT05273944 Bioequivalence Study of Doxorubicin Hydrochloride Liposome Injection (Lipodox®) in Chinese Patients RECRUITING Breast Cancer|Ovarian Cancer DRUG: Doxorubicin Hydrochloride Liposome Injection Shenzhen Kangzhe Pharmaceutical Co., Ltd. Sun Yat-Sen Memorial Hospital of Sun Yat-Sen University|Hunan Cancer Hospital|The Second Affiliated Hospital of Chongqing Medical University|First Affiliated Hospital of Jinan University|Zhejiang Provincial People’s Hospital|Yuncheng Central Hospital|Shantou Central Hospital|Yuebei People's Hospital|Cancer Hospital of Guizhou Province|Guangzhou Panyu Central Hospital|Dongguan People’s Hospital INTERVENTIONAL.
- NCT00004207 Liposomal Daunorubicin in Treating Patients With Metastatic Breast Cancer UNKNOWN Breast Cancer DRUG: liposomal daunorubicin citrate University of Leicester INTERVENTIONAL.
- NCT03591276 Phase 1b Study of Pegylated Liposomal Doxorubicin and Pembrolizumab in Endocrine-resistant Breast Cancer UNKNOWN Metastatic Breast Cancer DRUG: Chemotherapy Drugs, Cancer Shaare Zedek Medical Center INTERVENTIONAL.
- NCT05267899 A Phase I First in Human Study to Evaluate the Safety, Tolerability, and Pharmacokinetics of WGI-0301 in Patients With Advanced Solid Tumors RECRUITING Advanced Solid Tumors DRUG: WGI-0301 Zhejiang Haichang Biotech Co., Ltd. INTERVENTIONAL.
- NCT04138342 Topical Fluorescent Nanoparticles Conjugated Somatostatin Analog for Suppression and Bioimaging Breast Cancer UNKNOWN Breast Cancer|Skin Cancer|Skin Diseases DRUG: Quantum dots coated with veldoreotide|DRUG: Topical approved placebo Al-Azhar University INTERVENTIONAL.
- NCT04682847 Radiotherapy With Iron Oxide Nanoparticles (SPION) on MR-Linac for Primary & Metastatic Hepatic Cancers ACTIVE_NOT_RECRUITING Liver Neoplasms|Hepatic Cirrhosis|Hepatic Carcinoma|Liver Cancer|Liver Metastases|Liver Carcinoma|Hepatocellular Carcinoma|Hepatocellular Cancer|Hepatic Atrophy DRUG: Ferumoxytol injection Allegheny Singer Research Institute (also known as Allegheny Health Network Research Institute) OBSERVATIONAL.
- NCT04907422 Diagnostic and Prognostic Accuracy of Gold Nanoparticles in Salivary Gland Tumours COMPLETED Carcinoma Ex Pleomorphic Adenoma of Salivary Glands|Pleomorphic Adenoma of Salivary Glands DIAGNOSTIC_TEST: CD24-Gold Nanocomposite expression using Real-time quantitative polymerase chain reaction|DIAGNOSTIC_TEST: non-conjugated CD24 expression using Real-time quantitative polymerase chain reaction Amina Fouad Farag OBSERVATIONAL.
- NCT00147238 A Validation Study of MR Lymphangiography Using SPIO, a New Lymphotropic Superparamagnetic Nanoparticle Contrast TERMINATED Bladder Cancer|Genitourinary Cancer|Prostate Cancer DRUG: Ferumoxtran-10
(USPIO)|PROCEDURE: MR lymphangiography M. D. Anderson Cancer Center INTERVENTIONAL.
- NCT04484909 NBTXR3 Activated by Radiation Therapy for the Treatment of Locally Advanced or Borderline-Resectable Pancreatic Cancer RECRUITING Borderline Resectable Pancreatic Adenocarcinoma|Locally Advanced Pancreatic Ductal Adenocarcinoma|Resectable Pancreatic Ductal Adenocarcinoma|Stage III Pancreatic Cancer AJCC v8 OTHER: Hafnium Oxide-containing Nanoparticles NBTXR3|RADIATION: Radiation Therapy M. D. Anderson Cancer Center National Cancer Institute (NCI) INTERVENTIONAL.
- NCT00059605 Phase I Study of IV DOTAP: Cholesterol-Fus1 in Non-Small-Cell Lung Cancer COMPLETED Lung Cancer GENETIC: DOTAP:Chol-fus1 M.D. Anderson Cancer Center National Cancer Institute (NCI) INTERVENTIONAL.
- NCT01437007 TKM 080301 for Primary or Secondary Liver Cancer COMPLETED Colorectal Cancer With Hepatic Metastases|Pancreas Cancer With Hepatic Metastase|Gastric Cancer With Hepatic Metastase|Breast Cancer With Hepatic Metastase|Ovarian Cancer With Hepatic Metastase DRUG: TKM-080301 National Cancer Institute (NCI) INTERVENTIONAL.
- NCT02596373 A Study of Mitoxantrone Hydrochloride Liposome Injection in Advanced Recurrent or Metastatic Breast Cancer UNKNOWN Advanced Recurrent or Metastatic Breast Cancer DRUG: Mitoxantrone Hydrochloride Liposome Injection CSPC ZhongQi Pharmaceutical Technology Co., Ltd. INTERVENTIONAL.
- L. Wang, J. Cao, C. Li, X. Wang, Y. Zhao and T. Li, et al., Efficacy and safety of mitoxantrone hydrochloride liposome injection in Chinese patients with advanced breast cancer: a randomized, open-label, active-controlled, single-center, phase II clinical trial, Invest. New Drugs, 2022, 40(2), 330–339 CrossRef CAS PubMed
. - S. S. Prakash, R. V. Nagarkar, K. C. Puligundla, K. N. Lokesh, R. R. Boya and A. B. Patel, et al., Bioequivalence of a hybrid pegylated liposomal doxorubicin hydrochloride injection and Caelyx®: a single-dose, randomized, multicenter, open-label, two-period crossover study in patients with advanced ovarian cancer, Eur. J. Pharm. Sci., 2022, 176, 106248 CrossRef PubMed
. - T. Wang, J. Tang, H. Yang, R. Yin, J. Zhang and Q. Zhou, et al., Effect of Apatinib Plus Pegylated Liposomal Doxorubicin vs Pegylated Liposomal Doxorubicin Alone on Platinum-Resistant Recurrent Ovarian Cancer: The APPROVE Randomized Clinical Trial, JAMA Oncol., 2022, 8(8), 1169 CrossRef PubMed
. - N. Masuda, M. Ono, T. Mukohara, H. Yasojima, T. Shimoi and K. Kobayashi, et al., Phase 1 study of the liposomal formulation of eribulin (E7389-LF): results from the breast cancer expansion cohort, Eur. J. Cancer, 2022, 168, 108–118 CrossRef CAS PubMed
. - J. Michels, F. Ghiringhelli, J. S. Frenel, C. Brard, B. You and A. Floquet, et al., Pembrolizumab in combination with bevacizumab and pegylated liposomal doxorubicin in patients with platinum-resistant epithelial ovarian cancer, J. Clin. Oncol., 2021, 39(15_suppl), 5522 Search PubMed
. - E. H. Borazanci, F. Janku, E. P. Hamilton, J. S. Thomas, S. Sen and S. Fu, et al., A phase 1, first-in-human, dose-escalation and biomarker trial of liposomal gemcitabine (FF-10832) in patients with advanced solid tumors, J. Clin. Oncol., 2022, 40(16_suppl), 3097 CrossRef
. - P. C. Lyon, L. F. Griffiths, J. Lee, D. Chung, R. Carlisle and F. Wu, et al., Clinical trial protocol for TARDOX: a phase I study to investigate the feasibility of targeted release of lyso-thermosensitive liposomal doxorubicin (ThermoDox®) using focused ultrasound in patients with liver tumours, J. Ther. Ultrasound, 2017, 5(1), 28 CrossRef PubMed
. - NCT04759820 Carbon Nanoparticles vs Indocyanine Green RECRUITING Number of Lymph Node Retrieved DRUG: injecting Carbon Nanoparticles Suspension|DRUG: injecting indocyanine green LI XIN-XIANG INTERVENTIONAL.
- NCT02123407 Clinical Study on the Harvesting Lymph Nodes With Carbon Nanoparticles for Advanced Gastric Cancer UNKNOWN Advanced Gastric Cancer DRUG: Carbon Nanoparticles|PROCEDURE: Gastrectomy with D2 dissection Peking University INTERVENTIONAL.
- NCT01644890 A Phase III Study of NK105 in Patients With Breast Cancer COMPLETED Breast Cancer Nos Metastatic Recurrent DRUG: NK105|DRUG: Paclitaxel Nippon Kayaku Co., Ltd. INTERVENTIONAL.
- NCT05561036 A Randomized, Double-blind,Phase III Study of Liposome Doxorubicin in Desmoid Tumor RECRUITING tumor.
- NCT00606515 Pharmacokinetics Study of Liposomal Paclitaxel in Humans COMPLETED Cancer DRUG: Liposomal paclitaxel|DRUG: Paclitaxel Shandong Luye Pharmaceutical Co., Ltd. Nanjing Sike Pharmaceutical Co., Ltd. INTERVENTIONAL.
- NCT03817515 Expanded Access for ABI-009 in Patients With Advanced PEComa and Patients With a Malignancy With Relevant Genetic Mutations or mTOR Pathway Activation APPROVED_FOR_MARKETING PEComa, Malignant|TSC1|TSC2|mTOR Pathway Abberation DRUG: ABI-009 Aadi Bioscience, Inc. EXPANDED_ACCESS.
- S. N. Bhatia, X. Chen, M. A. Dobrovolskaia and T. Lammers, Cancer nanomedicine, Nat. Rev. Cancer, 2022, 22(10), 550–556 CrossRef CAS PubMed
. - R. van der Meel, E. Sulheim, Y. Shi, F. Kiessling, W. J. M. Mulder and T. Lammers, Smart cancer nanomedicine, Nat. Nanotechnol., 2019, 14(11), 1007–1017 CrossRef CAS PubMed
. - M. S. Muthu and B. Wilson, Challenges posed by the scale-up of nanomedicines, Nanomedicine, 2012, 7(3), 307–309 CrossRef CAS PubMed
. - A. A. Halwani, Development of Pharmaceutical Nanomedicines: From the Bench to the Market, Pharmaceutics, 2022, 14(1), 106 CrossRef CAS PubMed
. - S. Hua, M. B. C. de Matos, J. M. Metselaar and G. Storm, Current Trends and Challenges in the Clinical Translation of Nanoparticulate Nanomedicines: Pathways for Translational Development and Commercialization, Front. Pharmacol., 2018, 9, 790 CrossRef PubMed
. - J. P. Martins, J. das Neves, M. de la Fuente, C. Celia, H. Florindo and N. Günday-Türeli, et al., The solid progress of nanomedicine, Drug Deliv. Transl. Res., 2020, 10(3), 726–729 CrossRef PubMed
. - R. P. Das, V. V. Gandhi, B. G. Singh and A. Kunwar, Passive and Active Drug Targeting: Role of Nanocarriers in Rational Design of Anticancer Formulations, Clin. Prev. Dent., 2019, 25(28), 3034–3056 CAS
. - P.-B. Broncy, Clinical Impact of Circulating Tumor Cells in Patients with Localized Prostate Cancer, Cells, 2019, 8(7), 676 CrossRef PubMed
. - X. Zhang, Q. Guo and D. Cui, Recent Advances in Nanotechnology Applied to Biosensors, Sensors, 2009, 9(2), 1033–1053 CrossRef CAS PubMed
. - E. Forte, D. Fiorenza, E. Torino, A. Costagliola di Polidoro, C. Cavaliere and P. A. Netti, et al., Radiolabeled PET/MRI Nanoparticles for Tumor Imaging, J. Clin. Med., 2019, 9(1), 89 CrossRef PubMed
. - S. Wahab, M. Y. Alshahrani, M. F. Ahmad and H. Abbas, Current trends and future perspectives of nanomedicine for the management of colon cancer, Eur. J. Pharmacol., 2021, 910, 174464 CrossRef CAS PubMed
. - J. Qin, N. Gong, Z. Liao, S. Zhang, P. Timashev and S. Huo, et al., Recent progress in mitochondria-targeting-based nanotechnology for cancer treatment, Nanoscale, 2021, 13(15), 7108–7118 RSC
. - D. Caputo, D. Pozzi, T. Farolfi, R. Passa, R. Coppola and G. Caracciolo, Nanotechnology and pancreatic cancer management: state of the art and further perspectives, World J. Gastrointest. Oncol., 2021, 13(4), 231–237 CrossRef PubMed
. - L. Tang, J. Li, T. Pan, Y. Yin, Y. Mei and Q. Xiao, et al., Versatile carbon nanoplatforms for cancer treatment and diagnosis: strategies, applications and future perspectives, Theranostics, 2022, 12(5), 2290–2321 CrossRef CAS PubMed
.
Footnote |
† Authors sharing equal authorship includes: M. Joyce Nirmala, Uma Kizhuveetil and Athira Johnson. |
|
This journal is © The Royal Society of Chemistry 2023 |