DOI:
10.1039/D2QI00890D
(Research Article)
Inorg. Chem. Front., 2022,
9, 5140-5147
A new boron cluster anion pillared metal organic framework with ligand inclusion and its selective acetylene capture properties†
Received
25th April 2022
, Accepted 17th July 2022
First published on 19th July 2022
Abstract
The separation of acetylene (C2H2) from carbon dioxide (CO2) and ethylene (C2H4) is important in industry but challenging due to their similar physical properties. Herein, a novel microporous boron cluster pillared metal–organic framework BSF-10 was synthesized with ligand inclusion for efficient C2H2/CO2 and C2H2/C2H4 adsorption separation. The free dipyridyl ligands in the large pore reduce the porosity of BSF-10 but stabilize the framework. The available narrow pores without inclusion of ligands are suited for the accommodation of C2H2 by cooperative dihydrogen bonding. High C2H2 capacity and high C2H2 selectivity over CO2 and C2H4 are achieved. The practical separation ability was confirmed by the breakthroughs using C2H2/CO2 and C2H2/C2H4 gas mixtures with good recyclability. The dynamic separation factor of 2.8 for the equimolar C2H2/CO2 mixture is comparable to those of many benchmark materials.
The separation of C2H2/CO2 and C2H2/C2H4 to produce C2H2 and C2H4 in high purity is important in industry.1–4 C2H2 is a vital fundamental material for the synthesis of various organic chemicals and polymers. Produced from the cracking of hydrocarbons or partial combustion of natural gas, CO2 is a contaminant and needs to be removed.3 On the other hand, the deep removal of C2H2 is a must in the process of ethylene production to obtain polymer grade C2H4 (>99.996%) since trace C2H2 can poison the ethylene polymerization catalysts by forming metal acetylides.4 Current state-of-the-art technologies for the separation and purification of C2H2 from other gases largely rely on cryogenic distillation, partial hydrogenation, or solvent extraction, which are either energy-intensive or associated with pollution. When compared, physisorption separation using porous materials is more energy-efficient and eco-friendly.1,2 However, due to their similar molecular diameter and polarity (Table S1†), efficient C2H2/CO2 and C2H2/C2H4 separation is still difficult to achieve.
The design of advanced porous materials is vital for many applications such as gas separation5 and catalysis.6 Recent years have witnessed families of porous metal organic frameworks (MOFs) with excellent properties for physisorption separations.7–16 In this context, inorganic anion pillared MOFs as a unique subclass with strong Lewis basic functional anion sites, developed firstly by the Zaworotko group17 and Kitagawa group,18 have been of particular interest for selective gas separation due to their high molecular recognition competence. These inorganic anion pillared MOFs can be classified to five series to date according to the anions used: (1) MFSIX networks pillared by hexafluorometallate anions (e.g. SiF62−, GeF62−, and TiF62−),19,20 (2) MOxFy pillared networks with anions containing octahedral metal centers bonded through both O and F atoms (e.g. NbOF52− and TaOF52−),21–23 (3) networks cross-linked by tetrahedral MO42− oxyanions (e.g. CrO42−, MoO42−, SO42−),24 (4) DICRO coordination networks that are sustained with Cr2O72− dianions,25 and (5) boron cluster anion pillared supramolecular metal organic frameworks (BSFs) featuring closo-[B12H12]2− or closo-[B12H11I]2− as the inorganic pillars.26–32
The icosahedral closo-dodecaborate [B12H12]2− is a stable dianionic boron cluster consisting of 12 identical B–H vertices (Fig. 1A).33 It is considered as a three-dimensional aromatic analogue to two-dimensional aromatic benzene due to the electron delocalization.34 While other anions use electronegative F and O to coordinate transition metals, [B12H12]2− utilizes hydride, which is not common in coordination polymers. In 2019, we reported the first BSF material termed BSF-1, which is constructed from closo-[B12H12]2− dianions, Cu2+ ions and 1,2-di(pyridin-4-yl)ethyne (L4, Fig. 1B) with N⋯Cu and B–H⋯Cu coordination as well as B–H⋯H–C dihydrogen bonds (Fig. 1C).26 Every hexacoordinate Cu center serves as a six-connected node. Four pyridyl units from different ligands comprise the equatorial plane and the axial positions of the Cu nodes coordinate to hydrogen atoms from [B12H12]2−, which bridge two Cu nodes to generate an infinite Cu-dodecaborate chain (Fig. 1D). BSF-1 displays high separation selectivity for C3H8/CH4 and C2H6/CH4. The extension of the organic linker to L5 (Fig. 1B) produces a isostructural MOF BSF-3 with improved porosity, which shows superior C2H2 capacity as well as enhanced C2H2/C2H4 and C2H2/CO2 separation selectivity compared to BSF-1.28 BSF-4 with L2 and BSF-9 with L3 were expected to show similar gas separation performance due to the similar length of organic linkers.29,30 However, the interpenetration symmetry difference endows BSF-9 with symmetrical interpenetration mode and a record high C2H2/CO2 separation selectivity in robust MOFs without open metal sites, which is over 5 fold that in BSF-4 with asymmetrical interpenetration mode.30 Another approach to tune the porosity of BSFs is to alter the interpenetration degree. Eliminating the interpenetration of BSFs by controlling the binary solvent system can afford enhanced theoretical porosity. However, these non-interpenetrated MOFs (eg, BSF-5, 6, 7, 8) are actually very unstable and lose most of the porosity after guest removal due to the framework collapse.30 Thus, it is still very challenging to design BSFs with a desirable stable porous structure and the interpenetration mode in BSFs is still difficult to predict and control.
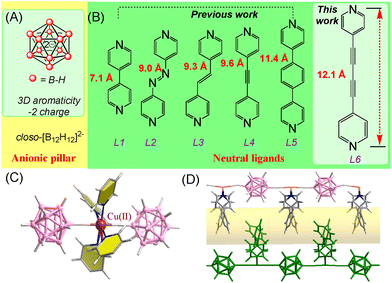 |
| Fig. 1 (A and B) Structure of closo-dodecaborate [B12H12]2− and organic ligands. (C) General coordination mode in BSFs. (D) General 1D pore channels of interpenetrated BSFs. | |
Herein, we would like to report another approach to tune the porosity and stability of BSFs. 1,4-di(pyridin-4-yl)buta-1,3-diyne (L6, Fig. 1B) with a N⋯N distance of 12.1 Å and negligible steric hindrance was utilized as the organic linker. A new boron cluster anion pillared metal organic framework BSF-10 with ligand inclusion was prepared. BSF-10 is asymmetrically interpenetrated. There is one large pore with a square shaped window and two small pores with narrow rectangular shaped windows. In the large pore, L6 is included and unable to be removed by soaking into polar solvents or heating under vacuum. This ligand inclusion reduces the porosity of BSF-10 but enhances the stability for practical applications, which has never been reported in BSFs.6 The available narrow pores without inclusion of ligands are suited for the accommodation of C2H2 by cooperative dihydrogen bonding. Thus, the high capacity of C2H2 (65.0 cm3 g−1) as well as good separation selectivity for C2H2/CO2 (13.52–5.86) and C2H2/C2H4 (4.09–2.93) under ambient conditions are achieved. The practical separation ability was further confirmed by the dynamic breakthroughs using C2H2/CO2 and C2H2/C2H4 gas mixtures with good recyclability. The dynamic separation factor of 2.8 for equimolar C2H2/CO2 mixtures is comparable to those of many benchmark materials.
BSF-10 was readily prepared by stirring a mixture of Na2[B12H12]·2H2O, Cu(NO3)2·3H2O and L6 in MeOH at 35 °C for 48 h. Single crystals of BSF-10 were produced by layering a MeOH solution of L6 onto an aqueous solution of Na2[B12H12]·2H2O and Cu(NO3)2·3H2O. X-ray structural analysis of BSF-10 revealed that it crystallizes in a three-dimensional (3D) framework in the monoclinic space group P21/c (Table S2†). The asymmetric interpenetration leads to the generation of two kinds of pore channels: large Pore I and small Pores II/III (Fig. 2A). The large Pore I features a square shaped window and the small Pores II/III display narrow rectangular shaped windows. Notably, L6 is included in the large pore, and unable to be removed by soaking BSF-10 into polar solvents or heating under vacuum (Fig. 2B). Analysis of the configuration of L6 indicates the existence of two forms of L6 in Pore I with a packing mode of AABB (Fig. 2C). Strong π⋯π interactions among guests as well as multiple weak B–H⋯H–C interaction exist, which stabilize the structure of L6@BSF-10 and prevent the release of L6. Notably, the Cu[B12H12]
:
L6 ratio has no influence on the structure of BSF-10. Even when excess Cu[B12H12] was used, the single crystals still show the composition of Cu[B12H12](L6)3 with free L6 inside Pore I (Table S3†).
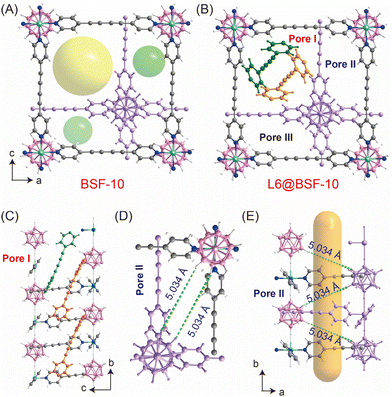 |
| Fig. 2 Porous structure of BSF-10. (A) Generation of two kinds of pores by asymmetrical interpenetration. (B) L6 included in the large Pore I. (C) The packing mode of L6 in Pore I. (D and E) Structure of Pore II in two directions highlighting the closest B–H⋯H–B distance after subtracting the two van der Waals radii of hydrogen. | |
Pore II and Pore III are nearly identical. The closest distance between two opposite B–H units in BSF-10 is 5.034 Å (Fig. 2D and E). This distance is suited to trap an acetylene molecule by cooperative dihydrogen bonding (B–Hδ−⋯Hδ+-CC-Hδ+⋯Hδ−–B)28 and thus be potential for C2H2/CO2 and C2H2/C2H4 separation. Besides, the pore window size of ca. 4 Å (Fig. 3) defined by the opposite H(Py)⋯H(Py) is also close to the diameter of C2H2 (kinetic diameter 3.3 Å, 3D diameter: 3.32 × 3.34 × 5.70 Å3), allowing tight C2H2 accommodation.
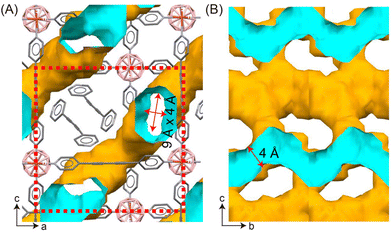 |
| Fig. 3 Voids and pore windows of BSF-10 generated by a probe with the radius of 1.2 Å seen along (A) b-axis and (B) a-axis. The pore window dimensions are determined after subtracting the two van der Waals radii of hydrogen. | |
Inspired by the structural analysis, we were interested to investigate the potential of BSF-10 for the selective adsorption separation of C2H2 from CO2 and C2H4. The first step is to confirm the permanent porosity of BSF-10 after solvent removal as BSFs with larger pores are not stable upon activation.31 N2 gas adsorption experiments at 77 K were conducted after activating BSF-10 under vacuum at 75 °C for 12 h. A typical Type I isotherm was observed, indicating the microporous character of BSF-10. The BET surface area was calculated to be 426.3 m2 g−1 with a total pore volume of 0.216 cm3 g−1 at P/P0 = 0.95, (Fig. 4A), which is quite close to the calculated pore volume of 0.222 cm3 g−1 based on the single crystal structure of BSF-10 using a probe with the radius of 1.2 Å (Fig. 3).
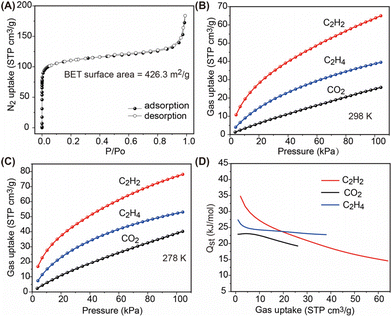 |
| Fig. 4 (A) N2 adsorption and desorption isotherms of BSF-10. (B and C) Single component adsorption isotherms of C2H2, CO2 and C2H4 on BSF-10 at 298 and 278 K. (D) Adsorption heats of C2H2, CO2 and C2H4 on BSF-10. | |
The establishment of permanent microporosity in BSF-10 motivated us to study the gas separation performance for C2H2/CO2 and C2H2/C2H4. First of all, single component adsorption isotherms of C2H2, CO2 and C2H4 were collected under 298 and 278 K. At 1.0 bar, the C2H2/CO2/C2H4 uptakes were 65.0/25.8/39.5 cm3 g−1 at 298 K and 78.1/53.0/40.2 cm3 g−1 at 278 K, respectively (Fig. 4B and C). The isosteric heat of adsorption (Qst) for BSF-10 was then calculated using the Clausius–Clapeyron equation after fitting the isotherms to the Langmuir–Freundlich equation with excellent accuracy (R2 > 0.9999, Tables S4 and 5†). Qst values at near-zero loading for C2H2, CO2, and C2H4 were 34.8, 27.4, and 22.9 kJ mol−1 (Fig. 4D). This trend of Qst values is consistent with the slope of the adsorption isotherms, indicating the preferential adsorption of C2H2 over CO2 and C2H4.
Additionally, the Qst value for C2H2 in BSF-10 is higher than that of BSF-1 (30.7 kJ mol−1), but lower than that of BSF-3 (42.7 kJ mol−1), consistent with the C2H2 adsorption uptake trend of BSF-1 (2.35 mmol g−1) < BSF-10 (2.90 mmol g−1) < BSF-3 (3.59 mmol g−1). Notably, this modest Qst renders BSF-10 a suitable porous material for practical application with a low energy footprint for regeneration.
Since selectivity is as important as capacity to evaluate the separation performance, the C2H2/CO2 and C2H2/C2H4 selectivity on BSF-10 at 298 K and 278 K was calculated using ideal adsorbed solution theory (IAST), which revealed that the selectivity for equimolar C2H2/CO2 and C2H2/C2H4 at 298 K and 1.0 bar was 13.52–5.86 and 4.09–2.93, respectively (Fig. 5A). The selectivity is slightly increased under low pressure when the temperature reduced to 278 K, and is 17.4–5.2 for C2H2/CO2 and 4.8–2.8 for C2H2/C2H4 (Fig. 5B). The C2H2/CO2 selectivity with different C2H2 molar ratios was further calculated for BSF-10, which indicates that the decrease of the C2H2 molar ratio leads to the increased C2H2/CO2 selectivity (Fig. 5C). The C2H2 capacity and the C2H2/CO2 selectivity on BSF-10 are superior to those of many well-performing materials such as BSF-1 (52.5 cm3 g−1, S = 3.4),26 BSF-2 (41.5 cm3 g−1, S = 5.1),27 TCuI (49.3 cm3 g−1, S = 5.3),35 JNU-1 (63 cm3 g−1, S = 5),36 iMOF-7C (15.7 cm3 g−1, S = 4),37 FJU-36a (52.2 cm3 g−1, S = 2.8),38 SNNU-150-In (34.9 cm3 g−1, S = 5.6),39 PCM-48 (25.5 cm3 g−1, S = 4.3),40 and JXNU-5a (55.9 cm3 g−1, S = 5),41 (Fig. 5D), but inferior to those of BSF-3 (80.4 cm3 g−1, S = 16.3).28 The C2H2/CO2 uptake ratio of 2.52 at 298 K and 1 bar is also relatively high. This value is superior to those of most MOFs that separate C2H2/CO2 by a thermodynamic mechanism,42–52 but slightly lower than those of CuI@UiO66-(COOH)2 (2.58),43 MAF-2 (3.68),47 and SIFSIX-21-Ni (3.1)17c as shown in Fig. 6 and Table S8.†
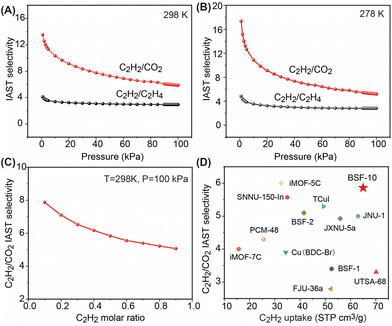 |
| Fig. 5 (A and B) IAST selectivity of C2H2/CO2 and C2H2/C2H4 at 298 and 278 K. (C) IAST selectivity of C2H2/CO2 with different C2H2 molar ratios. (D) Comparison of the C2H2 uptakes and C2H2/CO2 selectivity among BSF-10 and others well-performing MOFs. | |
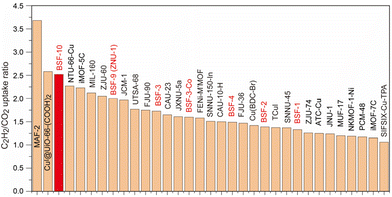 |
| Fig. 6 Comparison of the C2H2/CO2 uptake ratios at 298 K and 1 bar among BSF-10 and other well-performing materials in the context of C2H2/CO2 separation by a thermodynamic mechanism. | |
To confirm the practical separation ability of BSF-10 for C2H2/CO2 and C2H2/C2H4 mixtures, dynamic breakthrough experiments were conducted. 0.233 g of BSF-10 powder was packed into a stainless column with a size of Φ 4.6 mm × 5 cm. After activating the sample by Ar purge at 75 °C for 12 h, an equimolar C2H2/CO2 mixture was introduced. CO2 appeared at the outlet within 12 min while C2H2 was retained in the column until 20 min (Fig. 7A). After complete breakthrough, Ar gas with a flow rate of 5 mL min−1 was used to desorb the adsorbed C2H2 and CO2 gases as well as to regenerate the material. Notably, nearly all the C2H2 and CO2 gases are desorbed within 20 min at 75 °C and the material is regenerated for further use (Fig. 7D). Calculation of the areas of the breakthrough curves and desorption curves indicated that BSF-10 has a dynamic C2H2 capacity of 45 cm3 g−1 with a C2H2/CO2 separation factor of 2.8. This separation factor is slightly lower than that of benchmark materials such as CAU-10-H (3.4),42 CuI@UiO-66-(COOH)2 (3.4)43 and JCM-1 (4.4),44 but higher than that of NKMOF-1-Ni (2.6),45 FJU-90 (2.1)46 and HOF-3a (2).53 Besides, the dynamic C2H2 capacity of BSF-10 is also comparable to that of JCM-1 (49.3 cm3 g−1). The regenerated BSF-10 was further used for repeated tests. Nearly no performance reduction was observed over 3 cycles (Fig. 7B). The same sample was further used for C2H2/C2H4 (1/99) mixture breakthrough experiments. C2H4 was detected at 15 min and C2H2 at 28 min, suggesting a good capture ability of trace C2H2 from bulky C2H4 (Fig. 7C). Desorption curves further indicated that the captured C2H2 can be desorbed within 20 min by Ar purge at 75 °C (Fig. 7D). Combining the high dynamic C2H2 capacity, high separation factor as well as facile regeneration conditions, BSF-10 is a good candidate for practical C2H2/CO2 and C2H2/C2H4 separation.
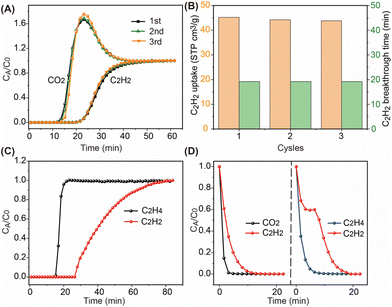 |
| Fig. 7 (A) Experimental breakthrough curves of C2H2/CO2 (50/50) on BSF-10 at 298 K. (B) Comparison of the dynamic C2H2 uptake from the C2H2/CO2 (50/50) mixture and breakthrough time among three cycles of breakthrough experiments. (C) Experimental breakthrough curves of C2H2/C2H4 (1/99) on BSF-10 at 298 K. (D) The desorption curves of BSF-10 after breakthrough experiments of C2H2/CO2 (50/50) mixtures and C2H2/C2H4 (1/99) mixtures. | |
In conclusion, we prepared a novel microporous boron cage pillared metal–organic framework BSF-10 with unprecedented ligand inclusion. Free dipyridyl ligands occupy the large pores and interact with the framework by multiple interactions, which reduces the porosity of BSF-10 but enhances the stability of the framework. The narrow pores without inclusion of ligands are suited for the selective accommodation of C2H2 by cooperative dihydrogen bonding. Thus, high C2H2 capacity and high C2H2 selectivity over CO2 and C2H4 are achieved. The practical separation ability was completely confirmed by the breakthroughs using C2H2/CO2 and C2H2/C2H4 gas mixtures with good recyclability and facile regeneration conditions. The dynamic separation factor of 2.8 for the equimolar C2H2/CO2 mixture is comparable to those of many benchmark materials. Boron cage pillared metal–organic frameworks constructed by non-linear organic linkers are under exploration. Besides, MOFs with dual C2H2/C2H4 and C2H2/CO2 separation ability will be increasingly important for separating multicomponent gas mixtures using a single adsorbent, which currently largely relies on synergistic/tandem packed adsorbents in fixed-beds.54
Conflicts of interest
There are no conflicts to declare.
Acknowledgements
Y. Zhang thanks the support of the Natural Science Foundation of China (No. 21908193) and Jinhua Industrial Key Project (2021A22648). L. Wang thanks the support of Open Research Fund of Key Laboratory of the Ministry of Education for Advanced Catalysis Materials and Zhejiang Key Laboratory for Reactive Chemistry on Solid Surfaces, Zhejiang Normal University (KLMEACM202111). S. Duttwyler thanks the support of the National Natural Science Foundation of China (No. 21871231) and the Special Funds for Basic Scientific Research of Zhejiang University (No. 2019QNA3010 and K20210335).
Notes and references
- D. S. Sholl and R. P. Lively, Seven chemical separations to change the world, Nature, 2016, 532, 435–438 CrossRef PubMed
.
- K. Adil, Y. Belmabkhout, R. S. Pillai, A. Cadiau, P. M. Bhatt, A. H. Assen, G. Maurin and M. Eddaoudi, Gas/vapour separation using ultra-microporous metal-organic frameworks: insights into the structure/separation relationship, Chem. Soc. Rev., 2017, 46, 3402–3430 RSC
.
- W. Sun, J. Hu, Y. Jiang, N. Xu, L. Wang, J. Li, Y. Hu, S. Duttwyler and Y. Zhang, Flexible molecular sieving of C2H2 from CO2 by a new cost-effective metal organic framework with intrinsic hydrogen bonds, Chem. Eng. J., 2022, 439, 135745 CrossRef CAS
.
- X. Cui, K. Chen, H. Xing, Q. Yang, R. Krishna, Z. Bao, H. Wu, W. Zhou, X. Dong, Y. Han, B. Li, Q. Ren, M. J. Zaworotko and B. Chen, Pore chemistry and size control in hybrid porous materials for acetylene capture from ethylene, Science, 2016, 353, 141–144 CrossRef CAS PubMed
.
-
(a) H. Zeng, M. Xie, T. Wang, R.-J. Wei, X.-J. Xie, Y. Zhao, W. Li and D. Li, Orthogonal-array dynamic molecular sieving of propylene/propane mixtures, Nature, 2021, 595, 542–548 CrossRef CAS PubMed
;
(b) Y. Chai, X. Han, W. Li, S. Liu, S. Yao, C. Wang, W. Shi, I. Da-Silva, P. Manuel, Y. Cheng, L. D. Daemen, A. J. Ramirez-Cuesta, C. C. Tang, L. Jiang, S. Yang, N. Guan and L. Li, Control of zeolite pore interior for chemoselective alkyne/olefin separations, Science, 2020, 368, 1002–1006 CrossRef CAS PubMed
;
(c) N. Kumar, S. Mukherjee, A. Bezrukov, M. Vandichel, M. Shivanna, D. Sensharma, A. Bajpai, V. Gascon, K. Otake, S. Kitagawa and M. J. Zaworotko, A square lattice topology coordination network that exhibits highly selective C2H2/CO2 separation performance, SmartMat, 2020, 1, e1008 CrossRef PubMed
;
(d) S. Sharma, S. Mukherjee, A. V. Desai, M. Vandichel, G. K. Dam, A. Jadhav, G. Kociok-Kohn, M. J. Zaworotko and S. K. Ghosh, Efficient Capture of Trace Acetylene by an Ultramicroporous Metal-Organic Framework with Purine Binding Sites, Chem. Mater., 2021, 33, 5800–5808 CrossRef CAS
;
(e) Z. Yao, Z. Zhang, L. Liu, Z. Liu, W. Zhou, Y. Zhao, Y. Han, B. Chen, R. Krishna and S. Xiang, Extraordinary Separation of Acetylene-Containing Mixtures with Microporous Metal-Organic Frameworks with Open O Donor Sites and Tunable Robustness through Control of the Helical Chain Secondary Building Units, Chem. – Eur. J., 2016, 22, 5676–5683 CrossRef CAS PubMed
;
(f) L. Feng, G. S. Day, K. Y. Wang, S. Yuan and H. C. Zhou, Strategies for Pore Engineering in Zirconium Metal-Organic Frameworks, Chem, 2020, 6, 2902–2923 CrossRef CAS
;
(g) Z. Ji, H. Wang, S. Canossa, S. Wuttke and O. M. Yaghi, Pore Chemistry of Metal-Organic Frameworks, Adv. Funct. Mater., 2020, 30, 2000238 CrossRef CAS
.
-
(a) S. Naghdi, A. Cherevan, A. Giesriegl, R. Guillet-Nicolas, S. Biswas, T. Gupta, J. Wang, T. Haunold, B. C. Bayer, G. Rupprechter, M. C. Toroker, F. Kleitz and D. Eder, Selective ligand removal to improve accessibility of active sites in hierarchical MOFs for heterogeneous photocatalysis, Nat. Commun., 2022, 13, 282 CrossRef CAS PubMed
;
(b) T. Qian, C. Zhao, R. Wang, X. Chen, J. Hou, H. Wang and H. Zhang, Synthetic azobenzene-containing metal-organic framework ion channels toward efficient light-gated ion transport at the subnanoscale, Nanoscale, 2021, 13, 17396–17403 RSC
.
- P.-Q. Liao, N.-Y. Huang, W.-X. Zhang, J.-P. Zhang and X.-M. Chen, Controlling guest conformation for efficient purification of butadiene, Science, 2017, 356, 1193–1196 CrossRef CAS PubMed
.
- X. Zhao, Y. Wang, D. S. Li, X. Bu and P. Feng, Metal-Organic Frameworks for Separation, Adv. Mater., 2018, 30, 1705189 CrossRef PubMed
.
- J.-W. Zhang, W.-J. Ji, M.-C. Hu, S.-N. Li, Y.-C. Jiang, X.-M. Zhang, P. Xu and Q.-G. Zhai, A superstable 3p-block metal-organic framework platform towards prominent CO2 and C1/C2-hydrocarbon uptake and separation performance and strong Lewis acid catalysis for CO2 fixation, Inorg. Chem. Front., 2019, 6, 813–819 RSC
.
- R.-B. Lin, L. Li, H.-L. Zhou, H. Wu, C. He, S. Li, R. Krishna, J. Li, W. Zhou and B. Chen, Molecular sieving of ethylene from ethane using a rigid metal-organic framework, Nat. Mater., 2018, 17, 1128–1133 CrossRef CAS PubMed
.
- P.-D. Zhang, X.-Q. Wu, T. He, L.-H. Xie, Q. Chen and J.-R. Li, Selective adsorption and separation of C2 hydrocarbons in a “flexible-robust” metal-organic framework based on a guest-dependent gate-opening effect, Chem. Commun., 2020, 56, 5520–5523 RSC
.
- M. K. Taylor, T. Runčevski, J. Oktawiec, J. E. Bachman, R. L. Siegelman, H. Jiang, J. A. Mason, J. D. Tarver and J. R. Long, Near-Perfect CO2/CH4 Selectivity Achieved through Reversible Guest Templating in the Flexible Metal-Organic Framework Co(bdp), J. Am. Chem. Soc., 2018, 140, 10324–10331 CrossRef CAS PubMed
.
- Y. Zhang, X. Cui and H. Xing, Recent advances in the capture and abatement of toxic gases and vapors by metal-organic frameworks, Mater. Chem. Front., 2021, 5, 5970–6013 RSC
.
- D. Lv, P. Zhou, J. Xu, S. Tu, F. Xu, J. Yan, H. Xi, W. Yuan, Q. Fu, X. Chen and Q. Xia, Recent advances in adsorptive separation of ethane and ethylene by C2H6-selective MOFs and other adsorbents, Chem. Eng. J., 2022, 431, 133208 CrossRef CAS
.
- P. Liu, K. Chen, Y. Chen, X. Wang, J. Yang, L. Li and J. Li, Linker micro-regulation of a Hofmann-based metal-organic framework for efficient propylene/propane separation, Inorg. Chem. Front., 2022, 9, 1082–1090 RSC
.
- H. Li, K. Wang, Y. Sun, C. T. Lollar, J. Li and H.-C. Zhou, Recent advances in gas storage and separation using metal-organic frameworks, Mater. Today, 2018, 21, 108–121 CrossRef CAS
.
-
(a) S. Subramanian and M. J. Zaworotko, Porous Solids by Design: [Zn(4,4′-bpy)2(SiF6)]n·xDMF, a Single Framework Octahedral Coordination Polymer with Large Square Channels, Angew. Chem., Int. Ed. Engl., 1995, 34, 2127–2129 CrossRef CAS
;
(b) S. Mukherjee, N. Kumar, A. A. Bezrukov, K. Tan, T. Pham, K. A. Forrest, K. A. Oyekan, O. T. Qazvini, D. G. Madden, B. Space and M. J. Zaworotko, Amino-Functionalised Hybrid Ultramicroporous Materials that Enable Single-Step Ethylene Purification from a Ternary Mixture, Angew. Chem., Int. Ed., 2021, 60, 10902–10909 CrossRef CAS PubMed
;
(c) N. Kumar, S. Mukherjee, N. C. Harvey-Reid, A. A. Bezrukov, K. Tan, V. Martins, M. Vandichel, T. Pham, P. E. Kruger and M. J. Zaworotko, Breaking the trade-off between selectivity and adsorption capacity for gas separation, Chem, 2021, 7, 3085–3098 CrossRef CAS PubMed
.
- S. Noro, S. Kitagawa, M. Kondo and K. Seki, A New, Methane Adsorbent, Porous Coordination Polymer [{CuSiF6(4,4′-bipyridine)2}n], Angew. Chem., Int. Ed., 2000, 39, 2081–2084 CrossRef PubMed
.
- Y. Jiang, J. Hu, L. Wang, W. Sun, N. Xu, R. Krishna, S. Duttwyler, X. Cui, H. Xing and Y. Zhang, Comprehensive Pore Tuning in an Ultrastable Fluorinated Anion Cross-Linked Cage-Like MOF for Simultaneous Benchmark Propyne Recovery and Propylene Purification, Angew. Chem., Int. Ed., 2022, 61, e20220094 Search PubMed
.
- Q. Dong, X. Zhang, S. Liu, R.-B. Lin, Y. Guo, Y. Ma, A. Yonezu, R. Krishna, G. Liu, J. Duan, R. Matsuda, W. Jin and B. Chen, Tuning Gate-Opening of a Flexible Metal-Organic Framework for Ternary Gas Sieving Separation, Angew. Chem., Int. Ed., 2020, 59, 22756–22762 CrossRef CAS PubMed
.
- X. Cui, Z. Niu, C. Shan, L. Yang, H. Hu, Q. Wang, P. C. Lan, Y. Li, L. Wojtas, S. Ma and H. Xing, Efficient separation of xylene isomers by a guest-responsive metal-organic framework with rotational anionic sites, Nat. Commun., 2020, 11, 5456 CrossRef CAS
.
- A. Cadiau, K. Adil, P. M. Bhatt, Y. Belmabkhout and M. Eddaoudi, A metal-organic framework-based splitter for separating propylene from propane, Science, 2016, 353, 137–140 CrossRef CAS PubMed
.
- L. F. Yang, X. L. Cui, Z. Q. Zhang, Q. W. Yang, Q. L. Ren and H. B. Xing, An Asymmetric Anion-Pillared Metal–Organic Framework as a Multisite Adsorbent Enables Simultaneous Removal of Propyne and Propadiene from Propylene, Angew. Chem., Int. Ed., 2018, 57, 13145–13149 CrossRef CAS PubMed
.
- D. Sensharma, D. J. O’Hearn, A. Koochaki, A. A. Bezrukov, N. Kumar, B. H. Wilson, M. Vandichel and M. J. Zaworotko, The First Sulfate-Pillared Hybrid Ultramicroporous Material, SOFOUR-1-Zn, and Its Acetylene Capture Properties, Angew. Chem., Int. Ed., 2022, 61, e202116145 CrossRef CAS PubMed
.
- H. S. Scott, N. Ogiwara, K.-J. Chen, D. G. Madden, T. Pham, K. Forrest, B. Space, S. Horike, J. J. Perry IV, S. Kitagawa and M. J. Zaworotko, Crystal engineering of a family of hybrid ultramicroporous materials based upon interpenetration and dichromate linkers, Chem. Sci., 2016, 7, 5470–5476 RSC
.
- Y. Zhang, L. Yang, L. Wang, S. Duttwyler and H. Xing, A Microporous Metal-Organic Framework Supramolecularly Assembled from a CuII Dodecaborate Cluster Complex for Selective Gas Separation, Angew. Chem., Int. Ed., 2019, 58, 8145–8150 CrossRef CAS
.
- Y. Zhang, L. Yang, L. Wang and H. Xing, Pillar iodination in functional boron cage hybrid supramolecular frameworks for high performance separation of light hydrocarbons, J. Mater. Chem. A, 2019, 7, 27560–27566 RSC
.
- Y. Zhang, J. Hu, R. Krishna, L. Wang, L. Yang, X. Cui, S. Duttwyler and H. Xing, Rational Design of Microporous MOFs with Anionic Boron Cluster Functionality and Cooperative Dihydrogen Binding Sites for Highly Selective Capture of Acetylene, Angew. Chem., Int. Ed., 2020, 59, 17664–17669 CrossRef CAS PubMed
.
- Y. Zhang, L. Wang, J. Hu, X. Cui and H. Xing, Solvent-dependent supramolecular self-assembly of boron cage pillared metal-organic frameworks for selective gas separation, CrystEngComm, 2020, 22, 2649–2655 RSC
.
- L. Wang, W. Sun, Y. Zhang, N. Xu, R. Krishna, J. Hu, Y. Jiang, Y. He and H. Xing, Interpenetration Symmetry Control Within Ultramicroporous Robust Boron Cluster Hybrid MOFs for Benchmark Purification of Acetylene from Carbon Dioxide, Angew. Chem., Int. Ed., 2021, 60, 22865–22870 CrossRef CAS PubMed
.
- W. Sun, J. Hu, S. Duttwyler, L. Wang, R. Krishna and Y. Zhang, Highly selective gas separation by two isostructural boron cluster pillared MOFs, Sep. Purif. Technol., 2022, 283, 120220 CrossRef CAS
.
- L. Wang, T. Jiang, S. Duttwyler and Y. Zhang, Supramolecular Cu(II)-dipyridyl frameworks featuring weakly coordinating dodecaborate dianions for selective gas separation, CrystEngComm, 2021, 23, 282–291 RSC
.
-
(a) J. C. Axtell, L. M. A. Saleh, E. A. Qian, A. I. Wixtrom and A. M. Spokoyny, Synthesis and Applications of Perfunctionalized Boron Clusters, Inorg. Chem., 2018, 57, 2333–2350 CrossRef CAS PubMed
;
(b) I. B. Sivaev, V. I. Bregadze and S. Sjöberg, Chemistry of closo-Dodecaborate Anion [B12H12]2−: A Review, Collect. Czech. Chem. Commun., 2002, 67, 679–727 CrossRef CAS
;
(c) K. I. Assaf, M. S. Ural, F. Pan, T. Georgiev, S. Simova, K. Rissanen, D. Gabel and W. M. Nau, Water Structure Recovery in Chaotropic Anion Recognition: High-Affinity Binding of Dodecaborate Clusters to γ-Cyclodextrin, Angew. Chem., Int. Ed., 2015, 54, 6852–6856 CrossRef CAS PubMed
;
(d) X. Zhao, Z. Yang, H. Chen, Z. Wang, X. Zhou and H. Zhang, Progress in three-dimensional aromatic-like closo-dodecaborate, Coord. Chem. Rev., 2021, 444, 214042 CrossRef CAS
.
-
(a) Y. Zhang, Y. Sun, F. Lin, J. Liu and S. Duttwyler, Rhodium(III)-Catalyzed Alkenylation-Annulation of closo-Dodecaborate Anions through Double B-H Activation at Room Temperature, Angew. Chem., Int. Ed., 2016, 55, 15609–15614 CrossRef CAS PubMed
;
(b) Y. Zhang, T. Wang, L. Wang, Y. Sun, F. Lin, J. Liu and S. Duttwyler, RhIII-Catalyzed Functionalization of closo-Dodecaborates by Selective B-H Activation: Bypassing Competitive C-H Activation, Chem. – Eur. J., 2018, 24, 15812–15817 CrossRef CAS PubMed
;
(c) Y. Zhang, J. Liu and S. Duttwyler, RhIII-Catalyzed Functionalization of closo-Dodecaborates by Selective B-H Activation: Bypassing Competitive C-H Activation, Eur. J. Inorg. Chem., 2015, 5158–5162 CrossRef CAS
;
(d) P.-F. Cui, X.-R. Liu, Y.-J. Lin, Z.-H. Li and G.-X. Jin, Highly Selective Separation of Benzene and Cyclohexane in a Spatially Confined Carborane Metallacage, J. Am. Chem. Soc., 2022, 144, 6558–6565 CrossRef CAS PubMed
.
- S. Mukherjee, Y. He, D. Franz, S. Q. Wang, W. R. Xian, A. A. Bezrukov, B. Space, Z. Xu, J. He and M. J. Zaworotko, Halogen-C2H2 Binding in Ultramicroporous Metal-Organic Frameworks (MOFs) for Benchmark C2H2/CO2 Separation Selectivity, Chem. – Eur. J., 2020, 26, 4923–4929 CrossRef CAS PubMed
.
- H. Zeng, M. Xie, Y. L. Huang, Y. F. Zhao, X. J. Xie, J. P. Bai, M. Y. Wan, R. Krishna, W. G. Lu and D. Li, Induced Fit of C2H2 in a Flexible MOF Through Cooperative Action of Open Metal sites, Angew. Chem., Int. Ed., 2019, 58, 8515–8519 CrossRef CAS PubMed
.
- S. Dutta, S. Mukherjee, O. T. Qazvini, A. K. Gupta, S. Sharma, D. Mahato, R. Babarao and S. K. Ghosh, Three-in-One C2H2-Selectivity-Guided Adsorptive
Separation across an Isoreticular Family of Cationic Square-Lattice MOFs, Angew. Chem., Int. Ed., 2022, 61, e202114132 CAS
.
- L. Z. Liu, Z. Z. Yao, Y. X. Ye, L. J. Chen, Q. J. Lin, Y. S. Yang, Z. J. Zhang and S. C. Xiang, Robustness, Selective Gas Separation, and Nitrobenzene Sensing on Two Isomers of Cadmium Metal-Organic Frameworks Containing Various Metal-O-Metal Chains, Inorg. Chem., 2018, 57, 12961–12968 CrossRef CAS PubMed
.
- H. Lv, Y. Li, Y. Xue, Y. Jiang, S. Li, M. Hu and Q. Zhai, Systematic Regulation of C2H2/CO2 Separation by 3p-Block Open Metal Sites in a Robust Metal-Organic Framework Platform, Inorg. Chem., 2020, 59(7), 4825–4834 CrossRef CAS PubMed
.
- J. Reynolds, K. Walsh, B. Li, P. Kunal, B. Chen and S. Humphrey, Highly selective room temperature acetylene sorption by an unusual triacetylenic phosphine MOF, Chem. Commun., 2018, 54, 9937–9940 RSC
.
- R. Liu, Q. Y. Liu, R. Krishna, W. J. Wang, C. T. He and Y. L. Wang, Water-Stable Europium 1,3,6,8-Tetrakis(4-carboxylphenyl)pyrene Framework for Efficient C2H2/CO2 Separation, Inorg. Chem., 2019, 58, 5089–5095 CrossRef CAS PubMed
.
- J. Pei, H.-M. Wen, X.-W. Gu, Q.-L. Qian, Y. Yang, Y. Cui, B. Li, B. Chen and G. Qian, Dense Packing of Acetylene in a Stable and Low-Cost Metal-Organic Framework for Efficient C2H2/CO2 Separation, Angew. Chem., Int. Ed., 2021, 60, 25068–25075 CrossRef CAS PubMed
.
- L. Zhang, K. Jiang, L. Yang, L. Li, E. Hu, L. Yang, K. Shao, H. Xing, Y. Cui, Y. Yang, B. Li, B. Chen and G. Qian, Benchmark C2H2/CO2 Separation in an Ultra-Microporous Metal–Organic Framework via Copper(I)-Alkynyl Chemistry, Angew. Chem., Int. Ed., 2021, 60, 15995–16002 CrossRef CAS PubMed
.
- J. Lee, C. Y. Chuah, J. Kim, Y. Kim, N. Ko, Y. Seo, K. Kim, T. H. Bae and E. Lee, Separation of Acetylene from Carbon Dioxide and Ethylene by a Water-Stable Microporous Metal–Organic Framework with Aligned Imidazolium Groups inside the Channels, Angew. Chem., Int. Ed., 2018, 57, 7869–7873 CrossRef CAS PubMed
.
- Y. Peng, T. Pham, P. Li, T. Wang, Y. Chen, K. Chen, K. Forrest, B. Space, P. Cheng, M. Zaworotko and Z. Zhang, Robust Ultramicroporous Metal-Organic Frameworks with Benchmark Affinity for Acetylene, Angew. Chem., Int. Ed., 2018, 57, 10971–10975 CrossRef CAS PubMed
.
- Y. Ye, Z. Ma, R. Lin, R. Krishna, W. Zhou, Q. Lin, Z. Zhang, S. Xiang and B. Chen, Pore Space Partition within a Metal–Organic Framework for Highly Efficient C2H2/CO2 Separation, J. Am. Chem. Soc., 2019, 141, 4130–4136 CrossRef CAS PubMed
.
- J. Zhang and X. Chen, Optimized Acetylene/Carbon Dioxide Sorption in a Dynamic Porous Crystal, J. Am. Chem. Soc., 2009, 131, 5516–5521 CrossRef CAS PubMed
.
- S. Chen, N. Behera, C. Yang, Q. Dong, B. Zheng, Y. Li, Q. Tang, Z. Wang, Y. Wang and J. Duan, A chemically stable nanoporous coordination polymer with fixed and free Cu2+ ions for boosted C2H2/CO2 separation, Nano Res., 2021, 14, 546–553 CrossRef CAS
.
- X. Duan, Q. Zhang, J. Cai, Y. Yang, Y. Cui, Y. He, C. Wu, R. Krishna, B. Chen and G. Qian, A new metal–organic framework with potential for adsorptive separation of
methane from carbon dioxide, acetylene, ethylene, and ethane established by simulated breakthrough experiments, J. Mater. Chem. A, 2014, 2, 2628–2633 RSC
.
- Y. Ye, S. Xian, H. Cui, K. Tan, L. Gong, B. Liang, T. Pjam, H. Pandey, R. Krishna, P. Lan, K. Forrest, B. Space, T. Thonhauser, J. Li and S. Ma, Metal–Organic Framework Based Hydrogen-Bonding Nanotrap for Efficient Acetylene Storage and Separation, J. Am. Chem. Soc., 2022, 144, 1681–1689 CrossRef CAS PubMed
.
- Z. Niu, X. Cui, T. Pham, G. Verma, P. C. Lan, C. Shan, H. Xing, K. A. Forrest, S. Suepaul, B. Space, A. Nafady, A. M. Al-Enizi and S. Ma, A MOF-based Ultra-Strong Acetylene Nano-trap for Highly Efficient C2H2/CO2 Separation, Angew. Chem., Int. Ed., 2021, 60, 5283–5288 CrossRef CAS PubMed
.
- H. Li, C. Liu, C. Chen, Z. Di, D. Yuan, J. Pang, W. Wei, M. Wu and M. Hong, An Unprecedented Pillar-Cage Fluorinated Hybrid Porous Framework with Highly Efficient Acetylene Storage and Separation, Angew. Chem., Int. Ed., 2021, 60, 7547–7552 CrossRef CAS PubMed
.
- P. Li, Y. He, Y. Zhao, L. Weng, H. Wang, R. Krishna, H. Wu, W. Zhou, M. O'Keeffe, Y. Han and B. Chen, A Rod-Packing Microporous Hydrogen-Bonded Organic Framework for Highly Selective Separation of C2H2/CO2 at Room Temperature, Angew. Chem., Int. Ed., 2015, 54, 574–577 CAS
.
-
(a) K. J. Chen, D. G. Madden, S. Mukherjee, T. Pham, K. A. Forrest, A. Kumar, B. Space, J. Kong, Q. Y. Zhang and M. J. Zaworotko, Synergistic sorbent separation for one-step ethylene purification from a four-component mixture, Science, 2019, 366, 241–246 CrossRef CAS PubMed
;
(b) X. Li, J. Wang, N. Bai, X. Zhang, X. Han, L. Silva, C. G. Morris, S. Xu, D. M. Wilary, Y. Sun, Y. Cheng, C. A. Murray, C. C. Tang, M. D. Frogley, G. Cinque, T. Lowe, H. Zhang, A. J. Ramirea-Cuesta, K. M. Thomas, L. W. Bolton, S. Yang and M. Schroder, Refinement of pore size at sub-angstrom precision in robust metal-organic frameworks for separation of xylenes, Nat. Commun., 2020, 11, 4280 CrossRef CAS PubMed
;
(c) J. W. Cao, S. Mukherjee, T. Pham, Y. Wang, T. Wang, T. Zhang, X. Jiang, H. J. Tang, K. A. Forrest, B. Space, M. J. Zaworotko and K. J. Chen, One-step ethylene production from a four component gas mixture by a single physisorbent, Nat. Commun., 2021, 12, 6507 CrossRef PubMed
.
Footnotes |
† Electronic supplementary information (ESI) available. CCDC 2168393 and 2168394. For ESI and crystallographic data in CIF or other electronic format see DOI: https://doi.org/10.1039/d2qi00890d |
‡ These authors contributed equally to this work. |
|
This journal is © the Partner Organisations 2022 |