DOI:
10.1039/D4RA03431G
(Review Article)
RSC Adv., 2024,
14, 20492-20515
Light-driven photocatalysis as an effective tool for degradation of antibiotics
Received
9th May 2024
, Accepted 22nd June 2024
First published on 27th June 2024
Abstract
Antibiotic contamination has become a severe issue and a dangerous concern to the environment because of large release of antibiotic effluent into terrestrial and aquatic ecosystems. To try and solve these issues, a plethora of research on antibiotic withdrawal has been carried out. Recently photocatalysis has received tremendous attention due to its ability to remove antibiotics from aqueous solutions in a cost-effective and environmentally friendly manner with few drawbacks compared to traditional photocatalysts. Considerable attention has been focused on developing advanced visible light-driven photocatalysts in order to address these problems. This review provides an overview of recent developments in the field of photocatalytic degradation of antibiotics, including the doping of metals and non-metals into ultraviolet light-driven photocatalysts, the formation of new semiconductor photocatalysts, the advancement of heterojunction photocatalysts, and the building of surface plasmon resonance-enhanced photocatalytic systems.
1. Introduction
Since antibiotics have the ability to affect humans and natural ecosystems, as well as to cause pathogenic bacteria to acquire antibiotic resistance at microconcentrations, the issue of water contamination via antibiotic residues is of concern globally.1 Treatment for infectious diseases and agricultural productivity2–5 have significantly improved as a result of the widespread use of antibiotics. On the basis of pharmacological characteristics, antibiotics are mainly divided into aminoglycosides, sulfonamides (SAs), glycopeptides macrolides, β-lactams, quinolones and tetracyclines.6 Antibiotics are more difficult to remove because of their strong chemical stability. The parent structure of various antibiotics, classification and their characteristics have been summarized in Table 1.
Table 1 Classification and characteristics of antibiotics
Antibiotic type |
Representative |
Function/hazard |
Ref. |
Tetracyclines |
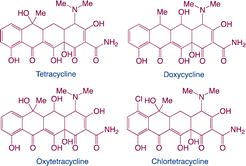 |
Function: tetracyclines prevent livestock illness and promote growth |
7 |
Hazard: result in significant persistence in the aquatic environment; increase the risk of certain infections, which may cause a negative effect on human; disturb the endocrine of aquatic species etc. |
Sulfonamides |
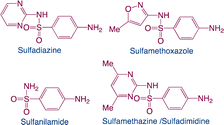 |
Function: sulfonamides are used in human and veterinary medicine as antibacterial, especially in animal husbandry |
8 |
Hazard: the toxicity of sulfonamides is not high to vertebrates. However, it can alter the function of microorganisms living in the environment. Additionally, the toxic effects of sulfonamides and other pollutants could show a synergism |
Fluoroquinolones |
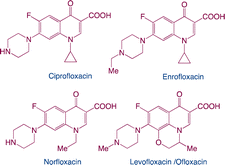 |
Function: fluoroquinolones can kill bacteria or inhibit bacterial growth. Their primary function is to block the replication of DNA by inhibiting the function of DNA helicase. For humans, fluoroquinolones are an essential antibiotic for the treatment of severe invasive infections such as anthrax or plague |
9 |
Hazard: promote resistance formation on microbial populations and induce toxic effects on aquatic organisms |
Macrolides |
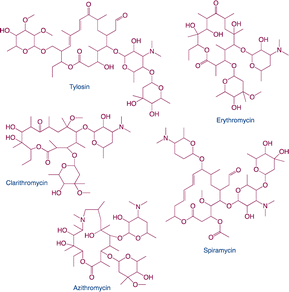 |
Function: macrolides can inhibit bacterial protein synthesis and use to treat upper respiratory tract infections and soft-tissue infectionsHazard: it may cause liver damage using for a long time and result in macrolide resistance |
10 |
β-lactams |
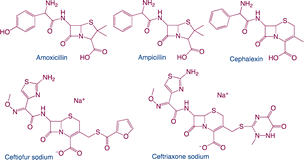 |
Function: β-lactams are used to treat a variety of infections caused by susceptible bacteria, treat human genital tract infections, and serious infections. For animals, they can cure respiratory tract infections and intramammary disturbs |
11 |
Hazard: it may cause an allergic reaction in sensitive person and influent plastid division in lower plants |
Nitroimidazoles |
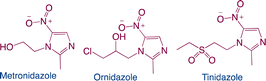 |
Function: nitroimidazoles have antiprotozoal and antibacterial activities as well as strong anti-anaerobic effects |
12 |
Hazard: potential nephrotoxicity, carcinogenesis, and neurotoxicity in human |
Glycopeptides |
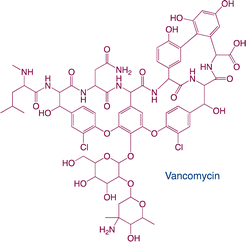 |
Function: glycopeptides are commonly used to treat infections caused by streptococcus or enterococcus |
13 |
Hazard: ototoxicity, nephrotoxicity, allergic reactions etc. |
Aminoglycosides |
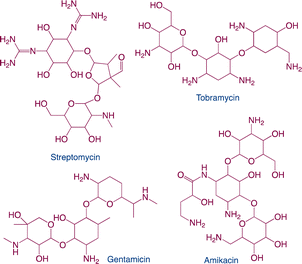 |
Function: aminoglycosides can promote the growth of animals |
14 |
Hazard: high toxicity and nephrotoxicity in human |
Chloramphenicol |
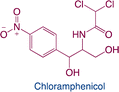 |
Function: chloramphenicol is used for several infectious diseases such as flu bacillus infection |
15 |
Hazard: may cause aplastic anemia and agranulocytosis |
Lincomycin |
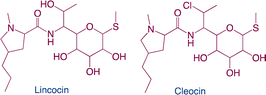 |
Function: lincomycin is applied in food animals for the therapy of dysentery porcine proliferative enteropathies in pig etc. |
16 |
Hazard: allergic reactions etc. |
Pharmaceutical antibiotics usually get poorly absorbed and metabolised by humans as well as animals. The release of polluted water, faeces, and urine from the aforementioned contact spots along with an escalated concentration of antibiotic residues, poses possible risks to the ecosystem (Fig. 1).17 Consequently, the advancement of an affordable and efficient antibiotic decontamination technique is required. Until lately a variety of strategies, including photoelectric Fenton, biological elimination, photocatalytic degradation, membrane filtering, and adsorption, have been used to remediate antibiotic wastewater contaminants.18a–h In the realm of environmental remediation, photocatalytic technology is widely employed to oxidise antibiotics into molecules that are easily biodegradable, less hazardous, and even harmless due to which it has received much concern from scientists.18i,j As we continue our work on photocatalyzed organic synthesis,19,20 this article provides an overview of current developments in the state-of-the-art design and production of photocatalysts with visible light sensitivity for the photocatalytic degradation of wastewater containing antibiotics.
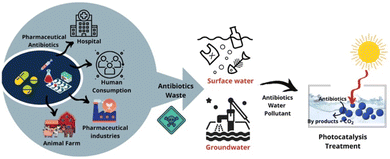 |
| Fig. 1 Schematic representation of antibiotics consumption routes and impact on water bodies along with proposal of treating the same with solar energy-driven photocatalysis technique. Reproduced with permission from ref. 17. Copyright 2021 Elsevier Publishers. | |
2. Methods for antibiotic degradation
There are now multiple techniques to remove antibiotic residues in water and wastewater before releasing them back into the environment. The primary approaches employed as of right now includes both long-standing methods and more contemporary ideas.21–24 Unfortunately, substantial mineralization is either extremely difficult to attain or would take excessively prolonged. Because of their poor selectivity, these techniques can have the unintended consequence of killing non-target creatures that leads to unintended damages.25,26 This approach also has significant operating and capital expenditures. When removing antibiotic residues from water, a combination of chemical and physical degradation methods can greatly lower the toxicity of treated effluents. However, these techniques are expensive and complicated.27
Conversely, having a distinct advantages of photocatalysis, makes it a viable option for environmental remediation because of its (1) easily attainable reaction conditions (i.e., almost ambient temperature and pressure), its ability to use air oxygen as a potent oxidant, and its ability to use solar radiation as an energy source; (2) the potential complete breakdown of organic pollutants into harmless inorganic molecules like carbon dioxide and water; and (3) its strong redox ability, low cost, lack of adsorption saturation, and long durability. As a result, photocatalysis has attracted attention from all around the world and been widely used in innovative methods of energy extraction and environmental control. Several methods28–47 for antibiotic degradation have been reported incorporating materials, operating conditions and disadvantages of antibiotics.
3. General mechanism of photocatalytic antibiotics degradation
Techniques have been developed to treat contaminated water and waste water with organic pollutants. Fig. 2 depicts the mechanism of the photocatalytic degradation. An equivalent number of positively charged holes are produced in the valence band (VB) of a semiconductor when it is subjected to radiation with energy greater than its optical band gap. This is caused by excited electrons that are moved from the VB to the CB. When the potential of VB vs. NHE is more positive than H2O/OH˙(+2.72 V vs. NHE) or OH−/˙OH(+1.89 V vs. NHE) and the potential of CB vs. NHE is more negative than O2/˙O2− (−0.33 V vs. NHE), the semiconductor will be able to generate OH˙ and ˙O2−. After that, the photoinduced electrons and holes separate out and go to the semiconductor's surface, where redox reactions take place at the reactive site (Fig. 2).21,48 The reaction mechanisms of semiconductor photocatalysis are typically expressed by the following equations:49 |
semiconductor + light energy (λ ≥ Eg) → semiconductor (ecb−+hvb+)
| (1) |
|
hvb+ + H2O → H+ + ˙OH (H2O/˙OH| + 2.72 V vs. NHE)
| (2) |
|
hvb+ + OH− → ˙OH (OH−/˙OH| + 1.89 V vs. NHE)
| (3) |
|
ecb− + O2 → ˙O2−(O2/˙O2−| − 0.33 V vs. NHE)
| (4) |
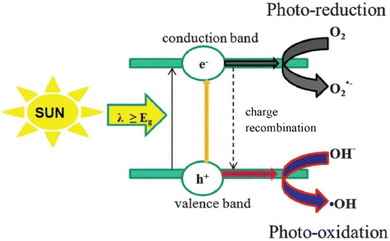 |
| Fig. 2 General mechanism of the semiconductor photocatalytic degradation of organic pollutants. Reproduced with permission from ref. 21. Copyright 2020 Elsevier B.V. All rights reserved. | |
By these chemical processes solar energy can be directly converted and utilized. The consequences of photocatalytic activity are, however, lessened by restricted optical usage and the rapid annihilation of photoexcited electron–hole pairs. If photocatalysts satisfy the following requirements, they can overcome these deficiencies: (1) suitable spectral absorption range; (2) appropriate band energy structure for sufficient electron–hole pair separation and transport; and (3) sufficient active sites for adsorption or reaction.50 To increase photocatalytic efficiency, it is imperative to meet the three previously mentioned requirements. Several attempts have been made to methodically design photocatalysts and enhance photocatalytic dynamics.
An acceptor is reduced by this excited electron, and donor molecules are oxidised by the acceptor's hole. The redox levels of the substrate51–64 and the respective locations of the semiconductor's valence and conduction bands determine what happens to the excited electron and hole.
While considering photoabsorption capability and photocatalytic efficiency, optical bandgap (Eg) plays a crucial role in predicting the applicability and efficacy of a particular type of photocatalytic material. Polyfluorene co-polymers acting as photocatalysts65,66 are classified as photonic and electrochemical bandgaps by Ghaedi et al., who also proposed a method and criterion for bandgap measurement. Furthermore, they came to the conclusion that by keeping charges from recombining, the active holes' lifetime would increase and their ability to degrade antibiotics would be improved. This approach to the interfacial charge transfer from a distinct energy surface to a molecular continuous surface from solids65,66 turned out to be highly effective in increasing the activity of photocatalysts under visible light.
Overall, the process of photocatalysis for the degradation of antibiotics can be broken down into five primary steps: (1) the antibiotics are transferred from the fluid phase to the surface; (2) they are adsorbed; (3) a reaction occurs in the adsorbed phase; (4) the products are desorption; and (5) the products are removed from the interface region.67,68 However, when the electrons that had been excited to CB quickly recombine with the separated holes in the VB before producing free radicals, photocatalytic degradation suffers from the issue of electron–hole recombination in the photocatalyst.68 Adoption of particular photocatalysts with a low CB–VB bandgap energy and photocatalyst modifications are proposed as solutions for these issues, however this depends on numerous variable alternatives, such as tailored experimental conditions.69,70
4. Synthesis techniques of nanostructured photocatalysts
Several synthesis techniques have been used as summarised in Fig. 3. It is noteworthy that the following characteristics are essential for an efficient photocatalyst: (a) robust absorption of visible and UV light (i.e., a suitable bandgap value, typically less than 3.0 eV); (b) stability against photocorrosion in terms of temperature, chemical composition, and mechanical properties; (c) high efficiency in quantum conversion; (d) rapid generation and efficient transfer of photocarriers (e− and h+); and (e) slow recombination rate of photogenerated charge carriers. Additionally, the nanopowder photocatalysts must be able to rapidly and easily recover from the solution while maintaining a sufficient level of reusability, or without noticeably losing effectiveness. To achieve the listed attributes, many tactics are now employed, such as tuning of particle dimensions, morphology, and size. Moreover, different photocatalyst compositions result in heterojunctions, composites, core–shell structures, element substitutions, intercalation compounds, and plasmon sensitization.51,71–75
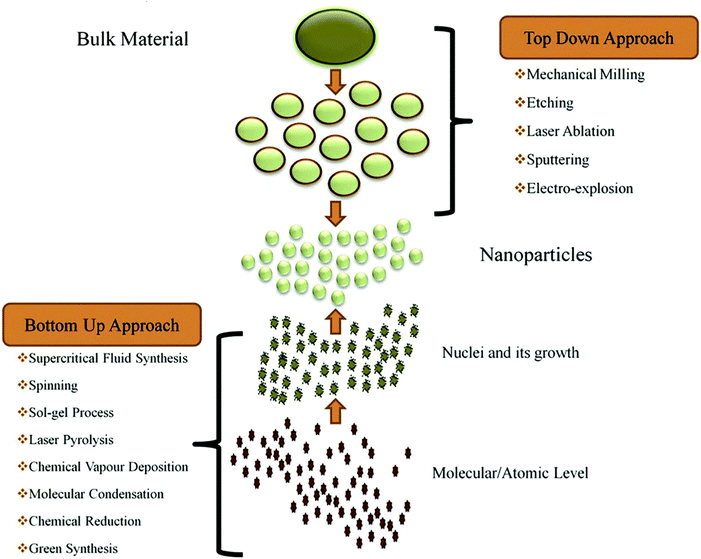 |
| Fig. 3 Synthesis techniques of nanostructured photocatalysts. Reproduced with permission from ref. 75a. Copyright ©2019 Elsevier B.V. All rights reserved. | |
5. Photocatalytic degradation of different antibiotics
5.1. Photocatalytic degradation of tetracyclines
Tetracycline is a broad-spectrum antibiotic that is commonly used to treat a wide range of illnesses. Because of its high efficacy and low cost, it is regarded as the second most frequently used antibiotic in human activities and livestock breeding.75–78 On the other hand, prolonged and excessive TC usage pollutes the environment and is a major social concern.79 Tetracycline has been removed using a variety of methods, such as adsorption,80 ion exchange,81 membrane filtering,82 biological processes,83 electrolysis,84 ozonation,85 advanced oxidation processes,86 and photocatalysis.87 The most efficient, affordable, simple to implement, and environmentally benign of these processes are thought to be the photocatalysis and advanced oxidation processes. Generating charges such as holes, hydroxyl radicals, electrons, and superoxide anion radicals efficiently is essential to the photocatalysis process. Again, the exciton creation and its subsequent dissociation into photo-induced electrons and holes are prerequisites for the production of hydroxyl radical and superoxide anion radical.
Tetracyclines are generally used worldwide. They have four linked rings with several ionizable functional groups. The most widely used tetracyclines are oxytetracycline, tetracycline, and chlortetracycline. The degradation mechanisms of tetracyclines are more intricate because of their complex molecular structure.77 Tetracycline degradation processes under various photocatalytic systems are summarised in Fig. 4. Tetracyclines are commonly degraded via four different processes: hydroxylation, deamidation, N-demethylation, and dehydration. Table 2 comprises a summary of the information regarding the photocatalytic degradation of tetracyclines using various photocatalysts.
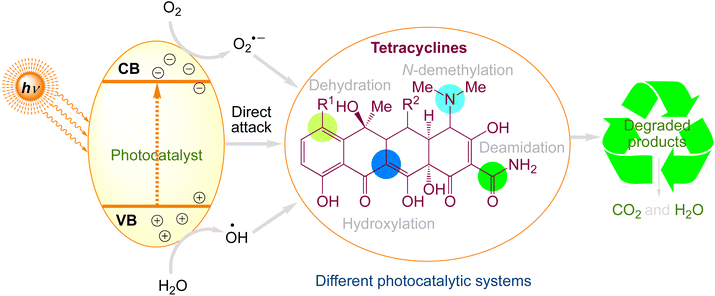 |
| Fig. 4 The proposed photocatalytic degradation pathways of tetracyclines. | |
Table 2 Photocatalytic degradation of tetracyclines at different conditions
Target antibiotic |
Photocatalyst |
Source of light |
Optimum conditions |
Degradation (%) |
Ref. |
Initial concentration |
Catalyst concentration |
Tetracycline |
C dots modified MoO3/g-C3N4 |
Visible light |
20 mg L−1 |
0.6 g L−1 |
88.4% (90 min) |
88 |
Tetracycline |
g-C3N4/Hydroxyapatite |
Simulated sunlight |
50 mg L−1 |
1 g L−1 |
Almost 100% (15 min) |
89 |
Tetracycline |
β-Bi2O3/g-C3N4 core/shell nanocomposites |
Visible light |
10 mg L−1 |
0.5 g L−1 |
80.2% (50 min) |
90 |
Tetracycline |
rGO/g-C3N4/BiVO4 |
Visible light |
35 mg L−1 |
1 g L−1 |
72.5% (150 min) |
91 |
Tetracycline |
C-doped C3N4/Bi12O17Cl2 |
Visible light |
20 mg L−1 |
1 g L−1 |
94.0% (60 min) |
92 |
Tetracycline |
CeVO4/3D rGO aerogel/BiVO4 |
Visible light |
20 mg L−1 |
0.5 g L−1 |
100% (60 min) |
93 |
Tetracycline |
NGQDs-BiOI/MnNb2O6 |
Visible light |
10 mg L−1 |
0.5 g L−1 |
87.2% (60 min) |
94 |
Tetracycline |
TiO2/g-C3N4 |
Simulated sunlight |
20 mg L−1 |
1 g L−1 |
100% (9 min) |
95 |
Tetracycline |
Amorphous TiO2/mesoporous-rutile TiO2 |
UV light |
50 mg L−1 |
0.5 g L−1 |
81.1% (300 min) |
96 |
Tetracycline |
Magnetic Fe2O3 ultrathin nanosheets/mesoporous black TiO2 |
Simulated sunlight |
10 mg L−1 |
0.3 g L−1 |
99.3% (50 min) |
97 |
Tetracycline |
Bi5FeTi3O15 |
Visible light |
5.0 mg L−1 |
0.4 g L−1 |
99.4% (60 min) |
98 |
Tetracycline |
Bi2WO6/CuBi2O4 |
Visible light |
15 mg L−1 |
0.5 g L−1 |
91.0% (60 min) |
99 |
Tetracycline |
AgI/BiVO4 |
Visible light |
20 mg L−1 |
3 g L−1 |
94.9% (60 min) |
100 |
Tetracycline |
AgI/WO3 |
Visible light |
35 mg L−1 |
3 g L−1 |
75.0% (60 min) |
101 |
Tetracycline |
Ag3VO4/WO3 |
Visible light |
10 mg L−1 |
0.5 g L−1 |
71.2% (30 min) |
102 |
Tetracycline |
Ag3PO4/Zn–Al LDH |
Simulated sunlight |
40 mg L−1 |
1 g L−1 |
96% (90 min) |
103 |
Tetracycline |
FeNi3/SiO2/CuS |
UV light |
10 mg L−1 |
5 g L−1 |
96.7% (90 min) |
104 |
Tetracycline |
Fe-based MOFs |
Visible light |
50 mg L−1 |
0.5 g L−1 |
96.6% (180 min) |
105 |
Tetracycline |
Pb/MoO4 |
Simulated sunlight |
20 mg L−1 |
1 g L−1 |
99.0% (120 min) |
106 |
Tetracycline |
Modified red mud |
Visible light |
10 mg L−1 |
|
88.4% (80 min) |
107 |
Tetracycline |
SnO2/g-C3N4 |
Visible light |
30 mg L−1 |
3 g L−1 |
95.9% (120 min) |
108 |
Tetracycline |
RGO-CdTe |
Visible light |
30 mg L−1 |
3 g L−1 |
83.6% (45 min) |
109 |
Tetracycline |
Cu2O–TiO2 |
Visible light |
100 mg L−1 |
1.5 g L−1 |
100% (60 min) |
110 |
Tetracycline |
Bi2Sn2O7/β-Bi2O3 |
Visible light |
40 mg L−1 |
2 g L−1 |
95.5% (60 min) |
111 |
Tetracycline |
MoS2/TiO2 |
Visible light |
10 mg L−1 |
0.1 g L−1 |
95.0% (100 min) |
112 |
Tetracycline |
Bi2Sn2O7/Bi2MoO6 |
Visible light |
35 mg L−1 |
0.02 g L−1 |
98.7% (100 min) |
113 |
Tetracycline |
Ti3C2@TiO2 |
Visible light |
20 mg L−1 |
|
90.0% (90 min) |
114 |
Tetracycline |
NiCo–S@CN |
Solar light |
100 mg L−1 |
2 g L−1 |
99.0% (60 min) |
115a |
Tetracycline |
Bi2Sn2O7/Bi2MoO6 |
Visible light |
20 mg L−1 |
0.035 g L−1 |
98.7% (100 min) |
115b |
Tetracycline |
Bi2WO6/Ta3N5 |
Visible light |
20 mg L−1 |
0.04 g L−1 |
86.7% (120 min) |
115c |
Tetracycline |
Ag/Ag2S/Bi2MoO6 |
Visible light |
20 mg L−1 |
0.03 g L−1 |
87.3% (120 min) |
115d |
Oxytetracycline |
Au–CuS–TiO2 nanobelts |
Simulated sunlight |
5.0 mg L−1 |
0.114 cm2 ml−1 |
96.0% (60 min) |
116 |
Oxytetracycline |
N–TiO2/graphene |
UV light |
30 mg L−1 |
|
63.0% (160 min) |
117 |
Oxytetracycline |
Ag3PO4/TiO2/MoS2 |
Visible light |
5 mg L−1 |
0.5 g L−1 |
90.0% |
118 |
Oxytetracycline |
Ti-MCM-41 |
UV light |
50 mg L−1 |
1 g L−1 |
92.0% (180 min) |
119 |
Oxytetracycline |
g-C3N4 |
Visible light |
20 mg L−1 |
0.3 g L−1 |
79.3% (60 min) |
120 |
Oxytetracycline |
Fe2.8Ce0.2O4/GO |
Visible light |
30 mg L−1 |
0.8 g L−1 |
82.0% (120 min) |
121 |
Oxytetracycline |
Rhombohedral corundum-type In2O3 |
UV light |
10 mg L−1 |
1 g L−1 |
89.5% (120 min) |
122 |
Oxytetracycline |
SnO2/BiOI |
Visible light |
10 mg L−1 |
1 g L−1 |
94.6% (90 min) |
123 |
Oxytetracycline |
MU-0.15 |
Simulated sunlight |
20 mg L−1 |
0.5 g L−1 |
86.6% (120 min) |
124 |
Oxytetracycline |
CoFe@NSC-1000 |
Visible light |
50 mg L−1 |
0.3 g L−1 |
82.7% (150 min) |
125 |
Oxytetracycline |
Fe3O4/rGO/Co-doped ZnO/g-C3N4 |
Visible light |
30 mg L−1 |
0.16 g L−1 |
82.0% (70 min) |
126 |
Oxytetracycline |
BiOI/NH2-MIL125(Ti) |
Visible light |
10 mg L−1 |
0.5 g L−1 |
96.2% (60 min) |
127 |
Oxytetracycline |
MnFe2O4/g-C3N4 |
Visible light |
10 mg L−1 |
|
80.5% (10 min) |
128 |
Oxytetracycline |
MIL-100(Fe) |
Visible light |
25 mg L−1 |
0.05 g L−1 |
99.0% (240 min) |
129 |
Oxytetracycline |
Ag/BiVO4/GO |
Visible light |
40 mg L−1 |
0.4 g L−1 |
90.43% (70 min) |
130 |
Oxytetracycline |
TiO2 |
Visible light |
10 mg L−1 |
0.5 g L−1 |
95.0% (180 min) |
131 |
Oxytetracycline |
MnFe2O4/g-C3N4 |
Visible light |
10 mg L−1 |
|
90.0% (1 min) |
132 |
Doxycycline |
SnO2/BiOI |
Visible light |
10 mg L−1 |
1 g L−1 |
90.0% (60 min) |
133 |
Doxycycline |
Ag/AgCl/CdMoO4 |
UV light |
10 mg L−1 |
|
82.4% (60 min) |
134 |
Doxycycline |
α-Bi2O3/g-C3N4 + H2O2 |
Visible light |
25 mg L−1 |
0.01 g L−1 |
79.0% (30 min) |
135 |
Doxycycline |
TiO2-MCM-41 |
UV light |
10 mg L−1 |
0.15 g L−1 |
85.0% (60 min) |
136 |
Doxycycline |
In2O3/g-C3N4 |
Simulated sunlight |
10 mg L−1 |
|
99.3% (60 min) |
137 |
Doxycycline |
Cu2O/SrBi4Ti4O15 |
Visible light |
40 mg L−1 |
|
92.2% (60 min) |
138 |
Chlorotetracycline |
N–TiO2/graphene |
UV light |
30 mg L−1 |
|
54.0% (160 min) |
139 |
Chlorotetracycline |
Bi2O3/MIL101(Fe) |
Visible light |
20 mg L−1 |
0.3 g L−1 |
88.2% (120 min) |
140 |
5.2. Photocatalytic degradation of sulfonamides
Sulfonamides are a class of synthetic pharmaceuticals that emerged in 1906 and contain the sulfonamide chemical group. Since 1940, more than 150 of these agents have been utilised as antimicrobials, making them the most commonly used antibiotics in the field of medicine with good hydrophilicity.141,142 Among these, sulfanilamide, sulfadiazine, sulfamethazine/sulfadimidine, and sulfamethoxazole are frequently used. These contaminants alter the biological population, which could have an adverse effect on human health. Numerous studies indicate that the paths and capabilities of sulfonamide degradation are connected to their substituents.143 Fig. 5 concludes the sulfonamide degradation routes in different photocatalytic systems. Sulfonamides would degrade primarily due to sulfonamide cleavage of the S–N and C–N bonds, amino group oxidation, hydroxylation, and cleavage of the S–C bond between the sulphur and benzene ring by attacking radicals, which would progressively produce the corresponding byproducts.77 Table 3 provides an overview of the results of the efficient degradation of sulphonamides using semiconductor photocatalytic technology.
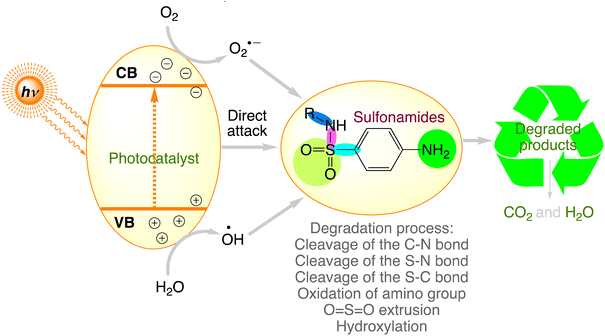 |
| Fig. 5 The proposed photocatalytic degradation pathways of sulfonamides. | |
Table 3 Photocatalytic degradation of sulfonamides at different conditions
Target antibiotic |
Photocatalyst |
Source of light |
Optimum conditions |
Degradation (%) |
Ref. |
Initial concentration |
Catalyst concentration |
Sulfamethoxazole |
Doped metals (Na, K, Ca, Mg) on g-C3N4 |
Visible light |
5.0 mg L−1 |
0.05 g L−1 |
g-CN–K > g-CN–Na > g-CN–Mg > g-CN–Ca > g-CN |
144 |
Sulfamethoxazole |
Ag–P co-doped-g-C3N4 |
Visible light |
5.0 mg L−1 |
1.0 g L−1 |
99% (30 min) |
145 |
Sulfamethoxazole |
Ag/P-g-C3N4 |
Visible light |
0.1 mg L−1 |
0.1 g L−1 |
100% (20 min) |
146 |
Sulfamethoxazole |
Ag/g-C3N4/Bi3TaO7 |
Visible light |
5.0 mg L−1 |
0.5 g L−1 |
98% (25 min) |
147 |
Sulfamethoxazole |
rGO/WO3 |
Visible light |
10.0 mg L−1 |
2.0 g L−1 |
98.0% (180 min) |
148 |
Sulfamethoxazole |
Ag3PO4/N-doped rGO |
Visible light |
20.0 mg L−1 |
0.2 g L−1 |
93.8% (60 min) |
149 |
Sulfamethoxazole |
TiO2-rGO |
Simulated sunlight |
0.10 mg L−1 |
0.1 g L−1 |
87.0 ± 4% (60 min) |
150 |
Sulfamethoxazole |
TiO2 supported on reed straw biochar |
UV light |
10.0 mg L−1 |
1.25 g L−1 |
91.3% (180 min) |
151 |
Sulfamethoxazole |
W Modified TiO2 |
Simulated sunlight |
1.0 mg L−1 |
0.25 g L−1 |
100% (90 min) |
152 |
Sulfamethoxazole |
F–Pd co-doped-TiO2 |
Simulated sunlight |
30.0 mg L−1 |
1.0 g L−1 |
94.2% (20 min) |
153 |
Sulfamethoxazole |
p(HEA/NMMA)-CuS |
UV light |
50.0 mg L−1 |
2.0 g L−1 |
95.9% (24 h) |
154 |
Sulfamethoxazole |
ZnO/fluoride ions |
UV light |
250.0 mg L−1 |
1.5 g L−1 |
97.0% (30 min) |
155 |
Sulfamethoxazole |
Mn-WO3 |
LED light |
3.25 mg L−1 |
2.3 g L−1 |
100% (70 min) |
156 |
Sulfamethoxazole |
Co–CuS@TiO2 |
Solar light |
5.0 mg L−1 |
1.0 g L−1 |
100% (120 min) |
157 |
Sulfamethoxazole |
ZnO/ZnIn2S4 |
Visible light |
2.5 mg L−1 |
0.20 g L−1 |
74.9% (6.5 h) |
158 |
Sulfamethoxazole |
TiO2-based materials |
Sunlight or LED |
10.0 mg L−1 |
|
90.0% (30 min) |
159 |
Sulfamethoxazole |
TiO2/BC |
UV light |
30.0 mg L−1 |
0.02 g L−1 |
89.0% (60 min) |
160 |
Sulfamethoxazole |
PAN-TiO2 and PAN-rGTi |
Solar light |
5.0 mg L−1 |
|
100% (120 min) |
161 |
Sulfamethoxazole |
Fe2O3/g-C3N4 |
Visible light |
10.0 mg L−1 |
0.3 g L−1 |
99.2% (30 min) |
162 |
Sulfamethoxazole |
P–TiO2/g-C3N4 |
Visible light |
10.0 mg L−1 |
0.7 g L−1 |
99.0% (90 min) |
163 |
Sulfamethoxazole |
TiO2@Fe2O3@g-C3N4 (MFTC) |
Solar light |
10.0 mg L−1 |
0.5 g L−1 |
96.8% (120 min) |
164 |
Sulfamethoxazole |
Pd–BiVO4 |
Visible light |
10 mg L−1 |
|
98.8% (210 min) |
165 |
Sulfamethoxazole |
CoP/BVO |
Simulated sunlight |
500 mg L−1 |
1.0 g L−1 |
89.0% (180 min) |
166 |
Sulfamethoxazole |
MoS2@CoS2 |
Visible light |
20.0 mg L−1 |
|
95.0% (80 min) |
167 |
Sulfamethoxazole |
ZrFe2O4@ZIF-8 |
Visible light |
5.0 mg L−1 |
0.02 g L−1 |
100% (180 min) |
168 |
Sulfamethoxazole |
CN/N2PG-0.02 |
Simulated sunlight |
10 mg L−1 |
|
90.0% (120 min) |
169 |
Sulfamethoxazole |
g-C3N4/GSBC |
Visible light |
10.0 mg L−1 |
|
87.2% (90 min) |
170 |
Sulfamethoxazole |
Pt/PtOx/BiVO4 |
Visible light |
10.0 mg L−1 |
0.5 g L−1 |
99.0% (150 min) |
171 |
Sulfamethoxazole |
Fe–Co/γ-Al2O3 |
UV light |
10 mg L−1 |
1.0 g L−1 |
98.0% (60 min) |
172 |
Sulfamethoxazole |
Sulfur-doped-Bi2O3/MnO2 (S-BOMO) |
Visible light |
5.0 mg L−1 |
0.5 g L−1 |
86.0% (240 min) |
173 |
Sulfamethoxazole |
Ag3PO4 |
UV light |
20.0 mg L−1 |
|
99.9% (60 min) |
174 |
Sulfamethoxazole |
Cd doped γ-Bi2MoO6 (Cd-BMO) |
Visible light |
5.0 mg L−1 |
0.05 g L−1 |
97.9% (210 min) |
175 |
Sulfamethoxazole |
AgNbO3 |
Visible light |
10.0 mg L−1 |
0.5 g L−1 |
98.0% (8 h) |
176 |
Sulfamethoxazole |
Fc@rGO-ZnO |
UV light |
10 mg L−1 |
|
95.0% (180 min) |
177 |
Sulfamethoxazole |
CoFe2O4/PMS |
UV light |
10 mg L−1 |
0.1 g L−1/0.4 g L−1 |
91.0% (10 min) |
178 |
Sulfamethazine |
g-C3N4 |
Visible light |
10.0 mg L−1 |
0.5 g L−1 |
95.0% (24 h) |
179 |
Sulfamethazine |
g-C3N4 |
Visible light |
10.0 mg L−1 |
1.0 g L−1 |
97.0% (60 min) |
180 |
Sulfamethazine |
g-C3N4 |
Visible light |
30.0 mg L−1 |
0.5 g L−1 |
99.7% (60 min) |
181 |
Sulfamethazine |
C Doping g-C3N4 |
Visible light |
10.0 mg L−1 |
1.0 g L−1 |
98.0% (60 min) |
182 |
Sulfamethazine |
2D/1D g-C3N4/TNTs |
Visible light |
5.0 mg L−1 |
0.2 g L−1 |
100% (5 h) |
183 |
Sulfamethazine |
TiO2 |
UV light |
20.0 mg L−1 |
0.5 g L−1 |
61.0% (120 min) |
184 |
Sulfamethazine |
AgI/Bi4V2O11 |
Visible light |
10.0 mg L−1 |
0.1 g L−1 |
91.5% (60 min) |
185 |
Sulfamethazine |
Bi2WO6/RGO |
Simulated sunlight |
10.0 mg L−1 |
|
57.6% (8 h) |
186 |
Sulfamethazine |
Graphene aerogel/Bi2WO6 |
Simulated sunlight |
10.0 mg L−1 |
|
55.8% (120 min) |
187 |
Sulfamethazine |
W10O324− |
Visible light |
13.9 mg L−1 |
0.33 g L−1 |
85.0% (4 h) |
188 |
Sulfamethazine |
g-C3N4/Cu, N–TiO2 |
Simulated sunlight |
10 mg L−1 |
|
95.8% (240 min) |
189 |
Sulfamethazine |
Cu–CuxO/TiO2 |
Visible light |
10 mg L−1 |
|
98.2% (60 min) |
190 |
Sulfamethazine |
PhC2Cu/Ag/Ag2MoO4 (PAM) |
Visible light |
10.0 mg L−1 |
0.4 g L−1 |
97.7% (20 min) |
191 |
Sulfamethazine |
G-CDs |
Simulated sunlight |
10.0 mg L−1 |
|
94.0% (75 min) |
192 |
Sulfanilamide |
WO3/Ag |
Visible light |
10.0 mg L−1 |
0.5 g L−1 |
96.2% (5 h) |
193 |
Sulfanilamide |
Ag/ZnFe2O4/Ag/BiTa1−xVxO4 |
Visible light |
10.0 mg L−1 |
1.0 g L−1 |
100% (6 h) |
194 |
Sulfanilamide |
Mo–BiOBr |
Visible light |
10.0 mg L−1 |
0.3 g L−1 |
48.3% (80 min) |
195 |
Sulfadiazine |
BiOCl–Au–CdS |
Simulated sunlight |
20.0 mg L−1 |
1.0 g L−1 |
100% (240 min) |
196 |
Sulfadiazine |
Cu2O/Bi/Bi2MoO6 |
Visible light |
10.0 mg L−1 |
|
98.6% (100 min) |
197 |
Sulfadiazine |
Porous g-C3N4 with C vacancies |
Visible light |
5.0 mg L−1 |
0.02 g L−1 |
100% (20 min) |
198 |
Sulfadiazine |
NSFe–TiO2 |
UV light |
20.0 mg L−1 |
0.01 g L−1 |
90.0% (120 min) |
199 |
Sulfadiazine |
Bi2O3–TiO2/PAC |
Visible light |
20.0 mg L−1 |
0.2 g L−1 |
72.0% (30 min) |
200 |
Sulfadiazine |
TiO2/ZEO |
UV light |
10.0 mg L−1 |
1.0 g L−1 |
90.0% (120 min) |
201 |
Sulfadiazine |
Degussa P25 TiO2 |
Visible light |
10.0 mg L−1 |
1.0 g L−1 |
99.0% (60 min) |
202 |
Sulfadiazine |
C, N–TiO2@C |
Visible light |
20.0 mg L−1 |
1.0 g L−1 |
99.3% (140 min) |
203 |
Sulfadiazine |
BC_TiO2_MagEx |
Visible light |
5.0 mg L−1 |
1.0 g L−1 |
76.0% (240 min) |
204 |
Sulfadiazine |
ZIF-67/Ag NPs/NaYF4 : Yb,Er |
Simulated sunlight |
10 mg L−1 |
|
95.4% (180 min) |
205 |
5.3. Photocatalytic degradation of fluoroquinolones
Since the late 1980s, fluoroquinolones have been used as medications for humans and animals to prevent bacterial infections.206 Fluoroquinolones are found in the environment in significant amounts due to animal waste from farms, human waste from residential areas and hospitals, and fertiliser dispersal in agriculture. Generally, fluoroquinolones are prepared primarily by adding fluorine and piperazine groups to form the quinolones core structure207 in which ciprofloxacin, norfloxacin, levofloxacin/ofloxacin, enrofloxacin are the common used fluoroquinolones.208,209 Since their longer half-life (10.6 days in surface water and 580 days in sediments), more than 70% fluoroquinolones are discharged unmetabolized.210 Moreover, due to their chemical stability, these fluoroquinolones are hard to be degraded thoroughly in the environment, which have potential harm to the ecological environment.209
Recent studies have demonstrated the development of highly effective photocatalytic devices for fluoroquinolone degradation. Table 4 displays the outcomes. The fluoroquinolone contaminants are discovered to be efficiently destroyed in the presence of light by employing photocatalysts. The chemical structures of fluoroquinolones and the conditions under which photocatalytic processes occur can be responsible for significant modification in the degradation capacity of fluoroquinolones by various photocatalytic processes.77 Fig. 6, comprises the fluoroquinolone degradation pathways under various photocatalytic processes.
Table 4 Photocatalytic degradation of fluoroquinolones at different conditions
Target antibiotic |
Photocatalyst |
Source of light |
Optimum conditions |
Degradation (%) |
Ref. |
Initial concentration |
Catalyst concentration |
Ciprofloxacin |
Ag/SiO2 |
Sunlight |
10.0 mg L−1 |
0.12 g L−1 |
98.0% (180 min) |
211 |
Ciprofloxacin |
ZnO/CD |
Sunlight |
10.0 mg L−1 |
0.6 g L−1 |
98.0% (110 min) |
212 |
Ciprofloxacin |
NCuTiO2/CQD |
Visible light |
20.0 mg L−1 |
0.8 g L−1 |
89.0% (180 min) |
213 |
Ciprofloxacin |
ZnO/Co3O4 |
Visible light |
10.0 mg L−1 |
2.4 g L−1 |
100% (30 min) |
214 |
Ciprofloxacin |
TiO2/Ce |
UV light |
40.0 mg L−1 |
6.0 g L−1 |
93.0% (180 min) |
215 |
Ciprofloxacin |
TiO2/WO3 |
UV light |
20.0 mg L−1 |
0.5 g L−1 |
100% (120 min) |
216 |
Ciprofloxacin |
CuO |
Visible light |
10.0 mg L−1 |
5.0 g L−1 |
60.0% (300 min) |
217 |
Ciprofloxacin |
CeO2/Co3O4 |
Visible light |
5.0 mg L−1 |
0.5 g L−1 |
87.8% (50 min) |
218 |
Ciprofloxacin |
TiO2/N |
UV light |
30.0 mg L−1 |
1.0 g L−1 |
94.5% (120 min) |
219 |
Ciprofloxacin |
TiO2/La (0.1%) |
Visible light |
10.0 mg L−1 |
0.6 g L−1 |
99.5% (300 min) |
220 |
Ciprofloxacin |
TiO2/Sm (0.1%) |
Visible light |
10.0 mg L−1 |
0.9 g L−1 |
99.0% (300 min) |
221 |
Ciprofloxacin |
TiO2/Er (0.1%) |
Visible light |
10.0 mg L−1 |
0.9 g L−1 |
99.0% (300 min) |
221 |
Ciprofloxacin |
ZnO/Nd (0.1%) |
Visible light |
6.0 mg L−1 |
0.9 g L−1 |
99.0% (120 min) |
222 |
Ciprofloxacin |
Fe3O4/Bi2WO6 |
Visible light |
10.0 mg L−1 |
0.3 g L−1 |
99.7% (25 min) |
223 |
Ciprofloxacin |
MMT/CuFe2O4 |
UV light |
32.5 mg L−1 |
0.78 g L−1 |
80.0% (47.5 min) |
224 |
Ciprofloxacin |
Au-RGO/TiO2 |
Visible light |
10.0 mg L−1 |
|
96.93% (180 min) |
225 |
Ciprofloxacin |
CeO2/ZnO |
UV light |
10.0 mg L−1 |
0.25 g L−1 |
92.0% (360 min) |
226 |
Ciprofloxacin |
MgFe2O4/UiO-67 |
Visible light |
10.8 mg L−1 |
|
99.62% (90 min) |
227 |
Ciprofloxacin |
B2O3/N-rGO |
Visible light |
15.0 mg L−1 |
0.25 g L−1 |
98.0% (180 min) |
228 |
Ciprofloxacin |
rGO/Bi4O5Br2 |
Visible light |
10.0 mg L−1 |
0.5 g L−1 |
97.6% (60 min) |
229 |
Ciprofloxacin |
CdS@CuS/rGO |
Visible light |
10.0 mg L−1 |
0.25 g L−1 |
91.5% (60 min) |
230 |
Ciprofloxacin |
NiAl LDH/Fe3O4–rGO |
Visible light |
10.0 mg L−1 |
0.25 g L−1 |
91.36% (150 min) |
231 |
Ciprofloxacin |
Ag2MoO4 |
UV light |
20.0 mg L−1 |
0.5 g L−1 |
98.0% (40 min) |
232 |
Ciprofloxacin |
SiC/g-C3N4 |
Visible light |
10.0 mg L−1 |
0.4 g L−1 |
95.0% (30 min) |
233 |
Ciprofloxacin |
B0.8Ce0.2TiO2/EPS film |
Sunlight |
10.0 mg L−1 |
1.0 g L−1 |
89.17% (240 min) |
234 |
Ciprofloxacin |
rGO–ZrO2 |
Sunlight |
10.0 mg L−1 |
|
93.1% (240 min) |
235 |
Ciprofloxacin |
SnO2 |
UV light |
50.0 mg L−1 |
0.5 g L−1 |
99.7% (120 min) |
236 |
Ciprofloxacin |
BFO/biochar |
Solar light |
10.0 mg L−1 |
2.0 g L−1 |
70.4% (120 min) |
237 |
Ciprofloxacin |
g-C3N4/Fe2O3 |
UV light |
10.0 mg L−1 |
0.3 g L−1 |
100% (60 min) |
238 |
Ciprofloxacin |
Bi2O2CO3 |
Visible light |
10.0 mg L−1 |
1.0 g L−1 |
76.8% (60 min) |
239 |
Ciprofloxacin |
Bi2WO6/BiO2−x |
Visible light |
10.0 mg L−1 |
0.5 g L−1 |
91.8% (120 min) |
240 |
Ciprofloxacin |
GO@Fe3O4@TiO2 |
Visible light |
10.0 mg L−1 |
0.1 g L−1 |
91.5% (240 min) |
241 |
Ciprofloxacin |
MIL-68(In, Bi)–NH2@BiOBr |
Visible light |
5.0 mg L−1 |
0.35 g L−1 |
91.1% (90 min) |
242 |
Ciprofloxacin |
Sm2O3/In2S3 |
Visible light |
20.0 mg L−1 |
0.05 g L−1 |
99.4% (55 min) |
243 |
Ciprofloxacin |
ZnCrLDO/FA |
Visible light |
10.0 mg L−1 |
|
98.0% (120 min) |
244 |
Ciprofloxacin |
2D Bi2O2CO3 |
UV-vis light |
10.0 mg L−1 |
1.0 g L−1 |
76.8% (60 min) |
245 |
Ciprofloxacin |
In2O3/BiOBr |
Visible light |
10.0 mg L−1 |
|
93.5% (90 min) |
246 |
Ciprofloxacin |
BiOI/MOF/F-BC |
Simulated sunlight |
10.0 mg L−1 |
|
94.4% (180 min) |
247 |
Ciprofloxacin |
BiOCl/diatomite |
Simulated sunlight |
10.0 mg L−1 |
0.5 g L−1 |
94.0% (10 min) |
248 |
Ciprofloxacin |
Ti3C2–Bi/BiOCl |
Visible light |
20.0 mg L−1 |
1.0 g L−1 |
89.0% (100 min) |
249 |
Ciprofloxacin |
3D tripyramid TiO2 |
Simulated sunlight |
10.0 mg L−1 |
1.0 g L−1 |
90.0% (60 min) |
250 |
Ciprofloxacin |
ZnSnO3 |
Simulated sunlight |
10.0 mg L−1 |
0.5 g L−1 |
85.9% (100 min) |
251 |
Ciprofloxacin |
ZnO–SnO2–Zn2SnO4 |
Simulated sunlight |
10.0 mg L−1 |
0.5 g L−1 |
95.8% (80 min) |
252 |
Levofloxacin |
WO12/g-C3N4 |
Visible light |
10.0 mg L−1 |
|
90.8% (70 min) |
253 |
Levofloxacin |
Au@ZnONPs-MoS2-rGO |
Visible light |
10.0 mg L−1 |
1.0 g L−1 |
99.8% (120 min) |
254 |
Levofloxacin |
LaFeO3/CdS |
Visible light |
10.0 mg L−1 |
|
97.3% (100 min) |
255 |
Levofloxacin |
Fe-doped BiOCl |
Visible light |
15.0 mg L−1 |
0.5 g L−1 |
94.7% (60 min) |
256 |
Levofloxacin |
Mn-doped ZnIn2S4 |
Visible light |
10.0 mg L−1 |
|
100% (30 min) |
257 |
Levofloxacin |
g-C3N4/TiO2 |
Solar light and UV irradiation |
5.0 mg L−1 |
0.5 g L−1 |
100% (50 min) |
258 |
Levofloxacin |
WO3/TiO2 |
Solar and UV light |
5.0 mg L−1 |
0.5 g L−1 |
66.0% (50 min) |
258 |
Levofloxacin |
Sb2S3/In2S3/TiO2 |
Visible light |
10.0 mg L−1 |
|
86.7% (160 min) |
259 |
Levofloxacin |
Fe–ZnO/WO3 |
Visible light |
10.0 mg L−1 |
0.5 g L−1 |
96.0% (60 min) |
260 |
Levofloxacin |
Co3O4/Bi2MoO6@ g-C3N4 |
Visible light |
10.0 mg L−1 |
|
95.21% |
261 |
Levofloxacin |
Bi2O2CO3/Ti3C2Tx |
Visible light |
10.0 mg L−1 |
|
95.4% (80 min) |
262 |
Ofloxacin |
g-C3N4/NH2-MIL-88B(Fe) |
Visible light |
10.0 mg L−1 |
0.4 g L−1 |
96.5% (150 min) |
263 |
Ofloxacin |
TS-1/C3N4 |
Visible light |
10.0 mg L−1 |
1.55 g L−1 |
90.0% (70 min) |
264 |
Ofloxacin |
BiFeO3 |
Visible light |
10.0 mg L−1 |
0.5 g L−1 |
80.0% (180 min) |
265 |
Ofloxacin |
Mg–Ni co-doped TiO2 |
Visible light |
40.0 mg L−1 |
2.0 g L−1 |
96.0% (60 min) |
266 |
Ofloxacin |
PEB-DBT/α-Fe2O3 |
Visible light |
40.0 mg L−1 |
|
98.0% (50 min) |
267 |
Ofloxacin |
UiO-66/wood |
Simulated sunlight |
10.0 mg L−1 |
0.02 g L−1 |
80.96% (270 min) |
268 |
Ofloxacin |
ZnFe2O4/BiVO4 |
Visible light |
20.0 mg L−1 |
1.0 g L−1 |
97.0% (30 min) |
269 |
Ofloxacin |
Ag2O-g-C3N4 |
Visible light |
10.0 mg L−1 |
0.5 g L−1 |
99.1% (15 min) |
270 |
Norfloxacin |
AgI/BiOI |
Visible light |
20.0 mg L−1 |
1.0 g L−1 |
98.8% (120 min) |
271 |
Norfloxacin |
Fe3O4@La–BiFeO3 |
Visible light |
10.0 mg L−1 |
|
93.8% (60 min) |
272 |
Norfloxacin |
Y–TiO2/5A/NiFe2O4 |
Visible light |
30.0 mg L−1 |
2.0 g L−1 |
96.55% (60 min) |
273 |
Norfloxacin |
AgI/BiOI |
Visible light |
10.0 mg L−1 |
1.0 g L−1 |
98.8% (120 min) |
274 |
Norfloxacin |
Ni2O3@PC |
UV light |
10.0 mg L−1 |
0.1 g L−1 |
59.0% (180 min) |
275 |
Norfloxacin |
ZnO/g-C3N4 |
Visible light |
15.0 mg L−1 |
1.8 g L−1 |
92.8% (120 min) |
276 |
Norfloxacin |
RGO–SnSe |
Visible light |
40.0 mg L−1 |
1.0 g L−1 |
90.7% (70 min) |
277 |
Norfloxacin |
SnS2 |
Solar light |
20.0 mg L−1 |
0.05 g L−1 |
80.0% (110 min) |
278 |
Norfloxacin |
Cu2O@WO3 |
Visible light |
10.0 mg L−1 |
0.2 g L−1 |
90.0% (90 min) |
279 |
Norfloxacin |
Fe(III)–SrTiO3-GO |
Visible light |
10.0 mg L−1 |
|
92.3% (120 min) |
280 |
Norfloxacin |
GCNQDs/Ni5P4 |
UV light |
40.0 mg L−1 |
0.1 g L−1 |
92.0% (120 min) |
281 |
Norfloxacin |
BiOCl/ZnS–VZn+O |
Visible light |
20.0 mg L−1 |
0.5 g L−1 |
97.9% (50 min) |
282a |
Norfloxacin |
Au/MIL-101(Fe)/BiOBr |
Visible light |
10.0 mg L−1 |
0.1 g L−1 |
100% (20 min) |
282b |
Enrofloxacin |
Strontium-doped TiO2/CDs |
Visible light |
10.0 mg L−1 |
0.05 g L−1 |
84.7% (70 min) |
283 |
Enrofloxacin |
Ag–ZnFe2O4–rGO |
Visible light |
10.0 mg L−1 |
|
99.1% (60 min) |
284 |
Enrofloxacin |
CsxWO3/BiOI |
Visible light |
10.0 mg L−1 |
0.5 g L−1 |
100% (60 min) |
285 |
Enrofloxacin |
Zero-valent copper (nZVC) |
Visible light |
10.0 mg L−1 |
0.5 g L−1 |
99.51% (70 min) |
286 |
Enrofloxacin |
CdS/CuAg |
Visible light |
10.0 mg L−1 |
0.02 g L−1 |
99.9% (45 min) |
287 |
Enrofloxacin |
Fe3−xS4−y/g-C3N4 |
Visible light |
10.0 mg L−1 |
0.5 g L−1 |
100% (30 min) |
288 |
Enrofloxacin |
P/O co-doped g-C3N4/TiO2 |
Visible light |
10.0 mg L−1 |
1.0 g L−1 |
98.5% (60 min) |
289 |
Enrofloxacin |
Ball-milled biochar |
Visible light |
20.0 mg L−1 |
0.2 g L−1 |
80.2% (150 min) |
290a |
Enrofloxacin |
MIL-101(Fe)/BiOBr |
Visible light |
10.0 mg L−1 |
0.1 g L−1 |
84.4% (40 min) |
290b |
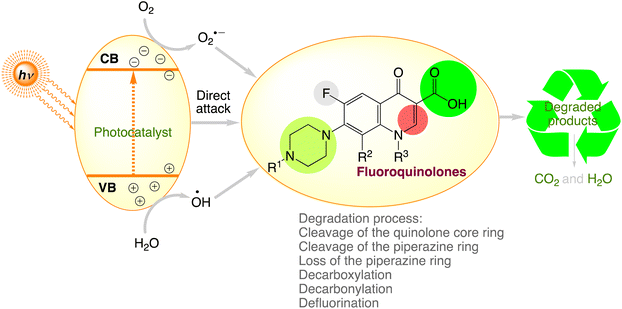 |
| Fig. 6 The proposed photocatalytic degradation pathways of fluoroquinolones. | |
5.4. Photocatalytic degradation of macrolides
Macrolides are monocyclic lactones with a high substitution rate having potency to prevent the synthesis of proteins.291 They belong to the class of large-ringed natural lactones, which typically have 12, 14, or 16 members. Examples of these lactones are tylosin, erythromycin, spiramycin, oleandomycin, clarithromycin, and azithromycin.292 Macrolides are not completely eradicated in sewage treatment plants, and it has been revealed that they do not readily hydrolyze in the environment, suggesting that they may continue to exist in the environment. Thus, it is important that we pay attention to the issue of macrolides causing environmental contamination.293 Tylosin is the most often utilised agent among macrolides, and one of the best technologies for their removal is photocatalytic oxidation.77,294 The photodegradation of macrolides by various photocatalysts can be briefly summarized in the Fig. 7. When a photon flows surpassing a semiconductor's band gap, an electron (e−) moves from the valence band (VB) to the conduction band (CB), generating a photogenerated hole on the VB. The chemical reaction will then occur when the separated charge carriers diffuse into the semiconductor/liquid interface's catalytically active regions (Fig. 7).
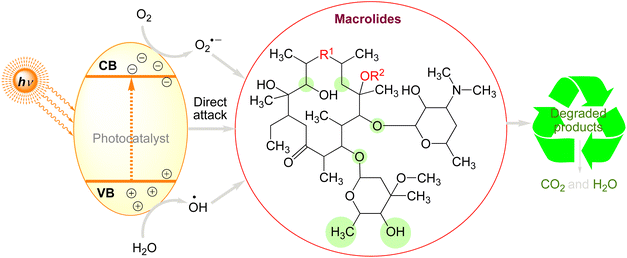 |
| Fig. 7 The proposed photocatalytic degradation pathways of macrolides. | |
Three types of radicals can be formed by holes: (1) directly oxidising macrolides into certain byproducts; (2) reacting with H2O to generate hydroxyl radicals (.OH) with high oxidation potential; and (3) reacting with O2 to form superoxide radicals (O2−.) with significant reducibility of electrons. In the end, these produced oxidation radicals can break down macrolides into hazardous or harmless byproducts, which can then be broken down further into CO2 and H2O by extending the reaction period. According to numerous research conducted recently, photocatalytic oxidation technologies are an excellent way to treat macrolides. Unfortunately, not much research has been done to fully understand how macrolides' complicated structure and enormous molecular weight affect their degradation processes. Table 5 summarises the photocatalytic degradation of macrolides under various circumstances.
Table 5 Photocatalytic degradation of macrolides at different conditions
Target antibiotic |
Photocatalyst |
Source of light |
Optimum conditions |
Degradation (%) |
Ref. |
Initial concentration |
Catalyst concentration |
Tylosin |
ZnCrNi/GO |
Visible light |
10.0 mg L−1 |
|
90.0% (80 min) |
295 |
Tylosin |
Au/TiO2-CCBs |
Visible light |
|
|
92.0% (180 min) |
296 |
Tylosin |
TiO2 |
UV light |
20 mg L−1 |
0.1 g L−1 |
80.0% (300 min) |
297 |
Tylosin |
g-C3N4 |
Simulated sunlight |
5 mg L−1 |
0.05 g L−1 |
99.0% (30 min) |
298 |
Tylosin |
Sm-doped gC3N4 |
Simulated sunlight |
25 mg L−1 |
0.5 g L−1 |
78.4% (90 min) |
299 |
Tylosin |
Er-doped g-C3N4 |
Simulated sunlight |
25 mg L−1 |
0.5 g L−1 |
70% (90 min) |
300 |
Tylosin |
Goethite-modified C3N4/ZnFe2O4 |
Simulated sunlight |
5 mg L−1 |
0.5 g L−1 |
99.0% (30 min) |
301 |
Erythromycin |
SnO2-doped TiO2 |
Visible light |
50 mg L−1 |
0.5 g L−1 |
67.0% (240 min) |
302 |
Erythromycin |
CaCO3 (nano-calcite) |
Sunlight |
30 mg L−1 |
0.5 g L−1 |
93.0% (360 min) |
303 |
Erythromycin |
Graphene-based TiO2 |
Simulated sunlight |
0.10 mg L−1 |
0.1 g L−1 |
84.0% (60 min) |
304 |
Erythromycin |
TiO2 |
UV light |
10 mg L−1 |
0.25 g L−1 |
90.0% (250 min) |
305 |
Erythromycin |
g-C3N4/CdS |
Simulated sunlight |
50 mg L−1 |
0.5 g L−1 |
81.02% (60 min) |
306 |
Erythromycin |
ZnIn2S4 |
Visible light |
10 mg L−1 |
0.05 g L−1 |
100% (180 min) |
307 |
Spiramycin |
TiO2 |
UV light |
25 mg L−1 |
0.25 g L−1 |
100% (180 min) |
308 |
Spiramycin |
TiO2 and ZnO |
UV/Visible light |
10 mg L−1 |
0.05 g L−1 |
100% (120 min) |
309 |
Spiramycin |
N-doped TiO2 |
Visible light |
40 mg L−1 |
3.0 g L−1 |
74.0% (240 min) |
310 |
Spiramycin |
g-C3N4/ZnFe2O4 |
Visible light |
20 mg L−1 |
1.0 g L−1 |
95.0% (240 min) |
311 |
Clarithromycin |
Graphene-based TiO2 |
Simulated sunlight |
0.10 mg L−1 |
0.1 g L−1 |
86.0 (60 min) |
312 |
Azithromycin |
ZrO2/Ag/TiO2 |
Visible light |
20 mg L−1 |
0.2 g L−1 |
90% (9 h) |
313 |
Azithromycin |
GO/Fe3O4/ZnO/SnO2 |
UV light |
30 mg L−1 |
1.0 g L−1 |
90.06% (120 min) |
314 |
Azithromycin |
Doped TiO2/fberglass-rubberized silicone |
UV light |
250 mg L−1 |
0.02 g L−1 |
70.0% (15 min) |
315 |
Azithromycin |
PAC/Fe/Ag/Zn |
UV light |
40 mg L−1 |
0.04 g L−1 |
99.5% (120 min) |
316 |
5.5. Photocatalytic degradation of β-lactams
β-Lactams as broad-spectrum antibiotics that are mainly classified as penicillin and cephalosporin. Amoxicillin (AMX) and ampicillin (AMP) are examples of penicillins that are generated from penicillium and have the ability to prevent amino acid chains in bacterial cell walls from cross-linking. The semisynthetic antibiotic class referred to as cephalosporins, which includes ceftiofur sodium (CFS), ceftriaxone sodium, cephalexin (CLX), and other similar antibiotics, is derived from 7-aminocephalosporanic acid (7-ACA).77,317,318
Investigations have shown that municipal wastewater treatment plants319 have greater quantities of penicillin and cephalosporin. β-Lactams, on the other hand, were not expected to survive in the environment because of their strong polarity, reduced adsorption capacity, and capacity to hydrolyze to the soil. Fig. 8 summarises the processes via which various, β-lactam antibiotics degrade. Table 6 summarises the results of the photocatalytic degradation of β-lactams using various photocatalysts.
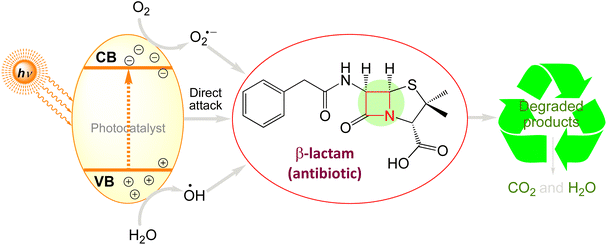 |
| Fig. 8 The proposed photocatalytic degradation pathways of β-lactams (antibiotics). | |
Table 6 Photocatalytic degradation of β-lactams (antibiotics) at different conditions
Target antibiotic |
Photocatalyst |
Source of light |
Optimum conditions |
Degradation (%) |
Ref. |
Initial concentration |
Catalyst concentration |
Amoxicillin |
Fe3O4@void@CuO/ZnO |
Visible light |
10.0 mg L−1 |
|
100% (70 min) |
320 |
Amoxicillin |
Iron nanoparticle (IPP) |
Visible light |
10.0 mg L−1 |
2.5 g L−1 |
60.0% (60 min) |
321 |
Amoxicillin |
TiO2–Cr |
Visible light |
10 mg L−1 |
0.33 g L−1 |
100% (90 min) |
322 |
Amoxicillin |
CuI/FePO4 |
Visible light |
10 mg L−1 |
|
90.0% (60 min) |
323 |
Amoxicillin |
GO/TiO2 |
UV light |
50 mg L−1 |
0.6 g L−1 |
99.84% (60 min) |
324 |
Amoxicillin |
CN-T |
Visible light |
50 mg L−1 |
0.3 g L−1 |
100% (48 h) |
325 |
Amoxicillin |
Magnetite/SCB biochar |
Visible light |
100 mg L−1 |
0.12 g L−1 |
73.51% (240 min) |
326 |
Amoxicillin |
TiO2@nZVI/PS |
Visible light |
20 mg L−1 |
1.0 g L−1 |
99.0% (60 min) |
327 |
Amoxicillin |
Ni doped ZnO |
UV-visible light |
10 mg L−1 |
|
86.21% (120 min) |
328 |
Amoxicillin |
ZnONPs |
UV light |
100 mg L−1 |
0.2 g L−1 |
90.0% (120 min) |
329 |
Amoxicillin |
TiO2/Fe2O3 |
Solar light |
50 mg L−1 |
1.0 g L−1 |
100% (180 min) |
330 |
Amoxicillin |
MIL-53(Al)/ZnO |
Visible light |
10 mg L−1 |
1.0 g L−1 |
100% (60 min) |
331 |
Amoxicillin |
Mn-doped Cu2O |
Sunlight |
15 mg L−1 |
1.0 g L−1 |
92.0% (180 min) |
332 |
Amoxicillin |
WO3 |
Simulated sunlight |
20 mg L−1 |
0.104 g L−1 |
99.99% (180 min) |
333 |
Amoxicillin |
TiO2 |
UV light |
10 mg L−1 |
0.25 g L−1 |
65.0% (150 min) |
334 |
Amoxicillin |
ZnO@TiO2 |
Visible light |
10 mg L−1 |
0.1 g L−1 |
80.0% (70 min) |
335 |
Amoxicillin |
Mesoporous g-C3N4 |
Visible light |
2 mg L−1 |
1.0 g L−1 |
99% (60 min) |
336 |
Amoxicillin |
Ag/TiO2/Mesoporous g-C3N4 |
Visible light |
5 mg L−1 |
1.0 g L−1 |
99% (60 min) |
337 |
Amoxicillin |
BiVO4 |
Visible light |
5 mg L−1 |
|
97.45% (90 min) |
338 |
Amoxicillin |
C-dots/Sn2Ta2O7/SnO2 |
Simulated sunlight |
20 mg L−1 |
|
88.3% (120 min) |
339 |
Ceftiofur sodium |
CdFe2O4/g-C3N4 |
Visible light |
30 mg L−1 |
|
68.6% (60 min) |
340 |
Ceftiofur sodium |
Ag–ZnO |
Visible light |
10 mg L−1 |
|
89.0% (6 h) |
341 |
Ceftiofur sodium |
Ag–TiO2 |
Visible light |
10 mg L−1 |
|
92.0% (90 min) |
342 |
Ceftriaxone sodium |
g-C3N4–ZnO |
UV light |
10 mg L−1 |
|
100% (60 min) |
343 |
Ceftriaxone sodium |
ZnO/ZnIn2S4 |
Visible light |
10 mg L−1 |
0.4 g L−1 |
83.5% (150 min) |
344 |
Ceftriaxone sodium |
CdS-g-C3N4 |
Visible light |
15 mg L−1 |
0.06 g L−1 |
92.55% (81 min) |
345 |
Ceftriaxone sodium |
CdSe QDs@MoS2 |
UV light |
20 mg L−1 |
0.012 g L−1 |
85.47% (180 min) |
346 |
Cephalexin |
ZnO |
Simulated sunlight |
20 mg L−1 |
0.1 g L−1 |
96.0% (25 min) |
347 |
Cephalexin |
Sodium persulfate (SPS) and fenton |
UV light |
10 mg L−1 |
0.1 g L−1 |
100% (60 min) |
348 |
Cephalexin |
g-C3N4/Zn doped Fe3O4 |
Visible light |
10 mg L−1 |
|
91.0% (5 h) |
349 |
Cephalexin |
CeO2@WO3 |
Visible light |
20 mg L−1 |
0.019 g L−1 |
98.8% (95 min) |
350 |
5.6. Photocatalytic degradation of nitroimidazoles
Nitroimidazoles are widely utilised in both human and veterinary medicine, mostly for the treatment of infectious illnesses. Nitroimidazoles are easily accumulated in hospitals, fish and poultry farms, animal husbandry, and the meat industry due to their high solubility, limited degradability, and carcinogenicity, all of which pose a major concern to human health and the ecosystem. As a result, creating effective strategies for the removal of nitroimidazoles77,351–354 is crucial. One popular method for treating nitroimidazoles is photocatalysis. The three most used nitroimidazoles are ornidazole, tinidazole, and metronidazole. The photocatalytic degradation and routes associated with metronidazole have been the subject of the greatest research among them. Table 7 provides an overview of the data from current investigations on the photocatalytic degradation of nitroimidazole.
Table 7 Photocatalytic degradation of nitroimidazoles at different conditions
Target antibiotic |
Photocatalyst |
Source of light |
Optimum conditions |
Degradation (%) |
Ref. |
Initial concentration |
Catalyst concentration |
Metronidazole |
Ag-doped- Ni0.5Zn0.5Fe2O4 (Ag-d-NZF) |
UV light |
50.0 mg L−1 |
0.01 g L−1 |
99.9% (360 min) |
355 |
Metronidazole |
Ag–N–SnO2 |
Visible light |
10.0 mg L−1 |
0.4 g L−1 |
97.03% (120 min) |
356 |
Metronidazole |
TiO2 decorated magnetic reduced graphene oxide |
Visible light |
20.0 mg L−1 |
0.75 g L−1 |
100% (120 min) |
|
Metronidazole |
Co–TiO2/sulphite |
Visible light |
20.0 mg L−1 |
0.8 g L−1 |
94.0% (18 min) |
357 |
Metronidazole |
ZEO/HDTMA-Br/CuS |
Simulated sunlight |
10.0 mg L−1 |
0.01 g L−1 |
100% (180 min) |
358 |
Metronidazole |
Co/g-C3N4/Fe3O4 |
Visible light |
5.0 mg L−1 |
0.7 g L−1 |
100% (60 min) |
359 |
Metronidazole |
UiO-66-NH2 |
Solar light |
5.0 mg L−1 |
0.125 g L−1 |
68.0% (360 min) |
360 |
Metronidazole |
PAC/Fe3O4 |
UV light |
30.0 mg L−1 |
0.6 g L−1 |
99.87% (90 min) |
361 |
Metronidazole |
ZnFe2O4@Uio-66 |
UV light |
90.0 mg L−1 |
0.05 g L−1 |
93.7% (120 min) |
362 |
Metronidazole |
ZnO/biochar |
Visible light |
10.0 mg L−1 |
|
97.1% (40 min) |
363 |
Metronidazole |
CN–PPy–MMt |
Visible light |
10.0 mg L−1 |
0.8 g L−1 |
99.3% (40 min) |
364 |
Metronidazole |
TiO2–Fe3O4 |
Visible light |
20.0 mg L−1 |
1.0 g L−1 |
96.0% (180 min) |
365 |
Metronidazole |
SBA-15/TiO2 |
UV light |
10.0 mg L−1 |
0.5 g L−1 |
87.7% (200 min) |
366 |
Metronidazole |
ZnO–ZnAl2O4 |
Sunlight |
20.0 mg L−1 |
0.4 g L−1 |
50.0% (120 min) |
367 |
Metronidazole |
CuS/NiS |
Visible light |
150.0 mg L−1 |
0.2 g L−1 |
23.31% (120 min) |
368 |
Metronidazole |
MoS2/Bi2S3 |
NIR light |
10 mg L−1 |
|
91.54% (40 min) |
369 |
Metronidazole |
HKUST-1-based SnO2 |
UV/Visible light |
40.0 mg L−1 |
2.0 g L−1 |
98.0% (240 min) |
370 |
Metronidazole |
Fe3O4@SiO2@TiO2/rGO |
UV light |
10.0 mg L−1 |
0.1 g L−1 |
94.0% (60 min) |
371 |
Metronidazole |
TiO2 |
UV light |
80.0 mg L−1 |
0.7 g L−1 |
100% (600 min) |
372 |
Metronidazole |
FeNi3/chitosan/BiOI |
Simulated sunlight |
20.0 mg L−1 |
0.04 g L−1 |
100% (200 min) |
373 |
Metronidazole |
Ag2S/BiVO4@α-Al2O3 |
Visible light |
30.0 mg L−1 |
1.0 g L−1 |
90.5% (120 min) |
374 |
Tinidazole |
rGO/BiOCl |
UV light |
18.0 mg L−1 |
0.001 g L−1 |
97.0% (5 min) |
375 |
Tinidazole |
Co/NCHPs |
UV/Visible light |
20.0 mg L−1 |
|
99.99% (6 min) |
376 |
Tinidazole |
Ag/HAp/In2S3 QDs |
Visible light |
20.0 mg L−1 |
0.24 g L−1 |
96.32% (30 min) |
377 |
Ornidazole |
TiO2 |
UV light |
50.0 mg L−1 |
1.0 g L−1 |
66.15% (180 min) |
378 |
Ornidazole |
Y3+-Bi5Nb3O15 |
Visible light |
20.0 mg L−1 |
2.0 g L−1 |
90.5% (180 min) |
379 |
Further observation from these investigations shows that the nitroimidazole degradation routes are comparable and may be summed up as denitration and the removal of their unique substituents. For instance, Fig. 9 illustrates the various stages of the metronidazole degradation process during the majority of the photocatalytic oxidation process. Three different reaction products were suggested for each of the two metronidazole degradation pathways. In pathway 1, metronidazole undergoes denitration and then loss of N-ethanol group, with the generation of products A, B, and E, respectively. In pathway 2, the N-ethanol group is first oxidized to carboxyl to produce C, which converts to D through loss of the N-acetic acid group. Besides, product D further transforms to E by denitration.
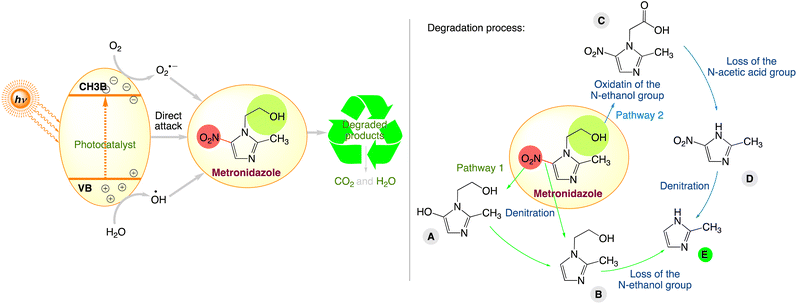 |
| Fig. 9 The proposed photocatalytic degradation pathways of metronidazole. | |
5.7. Photocatalytic degradation of other antibiotics
Apart from the previously stated antibiotic, some research continues to concentrate on the photocatalytic breakdown of antibiotics such as lincomycin, glycopeptides, aminoglycosides, and chloramphenicol. Table 8 provides an overview of the data regarding photocatalytic degradation of these antibiotics.
Table 8 Photocatalytic degradation of other antibiotics at different conditions
Target antibiotic |
Photocatalyst |
Source of light |
Optimum conditions |
Degradation (%) |
Ref. |
Initial concentration |
Catalyst concentration |
Chloramphenicol |
Fe/TaON/β-Si3N4/β-Si3Al3O3N5 |
Visible light |
20.0 mg L−1 |
0.01 g L−1 |
98.0% (30 min) |
380 |
Chloramphenicol |
SmVO4/g-C3N4 (SM/CN) |
Visible light |
10.0 mg L−1 |
0.5 g L−1 |
94.35% (105 min) |
381 |
Chloramphenicol |
BiOI/ZnO/rGO |
Visible light |
10.0 mg L−1 |
|
100% (180 min) |
382 |
Chloramphenicol |
CuInS2 |
Visible light |
10.0 mg L−1 |
0.2 g L−1 |
94.3% (120 min) |
383 |
Chloramphenicol |
Bi2S3/ZrO2 and Bi2WO6/ZrO2 |
Visible light |
10.0 mg L−1 |
0.2 g L−1 |
96.0% (15 min) |
384 |
Chloramphenicol |
PbS/TiO2 |
Sunlight |
10.0 mg L−1 |
0.06 g L−1 |
76.0% (240 min) |
385 |
Chloramphenicol |
rGO–ZnO |
UV light |
10.0 mg L−1 |
0.5 g L−1 |
90.0% (100 min) |
386 |
Gentamicin |
TiO2nps |
Visible light |
10.0 mg L−1 |
|
95.0% (80 min) |
387 |
Gentamicin |
ZnO |
UV light |
20.0 mg L−1 |
0.2 g L−1 |
93.0% (30 min) |
388 |
Lincomycin |
O-g/C3N4 |
Visible light |
100.0 mg L−1 |
|
99.0% (180 min) |
389 |
Lincomycin |
TNWs/TNAs |
Visible light |
500.0 mg L−1 |
|
85.0% (20 min) |
390 |
Vancomycin |
TNWs/TNAs |
Visible light |
500.0 mg L−1 |
|
100% (20 min) |
390 |
Vancomycin |
TiO2 |
UV light |
58.2 mg L−1 |
0.23 g L−1 |
93.0% (36.3 min) |
391 |
Vancomycin |
TiO2–clinoptilolite |
UV light |
30.0 mg L−1 |
0.2 g L−1 |
97.0% (50.9 min) |
392 |
6. Conclusions and perspective
The extensive discovery and application of antibiotics in recent decades has impacted human health and environmental systems to some extent. Antibiotic contamination has become a more significant scientific and practical issue overall. Since previous research has already acquired significant fundamental scientific and technical expertise, the photocatalytic technique represents an intriguing promise for attaining the elimination of antimicrobial contaminants. We are able to choose this technology for both indoor and outdoor water treatment systems owing to the freedom in selecting light sources. In addition, it is an industry-friendly technology because it is feasible to use sunlight. Photoscatalysis is a cost-effective method since it requires less space and maintenance than biodegradation. This review therefore provides an overview of the most recent advancements in the photocatalytic degradation of different antibiotics including tetracycline, sulfonamide, fluoroquinolones, macrolides, β-lactams, nitroimidazoles as well as miscellaneous antibiotics in aqueous solution under various reaction circumstances and critically examines recent methods for photocatalytic antibiotic degradation by involving the doping of metal and non-metal into ultraviolet light-driven photocatalysts, the generation of new semiconductor photocatalysts, the development of heterojunction photocatalysts, the building of surface plasmon resonance-enhanced photocatalytic systems that offers a basic understanding of the photocatalytic water treatment process. Utilising solar energy to reduce antimicrobial contaminants through photocatalytic technologies is promising from an industrialization and commercialization standpoint. A useful strategy for increasing photocatalytic activity, decreasing photogenerated carrier recombination, and improving charge separation and transfer efficiency at the photocatalyst interface is the development of heterojunctions. Building several heterojunctions with various semiconductors is therefore a typical tactic. As a result, due to their exceptional photocatalytic activity and acceptable redox ability, heterojunction photocatalysts have gained a lot of interest recently. The development of these photocatalysts on a wide scale and the formation of more efficient photocatalytic water purification systems will be greatly facilitated by future advancements.
Data availability
No primary research results, software or code have been included and no new data were generated or analysed as part of this review.
Conflicts of interest
There are no conflicts to declare.
References
- J. Niu, K. Wang, Z. Ma, F. Yang and Y. Zhang, ChemistrySelect, 2022, 5, 12353–12364 CrossRef.
- D. W. Graham, S. Olivares-Rieumont, C. W. Knapp, L. Lima, D. Werner and E. Bowen, Environ. Sci. Technol., 2011, 45, 418–424 CrossRef CAS PubMed.
- D. Li and W. Shi, Chin. J. Catal., 2016, 37, 792–799 CrossRef CAS.
- X. Bai, W. Chen, B. Wang, T. Sun, B. Wu and Y. Wang, Int. J. Mol. Sci., 2022, 23, 8130 CrossRef CAS PubMed.
- M. H. Abdurahman, A. Z. Abdullah and N. F. Shoparwe, Chem. Eng. J., 2021, 413, 127412 CrossRef CAS.
-
(a) J. O. Adeyemi, T. Ajiboye and D. C. Onwudiwe, Water, Air, Soil Pollut., 2021, 232, 219 CrossRef CAS;
(b) K. Kümmerer, Chemosphere, 2019, 75, 417–434 CrossRef PubMed.
-
(a) F. Zhao, D. Zhang, C. Y. Xu, J. S. Liu and C. S. Shen, Ecotoxicol. Environ. Saf., 2020, 196, 110552 CrossRef CAS PubMed;
(b) J. Jeong, W. Song, W. J. Cooper, J. Jung and J. Greaves, Chemosphere, 2010, 78(5), 533–540 CrossRef CAS PubMed;
(c) R. Daghrir and P. Drogui, Environ. Chem. Lett., 2013, 11(3), 209–227 CrossRef CAS.
-
(a) S. M. Marques, E. A. Enyedy, C. T. Supuran, N. I. Krupenko, S. A. Krupenko and M. A. Santos, Bioorg. Med. Chem., 2010, 18, 5081–5089 CrossRef CAS PubMed;
(b) W. Baran, E. Adamek, J. Ziemianska and A. Sobczak, J. Hazard. Mater., 2011, 196, 1–15 CrossRef CAS PubMed;
(c) Y. Zhang, J. Xu, Z. Zhong, C. Guo, L. Li, Y. He, W. Fan and Y. Chen, Environ. Sci. Pollut. Res. Int., 2013, 20(4), 2372–2380 CrossRef CAS PubMed.
-
(a) N. Casson and G. Greub, Int. J. Antimicrob. Agents, 2006, 27, 541–544 CrossRef CAS PubMed;
(b) M. Feng, Z. Wang, D. D. Dionysiou and V. K. Sharma, J. Hazard. Mater., 2018, 344, 1136–1154 CrossRef CAS PubMed;
(c) N. Janecko, L. Pokludova, J. Blahova, Z. Svobodova and I. Literak, Environ. Toxicol. Chem., 2016, 35(11), 2647–2656 CrossRef CAS PubMed.
-
(a) B. J. Ni, S. T. Zeng, W. Wei, X. H. Dai and J. Sun, Sci. Total Environ., 2020, 703, 134899 CrossRef CAS PubMed;
(b) C. S. McArdell, E. Molnar, M. J.-F. Suter and W. Giger, Environ. Sci. Technol., 2003, 37(24), 5479–5486 CrossRef CAS PubMed;
(c) S. Babic, L. Curkovic, D. Ljubas and M. Cizmic, Curr. Opin. Green Sustainable Chem., 2017, 6, 34–41 CrossRef.
-
(a) H. Cho, T. Uehara and T. G. Bernhardt, Cell, 2014, 159, 1300–1311 CrossRef CAS PubMed;
(b) I. Braschi, S. Blasioli, C. Fellet, R. Lorenzini, A. Garelli, M. Pori and D. Giacomini, Chemosphere, 2013, 93(1), 152–159 CrossRef CAS PubMed;
(c) S. Dancer, J. Antimicrob. Chemother., 2001, 48(4), 463–478 CrossRef CAS PubMed;
(d) P. Ray, K. F. Knowlton, C. Shang and K. Xia, PLoS One, 2014, 9(11), e112343 CrossRef PubMed;
(e) J. Dewdney, L. Maes, J. Raynaud, F. Blanc, J. Scheid, T. Jackson, S. Lens and C. Verschueren, Food Chem. Toxicol., 1991, 29(7), 477–483 CrossRef CAS PubMed;
(f) R. Gothwal and T. Shashidhar, Clean: Soil, Air, Water, 2015, 43(4), 479–489 CAS.
-
(a) P. W. Seo, N. A. Khan and S. H. Jhung, Chem. Eng. J., 2017, 315, 92–100 CrossRef CAS;
(b) A. Mantovani and A. Macri, J. Exp. Clin. Cancer Res., 2002, 21(4), 445–456 CAS.
-
(a) F. Santos-Beneit, M. Ordonez-Robles and J. F. Martin, Biochem. Pharmacol., 2017, 133, 74–85 CrossRef CAS PubMed;
(b) F. J. Angulo, O. E. Heuer, A. M. Hammerum, P. Collignon and H. C. Wegener, Clin. Infect. Dis., 2006, 43(7), 911–916 CrossRef PubMed.
-
(a) D. Sharma, M. Lata, M. Faheem, A. U. Khan, B. Joshi, K. Venkatesan, S. Shukla and D. Bisht, Microb. Pathog., 2019, 132, 150–155 CrossRef CAS PubMed;
(b) S. Ji, F. Zhang, X. Luo, B. Yang, G. Jin, J. Yan and X. Liang, J. Chromatogr. A, 2013, 1313, 113–118 CrossRef CAS PubMed.
-
(a) A. Chatzitakis, C. Berberidou, I. Paspaltsis, G. Kyriakou, T. Sklaviadis and I. Poulios, Water Res., 2008, 42(1–2), 386–394 CrossRef CAS PubMed;
(b) X. Liu, J. C. Steele and X.-Z. Meng, Environ. Pollut., 2017, 223, 161–169 CrossRef CAS PubMed.
-
(a) C.-H. Liu, Y.-H. Chuang, H. Li, B. J. Teppen, S. A. Boyd, J. M. Gonzalez, C. T. Johnston, J. Lehmann and W. Zhang, J. Environ. Qual., 2016, 45(2), 519–527 CrossRef CAS PubMed;
(b) L. Ge, Q. Liu, D. Jiang, L. Ding, Z. Wen, Y. Guo, C. Ding and K. Wang, Biosens. Bioelectron., 2019, 135, 145–152 CrossRef CAS PubMed.
- K. R. Davies, Y. Cherif, G. P. Pazhani, S. Anantharaj, H. Azzi, C. Terashima, A. Fujishima and S. Pitchaimuthu, J. Photochem. Photobiol., C, 2021, 48, 100437 CrossRef CAS.
-
(a) M. B. Ahmed, J. L. Zhou, H. H. Ngo, W. Guo, N. S. Thomaidis and J. Xu, J. Hazard. Mater., 2017, 323, 274–298 CrossRef CAS PubMed;
(b) S. Li, C. You, K. Rong, C. Zhuang, X. Chen and B. Zhang, Adv. Powder Mater., 2024, 3, 100183 CrossRef;
(c) M. Cai, Y. Liu, K. Dong, X. Chen and S. Li, Chin. J. Catal., 2023, 52, 239–251 CrossRef CAS;
(d) S. Li, M. Cai, Y. Liu, C. Wang, R. Yan and X. Chen, Adv. Powder Mater., 2023, 2, 100073 CrossRef;
(e) A. L. Valenzuela, M. Green and X. Chen, Gen. Chem., 2021, 7, 210006 Search PubMed;
(f) S. Li, M. Cai, Y. Liu, C. Wang, K. Lv and X. Chen, Chin. J. Catal., 2022, 43, 2652–2664 CrossRef CAS;
(g) X. Shen, B. Song, X. Shen, C. Shen, S. Shan, Q. Xueb, X. Chene and S. Li, Chem. Eng. J., 2022, 445, 136703 CrossRef CAS;
(h) S. Li, C. You, K. Rong, C. Zhuang, X. Chen and B. Zhang, Adv. Powder Mater., 2024, 3, 100183 CrossRef;
(i) S. Li, M. Cai, C. Wang and Y. Liu, Adv. Fiber Mater., 2023, 5, 994–1007 CrossRef CAS;
(j) C. Wang, K. Rong, Y. Liu, F. Yang and S. Li, Sci. China Mater., 2024, 67, 562–572 CrossRef CAS.
-
(a) P. P. Singh, S. Sinha, P. Nainwal, P. K. Singh and V. Srivastava, RSC Adv., 2024, 14, 2590–2601 RSC;
(b) P. P. Singh and V. Srivastava, ChemistrySelect, 2023, 8(44), e202302732 CrossRef CAS;
(c) V. Srivastava, S. Sinha, D. Kumar and P. P. Singh, Tetrahedron Green Chem., 2023, 1, 100009 CrossRef;
(d) P. P. Singh, J. Singh and V. Srivastava, RSC Adv., 2023, 13, 10958 RSC;
(e) P. P. Singh, P. K. Singh and V. Srivastava, Org. Chem. Front., 2023, 10, 216 RSC;
(f) P. P. Singh, S. Sinha, G. Pandey and V. Srivastava, RSC Adv., 2022, 12, 29826 RSC;
(g) V. Srivastava, P. K. Singh and P. P. Singh, J. Photochem. Photobiol., C, 2022, 50, 100488 CrossRef CAS;
(h) V. Srivastava, P. K. Singh, S. Tivari and P. P. Singh, Org. Chem. Front., 2022, 9, 1485 RSC;
(i) P. P. Singh, S. Sinha, P. Nainwal, S. Tivari and V. Srivastava, Org. Biomol. Chem., 2024, 22, 2523–2538 RSC.
-
(a) S. Tivari, M. Z. Beg, A. Kashyap, P. K. Singh, P. P. Singh, P. Gahtori and V. Srivastava, Results Chem., 2024, 7, 101249 CrossRef CAS;
(b) M. Z. Beg, S. Tivari, A. Kashyap, P. K. Singh, P. P. Singh, P. Nainwal and V. Srivastava, J. Heterocycl. Chem., 2024, 61, 458–465 CrossRef CAS;
(c) R. Kumar, K. Garima, V. Srivastava, P. P. Singh and P. K. Singh, Tetrahedron Lett., 2023, 133, 154841 CrossRef CAS;
(d) V. P. Singh, A. K. Singh, V. Srivastava and P. P. Singh, Tetrahedron, 2023, 147, 133658 CrossRef CAS;
(e) M. Mishra, P. P. Singh, P. Nainwal, S. Tivari and V. Srivastava, Tetrahedron Lett., 2023, 129, 154749 CrossRef CAS;
(f) V. Srivastava, S. Tivari, P. K. Singh and P. P. Singh, Catal. Lett., 2024, 154, 771–779 CrossRef CAS;
(g) M. Z. Beg, P. K. Singh, P. P. Singh, M. Srivastava and V. Srivastava, Mol. Divers., 2024, 28, 61–71 CrossRef CAS PubMed;
(h) S. P. Singh, V. Srivastava, P. K. Singh and P. P. Singh, Tetrahedron, 2023, 132, 133245 CrossRef CAS;
(i) M. Mishra, V. Srivastava, P. K. Singh and P. P. Singh, Croat. Chem. Acta, 2022, 95(1), 25–30 CrossRef CAS;
(j) S. Tivari, P. K. Singh, P. P. Singh and V. Srivastava, RSC Adv., 2022, 12, 35221 RSC.
- X. Yang, Z. Chen, W. Zhao, C. Liu, X. Qian, M. Zhang, G. Wei, E. Khan, Y. H. Ng and Y. S. Ok, Chem. Eng. J., 2021, 405, 126806 CrossRef CAS PubMed.
- B. Akyon, M. McLaughlin, F. Hernandez, J. Blotevogel and K. Bibby, Environ. Sci.: Processes Impacts, 2019, 21, 279–290 RSC.
- Z. Cetecioglu and M. Atasoy, Toxicity and Biodegradation Testing, 2018, pp. 29–55 Search PubMed.
-
(a) J. Zhang, H. Lin, J. Ma, W. Sun, Y. Yang and X. Zhang, Sci. Total Environ., 2019, 649, 396–404 CrossRef CAS PubMed;
(b) C. Zhuang, Y. Chang, W. Li, S. Li, P. Xu, H. Zhang, Y. Zhang, C. Zhang, J. Gao, G. Chen, T. Zhang, Z. Kang and X. Han, ACS Nano, 2024, 18, 5206–5217 CrossRef CAS PubMed.
- L. V. de Souza Santos, A. M. Meireles and L. C. Lange, J. Environ. Manage., 2015, 154, 8–12 CrossRef PubMed.
- M. S. Yahya, N. Oturan, K. El Kacemi, M. El Karbane, C. Aravindakumar and M. A. Oturan, Chemosphere, 2014, 117, 447–454 CrossRef CAS PubMed.
- V. Homem and L. Santos, J. Environ. Manage., 2011, 92, 2304–2347 CrossRef CAS PubMed.
- A. Boukhelkhal, O. Benkortbi and M. Hamadache, Environ. Technol., 2018, 40, 3328–3336 CrossRef PubMed.
- J. Luo, X. Li, C. Ge, K. Müller, H. Yu, P. Huang, J. Li, D. C. W. Tsang, N. S. Bolan, J. Rinklebe and H. Wang, Bioresour. Technol., 2018, 263, 385–392 CrossRef CAS PubMed.
- J. Wang, Q. Yao, C. Sheng, C. Jin and Q. Sun, J. Nanomater., 2017, 2017, 1–10 Search PubMed.
- Q. Zhao, S. Zhang, X. Zhang, L. Lei, W. Ma, C. Ma, L. Song, J. Chen, B. Pan and B. Xing, Environ. Sci. Technol., 2017, 51, 13659–13667 CrossRef CAS PubMed.
- I. A. Lawal and B. Moodley, J. Chem. Technol. Biotechnol., 2017, 92, 808–818 CrossRef CAS.
- C. H. Liu, Y. H. Chuang, H. Li, B. J. Teppen, S. A. Boyd, J. M. Gonzalez, C. T. Johnston, J. Lehmann and W. Zhang, J. Environ. Qual., 2016, 45, 519–527 CrossRef CAS PubMed.
- G. Li, Y. Feng, W. Zhu and X. Zhang, Korean J. Chem. Eng., 2015, 32, 2109–2115 CrossRef CAS.
- V. M. Nurchi, M. Crespo-Alonso, M. I. Pilo, N. Spano, G. Sanna and R. Toniolo, Arabian J. Chem., 2019, 12, 1141–1147 CrossRef CAS.
- W. C. Li and M. H. Wong, Int. J. Environ. Sci. Technol., 2014, 12, 2731–2740 CrossRef.
- A. Zhou, Y. Zhang, R. Li, X. Su and L. Zhang, Water Treat., 2016, 57, 388 CAS.
- S. Jia, Z. Yang, K. Ren, Z. Tian, C. Dong, R. Ma, G. Yu and W. Yang, J. Hazard. Mater., 2016, 317, 593–601 CrossRef CAS PubMed.
- O. A. Alsager, M. N. Alnajrani, H. A. Abuelizz and I. A. Aldaghmani, Ecotoxicol. Environ. Saf., 2018, 158, 114–122 CrossRef CAS PubMed.
- Y. Ji, Z. Pan, D. Yuan and B. Lai, Clean: Soil, Air, Water, 2018, 46, 1700666 Search PubMed.
- R. Kidak and S. Dogan, Ultrason. Sonochem., 2018, 40, 131–139 CrossRef CAS PubMed.
- M. Feng, L. Yan, X. Zhang, P. Sun, S. Yang, L. Wang and Z. Wang, Sci. Total Environ., 2016, 541, 167–175 CrossRef CAS PubMed.
- K. S. Tay and N. Madehi, Sci. Total Environ., 2015, 520, 23–31 CrossRef CAS PubMed.
- R. B. P. Marcelino, M. M. D. Leao, R. M. Lago and C. C. Amorim, J. Environ. Manage., 2017, 195, 110–116 CrossRef CAS PubMed.
- S. S. M. Hassan, H. I. Abdel-Shafy and M. S. M. Mansour, Arabian J. Chem., 2016, 12, 4074–4083 CrossRef.
- B. Kamarehie, F. Ahmadi, F. Hafezi, A. Abbariki, R. Heydari and M. A. Karami, Data Brief, 2018, 18, 96–101 CrossRef PubMed.
-
(a) P. Karaolia, I. Michael-Kordatou, E. Hapeshi, J. Alexander, T. Schwartz and D. FattaKassinos, Chem. Eng. J., 2017, 310, 491–502 CrossRef CAS;
(b) V. Sharma, R. Vinoth Kumar, K. Pakshirajan and G. Pugazhenthi, Powder Technol., 2017, 321, 259–269 CrossRef CAS;
(c) J. Zheng, C. Su, J. Zhou, L. Xu, Y. Qian and H. Chen, Chem. Eng. J., 2017, 317, 309–316 CrossRef CAS;
(d) M. R. Ramli, N. M. Sulaiman, M. A. Mohd and M. F. Rabuni, Water Sci. Technol., 2015, 72, 1611–1620 CrossRef CAS PubMed.
-
(a) S. Chang, X. Yang, Y. Sang and H. Liu, Chem. Asian J., 2016, 11, 2352–2371 CrossRef CAS PubMed;
(b) U. I. Gaya and A. H. Abdullah, J. Photochem. Photobiol., C, 2008, 9, 1–12 CrossRef CAS;
(c) J. C. Colmenares and R. Luque, Chem. Soc. Rev., 2014, 43, 765–778 RSC.
-
(a) E. S. Elmolla and M. Chaudhuri, Desalination, 2010, 252, 46–52 CrossRef CAS;
(b) A. C. Nogueira, L. E. Gomes, J. A. P. Ferencz, J. E. F. S. Rodrigues, R. V. Gonçalves and H. Wender, J. Phys. Chem. C, 2019, 123, 25680–25690 CrossRef CAS.
-
(a) W. Tu, Y. Zhou and Z. Zou, Adv. Funct. Mater., 2013, 23, 4996–5008 CrossRef CAS;
(b) H. L. Tan, F. F. Abdi and Y. H. Ng, Chem. Soc. Rev., 2019, 48, 1255–1271 RSC.
- A. A. Oladipo and F. S. Mustafa, Beilstein J. Nanotechnol., 2023, 14, 291–321 CrossRef CAS PubMed.
- X. Bai, W. Chen, B. Wang, T. Sun, B. Wu and Y. Wang, Int. J. Mol. Sci., 2022, 23, 8130 CrossRef CAS PubMed.
- Photocatalytic Degradation of Toxic Pesticides, ed. A. A. Oladipo, M. Gazi, A. O. Ifebajo, A. S. Oladipo and E. O. Ahaka, Wiley, Chicago, 2020, pp. 93–138, DOI:10.1002/9781119631422.ch4.
- Advanced Oxidation Processes for Waste Water Treatment, ed. S. C. Ameta and R. Ameta, Elsevier, Academic Press, 2018, DOI:10.1016/c2016-0-00384-4.
- Z. Wei, J. Liu and W. Shangguan, Chin. J. Catal., 2020, 41, 1440–1450 CrossRef CAS.
- T. Velempini, E. Prabakaran and K. Pillay, Mater. Today Chem., 2021, 19, 100380 CrossRef CAS.
- S. S. Imam, R. Adnan and N. H. M. Kaus, Toxicol. Environ. Chem., 2018, 100, 518–539 CrossRef.
- K. Qin, Q. Zhao, H. Yu, X. Xia, J. Li, S. He, L. Wei and T. An, Environ. Res., 2021, 199, 111360 CrossRef CAS PubMed.
- M. A. Gunawan, J.-C. Hierso, D. Poinsot, A. A. Fokin, N. A. Fokina, B. A. Tkachenko and P. R. Schreiner, New J. Chem., 2014, 38, 28–41 RSC.
- Y. Li, Y. Fu and M. Zhu, Appl. Catal., B, 2020, 260, 118149 CrossRef CAS.
- K. Soutsas, V. Karayannis, I. Poulios, A. Riga, K. Ntampegliotis, X. Spiliotis and G. Papapolymerou, Desalination, 2010, 250, 345–350 CrossRef CAS.
- X. Chen, J. Yao, B. Xia, J. Gan, N. Gao and Z. Zhang, J. Hazard. Mater., 2020, 383, 121220 CrossRef CAS PubMed.
- M. F. Pinto, M. Olivares, A. Vivancos, G. Guisado-Barrios, M. Albrecht and B. Royo, Catal. Sci. Technol., 2019, 9, 2421–2425 RSC.
- M. Muruganandham and M. Swaminathan, Sol. Energy Mater. Sol. Cells, 2004, 81, 439–457 CrossRef CAS.
- A. Taghizadeh, M. Taghizadeh, M. M. Sabzehmeidani, F. Sadeghfar and M. Ghaedi, Chapter 1—Electronic Structure: From Basic Principles to Photocatalysis, in Interface Science and Technology, ed. M. Ghaedi, Elsevier, Amsterdam, The Netherlands, 2021, vol. 32, pp. 1–53, ISBN 1573-4285 Search PubMed.
- F. Sadeghfar, Z. Zalipour, M. Taghizadeh, A. Taghizadeh and M. Ghaedi, Chapter 2—Photodegradation Processes, in Interface Science and Technology, ed. M. Ghaedi, Elsevier, Amsterdam, The Netherlands, 2021, vol. 32, pp. 55–124, ISBN 1573-4285 Search PubMed.
- O. Sacco, V. Vaiano, C. Han, D. Sannino and D. D. Dionysiou, Appl. Catal., B, 2015, 164, 462–474 CrossRef CAS.
- G. Zhao, J. Ding, F. Zhou, X. Chen, L. Wei, Q. Gao, K. Wang and Q. Zhao, Chem. Eng. J., 2021, 405, 126704 CrossRef CAS.
- E. Topkaya, M. Konyar, H. C. Yatmaz and K. Öztürk, J. Colloid Interface Sci., 2014, 430, 6–11 CrossRef CAS PubMed.
- M. Ni, M. K. H. Leung, D. Y. C. Leung and K. Sumathy, Renew. Sustain. Energy Rev., 2007, 11, 401–425 CrossRef CAS.
- V. Subhiksha, S. Kokilavani and S. S. Khan, Chemosphere, 2022, 290, 133228 CrossRef CAS PubMed.
- F. Zhu, Y. Lv, J. Li, J. Ding, X. Xia, L. Wei, J. Jiang, G. Zhang and Q. Zhao, Chemosphere, 2020, 252, 126577 CrossRef CAS PubMed.
- L. Wang, Z. Wang, L. Zhang and C. Hu, Chem. Eng. J., 2018, 352, 664–672 CrossRef CAS.
- M. Batool, M. F. Nazar, A. Awan, M. B. Tahir, A. Rahdar, A. E. Shalan, S. Lanceros-Méndez and M. N. Zafar, Nano-Struct. Nano-Objects, 2021, 27, 100762 CrossRef CAS.
-
(a) P. Khanna, A. Kaur and D. Goyal, J. Microbiol. Methods, 2019, 163, 105656 CrossRef CAS PubMed;
(b) N. Baig, I. Kammakakam and W. Falath, Adv. Mater., 2021, 2, 1821–1871 RSC.
- F. Ahmad, D. Zhu and J. Sun, Environ. Sci. Eur., 2021, 33, 64 CrossRef.
- Y. Chen, J. Yang, L. Zeng and M. Zhu, Crit. Rev. Environ. Sci. Technol., 2022, 52(8), 1401–1448 CrossRef CAS.
- S. Liu, X.-r. Zhao, H.-y. Sun, R.-p. Li, Y.-f. Fang and Y.-p. Huang, Chem. Eng. J., 2013, 231, 441–448 CrossRef CAS.
- F. Guo, W. Shi, H. Wang, M. Han, H. Li, H. Huang, Y. Liu and Z. Kang, Catal. Sci. Technol., 2017, 7, 3325–3331 RSC.
- D. Qiao, Z. Li, J. Duanb and X. He, Chem. Eng. J., 2020, 400, 125952 CrossRef CAS.
- G. Rathee, N. Singh and R. Chandra, ACS Omega, 2020, 5, 2368–2377 CrossRef CAS PubMed.
- Z. Liu, M. Zhu, L. Zhao, C. Deng, J. Ma, Z. Wang, H. Liu and H. Wang, Chem. Eng. J., 2017, 314, 59–68 CrossRef.
- J. Yang, Y. Lin, X. Yang, T. B. Ng, X. Ye and J. Lin, J. Hazard. Mater., 2017, 322, 525–531 CrossRef CAS PubMed.
- N. K. Eswar, S. A. Singh and G. Madras, Chem. Eng. J., 2018, 332, 757–774 CrossRef CAS.
- M. H. Khan, H. Bae and J. Y. Jung, J. Hazard. Mater., 2010, 181, 659–665 CrossRef CAS PubMed.
- Y. Ji, Y. Shi, W. Dong, X. Wen, M. Jiang and J. Lu, Chem. Eng. J., 2016, 298, 225–233 CrossRef CAS.
- K. Chakraborty, T. Pal and S. Ghosh, ACS Appl. Nano Mater., 2018, 1, 3137–3144 CrossRef CAS.
- Z. Xie, Y. Feng, F. Wang, D. Chen, Q. Zhang, Y. Zeng, W. Lv and G. Liu, Appl. Catal., B, 2018, 229, 96–104 CrossRef CAS.
- T. Xu, R. Zou, X. Lei, X. Qi, Q. Wu, W. Yao and Q. Xu, Appl. Catal., B, 2019, 245, 662–671 CrossRef CAS.
- Y. Hong, C. Li, B. Yin, D. Li, Z. Zhang, B. Mao, W. Fan, W. Gu and W. Shi, Chem. Eng. J., 2018, 338, 137–146 CrossRef CAS.
- D. Jiang, P. Xiao, L. Shao, D. Li and M. Chen, Ind. Eng. Chem. Res., 2017, 56(31), 8823–8832 CrossRef CAS.
- C. Zhou, C. Lai, P. Xu, G. Zeng, D. Huang, Z. Li, C. Zhang, M. Cheng, L. Hu, J. Wan, F. Chen, W. Xiong and R. Deng, ACS Sustain. Chem. Eng., 2018, 6(5), 6941–6949 CrossRef CAS.
- Q. Liu, J. Shen, X. Yang, T. Zhang and H. Tang, Appl. Catal., B, 2018, 232, 562–573 CrossRef CAS.
- M. Yan, Y. Hua, F. Zhu, W. Gu, J. Jiang, H. Shen and W. Shi, Appl. Catal., B, 2017, 202, 518–527 CrossRef CAS.
- W. Wang, J. Fang, S. Shao, M. Lai and C. Lu, Appl. Catal., B, 2017, 217, 57–64 CrossRef CAS.
- J. Lyu, J. Shao, Y. Wang, Y. Qiu, J. Li, T. Li, Y. Peng and F. Liu, Chem. Eng. J., 2019, 358, 614–620 CrossRef CAS.
- L. Ren, W. Zhou, B. Sun, H. Li, P. Qiao, Y. Xu, J. Wu, K. Lin and H. Fu, Appl. Catal., B, 2019, 240, 319–328 CrossRef CAS.
- R. Hailili, Z.-Q. Wang, M. Xu, Y. Wang, X.-Q. Gong, T. Xu and C. Wang, J. Mater. Chem. A, 2017, 5(40), 21275–21290 RSC.
- X. Yuan, D. Shen, Q. Zhang, H. Zou, Z. Liu and F. Peng, Chem. Eng. J., 2019, 369, 292–301 CrossRef CAS.
- F. Chen, Q. Yang, J. Sun, F. Yao, S. Wang, Y. Wang, X. Wang, X. Li, C. Niu, D. Wang and G. Zeng, ACS Appl. Mater. Interfaces, 2016, 8(48), 32887–32900 CrossRef CAS PubMed.
- T. Wang, W. Quan, D. Jiang, L. Chen, D. Li, S. Meng and M. Chen, Chem. Eng. J., 2016, 300, 280–290 CrossRef CAS.
- M. Yan, Y. Wu, F. Zhu, Y. Hua and W. Shi, Phys. Chem. Chem. Phys., 2016, 18(4), 3308–3315 RSC.
- C. Chen, H. Zeng, M. Yi, G. Xiao, S. Xu, S. Shen and B. Feng, Appl. Catal., B, 2019, 252, 47–54 CrossRef CAS.
- N. Nasseh, L. Taghavi, B. Barikbin and M. A. Nasseri, J. Clean. Prod., 2018, 179, 42–54 CrossRef CAS.
- D. Wang, F. Jia, H. Wang, F. Chen, Y. Fang, W. Dong, G. Zeng, X. Li, Q. Yang and X. Yuan, J. Colloid Interface Sci., 2018, 519, 273–284 CrossRef CAS PubMed.
- D. B. Hernandez-Uresti, A. Martınez-de la Cruz and L. M. Torres-Martınez, Ceram. Int., 2016, 42(2), 3096–3103 CrossRef CAS.
- W. Shi, H. Ren, M. Li, K. Shu, Y. Xu, C. Yan and Y. Tang, Chem. Eng. J., 2020, 382, 122876 CrossRef CAS.
- A. O. Oluwole and O. S. Olatunji, Environ. Sci. Eur., 2022, 34, 5 CrossRef CAS.
- S. Ghosh, K. Chakraborty, T. Pal and S. Ghosh, Sci. Rep., 2023, 13, 19028 CrossRef CAS PubMed.
- M. Sharma, M. K. Mandal, S. Pandey, R. Kumar and K. K. Dubey, ACS Omega, 2022, 7, 33572–33586 CrossRef CAS PubMed.
- B. Zhu, Q. Dong, J. Huang, M. Yang, X. Chen, C. Zhai, Q. Chen, B. Wang, H. Tao and L. Chen, ACS Omega, 2023, 8, 13702–13714 CrossRef CAS PubMed.
- Y. M. Hunge, A. A. Yadav, S.-W. Kang and H. Kim, J. Colloid Interface Sci., 2022, 606, 454–463 CrossRef CAS PubMed.
- S. Li, C. Wang, Y. Liu, M. Cai, Y. Wang, H. Zhang, Y. Guo, W. Zhao, Z. Wang and X. Chen, Chem. Eng. J., 2022, 429, 132519 CrossRef CAS.
- L. Biswal, B. P. Mishra, S. Das, L. Acharya, S. Nayak and K. Parida, Inorg. Chem., 2023, 62, 7584–7597 CrossRef CAS PubMed.
-
(a) T. B. Nguyen, P. N. T. Ho, C. W. Chen, C. P. Huang, R. Doong and C. D. Dong, Environ. Sci.: Nano, 2022, 9, 229 RSC;
(b) S. Li, C. Wang, Y. Liu, M. Cai, Y. Wang, H. Zhang, Y. Guoc, W. Zhaod, Z. Wange and X. Chen, Chem. Eng. J., 2022, 429, 132519 CrossRef CAS;
(c) S. Li, J. Chen, S. Hu, H. Wang, W. Jiang and X. Chen, Chem. Eng. J., 2020, 402, 126165 CrossRef CAS;
(d) S. Li, C. Wang, Y. Liu, B. Xue, W. Jiang, Y. Liu, L. Mo and X. Chen, Chem. Eng. J., 2021, 415, 128991 CrossRef CAS.
- Q. Chen, S. Wu and Y. Xin, Chem. Eng. J., 2016, 302, 377–387 CrossRef CAS.
- W. Zhao, J. Duan, B. Ji, L. Ma and Z. Yang, J. Environ. Chem. Eng., 2020, 8(1), 102206 CrossRef CAS.
- N. Shao, J. Wang, D. Wang and P. Corvini, Appl. Catal., B, 2017, 203, 964–978 CrossRef CAS.
- H. Chen, Y. P. Peng, K. F. Chen, C. H. Lai and Y. C. Lin, J. Environ. Sci., 2016, 44, 76–87 CrossRef CAS PubMed.
- Y. Yang, G. Zeng, D. Huang, C. Zhang, D. He, C. Zhou, W. Wang, W. Xiong, X. Li, B. Li, W. Dong and Y. Zhou, Appl. Catal., B, 2020, 272, 118970 CrossRef CAS.
- R. Hassandoost, S. R. Pouran, A. Khataee, Y. Orooji and S. W. Joo, J. Hazard. Mater., 2019, 376, 200–211 CrossRef CAS PubMed.
- B. Zhou, Y. Li, J. Bai, X. Li, F. Li and L. Liu, Appl. Surf. Sci., 2019, 464, 115–124 CrossRef CAS.
- X.-J. Wen, C.-G. Niu, L. Zhang and G.-M. Zeng, ACS Sustain. Chem. Eng., 2017, 5(6), 5134–5147 CrossRef CAS.
- Y. Gao, W. Yang, F. Wang, Y. Li, S. Cui, X. Liao and J. Yang, J. Taiwan Inst. Chem. Eng., 2023, 152, 105160 CrossRef CAS.
- S. Zhang, S. Zhao, S. Huang, B. Hu, M. Wang, Z. Zhang, L. He and M. Du, Chem. Eng. J., 2021, 420, 130516 CrossRef CAS.
- J. Shan, X. Wu, C. Li, J. Hu, Z. Zhang, H. Liu, P. Xia and X. Huang, Mater. Today Commun., 2022, 33, 104941 CrossRef CAS.
- J. Mehralipour, S. Darvishali, S. Bagheri and M. Kermani, Sci. Rep., 2023, 13, 11113 CrossRef CAS PubMed.
- H. Sun, T. Zhou, J. Kang, Y. Zhao, Y. Zhang, T. Wang and X. Yin, Sep. Purif. Technol., 2022, 299, 121771 CrossRef CAS.
- J. H. Kim, H. Y. Jang, S. B. Kim, J. W. Choi and J. A. Park, Water, Air, Soil Pollut., 2022, 233, 480 CrossRef CAS.
- K. Ouyang, C. Yang, B. Xu, H. Wang and S. Xie, Colloids Surf., A, 2021, 625, 126978 CrossRef CAS.
- J. Y. Chin, A. L. Ahmad and S. C. Low, J. Environ. Manage., 2023, 343, 118231 CrossRef CAS PubMed.
- Y. Li, S. Wang, H. Guo, J. Zhou, Y. Liu, T. Wang and X. Yin, Chemosphere, 2024, 352, 141371 CrossRef CAS PubMed.
- J. Gao, Y. Gao, Z. Sui, Z. Dong, S. Wang and D. Zou, J. Alloys Compd., 2018, 732, 43–51 CrossRef CAS.
- X.-J. Wen, C.-H. Shen, C.-G. Niu, D.-C. Lai, M.-S. Zhu, J. Sun, Y. Hu and Z.-H. Fei, J. Mol. Liq., 2019, 288, 111063 CrossRef CAS.
- W. Liu, Z. Li, Q. Kang and L. Wen, Environ. Res., 2021, 197, 110925 CrossRef CAS PubMed.
- F. Berdini, J. O. Otalvaro, M. Avena and M. Brigante, Results Eng., 2022, 16, 100765 CrossRef CAS.
- W. Liu, J. Zhang, J. Jia and C. Yuan, Powder Technol., 2024, 433, 119201 CrossRef CAS.
- K. Wang, X. Yu, F. Yang, Z. Liu, T. Zhang, J. Niu and B. Yao, Adv. Powder Technol., 2023, 34, 104157 CrossRef CAS.
- Y. Zhao, Y. Wang, E. Liu, J. Fan and X. Hu, Appl. Surf. Sci., 2018, 436, 854–864 CrossRef CAS.
- C.-c. Hao, F.-y. Chen, K. Bian, Y.-b. Tang and W.-l. Shi, Beilstein J. Nanotechnol., 2022, 13, 1038–1050 CrossRef CAS PubMed.
- W. Baran, E. Adamek, J. Ziemianska and A. Sobczak, J. Hazard. Mater., 2011, 196, 1–15 CrossRef CAS PubMed.
- Q. Dinh, E. Moreau-Guigon, P. Labadie, F. Alliot, M. J. Teil, M. Blanchard, J. Eurin and M. Chevreuil, Sci. Total Environ., 2017, 575, 758–766 CrossRef PubMed.
- R. Yin, W. Guo, N. Ren, L. Zeng and M. Zhu, Water Res., 2020, 171, 115374 CrossRef CAS PubMed.
- W. Yan, L. Yan and C. Jing, Appl. Catal., B, 2019, 244, 475–485 CrossRef CAS.
- T.-B. Nguyen, C. Huang, R.-a. Doong, C.-W. Chen and C.-D. Dong, Chem. Eng. J., 2020, 384, 123383 CrossRef CAS.
- M. Chen, C. Guo, S. Hou, L. Wu, J. Lv, C. Hu, Y. Zhang and J. Xu, J. Hazard. Mater., 2019, 366, 219–228 CrossRef CAS PubMed.
- M. Ren, Y. Ao, P. Wang and C. Wang, Chem. Eng. J., 2019, 378, 122122 CrossRef CAS.
- W. Zhu, F. Sun, R. Goei and Y. Zhou, Appl. Catal., B, 2017, 207, 93–102 CrossRef CAS.
- S. Naraginti, Y.-Y. Yu, Z. Fang and Y.-C. Yong, Chem. Eng. J., 2019, 375, 122035 CrossRef CAS.
- P. Karaolia, I. Michael-Kordatou, E. Hapeshi, C. Drosou, Y. Bertakis, D. Christofilos, G. S. Armatas, L. Sygellou, T. Schwartz, N. P. Xekoukoulotakis and D. Fatta-Kassinos, Appl. Catal., B, 2018, 224, 810–824 CrossRef CAS.
- H. Zhang, Z. Wang, R. Li, J. Guo, Y. Li, J. Zhu and X. Xie, Chemosphere, 2017, 185, 351–360 CrossRef CAS PubMed.
- E. Ioannidou, Z. Frontistis, M. Antonopoulou, D. Venieri, I. Konstantinou, D. I. Kondarides and D. Mantzavinos, Chem. Eng. J., 2017, 318, 143–152 CrossRef CAS.
- M. Jahdi, S. B. Mishra, E. N. Nxumalo, S. D. Mhlanga and A. K. Mishra, Appl. Catal., B, 2020, 267, 118716 CrossRef CAS.
- J. Yang, Z. Li and H. Zhu, Appl. Catal., B, 2017, 217, 603–614 CrossRef CAS.
- A. Mirzaei, L. Yerushalmi, Z. Chen, F. Haghighat and J. Guo, Water Res., 2018, 132, 241–251 CrossRef CAS PubMed.
- A. Yazdanbakhsh, A. Eslami, M. Massoudinejad and M. Avazpour, Chem. Eng. J., 2020, 380, 122497 CrossRef CAS.
- O. Mertah, A. Gómez-Avilés, A. Slassi, A. Kherbeche, C. Belver and J. Bedia, Catal. Commun., 2023, 157, 106611 CrossRef.
- X. Gan, Y. Song, G. Liu, H. Zhang and J. Yang, Front. Environ. Sci., 2023, 11, 1314536 CrossRef.
- J. Musial, D. T. Mlynarczyk and B. J. Stanisz, Sci. Total Environ., 2023, 856, 159122 CrossRef CAS PubMed.
- J. Dang, W. Pei, F. Hu, Z. Yu, S. Zhao, J. Hu, J. Liu, D. Zhang, Z. Jing and X. Lei, Toxics, 2023, 11(10), 818 CrossRef CAS PubMed.
- X. Lin, H. Fang, L. Wang, D. Sun, G. Zhao and J. Xu, Water, 2024, 16, 218 CrossRef CAS.
- J. Zhang, S. Gou, Z. Yang, C. Li and W. Wang, Water Cycle, 2024, 5, 1–8 CrossRef.
- D. Yongheng, Y. Huayu, L. Jiang, S. Qia, Y. Qianwena and Z. Yuntao, RSC Adv., 2023, 13, 5957–5969 RSC.
- J.-Y. Zhang, J. Ding, L.-M. Liu, R. Wu, L. Ding, J.-Q. Jiang, J.-W. Pang, Y. Li, N.-Q. Ren and S.-S. Yang, Environ. Sci. Ecotechnology, 2024, 17, 100308 CrossRef CAS PubMed.
- N. Le-Duy, L.-A. T. Hoang, T. D. Nguyen and T. Lee, Chemosphere, 2023, 321, 138118 CrossRef CAS PubMed.
- A. A. Ioannidi, J. Zappa, A. Petala, M. Souliotis, D. Mantzavinos and Z. Frontistis, Water, 2023, 15(7), 1370 CrossRef CAS.
- L. Pan, C. Jiao, Y. Liang, J. Xiong, S. Wang, H. Zhu, G. Chenc and H. Song, New J. Chem., 2021, 45, 5645–5653 RSC.
- A. Adewuyi, O. A. Ogunkunlec and R. A. Oderinde, RSC Adv., 2023, 13, 9563–9575 RSC.
- Y. Fang, H. Chen, T. Sheng, S. Lv, S. Cai, Y. Liu and K. Zhang, Surface. Interfac., 2024, 45, 103848 CrossRef CAS.
- H. Chen, Y. Fang, P. Duan, X. Zhang and K. Zhang, ChemistrySelect, 2023, 8, e202300658 CrossRef CAS.
- N. Le-Duy, L.-A. T. Hoang, T. D. Nguyen and T. Lee, ACS Appl. Nano Mater., 2023, 6(16), 14798–14809 CrossRef CAS.
- P. Wang, X. Gu, S. Li, J. Shen, J. Liu, C. Gong and Y. Chen, Environ. Technol., 2023, 1–16 Search PubMed.
- V. K. Parida, S. K. Srivastava, S. Chowdhury and A. K. Gupta, Langmuir, 2023, 39(51), 18846–18865 CrossRef CAS PubMed.
- X. Liu, J. Xu, T. Zhang, J. Zhang, D. Xia, Y. Du, Y. Jiang and K. Lin, J. Colloid Interface Sci., 2023, 629, 989–1002 CrossRef CAS PubMed.
- B. Zhang, C. Fang, J. Ning, R. Dai, Y. Liu, Q. Wu, F. Zhang, W. Zhang, S. Dou and X. Liu, Carbon Neutralization, 2023, 2, 646–660 CrossRef CAS.
- C.-S. Lu, H.-Y. Tsai, J. Shaya, V. B. Golovko, S.-Y. Wang, W.-J. Liug and C.-C. Chen, RSC Adv., 2022, 12, 29709–29718 RSC.
- N. Roy, K. Kannabiran and A. Mukherjee, Chemosphere, 2023, 333, 138912 CrossRef CAS PubMed.
- Y. Li, W. Zhu, Q. Guo, X. Wang, L. Zhang, X. Gao and Y. Luo, Sep. Purif. Technol., 2021, 276, 119403 CrossRef CAS.
- B. Yang, X. Mao, L. Pi, Y. Wu, H. Ding and W. Zhang, Environ. Sci. Pollut. Res. Int., 2017, 24(9), 8658–8670 CrossRef CAS PubMed.
- C. Zhou, P. Xu, C. Lai, C. Zhang, G. Zeng, D. Huang, M. Cheng, L. Hu, W. Xiong, X. Wen, L. Qin, J. Yuan and W. Wang, Chem. Eng. J., 2019, 359, 186–196 CrossRef CAS.
- C. Zhou, Z. Zeng, G. Zeng, D. Huang, R. Xiao, M. Cheng, C. Zhang, W. Xiong, C. Lai, Y. Yang, W. Wang, H. Yi and B. Li, J. Hazard. Mater., 2019, 380, 120815 CrossRef CAS PubMed.
- C. Zhou, C. Lai, D. Huang, G. Zeng, C. Zhang, M. Cheng, L. Hu, J. Wan, W. Xiong, M. Wen, X. Wen and L. Qin, Appl. Catal., B, 2018, 220, 202–210 CrossRef CAS.
- H. Ji, P. Du, D. Zhao, S. Li, F. Sun, E. C. Duin and W. Liu, Appl. Catal., B, 2020, 263, 118357 CrossRef CAS.
- T.-W. Tzeng, S.-L. Wang, C.-C. Chen, C.-C. Tan, Y.-T. Liu, T.-Y. Chen, Y.-M. Tzou, C. C. Chen and J. T. Hung, RSC Adv., 2016, 6(73), 69301–69310 RSC.
- X.-J. Wen, X.-X. Lv, J. Sun, J. Guo, Z.-H. Fei and C.-G. Niu, J. Hazard. Mater., 2020, 385, 121508 CrossRef CAS PubMed.
- S. Dong, X. Ding, T. Guo, X. Yue, X. Han and J. Sun, Chem. Eng. J., 2017, 316, 778–789 CrossRef CAS.
- S. Dong, L. Cui, C. Liu, F. Zhang, K. Li, L. Xia, X. Su, J. Feng, Y. Zhu and J. Sun, J. Taiwan Inst. Chem. Eng., 2019, 97, 288–296 CrossRef CAS.
- M.-A. Edaala, L. E. Mersly, A. A. Tahiri, P. Wong-Wah-Chung, L. E. Blidi, M. M. Alrashed and S. Rafqah, Water, 2023, 15(23), 4058 CrossRef CAS.
- X. Zeng, S. Shu, Y. Meng, H. Wang and Y. Wang, Chem. Eng. J., 2023, 456, 141105 CrossRef CAS.
- S. Shu, H. Wang, X. Guo, Y. Wang and X. Zeng, Sep. Purif. Technol., 2023, 323, 124458 CrossRef CAS.
- D. Liang, Z. Lin, Y. Wu, D. Li, J. Chen, X. Jin, Y. Chen, J. Zhang, H. Liu, P. Chen, W. Lv and G. Liu, Environ. Sci.: Nano, 2023, 10, 1053–1064 RSC.
- Y. M. Bakier and H. M. El-Bery, J. Environ. Chem. Eng., 2023, 11, 111493 CrossRef CAS.
- W. Zhu, J. Liu, S. Yu, Y. Zhou and X. Yan, J. Hazard. Mater., 2016, 318, 407–416 CrossRef CAS PubMed.
- R. Wang, J. Tang, X. Zhang, D. Wang, X. Wang, S. Xue, Z. Zhang and D. D. Dionysiou, J. Hazard. Mater., 2019, 375, 161–173 CrossRef CAS PubMed.
- Y. Wu, H. Ji, Q. Liu, Z. Sun, P. Li, P. Ding, M. Guo, X. Yi, W. Xu, C.-C. Wang, S. Gao, Q. Wang, W. Liu and S. Chen, J. Hazard. Mater., 2022, 424, 127563 CrossRef CAS PubMed.
- Q. Li, Z. Guan, D. Wu, X. Zhao, S. Bao, B. Tian and J. Zhang, ACS Sustain. Chem. Eng., 2017, 5(8), 6958–6968 CrossRef CAS.
- X. Xu, L. Meng, Y. Dai, M. Zhang, C. Sun, S. Yang, H. He, S. Wang and H. Li, J. Hazard. Mater., 2020, 381, 120953 CrossRef CAS PubMed.
- M. Liu, D. Zhang, J. Han, C. Liu, Y. Ding, Z. Wang and A. Wang, Chem. Eng. J., 2020, 382, 123017 CrossRef CAS.
- X. Xin, H. Liu, J. Sun, K. Gao and R. Jia, Int. J. Environ. Sci. Technol., 2023, 20, 11865–11876 CrossRef CAS PubMed.
- N. Wang, X. Li, Y. Yang, Z. Zhou, Y. Shang and X. Zhuang, J. Water Process Eng., 2020, 36, 101335 CrossRef.
- X. Liu, Y. Liu, S. Lu, W. Guo and B. Xi, Chem. Eng. J., 2018, 350, 131–147 CrossRef CAS.
- D. Li, N. Zhang, R. Yuan, H. Chen, F. Wang and B. Zhou, J. Environ. Chem. Eng., 2021, 9, 106243 CrossRef CAS.
- Q. Li, H. Y. Yang, P. Z. Zhong, N. J. Jiancong, W. Yang, J. Chen, Y. Zhang and L. Jianmin, Sep. Purif. Technol., 2022, 299, 121814 CrossRef CAS.
- C. P. Silva, D. Pereira, V. Calisto, M. A. Martins, M. Otero, V. Esteves and D. L. D. Lima, J. Environ. Manag., 2021, 294, 112937 CrossRef CAS PubMed.
- W. Yang, C. Bu, M. Zhao, Y. Li, S. Cui, J. Yang and H. Lian, Small, 2024, 2309972 CrossRef PubMed.
- M. Feng, Z. Wang, D. D. Dionysiou and V. K. Sharma, J. Hazard. Mater., 2018, 344, 1136–1154 CrossRef CAS PubMed.
- D. M. Whitacre, Reviews of Environmental Contamination and Toxicology, Springer, 2009, vol. 217 Search PubMed.
- N. Janecko, L. Pokludova, J. Blahova, Z. Svobodova and I. Literak, Environ. Toxicol. Chem., 2016, 35(11), 2647–2656 CrossRef CAS PubMed.
- X. Van Doorslaer, J. Dewulf, H. Van Langenhove and K. Demeestere, Sci. Total Environ., 2014, 500–501, 250–269 CrossRef CAS PubMed.
- M. Feng, Z. Wang, D. D. Dionysiou and V. K. Sharma, J. Hazard. Mater., 2018, 344, 1136–1154 CrossRef CAS PubMed.
- M. Golmohammadi, H. Hanafi-Bojd and M. Shiva, Ceram. Int., 2023, 49, 7717–7726 CrossRef CAS.
- I. Mukherjee, V. Cilamkoti and R. K. Dutta, ACS Appl. Nano Mater., 2021, 4(8), 7686–7697 CrossRef CAS.
- R. Noroozi, M. Gholami, V. Oskoei, M. H. Arani, S. A. Mousavifard, B. N. Le and M. Fattahi, Sci. Rep., 2023, 13, 16287 CrossRef CAS PubMed.
- H. Alshaikh, A. Shawky, R. M. Mohamed, J. K. Knight and L. S. Roselin, J. Mol. Liq., 2021, 334, 116092 CrossRef CAS.
- M. Manasa, P. R. Chandewar and H. Mahalingam, Catal. Today, 2021, 375(375), 522–536 CrossRef CAS.
- N. Moghni, H. Boutoumi, H. Khalaf, N. Makaoui and G. Colon, J. Photochem. Photobiol., A, 2022, 428, 113848 CrossRef CAS.
- C. Gherasim, P. Pascariu, M. Asandulesa, M. Dobromir, F. Doroftei, N. Fifere, A. Dascalu and A. Airinei, Ceram. Int., 2022, 48, 25556–25568 CrossRef CAS.
- C. H. Shen, X. J. Wen, Z. H. Fei, Z. T. Liu and Q. M. Mu, Chem. Eng. J., 2020, 391, 123612 CrossRef CAS.
- S. A. Abdulrahman, Z. Y. Shnain, S. S. Ibrahim and H. S. Majdi, Catalysts, 2022, 12, 1663 CrossRef CAS.
- P. Pascariu, C. Cojocaru, M. Homocianu, P. Samoila, A. Dascalu and M. Suchea, Ceram. Int., 2022, 48, 4953–4964 CrossRef CAS.
- P. Pascariu, C. Cojocaru, M. Homocianu and P. Samoila, J. Environ. Manag., 2022, 316, 115317 CrossRef CAS PubMed.
- P. Pascariu, C. Cojocaru, P. Samoila and C. Romanitan, Int. J. Mol. Sci., 2023, 24, 6436 CrossRef CAS PubMed.
- B. Zhu, D. Song, T. Jia, W. Sun, D. Wang, L. Wang, J. Guo, L. Jin, L. Zhang and H. Tao, ACS Omega, 2021, 6(2), 1647–1656 CrossRef CAS PubMed.
- T. J. Al-Musawi, N. Mengelizadeh, A. I. Alwared, D. Balarak and R. Sabaghi, Environ. Sci. Pollut. Res., 2023, 30, 70076–70093 CrossRef CAS PubMed.
- J. Zuo, X. Ma, C. Tan, Z. Xia, Y. Zhang, S. Yu, Y. Li, Yu. Li and J. Li, Anal. Methods, 2023, 15, 519–528 RSC.
- L. Wolski, K. Grzelak, M. Muńko, M. Frankowski, T. Grzyb and G. Nowaczyk, Appl. Surf. Sci., 2021, 563, 150338 CrossRef CAS.
- Z. Nasari and M. Taherimehr, Langmuir, 2023, 39(40), 14357–14373 CrossRef CAS PubMed.
- N. Kumar, R. Gusain, M. Masukume and S. S. Ray, Sol. RRL, 2023, 7, 2300475 CrossRef CAS.
- M. Xu, Y. Wang, E. Ha, H. Zhang and C. Li, Chemosphere, 2021, 265, 129013 CrossRef CAS PubMed.
- Y. Zhao, Y. Zuo, G. He, Q. Chen, Q. Meng and H. Chen, J. Alloys Compd., 2021, 869, 159305 CrossRef CAS.
- Z. Chen, J. Liang, X. Xu, G. He and H. Chen, J. Mater. Sci., 2020, 55, 6065 CrossRef CAS.
- J. V. Kumar, R. Karthik, S.-M. Chen, V. Muthuraj and C. Karuppiah, Sci. Rep., 2016, 6, 34149 CrossRef CAS PubMed.
- H. Zhu, B. Yang, J. Yang, Y. Yuan and J. Zhang, Chemosphere, 2021, 276, 130217 CrossRef CAS PubMed.
- M. Manjunatha and H. Mahalingam, Sci. Rep., 2013, 13, 14631 CrossRef PubMed.
- S. Kaushal, V. Kumari and P. P. Singh, Environ. Sci. Pollut. Res., 2023, 30, 65602–65617 CrossRef CAS PubMed.
- A. R. Dash, A. J. Lakhani, D. D. Priya, T. V. Surendra, M. M. R. Khan, E. J. J. Samuel and S. M. Roopan, J. Cluster Sci., 2023, 34, 121–133 CrossRef CAS.
- N. A. A. M. Azan, S. Sagadevan, A. R. Mohamed, A. H. N. Azazi, F. B. M. Suah, T. Kobayashi, R. Adnan and N. H. M. Kaus, Catalysts, 2020, 12(10), 1269 CrossRef.
- P. Rajiv, N. Mengelizadeh, G. McKay and D. Balarak, Int. J. Environ. Anal. Chem., 2023, 103, 2193–2207 CrossRef CAS.
- H. Qin, Y. Yang, W. Shia and Y. She, RSC Adv., 2021, 11, 13731–13738 RSC.
- H. Zhang, Z. Fan, Q. Chai and J. Li, Catalysts, 2023, 13(3), 469 CrossRef CAS.
- S. Uruş, M. Çaylar, H. Eskalen and Ş. Özgan, J. Mater. Sci.: Mater. Electron., 2022, 33, 4314–4329 CrossRef.
- Y. Peng, J. Lin, J.-L. Niu, X. Guo, Y. Chen, T. Hu, J. Cheng and Y. Hu, ACS Appl. Mater. Interfaces, 2024, 16(2), 2351–2364 CrossRef CAS PubMed.
- M. Murugalakshmi, K. Govindan, M. Umadevi, C. B. Breslin and V. Muthuraj, Environ. Sci.: Water Res. Technol., 2023, 9, 1385–1402 RSC.
- C. Chuaicham, T. Inoue, V. Balakumar, Q. Tian, B. Ohtani and K. Sasaki, J. Environ. Chem. Eng., 2022, 10, 106970 CrossRef CAS.
- H. Qin, Y. Yang, W. Shia and Y. She, RSC Adv., 2021, 11, 13731–13738 RSC.
- J. Cheng, Z. Deng, X. Zheng, C. Chu and Y. Guo, J. Alloys Compd., 2024, 971, 172779 CrossRef CAS.
- S. G. Khasevani, D. Nikjoo, C. Chaxel, K. Umeki, S. Sarmad, J.-P. Mikkola and I. Concina, ACS Omega, 2023, 8(46), 44044–44056 CrossRef CAS PubMed.
- Z. Jia, T. Li, Z. Zheng, J. Zhang, J. Liu, R. Li, Y. Wang, X. Zhang, Y. Wang and C. Fan, Chem. Eng. J., 2020, 380, 122422 CrossRef CAS.
- S. Wu, Y. Su, Y. Zhu, Y. Zhang and M. Zhu, Appl. Surf. Sci., 2020, 520, 146339 CrossRef CAS.
- Y. Li, Y. Fu and M. Zhu, Appl. Catal., B, 2020, 260, 118149 CrossRef CAS.
- S. Dong, L. Cui, W. Zhang, L. Xia, S. Zhou, C. K. Russell, M. Fan, J. Feng and J. Sun, Chem. Eng. J., 2020, 384, 123279 CrossRef CAS.
- S. Dong, L. Cui, Y. Tian, L. Xia, Y. Wu, J. Yu, D. M. Bagley, J. Sun and M. Fan, J. Hazard. Mater., 2020, 399, 123017 CrossRef CAS PubMed.
- S. L. Prabavathi, K. Saravanakumar, C. M. Park and V. Muthuraj, Sep. Purif. Technol., 2021, 257, 117985 CrossRef CAS.
- A. Machín, L. Soto-Vázquez, D. García, M. C. Cotto, D. Ortiz, P. J. Berríos-Rolón, K. Fontánez, E. Resto, C. Morant, F. Petrescu and F. Márquez, Catalysts, 2023, 13(3), 538 CrossRef.
- H. Qi, M. Wu, J. Wang, B. Zhang, C. Dai, F. Teng, M. Zhao and L. He, ChemistrySelect, 2023, 8, e202204121 CrossRef CAS.
- X. Zhong, K.-X. Zhang, D. Wu, X.-Y. Ye, W. Huang and B.-X. Zhou, Chem. Eng. J., 2020, 383, 123148 CrossRef CAS.
- L. Wen, P. Liu, Z. Zhou, K. Wang and Y. Zhou, Chem.–Asian J., 2023, 18, e202300161 CrossRef CAS PubMed.
- B. Trifi, A. Nahdi, A. Othmani, Z. Aloui, M. Essid and H. Dhaouadi, Int. J. Environ. Sci. Technol., 2024, 21, 3747–3760 CrossRef CAS.
- J. Li, Z. Yin, J. Guo, W. Gan, R. Chen, M. Zhang and Z. Sun, RSC Adv., 2024, 14, 4975–4989 RSC.
- C.-K. Tsai, Y.-C. Lee, T. T. Nguyen and J.-J. Horng, Chemosphere, 2022, 298, 134285 CrossRef CAS PubMed.
- H. Wei, F. Meng, W. Yu, J. Li and H. Zhang, Sep. Purif. Technol., 2023, 318, 123940 CrossRef CAS.
- L. Zhang, L. Tan, Z. Yuan, B. Xu, W. Chen, Y. Tang, L. Li and J. Wang, Chem. Eng. J., 2023, 452, 139327 CrossRef CAS.
- Q. Su, J. Li, H. Yuan, B. Wang, Y. Wang, Y. Li and Y. Xing, Chem. Eng. J., 2022, 427, 131594 CrossRef CAS.
- Q. Shang, X. Liu, M. Zhang, P. Zhang, Y. Ling, G. Cui, W. Liu, X. Shi, J. Yue and B. Tang, Chem. Eng. J., 2022, 443, 136354 CrossRef CAS.
- G. Gupta, S. K. Kansal, A. Umar and S. Akbar, Chemosphere, 2023, 314, 137611 CrossRef CAS PubMed.
- M. Malhotra, A. Garg and M. Rawat, Chem. Eng. Technol., 2024, 47, 683–691 CrossRef CAS.
- Z. Xiao, J. Xiao, L. Yuan, M. Ai, F. Idrees, Z.-F. Huang, C. Shi, X. Zhang, L. Pan and J.-J. Zou, J. Mater. Chem. A, 2024, 12, 5366–5376 RSC.
- L. Shi, X. Zou, T. Wang, D. Wang, M. Fan and Z. Gong, Chin. Chem. Lett., 2022, 33, 442–446 CrossRef CAS.
- B. Wang, K. Qian, W. Yang, W. An, L.-L. Lou, S. Liu and K. Yu, Front. Chem. Sci. Eng., 2023, 17, 1728–1740 CrossRef CAS.
- H. Yin, H. Shi, L. Sun, D. Xia and X. Yuan, Environ. Sci. Pollut. Res., 2021, 28, 11650–11664 CrossRef CAS PubMed.
- X. Liu, Y. Zhong, H. Feng, Y. Zhao, Q. Li and T. Huang, New J. Chem., 2024, 48, 800–810 RSC.
- Z. Shan, F. Ma, M. Xu, X. Shan, L. Shan, Ch. Cui and H. Guo, Chem. Eng. J., 2023, 466, 143229 CrossRef CAS.
- J. Xu, Z. Ren, X. Qiu, P. Zhu, D. Chen, L. Xie and C. Zhang, ChemistrySelect, 2021, 6, 7295–7305 CrossRef CAS.
- X. Liu, Y. Zhong, H. Feng, Y. Zhao, Q. Li and T. Huang, New J. Chem., 2024, 48, 800–810 RSC.
- A. A. Mashentseva, D. T. Nurpeisova and M. Barsbay, RSC Adv., 2024, 14, 4424–4435 RSC.
- Q. Chen, Y. H. Z. Song, M. Liu, D. Chen, B. Zhu, J. Chen and Z. Chen, Ecotoxicol. Environ. Saf., 2021, 225, 112742 CrossRef CAS PubMed.
- S. Kar, T. Pal and S. Ghosh, ACS Appl. Nano Mater., 2024, 7, 6516–6524 CrossRef CAS.
- S. Kar, T. Pal and S. Ghosh, ChemistrySelect, 2023, 8, e202300878 CrossRef CAS.
- H. Cai, Y. Ma, J. Li, Y. Jin, P. Zhu and M. Chen, Ind. Eng. Chem. Res., 2022, 61(30), 11237–11248 CrossRef CAS.
- M. Passi and B. Pal, Chem. Eng. J., 2024, 479, 147685 CrossRef CAS.
- C. Feng, Y. Wang, Z. Lu, Q. Liang, Y. Zhang, Z. Li and S. Xu, Sep. Purif. Technol., 2022, 282, 120107 CrossRef CAS.
-
(a) W. Zhang, Z. Huang, L. Zhang, Y. Meng, Z. Ni, H. Tang and S. Xia, J. Environ. Chem. Eng., 2023, 11, 109979 CrossRef CAS;
(b) S. Li, K. Dong, M. Cai, X. Li and X. Chen, eScience, 2024, 4, 100208 CrossRef.
- H. Guo, J. Cui, X. Chai, Y. Shi, S. Gao and J. Gao, Environ. Sci. Pollut. Res., 2023, 30, 68403–68416 CrossRef CAS PubMed.
- K. Wang, S. Zhan, D. Zhang, H. Sun, X. Jina and J. Wang, RSC Adv., 2021, 11, 4723–4739 RSC.
- T. Li, J. Liu, F. Shi, H. Zhang, H. Zhang, C. Ma and M. Wasim, J. Environ. Chem. Eng., 2023, 11, 109301 CrossRef CAS.
- Z. Gong, J. Xie, J. Liu, T. Liu, J. Chen, J. Li and J. Gan, Environ. Sci. Pollut. Res., 2023, 30, 38700–38712 CrossRef CAS PubMed.
- H. Rahmani, A. R. Mahjoub and Z. Khazaee, ACS Appl. Nano Mater., 2023, 6(6), 4554–4566 CrossRef CAS.
- P. Zhou, Y. Wang, L. Jing, X. Yan, Y. Gan, C. Xia, M. Xie and Y. Xu, Colloids Surf., A, 2024, 686, 133380 CrossRef CAS.
- J. Huang, D. Li, R. Li, P. Chen, Q. Zhang, H. Liu, W. Lv, G. Liu and Y. Feng, J. Hazard. Mater., 2020, 386, 121634 CrossRef CAS PubMed.
-
(a) Y. Xiao, H. Lyu, J. Tang, K. Wang and H. Sun, Chem. Eng. J., 2020, 384, 123311 CrossRef CAS;
(b) S. Li, C. Wang, K. Dong, P. Zhang, X. Chen and X. Li, Chin. J. Catal., 2023, 51, 101–112 CrossRef CAS.
- V. Homem and L. Santos, J. Environ. Manage., 2011, 92(10), 2304–2347 CrossRef CAS PubMed.
- C. S. McArdell, E. Molnar, M. J.-F. Suter and W. Giger, Environ. Sci. Technol., 2003, 37(24), 5479–5486 CrossRef CAS PubMed.
- S. M. Mitchell, J. L. Ullman, A. L. Teel and R. J. Watts, Chemosphere, 2015, 134, 504–511 CrossRef CAS PubMed.
- C.-H. Huang, J. E. Renew, K. L. Smeby, K. Pinkston and D. L. Sedlak, J. Contemp. Water Res. Educ., 2011, 120, 4 Search PubMed.
- C. Guo and P. Li, Environ. Monit. Assess., 2023, 195, 1423 CrossRef CAS PubMed.
- P. Zhao, Y. Yang, Y. Pei and X. Luo, Cellulose, 2023, 30, 1133–1147 CrossRef CAS.
- A. Ounnar, A. Bouzaza, L. Favier and F. Bentahar, Rev. Energ. Renouv., 2017, 20, 683–691 Search PubMed.
- H. Dong, X. Guo, C. Yang and Z. Ouyang, Appl. Catal., B, 2018, 230, 65–76 CrossRef CAS.
- G. Li, R. Wang, B. Wang and J. Zhang, Appl. Surf. Sci., 2020, 517, 146212 CrossRef CAS.
- G. Li, B. Wang, J. Zhang, R. Wang and H. Liu, Chem. Eng. J., 2019, 391, 123500 CrossRef.
- H. Dong, X. Guo and Y. Yin, Res. Chem. Intermed., 2018, 44(5), 3151–3167 CrossRef CAS.
- L. L. Albornoz, S. W. da Silva, J. P. Bortolozzi, E. D. Banús, P. Brussino, M. A. Ulla and A. M. Bernardes, Chemosphere, 2021, 268, 128858 CrossRef CAS PubMed.
- M. Mohsin, I. A. Bhatti, M. Iqbal, S. Naz, A. Ashar, J. Nisar, F. F. Al-Fawzan and S. A. Alissa, J. Water Proc. Eng., 2021, 44, 102433 CrossRef.
- P. Karaolia, I. Michael-Kordatou, E. Hapeshi, C. Drosou, Y. Bertakis, D. Christofilos, G. S. Armatas, L. Sygellou, T. Schwartz, N. P. Xekoukoulotakis and D. Fatta-Kassinos, Appl. Catal., B, 2018, 224, 810–824 CrossRef CAS.
- N. P. Xekoukoulotakis, N. Xinidis, M. Chroni, D. Mantzavinos, D. Venieri, E. Hapeshi and D. Fatta-Kassinos, Catal. Today, 2010, 151(1–2), 29–33 CrossRef CAS.
- G. Li, B. Wang, J. Zhang, R. Wang and H. Liu, Appl. Surf. Sci., 2019, 478, 1056–1064 CrossRef CAS.
- B. Gao, S. Dong, J. Liu, L. Liu, Q. Feng, N. Tan, T. Liu, L. Bo and L. Wang, Chem. Eng. J., 2016, 304, 826–840 CrossRef CAS.
- R. Hendili, A. Alatrache, M. Ben-Attia and M.-N. Pons, C. R. Chim., 2017, 20(7), 710–716 CrossRef CAS.
- D. Tassalit, N. Chekir, O. Benhabiles, F. Bentahar and N. A. Laoufi, Green Energy and Technology, Springer, Cham, 2016, pp. 695–706. DOI:10.1007/978-3-319-30127-3_51.
- V. Vaiano, O. Sacco, D. Sannino and P. Ciambelli, Chem. Eng. J., 2015, 261, 3–8 CrossRef CAS.
- L. Chen, W. Ma, J. Dai, J. Zhao, C. Li and Y. Yan, J. Photochem. Photobiol., A, 2016, 328, 24–32 CrossRef CAS.
- P. Karaolia, I. Michael-Kordatou, E. Hapeshi, C. Drosou, Y. Bertakis, D. Christofilos, G. S. Armatas, L. Sygellou, T. Schwartz, N. P. Xekoukoulotakis and D. Fatta-Kassinos, Appl. Catal., B, 2018, 224, 810–824 CrossRef CAS.
- S. Naraginti, Y.-Y. Yu, Z. Fang and Y.-C. Yong, Process Saf. Environ. Prot., 2019, 125, 39–49 CrossRef CAS.
- M. H. Sayadi, S. Sobhani and H. Shekari, J. Clean. Prod., 2019, 232, 127–136 CrossRef CAS.
- G.-I. Lupu, C. Orbeci, C. Bobirică, L. Bobirică, E. S. Lazăr, J. Pandele-Cusu, M. N. Verziu, C. Pîrvu and R.-G. Irodia, Sustainable Environ. Res., 2023, 33, 36 CrossRef CAS.
- A. Mehrdoost, R. J. Yengejeh, M. K. Mohammadi, A. Haghighatzadeh and A. A. Babaei, Environ. Sci. Pollut. Res., 2022, 29, 33514–33527 CrossRef CAS PubMed.
- B. Loder, G. Newton and E. Abraham, Biochem. J., 1961, 79, 408–416 CrossRef CAS PubMed.
- Y. Wang, R. L. Tennyson and D. Romo, Heterocycles, 2004, 64, 605–658 CrossRef CAS PubMed.
- V. Vaiano, O. Sacco, D. Sannino and P. Ciambelli, Chem. Eng. J., 2015, 261, 3–8 CrossRef CAS.
- S. Fallahizadeh, M. Gholami, M. R. Rahimi, A. Esrafili, M. Farzadkia and M. Kermani, Sci. Rep., 2023, 13, 16185 CrossRef CAS PubMed.
- R. M. B. Ramos, L. C. Paludo, P. I. Monteiro, L. V. M. da Rocha, C. V. de Moraes, O. O. Santos, E. R. Alves and T. L. P. Dantas, Talanta, 2023, 260, 124658 CrossRef PubMed.
- E. T. Wahyuni, R. N. Cahyono, M. Nora, E. Z. Alharissa and E. S. Kunarti, Results Chem., 2024, 7, 101302 CrossRef CAS.
- F. Beshkar, A. Al-Nayili, O. Amiri, M. Salavati-Niasari and M. Mousavi-Kamazani, J. Alloys Compd., 2022, 892, 162176 CrossRef CAS.
- D. Balarak, N. Mengelizadeh, P. Rajiv and K. Chandrika, Environ. Sci. Pollut. Res., 2011, 28, 49743–49754 CrossRef PubMed.
- I. F. Silva, I. F. Teixeira, R. D. F. Rios, G. M. do Nascimento, I. Binatti, H. F. V. Victória, K. Krambrock, L. A. Cury, A. P. C. Teixeira and H. O. Stumpf, J. Hazard. Mater., 2021, 401, 123713 CrossRef CAS PubMed.
- N. Zulfiqar, R. Nadeem and O. A. Musaimi, ACS Omega, 2024, 9(7), 7986–8004 CAS.
- Z.-H. Diao, J.-C. Jin, M.-Y. Zou, H. Liu, J.-Q. Qin, X.-H. Zhou, W. Qian, P.-R. Guo, L.-J. Kong and W. Chu, Sep. Purif. Technol., 2021, 278, 119620 CrossRef.
- G. Rana, P. Dhiman, J. Sharma, A. Kumar and G. Sharma, Inorg.
Chem. Commun., 2023, 158, 111596 CrossRef CAS.
- P. Debnath, K. Sen, A. Mondal, A. Mondal and N. K. Mondal, Int. J. Environ. Res., 2021, 15, 571–583 CrossRef CAS.
- F. M. d. Rosa, J. Papac, S. Garcia-Ballesteros, M. Kovačić, Z. Katančić, H. Kušić and A. L. Božić, Adv. Sustainable Syst., 2021, 5, 2100119 CrossRef.
- A. Fawzy, H. Mahanna and M. Mossad, Environ. Sci. Pollut. Res., 2022, 29, 68532–68546 CrossRef CAS PubMed.
- Y. T. Gaim, S. M. Yimanuh, Z. G. Kidanu and J. Compos, Science, 2022, 6(10), 317 CAS.
- T. T. Nguyen, S.-N. Nam, J. Son and J. Oh, Sci. Rep., 2019, 9, 9349 CrossRef PubMed.
- M. Verma and A. K. Haritash, Environ. Technol. Innovation, 2020, 20, 101072 CrossRef CAS.
- T. D. N. Thi, L. H. Nguyen, X. H. Nguyen, H. V. Phung, T. H. T. Vinh, P. V. Viet, N. V. Thai, H. N. Le, D. T. Pham, H. T. Van, L. H. T. Thi, T. D. P. Thi, T. L. Minh, H. H. P. Quang, H. P. N. Vu, T. T. Duc and H. M. Nguyen, Mater. Sci. Semicond. Process., 2022, 142, 106456 CrossRef.
- M. Dou, J. Wang, B. Gao, C. Xu and F. Yang, Chem. Eng. J., 2020, 383, 123134 CrossRef CAS.
- B. Gao, J. Wang, M. Dou, C. Xu and X. Huang, Environ. Sci. Pollut. Res., 2020, 27(7), 7025–7039 CrossRef CAS PubMed.
- M. Chahkandi and M. Zargazi, J. Hazard. Mater., 2020, 389, 121850 CrossRef CAS PubMed.
- S. Le, W. Yang, G. Chen, A. Yan and X. Wang, Environ. Pollut., 2020, 263, 114550 CrossRef CAS PubMed.
- M.-M. Fang, J.-X. Shao, X.-G. Huang, J.-Y. Wang and W. Chen, J. Mater. Sci. Technol., 2020, 56, 133–142 CrossRef CAS.
- N. Pugazhenthiran, P. Sathishkumar, O. Albormani, S. Murugesan, M. Kandasamy, M. Selvaraj, S. Suresh, S. K. Kumar, D. Contreras, H. Váldes and R. V. Mangalaraja, Chemosphere, 2023, 313, 137515 CrossRef CAS PubMed.
- N. Pugazhenthiran, S. Murugesan, H. Valdés, M. Selvaraj, P. Sathishkumar, P. G. Smirniotis, S. Anandan and R. V. Mangalaraja, J. Ind. Eng. Chem., 2022, 105, 384–392 CrossRef CAS.
- K. Portillo-Cortez, J. E. Romero-Ibarra, D. Dominguez, G. Alonso-Nuñez and U. Caudillo-Flores, J. Photochem. Photobiol., A, 2023, 445, 115090 CrossRef CAS.
- J. Yang, L. Fang, Z. Li, G. Meng, Y. Jia, Y. Jiang, J. Lian and X. Gan, Chemosphere, 2023, 314, 137618 CrossRef CAS PubMed.
- N. AttariKhasraghi, K. Zare, A. Mehrizad, N. Modirshahla and M. A. Behnajady, J. Inorg. Organomet. Polym., 2021, 31, 3164–3174 CrossRef CAS.
- M. Zhou, L. Cheng, Z. Chen, L. Chen and Y. Ma, J. Alloys Compd., 2021, 869, 159322 CrossRef CAS.
- J. He, Y. Zhang, Y. Guo, G. Rhodes, J. Yeom, H. Li and W. Zhang, Environ. Int., 2019, 132, 105105 CrossRef CAS PubMed.
- A. Almasi, R. Esmaeilpoor, H. Hoseini, V. Abtin and M. Mohammadi, J. Environ. Health Sci. Eng., 2020, 18, 1359–1373 CrossRef CAS PubMed.
- N. S. Nguyen, T. D. Pham, H. T. Vo and K. D. Ngo, Environ. Technol., 2019, 42(8), 1292–1301 CrossRef PubMed.
- S. Wang, J. Liu, I. Albaijan, A. Shawabkeh, H. Lin, I. Ahmad, S. O. Rab and M. Y. Alshahrani, J. Ind. Eng. Chem., 2024, 135, 213–231 CrossRef CAS.
- A. Bendesky, D. Menendez and P. Ostrosky-Wegman, Mutat. Res. Rev. Mutat. Res., 2022, 511(2), 133–144 CrossRef PubMed.
- S. Dong, J. Sun, Y. Li, C. Yu, Y. Li and J. Sun, Appl. Catal., B, 2014, 144, 386–393 CrossRef CAS.
- J. Li, W. Zhao, Y. Guo, Z. Wei, M. Han, H. He, S. Yang and C. Sun, Appl. Surf. Sci., 2015, 351, 270–279 CrossRef CAS.
- M. Malakootian, N. Olama, M. Malakootian and A. Nasiri, Int. J. Environ. Sci. Technol., 2019, 16(8), 4275–4284 CrossRef CAS.
- F. S. Mustafa and A. A. Oladipo, J. Water Proc. Eng., 2021, 42, 102132 CrossRef.
- M. S. H. Shuvo, R. A. Putul, K. S. Hossain, S. M. Masum and M. A. I. Molla, Toxics, 2024, 12(1), 36 CrossRef CAS PubMed.
- Y. Zhang and W. Chu, Sep. Purif. Technol., 2022, 291, 120900 CrossRef CAS.
- T. J. Al-Musawi, N. S. M. Moghaddam, S. M. Rahimi, M. Amarzadeh and N. Nasseh, J. Environ. Manage., 2022, 319, 115697 CrossRef CAS PubMed.
- M. Yeganeh, H. R. Sobhi and A. Esrafili, Environ. Sci. Pollut. Res., 2022, 29, 25486–25495 CrossRef CAS PubMed.
- Y.-L. Wang, A. Gómez-Avilés, S. Zhang, J. J. Rodriguez, J. Bedia and C. Belver, J. Environ. Chem. Eng., 2023, 11, 109744 CrossRef CAS.
- A. Seidmohammadi, Y. Vaziri, A. Dargahi and H. Z. Nasab, Biomass Conv. Bioref., 2023, 13, 9057–9073 CrossRef CAS.
- Z. M. Niaki, M. Ghorbani and S. A. Ghoreishi, J. Environ. Health Sci. Eng., 2021, 19, 1583–1596 CrossRef CAS PubMed.
- H. Cai, D. Zhang, X. Ma and Z. Ma, Sep. Purif. Technol., 2022, 288, 120633 CrossRef CAS.
- V. Balakumar, K. Sekar, C. Chuaicham, R. Manivannan and K. Sasaki, Environ. Sci.: Nano, 2021, 8, 2261–2276 RSC.
- A. Kubiak, Sci. Rep., 2023, 13, 12075 CrossRef PubMed.
- G. S. Sales, A. A. C. França, J. F. Cruz-Filho, C. A. F. Moraes, A. R. S. Neto, A. G. C. Sales, R. S. Santos and G. E. Luz Jr, J. Environ. Chem. Eng., 2023, 11, 110335 CrossRef CAS.
- F. Ghribi, M. Sehailia, L. Aoudjit, F. Touahra, D. Zioui, A. Boumechhour, D. Halliche, K. Bachari and Z. Benmaamar, J. Photochem. Photobiol.,
A, 2020, 397, 112510 CrossRef CAS.
- B. Farahani, M. Giahi, M. H. Ghorbani, R. Fazaeli and O. Moradi, J. Nanostruct. Chem., 2023, 13, 303–320 CrossRef CAS.
- H. Pasdar, N. E. Fard and M. Rezvani, Appl. Phys. A, 2023, 129, 380 CrossRef CAS.
- R. Sheikhsamany, H. Faghihian and R. Fazaeli, Mater. Sci. Semicond. Process., 2022, 138, 106310 CrossRef CAS.
- B. Kakavandi, E. Dehghanifard, P. Gholami, M. Noorisepehr and B. MirzaHedayat, Appl. Surf. Sci., 2021, 570, 151145 CrossRef CAS.
- S. Akter, M. S. Islam, M. H. Kabir, M. A. A. Shaikh and M. A. Gafur, Arabian J. Chem., 2022, 15, 103900 CrossRef CAS.
- F. S. Arghavan, T. J. Al-Musawi, G. A. Rumman, R. Pelalak, A. Khataee and N. Nasseh, J. Environ. Chem. Eng., 2021, 9, 105619 CrossRef CAS.
- S. Fakhravar, M. Farhadian and S. Tangestaninejad, J. Environ. Chem. Eng., 2020, 8, 104136 CrossRef CAS.
- S. Sohani, B. Ara, H. Khan, K. Gul and M. Khan, Environ. Res., 2022, 215, 114262 CrossRef CAS PubMed.
- Z. Li, S. Yuan, Z. Zhang, S. Liu, H. Guo, X. Qi, Z. Wu and J. Guo, Mater. Res. Bull., 2024, 175, 112755 CrossRef CAS.
- S. R. Mishra, V. Gadore and M. Ahmaruzzaman, J. Clean. Prod., 2023, 427, 139221 CrossRef CAS.
- W. Ahmad, A. Singh, K. K. Jaiswal and P. Gupta, J. Inorg. Organomet. Polym., 2021, 31, 614–623 CrossRef CAS.
- J. Zhao, B. Yao, Q. He and T. Zhang, J. Hazard. Mater., 2012, 229–230, 51–158 Search PubMed.
- L. N. Skvortsova, K. I. Kazantseva, K. A. Bolgaru, A. A. Reger, I. A. Artyukh and K. A. Dychko, Inorg. Mater., 2023, 59, 321–328 CrossRef CAS.
- K. Leeladevi, J. Vinoth Kumar, M. Arunpandian, M. Thiruppathi and E. R. Nagarajan, Mater. Sci. Semicond. Process., 2021, 123, 105563 CrossRef CAS.
- Z. Zhu, F. Guo, A. Li, W. Xu and X. Zhang, J. Environ. Sci., 2023, 134, 65–76 CrossRef CAS PubMed.
- C. Zhu, J. Li, Y. Chai, Y. Zhang, Y. Li, X. Zhang, J. Liu and Y. Li, Front. Chem., 2022, 10, 964008 CrossRef CAS PubMed.
- C.-W. Chien, N. Dhenadhayalan and K.-C. Lin, J. Environ. Chem. Eng., 2023, 11, 110613 CrossRef CAS.
- T. L. Palma, B. Vieira, J. Nunes, J. P. Lourenço, O. C. Monteiro and M. C. Costa, J. Iran. Chem. Soc., 2020, 17, 2013–2031 CrossRef CAS.
- K. O. Sodeinde, S. O. Olusanya, O. S. Lawal, M. Sriariyanun and A. A. Adediran, Sci. Rep., 2022, 12, 17054 CrossRef CAS PubMed.
- H. Singh, A. Ahlawat, T. K. Dhiman and P. R. Solanki, Mater. Lett., 2023, 346, 134504 CrossRef CAS.
- M. Baneshi, S. Jahanbin, A. Mousavizadeh, S. Sadat, A. Rayegan-Shirazi and H. Biglari, Pol. J. Environ. Stud., 2018, 27(4), 1433–1439 CrossRef CAS.
- Y. Deng, J. Liu, Y. Huang, M. Ma, K. Liu, X. Dou, Z. Wang, S. Qu and Z. Wang, Adv. Funct. Mater., 2020, 30, 2002353 CrossRef CAS.
- T. Do, D. Q. Nguyen, K. T. Nguyen and P. H. Le, Materials, 2019, 12(15), 2434 CrossRef CAS PubMed.
- F. Dehghani, S. Yousefinejad, M. Dehghani, S. M. Borghei and A. H. Javid, Int. J. Environ. Sci. Technol., 2022, 19, 8957–8968 CrossRef CAS.
- F. Dehghani, S. Yousefinejad, M. Dehghani, S. M. Borghei and A. H. Javid, Int. J. Environ. Anal. Chem., 2022, 1–9 CrossRef CAS.
|
This journal is © The Royal Society of Chemistry 2024 |
Click here to see how this site uses Cookies. View our privacy policy here.