DOI:
10.1039/C9CC08879B
(Communication)
Chem. Commun., 2020,
56, 1444-1447
Copper mediated C(sp2)–H amination and hydroxylation of phosphinamides†
Received
13th November 2019
, Accepted 20th December 2019
First published on 21st December 2019
Abstract
Copper mediated C(sp2)–H amination and hydroxylation of arylphosphinic acid are accomplished by adopting phosphinamide as the directing group. This method is distinguished by its wide substrate scope and excellent functional group tolerance, thus allowing for the rapid preparation of organophosphorus compounds in organic synthesis.
Organophosphorus compounds represent an important and fundamental class of molecules due to their extensive application in medicinal chemistry, organic materials and catalysis.1 Traditionally, their preparation or modification needs tedious manipulation and usually suffers from a limited substrate scope.2 In recent years, transition metal catalyzed C–H functionalization reactions have become a useful tool in organic synthesis.3 In this regard, a directing group (DG) is often required to locate the metal catalyst to proximate C–H bonds. To date, DGs based on the carbonyl group such as carboxyl acids, amides, and ketones have been frequently used in C–H functionalization reactions.4 However, examples employing P-containing functional groups as DGs are relatively rare, which extremely limits their utility in rapid preparation of important organophosphorus compounds.5 In 2013, Kim and co-worker introduced benzyl and phenoxyl phosphonic acids as DGs for Pd-catalyzed oxidative Heck reaction.6 Since then, Pd, Rh, Ru, and Ir-catalyzed C–H transformations such as oxidative annulation, arylation, and amination in phosphorus compounds have been reported in succession (eqn (1), Scheme 1).7 However, the high price and toxicity of these precious metals limit their further industrial applications. Compared with precious metals, the first-row transition metals such as Fe, Co, Ni, and Cu are earth abundant and low toxic.8 Recently, Daugulis and co-workers first employed arylphosphinic acid aminoquinoline amides as the substrates for cobalt catalyzed C–H annulation with alkynes and alkenes (eqn (2), Scheme 1).9 To the best of our knowledge, there are no examples of copper catalyzed C–H functionalization for arylphosphinic acid. Herein, we disclose a copper-mediated amination and hydroxylation of phosphinamide C(sp2)–H bonds (eqn (3), Scheme 1).
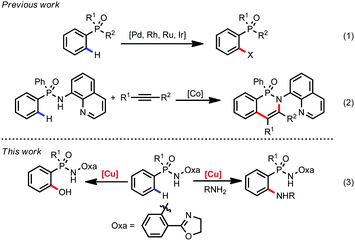 |
| Scheme 1 C–H functionalization of organophosphorus compounds. | |
Recently, we developed an efficient amide-tethered oxazoline bidentate auxiliary for copper-mediated C–H amination, trifluoromethylation, alkynylation, hydroxylation, arylation, and thiolation of arenes and heteroarenes.10 Inspired by these results, we were curious whether the oxazoline-containing bidentate auxiliary could facilitate the C(sp2)–H functionalization of arylphosphinic amide. Though arylphosphinic amide containing oxazoline has a similar coordination model to its benzoic amide analogues, the relative acidity of the N–H bond and subtle changes of the molecular structure blurred this idea. Considering the importance of the N,P-bidentate ligand in homogenous catalysis,11 we first chose Cu(OAc)2-mediated ortho C–H amidation as our target reaction. Gratifyingly, amidation occurred smoothly on diphenylphosphinic amide 1a with exclusive ortho-selectivity (Table 1, entry 1). Subsequently, we screened a variety of bases including K2CO3, Li2CO3, Cs2CO3, NaOAc, and KOAc, and found that Na2CO3 was the optimal choice (Table 1, entries 2–6). The yields dramatically decreased when DMSO was replaced with other polar solvents, such as DMF, DMA, NMP and MeCN (Table 1, entries 7–10). The yield could be improved to 84% by increasing the reaction temperature to 100 °C (Table 1, entries 11–14). Finally, a small improvement was observed by enhancing the loading of Na2CO3 to 2.5 equivalents, affording the amidated product in 88% yield (Table 1, entry 15). When we decreased the loading of Cu(OAc)2 to 20 mol%, only 12% yield of product 3a was obtained. The yield decreased to 30% when the reaction was carried out under a N2 atmosphere, indicating that air was crucial for the reaction.
Table 1 Optimization of amination reaction conditionsa
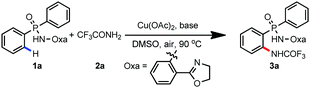
|
Entry |
Base |
Solvent |
Temp. (°C) |
Yieldb (%) |
Reaction conditions: 1a (0.1 mmol), 2a (0.2 mmol), Cu(OAc)2 (0.1 mmol), base (0.2 mmol), solvent (1.0 mL), temp., air, 6 h.
The yield was determined by 1H NMR analysis of a crude reaction mixture using CH2Br2 as an internal standard.
Na2CO3 (0.25 mmol).
Isolated yield.
20 mol% Cu(OAc)2.
N2 atmosphere.
|
1 |
Na2CO3 |
DMSO |
90 |
69 |
2 |
K2CO3 |
DMSO |
90 |
66 |
3 |
Li2CO3 |
DMSO |
90 |
21 |
4 |
Cs2CO3 |
DMSO |
90 |
25 |
5 |
NaOAc |
DMSO |
90 |
41 |
6 |
KOAc |
DMSO |
90 |
54 |
7 |
Na2CO3 |
DMF |
90 |
15 |
8 |
Na2CO3 |
DMA |
90 |
10 |
9 |
Na2CO3 |
NMP |
90 |
20 |
10 |
Na2CO3 |
MeCN |
90 |
n.r. |
11 |
Na2CO3 |
DMSO |
60 |
43 |
12 |
Na2CO3 |
DMSO |
80 |
66 |
13 |
Na2CO3 |
DMSO |
100 |
84 |
14 |
Na2CO3 |
DMSO |
110 |
82 |
15
|
Na
2
CO
3
|
DMSO
|
100
|
88 (76)
|
16c |
Na2CO3 |
DMSO |
100 |
12e, 30f |
Having identified the optimal conditions for C–H amidation, we next explored the substrate scope (Table 2). To our delight, a variety of phosphinic amides with electron-donating methyl-, methoxy-, and tert-butyl substituents could be amidated smoothly, giving the corresponding products in moderate to good yields (3a–3g, 44–76%). To our surprise, only 21% yield of the amidation product was obtained when 8-aminoquinoline was used as the directing group (ESI†). Moreover, electron-deficient phosphinamide 1h was also compatible with the reaction, providing the amidation product 3h in 94% yield. For benzodioxole derived phosphinamide 1i, two regioisomers were formed, with the less sterically hindered C6-position-amidated product as the major one (3i, 74%).
Table 2 The scope of phosphinamides for C–H aminationab
Reaction conditions: 1a–1i (0.1 mmol), 2a (0.2 mmol), Cu(OAc)2 (0.1 mmol), Na2CO3 (0.25 mmol), DMSO (1 mL), 100 °C, air, 6 h.
Isolated yield.
|
|
In addition to trifluoroacetamide, a wide range of sulfonamides and (hetero)anilines were also compatible with this transformation (Table 3). For example, amidation proceeded smoothly with sulfonamides bearing both electron-donating and electron-withdrawing groups (4a–4f, 60–73%). To our surprise, electron-deficient anilines and heteroanilines could also serve as amine donors, providing a useful method for the preparation of organophosphorus compounds which have potential applications in homogenous catalysis and medicinal chemistry (4g–4k, 33–62%).11,12
Table 3 Scope of amine coupling partnersab
Reaction conditions: 1a (0.1 mmol), 2b–2l (0.2 mmol), Cu(OAc)2 (0.1 mmol), Na2CO3 (0.25 mmol), DMSO (1 mL), 100 °C, air, 6 h.
Isolated yield.
|
|
With the success of achieving C–H amidation and amination of phosphinamides, we wondered whether this protocol could be compatible with C–H hydroxylation reaction as well.13 To our delight, we could introduce a free hydroxyl group into a variety of phosphinamides by adopting our previous reaction conditions.10f As shown in Table 4, regardless of the electronic properties of the substituents, substrates bearing both electron-donating and electron-withdrawing groups were well tolerated, giving the desired hydroxylated products in moderate to good yields (5a–5g, 39–70%). In accordance with previous work, the yield of 5a decreased to 22% when the reaction was carried out under a N2 atmosphere.10f
Table 4 Scope of phosphinamides for C–H hydroxylationa,b
Reaction conditions: 1 (0.1 mmol), Cu(OAc)2 (0.1 mmol), Na2CO3 (0.1 mmol), DMSO (1 mL), 80 °C, O2, 6 h.
Isolated yield.
N2 atmosphere.
|
|
Finally, the removal of this amide-oxazoline directing group was demonstrated by treating product 4a with 2 N KOH/EtOH at 80 °C, releasing the corresponding amino-phosphinic acid 6 in 75% yield with 93% recovery of the directing group (Scheme 2).
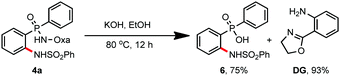 |
| Scheme 2 Removal of the directing group. | |
In conclusion, we developed a Cu-promoted C–H amination and hydroxylation for phosphinamide compounds. Unlike previously reported copper-catalyzed or copper-mediated C–H functionalization, in this study we developed phosphinamide as a new directing group. The technique showed a broad scope and excellent functional group tolerance, providing a new strategy for the preparation of various amino- and hydroxyl-contained arylphosphinic compounds in organic synthesis.
We gratefully acknowledge the Shanghai Institute of Materia Medica, Chinese Academy of Sciences, NSFC (21772211), the Youth Innovation Promotion Association CAS (No. 2014229 and 2018293), the Institutes for Drug Discovery and Development, Chinese Academy of Sciences (No. CASIMM0120163006), the Science and Technology Commission of Shanghai Municipality (17JC1405000), the Program of Shanghai Academic Research Leader (19XD1424600), the National Science & Technology Major Project “Key New Drug Creation and Manufacturing Program”, China (2018ZX09711002-006), and the State Key Laboratory of Natural and Biomimetic Drugs for financial support.
Conflicts of interest
The authors declare no conflict of interest.
Notes and references
-
(a)
C. Dousson, D. Surleraux, J. Paparin, C. Pierra, J.-F. Griffon, F. Leroy and D. Dukhan, WO2010101967 A2, 2010;
(b) D. S. Surry and S. L. Buchwald, Angew. Chem., Int. Ed., 2008, 47, 6338–6361 CrossRef CAS PubMed;
(c) N. Deligonul, B. J. Laughlin, R. C. Smith, L. J. Higham and J. D. Protasiewicz, Dalton Trans., 2012, 41, 12016–12022 RSC;
(d)
Phosphorus(III) Ligands in Homogeneous Catalysis: Design and Synthesis, ed. P. C. J. Kamer and P. W. N. M. van Leeuwen, Wiley-VCH, New York, 2012 Search PubMed;
(e)
Phosphorus: Chemistry, Biochemistry and Technology, ed. D. E. C. Corbridge, CRC Press, Taylor & Francis Group, 6th edn, 2013 Search PubMed.
-
(a) O. I. Kolodiazhnyi, Russ. Chem. Rev., 2011, 80, 883–910 CrossRef CAS;
(b)
J. C. Tebby, D. W. Allen, D. Loakes and R. Pajkert, Organophosphorus Chemistry, RSC, Cambridge, 2010, vol. 39 RSC;
(c)
R. Engel, Handbook of Organophosphorus Chemistry, New York, 1992 Search PubMed;
(d)
L. D. Quin, A Guide to Organophosphorus Chemistry, Wiley-VCH, New York, 2000 Search PubMed;
(e)
J.-P. V. Majoral, New Aspects in Phosphorus Chemistry, Springer, 2004 CrossRef.
- For selected reviews on C–H functionalization using transition metals, see:
(a) F. Kakiuchi, S. Sekine, Y. Tanaka, A. Kamatani, M. Sonoda, N. Chatani and S. Murai, Bull. Chem. Soc. Jpn., 1995, 68, 62–83 CrossRef CAS;
(b) O. Daugulis, H.-Q. Do and D. Shabashov, Acc. Chem. Res., 2009, 42, 1074–1086 CrossRef CAS PubMed;
(c) X. Chen, K. M. Engle, D.-H. Wang and J.-Q. Yu, Angew. Chem., Int. Ed., 2009, 48, 5094–5115 CrossRef CAS PubMed;
(d) R. Giri, B.-F. Shi, K. M. Engle, N. Maugel and J.-Q. Yu, Chem. Soc. Rev., 2009, 38, 3242–3272 RSC;
(e) T. W. Lyons and M. S. Sanford, Chem. Rev., 2010, 110, 1147–1169 CrossRef CAS PubMed;
(f) D. A. Colby, R. G. Bergman and J. A. Ellman, Chem. Rev., 2010, 110, 624–655 CrossRef CAS PubMed;
(g) J. Wencel-Delord, T. Dröge, F. Liu and F. Glorius, Chem. Soc. Rev., 2011, 40, 4740–4761 RSC;
(h) P. B. Arockiam, C. Bruneau and P. H. Dixneuf, Chem. Rev., 2012, 112, 5879–5918 CrossRef CAS PubMed;
(i) L. Ackermann, Acc. Chem. Res., 2014, 47, 281–295 CrossRef CAS PubMed.
-
(a) Z. Huang, H. N. Lim, F. Mo, M. C. Young and G. Dong, Chem. Soc. Rev., 2015, 44, 7764–7786 RSC;
(b) K. M. Engle, T.-S. Mei, M. Wasa and J.-Q. Yu, Acc. Chem. Res., 2012, 45, 788–802 CrossRef CAS PubMed;
(c) R. Giri, N. L. Maugel, J.-J. Li, D.-H. Wang, S. P. Breazzano, L. B. Saunders and J.-Q. Yu, J. Am. Chem. Soc., 2007, 129, 3510–3511 CrossRef CAS PubMed;
(d) D.-H. Wang, M. Wasa, R. Giri and J.-Q. Yu, J. Am. Chem. Soc., 2008, 130, 7190–7191 CrossRef CAS PubMed;
(e) G. Li, L. Wan, G. Zhang, D. Lewo, J. Spangler and J.-Q. Yu, J. Am. Chem. Soc., 2015, 137, 4391–4397 CrossRef CAS PubMed.
- For transition-metal-catalyzed C–H functionalization of phosphorus-containing molecules, see:
(a) Z. Zhang, P. H. Dixneuf and J.-F. Soulé, Chem. Commun., 2018, 54, 7265–7280 RSC;
(b) Y.-N. Ma, S.-X. Li and S.-D. Yang, Acc. Chem. Res., 2017, 50, 1480–1492 CrossRef CAS PubMed.
-
(a) L. Y. Chan, S. Kim, T. Ryu and P. H. Lee, Chem. Commun., 2013, 49, 4682–4684 RSC;
(b) X. Meng and S. Kim, Org. Lett., 2013, 15, 1910–1913 CrossRef CAS PubMed.
-
(a) H.-L. Wang, R.-B. Hu, H. Zhang, A.-X. Zhou and S.-D. Yang, Org. Lett., 2013, 15, 5302–5305 CrossRef CAS PubMed;
(b) H.-Y. Zhang, H.-M. Yi, G.-W. Wang, B. Yang and S.-D. Yang, Org. Lett., 2013, 15, 6186–6189 CrossRef CAS PubMed;
(c) Y. Unoh, Y. Hashimoto, D. Takeda, K. Hirano, T. Satoh and M. Miura, Org. Lett., 2013, 15, 3258–3261 CrossRef CAS PubMed;
(d) D. Zhao, C. Nimphius, M. Lindale and F. Glorius, Org. Lett., 2013, 15, 4504–4507 CrossRef CAS PubMed;
(e) S. Park, B. Seo, S. Shin, J.-Y. Son and P. H. Lee, Chem. Commun., 2013, 49, 8671–8673 RSC;
(f) M. Itoh, Y. Hashimoto, K. Hirano, T. Satoh and M. Miura, J. Org. Chem., 2013, 78, 8098–8104 CrossRef CAS PubMed;
(g) D. Gwon, D. Lee, J. Kim, S. Park and S. Chang, Chem. – Eur. J., 2014, 20, 12421–12425 CrossRef CAS PubMed;
(h) Y.-N. Ma, H.-Y. Zhang and S.-D. Yang, Org. Lett., 2015, 17, 2034–2037 CrossRef CAS PubMed;
(i) Z.-J. Du, J. Guan, G.-J. Wu, P. Xu, L.-X. Gao and F.-S. Han, J. Am. Chem. Soc., 2015, 137, 632–635 CrossRef CAS PubMed;
(j) S.-X. Li, Y.-N. Ma and S.-D. Yang, Org. Lett., 2017, 19, 1842–1845 CrossRef CAS PubMed;
(k) Y. Sun and N. Cramer, Angew. Chem., Int. Ed., 2017, 56, 364–367 CrossRef CAS PubMed;
(l) Y.-S. Jang, M. Dieckmann and N. Cramer, Angew. Chem., Int. Ed., 2017, 56, 15088–15092 CrossRef CAS PubMed;
(m) Y. Sun and N. Cramer, Chem. Sci., 2018, 9, 2981–2985 RSC.
- For reviews on inexpensive metal catalyzed C–H activation reactions, see:
(a) B. Su, Z.-C. Cao and Z.-J. Shi, Acc. Chem. Res., 2015, 48, 886 CrossRef CAS PubMed;
(b) B. Zhan, B. Liu and B.-F. Shi, Chin. Sci. Bull., 2015, 60, 2907–2917 CrossRef;
(c) M. Moselage, J. Li and L. Ackermann, ACS Catal., 2016, 6, 498–525 CrossRef CAS;
(d) G. Cera and L. Ackermann, Top. Curr. Chem., 2016, 374, 191–224 Search PubMed;
(e) J. Liu, G. Chen and Z. Tan, Adv. Synth. Catal., 2016, 358, 1174–1194 CrossRef CAS;
(f) W.-H. Rao and B.-F. Shi, Org. Chem. Front., 2016, 3, 1028–1047 RSC;
(g) M. Shang, S.-Z. Sun, H.-L. Wang, M.-M. Wang and H.-X. Dai, Synthesis, 2016, 4381–4399 CAS;
(h) A. Dey, N. Thrimurtulu and C. M. R. Volla, Org. Lett., 2019, 21, 3871–3875 CrossRef CAS PubMed.
-
(a) T. T. Nguyen, L. Grigorjeva and O. Daugulis, ACS Catal., 2016, 6, 551–554 CrossRef CAS PubMed;
(b) X. Yao, L. Jin and Y. Rao, Asian J. Org. Chem., 2017, 6, 825–830 CrossRef CAS.
-
(a) M. Shang, S.-Z. Sun, H.-X. Dai and J.-Q. Yu, J. Am. Chem. Soc., 2014, 136, 3354–3357 CrossRef CAS PubMed;
(b) M. Shang, S.-Z. Sun, H.-L. Wang, B. N. Laforteza, H.-X. Dai and J.-Q. Yu, Angew. Chem., Int. Ed., 2014, 53, 10439–10442 CrossRef CAS PubMed;
(c) M. Shang, H.-L. Wang, S.-Z. Sun, H.-X. Dai and J.-Q. Yu, J. Am. Chem. Soc., 2014, 136, 11590–11593 CrossRef CAS PubMed;
(d) M. Shang, S.-Z. Sun, H.-X. Dai and J.-Q. Yu, Org. Lett., 2014, 16, 5666–5669 CrossRef CAS PubMed;
(e) H.-L. Wang, M. Shang, S.-Z. Sun, Z.-L. Zhou, B. N. Laforteza, H.-X. Dai and J.-Q. Yu, Org. Lett., 2015, 17, 1228–1231 CrossRef CAS PubMed;
(f) S.-Z. Sun, M. Shang, H.-L. Wang, H.-X. Lin, H.-X. Dai and J.-Q. Yu, J. Org. Chem., 2015, 80, 8843–8848 CrossRef CAS PubMed;
(g) M. Shang, M.-M. Wang, T. G. Saint-Denis, M.-H. Li, H.-X. Dai and J.-Q. Yu, Angew. Chem., Int. Ed., 2017, 56, 5317–5321 CrossRef CAS PubMed;
(h) L.-L. Xu, X. Wang, B. Ma, M.-X. Yin, H.-X. Lin, H.-X. Dai and J.-Q. Yu, Chem. Sci., 2018, 9, 5160–5164 RSC;
(i) X. Wang, X. Yi, H. Xu and H.-X. Dai, Org. Lett., 2019, 21, 5981–5985 CrossRef CAS PubMed.
-
(a) T. Hayashi, M. Fukushima, M. Konishi and M. Kumada, Tetrahedron Lett., 1980, 21, 79–82 CrossRef CAS;
(b) T. Hayashi, M. Konishi, M. Fukushima, K. Kanehira, T. Hioki and M. Kumada, J. Org. Chem., 1983, 48, 2195–2202 CrossRef CAS;
(c) Y. Wang, D. Liu, Q. Meng and W. Zhang, Tetrahedron: Asymmetry, 2009, 20, 2510–2512 CrossRef CAS;
(d) A. Lumbroso, N. R. Vautravers and B. Breit, Org. Lett., 2010, 12, 5498–5501 CrossRef CAS PubMed;
(e) P. J. Guiry and C. P. Saunders, Adv. Synth. Catal., 2004, 346, 497–537 CrossRef CAS.
-
(a) W.-S. Huang, S. Liu, D. Zou, M. Thomas, Y. Wang, T. Zhou, J. Romero, A. Kohlmann, F. Li, J. Qi, L. Cai, T. A. Dwight, Y. Xu, R. Xu, R. Dodd, A. Toms, L. Parillon, X. Lu, R. Anjum, S. Zhang, F. Wang, J. Keats, S. D. Wardwell, Y. Ning, Q. Xu, L. E. Moran, Q. K. Mohemmad, H. G. Jang, T. Clackson, N. I. Narasimhan, V. M. Rivera, X. Zhu, D. Dalgarno and W. C. Shakespeare, J. Med. Chem., 2016, 59, 4948–4964 CrossRef CAS PubMed;
(b) Y. Chen, J. Wu, A. Wang, Z. Qi, T. Jiang, C. Chen, F. Zou, C. Hu, W. Wang, H. Wu, Z. Hu, W. Wang, B. Wang, L. Wang, T. Ren, S. Zhang, Q. Liu and J. Liu, Eur. J. Med. Chem., 2017, 139, 674–697 CrossRef CAS PubMed;
(c) J. Jang, J. B. Son, C. To, M. Bahcall, S. Y. Kim, S. Y. Kang, M. Mushajiang, Y. Lee, P. A. Jänne, H. G. Choi and N. S. Gray, Eur. J. Med. Chem., 2017, 136, 497–510 CrossRef CAS PubMed;
(d) C. Alonso, M. Fuertes, E. Martín-Encinas, A. Selas, G. Rubiales, C. Tesauro, B. K. Knudssen and F. Palacios, Eur. J. Med. Chem., 2018, 149, 225–237 CrossRef CAS PubMed.
- For selected examples of C–H hydroxylation, see:
(a) T. Jintoku, K. Nishimura, K. Takaki and Y. Fujiwara, Chem. Lett., 1990, 1687–1688 CrossRef CAS;
(b) Y.-H. Zhang and J.-Q. Yu, J. Am. Chem. Soc., 2009, 131, 14654–14655 CrossRef CAS PubMed;
(c) Y. Yan, P. Feng, Q.-Z. Zheng, Y.-F. Liang, J.-F. Lu, Y. Cui and N. Jiao, Angew. Chem., Int. Ed., 2013, 52, 5827–5831 CrossRef CAS PubMed;
(d) Y. Yang, Y. Lin and Y. Rao, Org. Lett., 2012, 14, 2874–2877 CrossRef CAS PubMed;
(e) V. S. Thirunavukkarasu, J. Jonathan Hubrich and L. Ackermann, Org. Lett., 2012, 14, 4210–4213 CrossRef CAS PubMed;
(f) X. Yang, G. Shan and Y. Rao, Org. Lett., 2013, 15, 2334–2337 CrossRef CAS PubMed;
(g) W. Liu and L. Ackermann, Org. Lett., 2013, 15, 3484–3486 CrossRef CAS PubMed;
(h) F. Yang, K. Rauch, K. Kettelhoit and L. Ackermann, Angew. Chem., Int. Ed., 2014, 53, 11285–11288 CrossRef CAS PubMed;
(i) X. Chen, X.-S. Hao, C. E. Goodhue and J.-Q. Yu, J. Am. Chem. Soc., 2006, 128, 6790–6791 CrossRef CAS PubMed;
(j) X. Li, Y.-H. Liu, W.-J. Gu, B. Li, F.-J. Chen and B.-F. Shi, Org. Lett., 2014, 16, 3904–3907 CrossRef CAS PubMed;
(k) X.-Y. Chen, S. Ozturk and E. J. Sorensen, Org. Lett., 2017, 19, 6280–6283 CrossRef CAS PubMed.
Footnotes |
† Electronic supplementary information (ESI) available: Data for new compounds and experimental procedures. See DOI: 10.1039/c9cc08879b |
‡ These authors contributed equally to this work. |
|
This journal is © The Royal Society of Chemistry 2020 |
Click here to see how this site uses Cookies. View our privacy policy here.