Investigation of metal modulation of oxytocin structure receptor-mediated signaling†
Received
9th November 2022
, Accepted 16th December 2022
First published on 4th January 2023
Abstract
Oxytocin is a 9-amino acid peptide hormone. Since its discovery in 1954, it has most commonly been studied in relation to its role in stimulating parturition and lactation. However, it is now known that oxytocin has a widely diverse set of functions throughout the body including neuromodulation, bone growth, and inflammation. Previous research has suggested that divalent metal ions may be required for oxytocin activity, but the exact metal species and specific pathways have yet to be fully elucidated. In this work, we focus on characterizing copper and zinc bound forms of oxytocin and related analogs through far-UV circular dichroism. We report that Cu(II) and Zn(II) bind uniquely to oxytocin and all analogs investigated. Furthermore, we investigate how these metal bound forms may affect downstream signaling of MAPK activation upon receptor binding. We find that both Cu(II) and Zn(II) bound oxytocin attenuates the activation of the MAPK pathway upon receptor binding relative to oxytocin alone. Interestingly, we observed that Zn(II) bound forms of linear oxytocin facilitate increased MAPK signaling. This study lays the foundation for future work on elucidating the metal effects on oxytocin's diverse bioactivity.
Introduction
Oxytocin (OT) is a peptide hormone that is most widely known for its clinical applications in stimulating childbirth as well as its role in stimulating parturition and lactation. OT and its synthetic analogs have been extensively used in clinical applications since the 1960s; however, the dosing and side effects are often unpredictable in large part due to an incomplete understanding of its regulation and mechanism of action. Several decades ago, in an effort to understand the drivers of OT action, divalent metal ions were found to influence the hormonal activities of OT.1 However, it remains unclear how metals influence OT function at the molecular level and the downstream bioactivity.
Early studies suggested that divalent metals could enhance the binding of OT to the OT receptor (OTR) on mammary gland tissues.2 Researchers initially postulated that the enhanced receptor binding was due to metal ions interacting with the OTR to increase the affinity and concentration of OT binding sites, though the molecular nature of these sites were not elucidated.1 However, several studies in the 1990s began investigating divalent metal interactions directly with the OT peptide itself, primarily employing mass spectrometry, computational calculations, or a combination thereof. These studies demonstrated that both Cu(II) and Zn(II) can coordinate to OT and change its conformation, albeit with different binding modes.3–6
Zn(II) is posited to interact with OT through interaction of backbone carbonyls under acidic, but not basic, conditions.3,4 Bowers and coworkers used Cross Section and Hydration Energy Mass Spectrometry (MS) alongside Chemistry at Harvard Macromolecular Mechanics (CHARMM) force field and Density Functional Theory (DFT) calculations to predict that Zn(II) binds to OT via six of the backbone carbonyl oxygens to form a near-perfect octahedral complex that stabilizes a near-planar surface ideal for OTR binding and pinpointed these six backbone carbonyls to those of the Tyr2, Ile3, Gln4, Cys6, and Lys8 and Gly9.3
Cu(II) has been shown to stably coordinate OT in the gas phase under both acidic and basic conditions, with evidence pointing to a highly stable, planar 4N coordination mode.3,4 This was initially demonstrated with both spectroscopic and potentiometric studies by Danyi et al.7 and Bal et al.8 While collective data on Zn(II)-binding is generally in good agreement with respect to which residues are involved, reports on Cu(II)-binding show some discrepancy as to the exact location of binding. While the early spectroscopic studies revealed that Cys1, Tyr2, Ile3, and Gln4 are involved in Cu(II) coordination,8 later studies by collision-induced dissociation (CID) mass spectrometry pointed to complexation involving Cys1, Cys6, Leu8, and Gly9.3 These discrepancies may be in part due to the strong pH-dependence of Cu(II) complexation.4,9,10 CID and electron-capture dissociation (ECD) MS measurements alongside calculations have shown that at pH 10, Cu(II) coordinates the backbone nitrogens of Cys1, Cys6, Leu8, and Gly9.11 Lowering the pH to pH 5 allows Cu(II) to coordinate the backbone nitrogens of the Cys1, Tyr2, Ile3, Gln4 segment.8 As the pH is lowered further to pH 3, Cu(II) coordination shifts to 4O involving the Tyr2, Ile3, Cys6, and Gly9.12 Calculations have predicted that at pH 7, Cu(II) coordinates to the backbone nitrogens of Ile3, Gln4, Asn4, Cys6.3 Very recently, further PRE NMR analysis indicated that a free amine of Cys1 is required, with Cu(II) binding at that –NH2 first, followed by Tyr2, Ile3, and Gln4.13 The low-energy ensemble from this analysis showed structural rigidity at the Tyr2 side chain ring, indicating the possible presence of pi-cation interaction. Taken together, this would suggest that the binding mode of copper is heavily influenced by solvent environments and pH.
Despite the repeated observations that Cu(II) and Zn(II) can bind OT and that their binding modes are distinct, it remains unclear how these molecular interactions alter peptide conformation with respect to receptor interactions and downstream hormone regulatory pathways. Majority of the studies that have investigated the consequences of OT/metal on bioactivity assess physiological effects at the whole tissue rather than the molecular or biochemical level. Moreover, such studies focus on the metal effects on OT-induced uterine contractions. Recent years have revealed new and divergent functions of OT in different tissues including neuromodulation, metabolic regulation, and inflammation, opening up new questions as to what effectors, allosteric modulators, and regulators might be involved in directing or tuning OT signaling pathways.14–17 In particular, many of these functions are associated with activation of the ERK1/2 MAPK pathway.18–22
To this end, we hypothesize that Zn(II) and Cu(II) play differential roles in modulating and directing OT towards the various signaling pathways that it can activate, and that a closer investigation of the molecular components that govern OT/metal interactions is warranted. In this work, we assess the impact of Zn(II) and Cu(II) on peptide secondary structure under biologically relevant conditions not only with native oxytocin, but in both cyclic and linear analogs where potential binding regions are perturbed (Fig. 1; full chemical structures in Fig. S1, ESI†). While the most-studied form of OT is its cyclized 9-amino acid form wherein the Cys1 and Cys6 are oxidized to form a disulfide bond (termed here oxOT for oxidized OT), emerging studies suggest that modified analogs, including its linear reduced form, may exert differing functions from the canonical oxOT form.23 In addition to studying both oxOT and reduced OT (rOT), we investigated carbetocin, a synthetic analog used clinically, where the disulfide linkage is replaced with a thioether and methoxy-tyrosine replaces Tyr2 position. Additionally, carbetocin is deaminated at the C-terminus whereas oxytocin has its C-terminus amidated. Furthermore, we synthesized and studied the mutant OT Y2F with reduced cysteines (rOTF) where the tyrosine is replaced with a phenyalanine to determine if the tyrosine hydroxyl is required for binding.
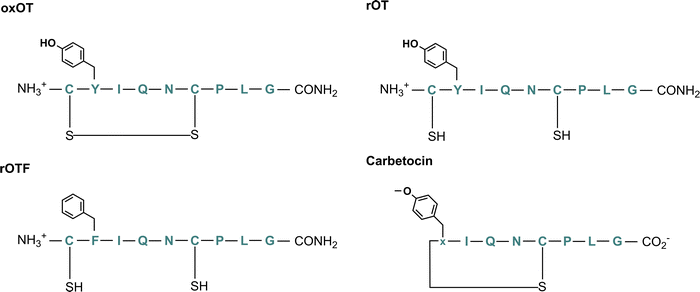 |
| Fig. 1 Chemical structure of oxytocin (oxOT), reduced oxytocin (rOT), reduced Y2F-oxytocin (rOTF), and carbetocin. | |
Our spectroscopic studies find that both Cu(II) and Zn(II) can bind to all analogs, but the binding modes and conformational consequences differ between the two metals ions and the analogs. We find the native Tyr2 residue is involved in conformational dynamics associated with Cu(II) binding, as evidenced by large perturbations in the spectroscopic signatures corresponding to the aromatic side chain. In contrast, Zn(II) does not appear to bind near the Tyr2. In contrast, Zn(II) induces more notable changes in the spectroscopic region of the peptide that corresponds to the backbone and tail, particularly with rOT. Furthermore, we demonstrate that binding of Cu(II) or Zn(II) to oxOT is competitive. We extend these studies to investigate how the two metals influence ERK1/2 MAPK activation. Our data show that both Zn(II)- and Cu(II)-binding to OT decrease MAPK activation relative to OT alone, suggesting that these metal bound forms have differing interactions with the oxytocin receptor in association with the MAPK signaling pathway. In contrast, Zn(II) enhances MAPK activation by linear OT, suggesting that the metal ion may stabilize the receptor recognition site on an otherwise less-ordered linear form.
Results and discussion
Selection of OT analogs used for this study
We aimed to first assess and compare how Cu(II) and Zn(II) binding to oxOT affects the peptide's structure under buffered aqueous conditions at physiologically relevant pH. We applied circular dichroism (CD) spectroscopy, a common technique for evaluating protein and peptide structure via optical activity. Most reports on oxytocin structure have evaluated the CD spectra of the peptide and related peptides in the near-UV region (250–400 nm), or, in the case of Cu(II)-binding, in the d–d transition region (400–800 nm). The focus on the near-UV region may be attributed to the presence of the chromophoric amino acid, tyrosine (at Tyr2), and a disulfide bond, which both have intense optical activities in this spectral region. However, this region may also be sensitive to charge-transfer transitions with Cu(II) that may not occur with the d10 configuration of Zn(II). To allow for direct comparisons between the two metal ions, we instead investigated the impact of Cu(II) and Zn(II) on the far-UV region of the CD. To facilitate spectral interpretation, the CD spectra of additional analogs were acquired. Three were selected wherein either putative metal ion binding site or the optically-active features are altered: reduced OT (rOT), which lacks the disulfide bond and instead has two free thiols at Cys1 and Cys6; reduced OTF (rOTF), which similarly lacks the disulfide bond but also has a phenylalanine substituted for Tyr2; and carbetocin, which has a methylated Tyr2 and a thioether bond instead of a disulfide bond bridging positions 1 and 6 (Fig. 1). Carbetocin is of added interest as it is a clinically used analog that is reportedly more shelf-stable and resistant to degradation once administered than OT. Despite the increased chemical robustness of carbetocin, research shows that the effect on potency can vary by application. For instance, while potency is increased with respect to prevention of post-partum hemorrhage, decreased potency in single-dose induction of uterine contractions is observed.24,25 This may suggest tissue-specific or co-factor differences in the action of oxOT as compared to carbetocin. Indeed, molecular pharmacology studies have shown that OT including carbetocin can elicit functionally different effects despite interactions with the same receptor.26,27
Far-UV circular dichroism spectra of oxytocin and its analogs
As previously reported in literature, the spectrum of oxOT in the absence of metals (apo-oxOT) shows a positive band centered at 225 nm and a positive shoulder (Fig. 2), which has previously been attributed primarily to a Tyr2 transition, but with possible contributions from the amide backbone and disulfide bond.28 The spectrum also exhibits a minimum centered at 196 nm, which may represent the disordered region of the C-terminal amino acid tail that is excluded from the tocin ring. In comparing the CD spectra of the apo forms of oxOT and redOT the peak profiles look similar at wavelengths higher than 200 nm, but redOT adapts a more negative ellipticity at <200 nm, which influences the relative values of the remainder of the spectrum. Negative ellipticity values below 200 nm are frequently suggestive of random coil formations as might be expected with redOT when the cyclic structure is no longer stabilized by the disulfide linkage. The 225 nm band is both reduced and red-shifted in the CD spectra of rOTF as would be expected for a Phe substitution, further corroborating the strong contribution of Tyr2 transitions to this peak. Interestingly, rOTF exhibits a less negative ellipticity below 200 nm, possibly indicating that this analog exhibits less disorder than rOT. Surprisingly, carbetocin shows marked differences at <200 nm despite having the same tail region as oxOT as well the structural constraints of cyclization. Given that the thioether bond of carbetocin that replaces the disulfide bond of oxOT is proximal to the tail region, it is possible that conformational changes to the tocin ring that stems from the –CH2 replacement may reorient the tail region to restrict structural disorder. The carbetocin CD spectrum shows additional differences in the 225 nm band, suggesting that tyrosine methylation red-shifts and reduces the molar ellipticity of this transition.
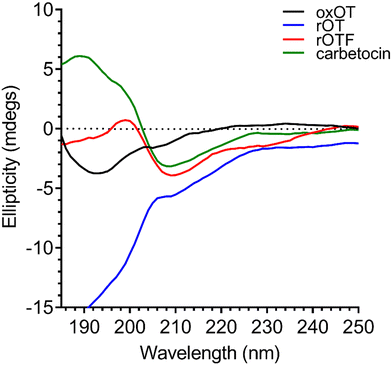 |
| Fig. 2 Circular dichroism spectra of 40 μM oxOT, rOT, rOTF, and carbetocin (15 mM phosphate, pH 7.4). | |
Effects of Cu(II) and Zn(II) Binding to oxOT structure
Using the far-UV CD spectroscopy, we probed how Cu(II) and Zn(II) affects the secondary structural features of oxOT. Both Cu(II) and Zn(II) increase the molar ellipticity of the band at 196 nm (Fig. 3). Cu(II) increases the molar ellipticity value relative to apo-oxOT from −3 to −1 millidegrees, in contrast to Zn(II) which increases it from −3 to 0 millidegrees, suggesting that metal ion binding may alter the conformation of the tail region. Stoichiometric addition of Cu(II) also increases the intensity of the band centered at 225 nm. Zn(II) has a similar but less pronounced effect on this 225 nm band (Fig. 3) suggesting that Zn(II) does not induce the same structural effects as Cu(II), which are likely on the Tyr2 transition. Previous experiments performed at pH 10.3 in the gas phase suggest that Zn(II) binding orients Ile3, Gln4, and Asn5 differently than apo and Cu(II)-oxOT, but changes to these residues may not be observable by CD. Titration of sub-(0.5 equivalents) to super-(4.0 equivalents) of Cu(II) into oxOT show the increase at 225 nm up to 1.0 equivalents of Cu(II) (Fig. S2, ESI†), suggesting a 1
:
1 Cu(II) to oxOT ratio in the complex. Titration of Zn(II) into oxOT also suggests a 1
:
1 binding ratio (Fig. S2, ESI†).
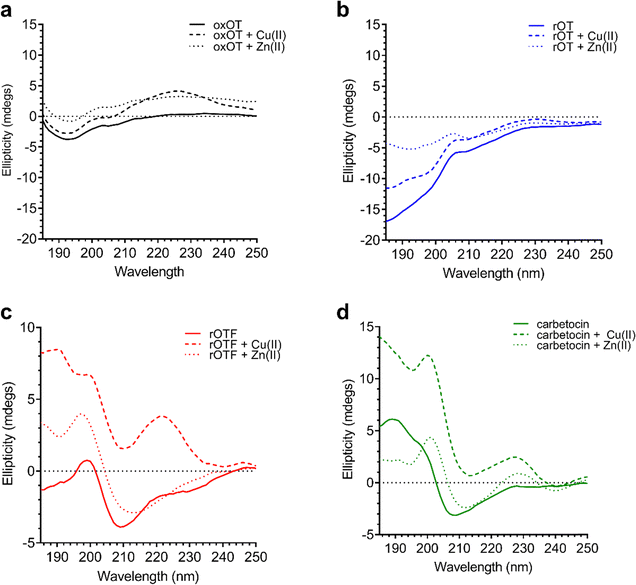 |
| Fig. 3 Circular dichroism spectra of 40 μM oxOT, rOT, rOTF, and carbetocin in the absence or presence of one equivalent of either Cu(II) or Zn(II) (15 mM phosphate, pH 7.4). | |
We further applied CD spectroscopy to assess binding competition between the two metal ions. Understanding binding competition would provide insight into how these metals could influence, and possibly regulate, oxOT function in vivo. When added to oxOT pre-incubated with one equivalent of Zn(II) (Zn(II)-oxOT), Cu(II) addition increases the band at 225 nm (Fig. S3, ESI†), but additional Cu(II) equivalents (2.0 equivalents) are required to reach the maximum change relative to apo-oxOT. At 2.0 equivalents of Cu(II) to Zn(II)-oxOT, the spectrum resembles that of oxOT pre-incubated with stoichiometric amounts of Cu(II). In contrast, while addition of Zn(II) to Cu(II)-oxOT at one equivalent of Cu(II) leads to a increase in the band at 196 nm (Fig. S3B, ESI†), even 4.0 equivalents of Zn(II) does not restore the spectrum to that of Zn(II)-oxOT. These data show that both metal ions bind to oxOT at neutral pH and either compete with each other for the same ligand set or preclude the other metal ion from binding. Moreover, Cu(II) binding seems to drastically impact the ability of Zn(II) to complex to the peptide, suggesting a shared region of binding with preferential affinity for Cu(II).
Given the ability of Cu(II) to change the 225 nm band in the CD spectrum of oxOT alongside the recent paramagnetic relaxation enhancement nuclear magnetic resonance (PRE NMR) studies pointing to pi-cation interactions, we applied electronic absorption spectra to assess the impact of the metal ion on the Tyr phenolic absorption spectrum. Apo-oxOT exhibits characteristic bands at 220–230 nm from the peptide backbone and 280 nm due to absorption by the Tyr2 ring (Fig. 4A). Ionization of tyrosine hydroxyl groups has been shown to red-shift the spectrum of the amino acid to 295 nm.29,30 Stoichiometric addition of Cu(II), however, induces a blue-shift from 275 nm to 270 nm alongside a notable increase in intensity. This spectral change is accompanied by a rise in a band centered at 515 nm, which is consistent with a d–d transition at the Cu(II) center (Fig. 4A). This energy and extinction coefficient correlate well with backbone amide coordination, which is consistent with previously published findings.12 Addition of one stoichiometric equivalent of Zn(II) or other d-block transition metals (Cr(III), Mn(II), Fe(III), Co(II), and Ni(II)) does not yield changes to the UV-vis spectra of apo-oxOT indicating a lack of detectable metal binding interaction with oxOT that would perturb either the Tyr2 residue or the peptide backbone absorbances. Control absorbance spectra of the metal salts alone can be found in the ESI† (Fig. S4). We further probed the binding by titration of sub-(0.5 equivalents) to super-(5.0 equivalents) stoichiometric Cu(II) into oxOT (Fig. 4B). The resulting spectra show both the increase and blue-shift of the tyrosine band and the increase of the d–d transition up to 1 equivalent Cu(II), corroborating the presence of a 1
:
1 Cu(II)
:
OT complex that was observed in the CD spectra (Fig. 4B). It should be noted that at higher copper stoichiometries (4 equivalents) the baseline in the absorption spectrum appears to be floated indicating possible aggregation under high copper ratios. We also measured the emission spectra of oxOT with excitation at 275 nm, which is attributed to the tyrosine residue (Fig. S4, ESI†). While a reduction in emission intensity is observed with Cu(II) addition, the decrease is modest, and no wavelength shifts are observed. This would further indicate that Cu(II) binding is not likely occurring through direct coordination to the tyrosine ring system.
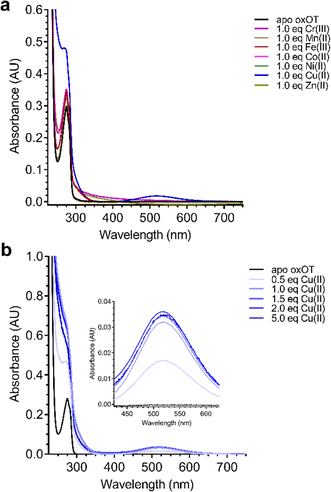 |
| Fig. 4 Electronic absorption spectra of (A) 200 μM oxOT (15 mM phosphate, pH 7.4) in the absence (apo) and presence of 200 μM CrCl3, MnCl2, FeCl3, CoCl2, NiCl2, CuCl2, ZnCl2 and (B) CuCl2 titrated into 200 μM oxOT (15 mM MOPS, pH 7.4) from sub-(0.5 eq.) to super-stoichiometric (5.0 eq.) ratios. CrCl3, MnCl2, FeCl3, CoCl2, and NiCl2 have negligible changes to the spectrum of oxOT, whereas CuCl2 alters the spectrum of oxytocin by blue-shifting the tyrosine band from 275 to 270 nm and forming a d–d band centered at 515 nm. | |
We investigated whether the modifications present in the analogs would affect their metal-binding capacity relative to oxOT. Surprisingly, the CD spectra are shifted with the addition of Cu(II) or Zn(II) for all the analogs, suggesting that these can still interact with the metal ions. This supports the notion that the peptide backbone plays a significant role in binding to both metal ions. The differences in molar ellipticity with metal addition were parsed between the peaks at ≤200 nm, which likely represents the overall peptide secondary structure (amide bond transitions) and tail region and the peaks in the 225–230 nm range, which is expected to have the strongest contribution from the aromatic ring at the 2 position (Fig. 5). With all analogs, Cu(II) increased the ellipticity at the ≤200 nm region to a similar degree, while Zn(II) increased the ellipticity for oxOT, rOT, and rOTF while decreasing the ellipticity for the carbetocin. The increase in ellipticity with the linear peptides is more pronounced with rOT than rOTF. The increase in ellipticity may indicate that Zn(II) can reduce the disorder of the linear analog structures. Differences in metal-dependent effects are observed in the 220–230 nm range as compared to the ≤200 nm range, with Cu(II) showing a more notable effect and increase in ellipticity in all cases as compared to Zn(II). This Cu(II)-dependent effect is most prominent with carbetocin, followed by oxOT, suggesting that the ring structure may help orient interactions of the metal ion near the Tyr region. For the reduced analogs (rOT and rOTF) mass spectrometry analysis revealed that the addition of Cu(II) oxidizes the free thiols to disulfides as would be expected given their respective redox potentials (Fig. S6, ESI†). The mass spectra observed were consistent with intra-disulfide bonding as opposed to dimerized inter-disulfide bonding or other oxidation products (Fig. S6, ESI†). Furthermore, it appears that the oxidized forms of rOT and rOTF subsequently form complexes with copper as suggested by copper adducts present in the mass spectra. CD spectra further show that rOT plus Cu(II) is distinct from oxOT both with and without Cu(II).
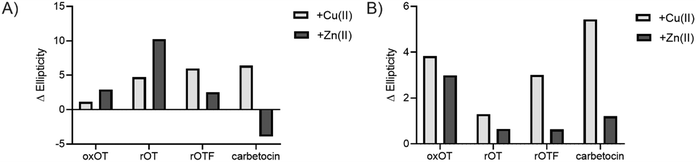 |
| Fig. 5 Difference in ellipticity of the peaks in the circular dichroism spectra of oxOT and its analogs in the presence of Cu(II) (light gray) or Zn(II) (dark gray), calculated as the difference in apo-peptide and metal-treated peptide (Δ Ellipticity = Ellipticity (metal-treated) – Ellipticity (apo-treated)). Values were calculated for the peaks at (A) ≤200 nm (195 nm, 190 nm, 200 nm, and 190 nm for oxOT, rOT, rOTF, and carbetocin, respectively) to assess changes in the tail or overall structure and (B) the 225–230 nm range (225 nm, 230 nm, 230 nm, and 228 nm for oxOT, rOT, rOTF, and carbetocin, respectively) to assess changes in the conformation of the aromatic ring of the Tyr, Phe, or O-Me Tyr side chains. | |
Metal effects on oxytocin/receptor-mediated MAPK signaling
Ligand binding to the oxytocin receptor can elicit a variety of signaling pathways in tissue-specific ways including increased cytosolic calcium, cAMP activation, and activation of MAP kinases. The mechanistic or structural underpinnings of this pathway divergence remain unclear but may point to distinct active conformations of the ligand–receptor complex.31 Whether metal ions are involved in activating the oxOT/OTR axis remains unexplored. The CD data strongly support that both Cu(II) and Zn(II) interactions with oxOT and its induce structural changes in distinct manners. We thus investigated the potential consequences of the metal-induced structural changes on their receptor-mediated bioactivity. We focused on the ability of oxOT/OTR binding to activate MAPK to begin querying the influence of metals on receptor interactions. HEK293T cells were transiently transfected to express a GFP-tagged human OTR. Cells were stimulated for five minutes with the apo peptides or peptides preincubated with Cu(II) or Zn(II). Lysates were collected and western blots were used to analyze total and phosphorylated MAPK expression. Densitometry was performed to quantify the ratio of phosphorylated MAPK to total MAPK where increased phosphorylation indicates activation of the MAPK pathway (Fig. 6; representative blots in Fig. S7, ESI†).
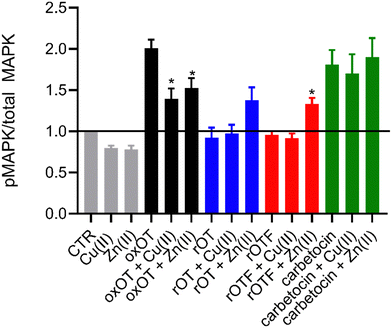 |
| Fig. 6 Densitometry of western blots for total and phosphorylated MAPK reveal metal-dependent modulation of MAPK activation by oxytocin (n = 3 to 4). Statistical significance of peptide alone compared to metal bound peptide was assessed by calculating p-values using unpaired t-test, * p < 0.05. | |
As expected, we observe strong activation of the MAPK pathway by oxOT alone relative to the no-peptide control. Interestingly, Cu(II) and Zn(II) addition to oxOT both attenuate this activation, suggesting that the metal-bound forms of oxOT either do not interact with the OT receptor as strongly or that the receptor-ligand conformation does not mediate MAPK signaling. Interestingly, a previous study using preformed Cu-oxOT complex showed no significant difference between uterine contraction with or without copper complexation.32 Taken together, this warrants and provides a foundation for further investigations of metal-oxytocin complexes and subsequent receptor activation and downstream signaling. Consistent with the traditional dogma that oxOT is the bioactive form, we observe that neither rOT nor rOTF activate MAPK signaling. However, both reduced OT and reduced OTF show an increase in activation when bound by Zn(II) but no change relative to peptide alone when bound by Cu(II). Alongside the CD data (Fig. 3), the Zn(II)-dependent gain-of-function of the linear peptides may suggest that Zn(II) may reduce the disorder of the linear peptide towards a more rigid structure like the cyclic peptides, facilitating receptor binding, but further studies are required to substantiate this hypothesis. While carbetocin promotes MAPK activation, it does so to a lesser degree than oxOT, and Zn(II) showed no effect on its activity. While a modest decrease in activation was observed with the addition of Cu(II) relative to apo-carbetocin, the difference was not statistically significant. Thus, while metal-binding is indeed observed for carbetocin, the lack of effects on MAPK activation may suggest that any induced conformational change by Cu(II) or Zn(II) does not significantly alter the synthetic analog's interaction with the receptor.
Conclusion
This work offers molecular insight into how metal ions bind to a peptide hormone and alters its structure as well as bioactivity. We first used electronic absorption spectroscopy to show a direct interaction between Cu(II) and oxOT in a 1
:
1 binding stoichiometry. Circular dichroism then showed that Cu(II) induces structural changes by means of reorienting the disulfide bond and the phenol ring of Tyr2 and Zn(II) induces structural changes in the carboxy terminal tail. In conjunction with fluorescence spectrometry of oxOT we show that the hydroxyl group of Tyr2 most likely coordinates to Cu(II) and alters the structure of the 20-membered ring formed by the disulfide bond. Furthermore, the competition studies show that Cu(II) is able to out-compete Zn(II) for oxOT. We then showed a metal-modulated effect on downstream signaling upon oxytocin receptor binding of oxytocin and where both Cu(II) and Zn(II) bound oxOT attenuate MAPK signaling relative to oxOT alone. Additionally, we note that Zn(II) bound rOT shows an increase in signaling from rOT alone and carbetocin with and without metal bound shows no significant changes in MAPK activation and lower MAPK signaling overall relative to oxOT. Taken together, this lays a foundation for future research at the receptor level of oxytocin and metal mediated bioactivity.
Metal-induced structural changes to a peptide hormone may have a number of effects on storage, secretion, stability, and/or signaling. In this case, it is possible that both Zn(II) and Cu(II) lend different structural integrities to oxOT at different points in oxOT lifetime. Physiologically, it is possible that Cu(II) is bound to oxOT in serum but, because the concentrations of metal ions surrounding specific tissues are believed to fluctuate, Zn(II) may be able to displace Cu(II) under specific concentrations. It still remains to be seen how Cu(II) would affect oxOT in serum, however, metal binding may be a key player in prevention of degradation; further studies in our lab are underway to test this hypothesis. The binding of metal ions to peptide hormones, particularly oxOT, may provide an additional source for regulation, and this study provides the foundation for future studies.
Author contributions
The manuscript was written through contributions of all authors. All authors have given approval to the final version of the manuscript.
Abbreviations
OT | Oxytocin |
oxOT | Oxidized oxytocin |
rOT | Reduced oxytocin |
rOTF | Reduced oxytocin derivative with phenylalanine at the second position |
CD | Circular dichroism spectroscopy |
Conflicts of interest
There are no conflicts to declare.
Acknowledgements
This work was supported by the NIH MIRA 1R35GM133684-01 and the Hartwell Foundation's Individual Biomedical Research Award. M. C. H. would also like to thank the ADVANCE CAMPOS Faculty Scholar Award for their support. We thank Jillian Emerson of Earth and Physical Sciences for the use of the CD. We also thank Martin Arcibal, Danielle J. Asiain, and Kelly Goodnight for their assistance in sample preparation and all members of the Heffern lab for valuable discussions.
References
- A. F. Pearlmutter and M. S. Soloff, Characterization of the Metal Ion Requirement for Oxytocin-Receptor Interaction in Rat Mammary Gland Membranes, J. Biol. Chem., 1979, 254(10), 3899–3906, DOI:10.1016/S0021-9258(18)50672-4
.
- M. S. Soloff and T. L. Swartz, Characterization Receptor in Rat of a Proposed Oxytocin Mammary Gland, J. Biol. Chem., 1973, 248(18), 6471–6478, DOI:10.1016/S0021-9258(19)43469-8
.
- T. Wyttenbach, D. Liu and M. T. Bowers, Interactions of the Hormone Oxytocin with Divalent Metal Ions, J. Am. Chem. Soc., 2008, 130(17), 5993–6000 CrossRef CAS PubMed
.
- H. Wei, X. Luo, Y. Wu, Y. Yao, Z. Guo and L. Zhu, Compositions and Conformations of Several Tranistion Metal Complexes with a Nonapeptide Hormone Oxytocin, J. Chem. Soc., Dalton Trans., 2000, 4196–4200, 10.1039/b004254o
.
- E. Mervinetsky, I. Alshanski, K. K. Tadi, A. Dianat, J. Buchwald, R. Gutierrez, G. Cuniberti, M. Hurevich and S. Yitzchaik, A Zinc Selective Oxytocin Based Biosensor, J. Mater. Chem. B, 2020, 8, 155–160, 10.1039/c9tb01932d
.
- A. J. Kleinnijenhuis, R. Mihalca, R. M. A. Heeren and A. J. R. Heck, Atypical Behavior in the Electron Capture Induced Dissociation of Biologically Relevant Transition Metal Ion Complexes of the Peptide Hormone Oxytocin, Int. J. Mass Spectrom., 2006, 253, 217–224, DOI:10.1016/j.ijms.2006.03.005
.
- P. Danyi, K. Varnagy, I. Sovago, I. Schon, D. Sanna and G. Micera, Potentiometric and Spectroscopic Studies on the Copper(II) Complexes of Peptide Hormones Containing Disulfide Bridges, J. Inorg. Biochem., 1995, 78, 69–78 CrossRef PubMed
.
- W. Bal, H. Kozlowski, B. Lammek and L. D. Pettit, Potentiometric and Spectroscopic Studies of the Cu(II) Complexes of Ala-Arg8 -Vasopressin and Oxytocin: Two Vasopressin-Like Peptides, J. Inorg. Biochem., 1992, 45, 193–202 CrossRef CAS
.
- H. Jeong and H. Kim, Determination of a Binding Site of Cu and Ni Metal Ions with Oxytocin Peptide by Electrospray Tandem Mass Spectrometry and Multiple Mass Spectrometry, Eur. J. Mass Spectrom., 2009, 15, 67–72, DOI:10.1255/ejms.977
.
- L. Joly, R. Antoine, A. Allouche and M. Broyer, Optical Properties of Isolated Hormone Oxytocin Dianions: Ionization, Reduction, and Copper Complexation Effects, J. Phys. Chem. A, 2009, 113, 6607–6611 CrossRef CAS PubMed
.
- D. Liu, A. B. Seuthe, O. T. Ehrler, X. Zhang, T. Wyttenbach, J. F. Hsu and M. T. Bowers, Oxytocin-Receptor Binding: Why Divalent Metals Are Essential, J. Am. Chem. Soc., 2005, 127, 2024–2025 CrossRef CAS PubMed
.
- L. Joly, R. Antoine, F. Albrieux, R. Ballivian, M. Broyer, F. Chirot, P. Dugourd, C. Greco and R. Mitric, Optical and Structural Properties of Copper - Oxytocin Dications in the Gas Phase, J. Phys. Chem. B, 2009, 113, 11293–11300 CrossRef CAS PubMed
.
- I. Alshanski, D. E. Shalev, S. Yitzchaik and M. Hurevich, Determining the Structure and Binding Mechanism of Oxytocin - Cu 2 + Complex Using Paramagnetic Relaxation Enhancement NMR Analysis, J. Biol. Inorg. Chem., 2021, 26(7), 809–815, DOI:10.1007/s00775-021-01897-1
.
- O. Chatterjee, K. Patil, A. Sahu, L. Gopalakrishnan and T. S. K. Prasad, An Overview of the Oxytocin-Oxytocin Receptor Signaling Network, J. Cell Commun. Signaling, 2016, 10, 355–360, DOI:10.1007/s12079-016-0353-7
.
- C. S. Carter, H. K. Caldwell, O. J. Bosch and C. S. Carter, The Oxytocin – Vasopressin Pathway in the Context of Love and Fear, Front. Endocrinol., 2017, 8, 356, DOI:10.3389/fendo.2017.00356
.
- C. S. Carter, W. M. Kenkel, E. L. Maclean, S. R. Wilson, A. M. Perkeybile, J. R. Yee, C. F. Ferris, H. P. Nazarloo, S. W. Porges and J. M. Davis,
et al., Is Oxytocin “Nature's Medicine”?, Pharmacol. Rev., 2020, 72, 829–861, DOI:10.1124/pr.120.019398
.
- C. Camerino, Oxytocin Involvement in Body Composition Unveils the True Identity of Oxytocin, Int. J. Mol. Sci., 2021, 22, 6383 CrossRef CAS PubMed
.
- K. Koike, A. Nohara, Z. X. Zhang and K. Hirota, Oxytocin Stimulates Mitogen-Activated Protein Kinase in Cultured Human Puerperal Uterine Myometrial Cells, Endocrinology, 1995, 136(5), 2082–2087 CrossRef PubMed
.
- V. Rimoldi, A. Reversi, E. Taverna, P. Rosa, M. Francolini, M. Parenti and B. Chini, Oxytocin Receptor Elicits Different EGFR/MAPK Activation Patterns Depending on Its Localization in Caveolin-1 Enriched Domains, Oncogene, 2003, 22, 6054–6060, DOI:10.1038/sj.onc.1206612
.
- B. M. Sanborn, M. Yang and M. Zhong, Myometrial Oxytocin Receptor Involves GG and Epidermal Growth Factor Receptor Tyrosine, Endocrinology, 2003, 144(7), 2947–2956, DOI:10.1210/en.2002-221039
.
- A. Blume, O. J. Bosch, S. Miklos, L. Torner, L. Wales, M. Waldherr and I. D. Neumann, Oxytocin Reduces Anxiety via ERK1/2 Activation: Local Effect within the Rat Hypothalamic Paraventricular Nucleus, Eur. J. Neurosci., 2008, 27, 1947–1956, DOI:10.1111/j.1460-9568.2008.06184.x
.
- J. Wei, L. Ma, P. Ju, B. Yang, Y. Wang and Y. Wang, Involvement of Oxytocin Receptor/Erk/MAPK Signaling in the MPFC in Early Life Stress-Induced Autistic-Like Behaviors, Front. Cell Dev. Biol., 2020, 8, 564485, DOI:10.3389/fcell.2020.564485
.
- K. Uvnäs-moberg, A. Ekström-bergström, M. Berg, S. Buckley and Z. Pajalic, Maternal Plasma Levels of Oxytocin during Physiological Childbirth – a Systematic Review with Implications for Uterine Contractions and Central Actions of Oxytocin, BMC Pregnancy Childbirth, 2019, 9, 285 CrossRef PubMed
.
- W. Rath, Prevention of Postpartum Haemorrhage with the Oxytocin Analogue Carbetocin, Eur. J. Obstet. Gynecol. Reprod. Biol., 2009, 147, 15–20, DOI:10.1016/j.ejogrb.2009.06.018
.
- A. B. Patel, G. Carroli, B. Fawole, S. S. Goudar, Y. V. Pujar, J. Neilson and G. J. Hofmeyr, Heat-Stable Carbetocin versus Oxytocin to Prevent Hemorrhage after Vaginal Birth, N. Engl. J. Med., 2018, 379, 743–752, DOI:10.1056/NEJMoa1805489
.
- I. Passoni, M. Leonzino, V. Gigliucci, B. Chini and M. Busnelli, Carbetocin Is a Functional Selective Gq Agonist That Does Not Promote Oxytocin Receptor Recycling After Inducing b -Arrestin-Independent Internalisation Neuroendocrinology, J. Neuroendocrinol., 2016, 28(12), 12363, DOI:10.1111/jne.12363
.
-
M. Busnelli and B. Chini, Molecular Basis of Oxytocin Receptor Signalling in the Brain: What We Know and What We Need to Know. In Behavioral Pharmacology of Neuropeptides: Oxytocin, R. Hurlemann and V. Grinevich, ed. Springer, 2018, pp. 3–30 Search PubMed
.
- S. Beychok and E. Breslow, Circular Oxytocin Dichroism * of Oxytocin and Several, J. Biol. Chem., 1968, 243(1), 151–154, DOI:10.1016/S0021-9258(18)99336-1
.
- D. Shugar and J. M. Antosiewicz, UV–Vis Spectroscopy of Tyrosine Side-Groups in Studies of Protein Structure. Part 1: Basic Principles and Properties of Tyrosine Chromophore, Biophys. Rev., 2016, 8, 151–161, DOI:10.1007/s12551-016-0198-6
.
- J. M. Antosiewicz and D. Shugar, UV–Vis Spectroscopy of Tyrosine Side-Groups in Studies of Protein Structure. Part 2: Selected Applications, Biophys. Rev., 2016, 8, 163–177, DOI:10.1007/s12551-016-0197-7
.
- G. Rousseau, T. Kohout, M. Bouvier, M. Azzi and P. G. Charest, Arrestin-Mediated Activation of MAPK by Inverse Agonists Reveals Distinct Active Conformations for G Protein-Coupled Receptors, Proc. Natl. Acad. Sci. U. S. A., 2003, 100(20), 11406–11411, DOI:10.1073/pnas.1936664100
.
- T. Kleszczewski, B. Modzelewska, W. Bal, M. Sipowicz, E. Kleszczewsa and A. Kostrzewska, Cu(II) complexation does not affect oxytocin action on pregnant human myometrium in vitro, Reprod. Toxicol., 2016, 59, 60–65 CrossRef CAS PubMed
.
Footnotes |
† Electronic supplementary information (ESI) available. See DOI: https://doi.org/10.1039/d2cb00225f |
‡ Denotes equal contributions. |
§ Department of Chemistry, University of California, Davis, Davis, CA 95616, USA. |
¶ Department of Chemistry, University of California, Irvine, Irvine, CA 92697, USA. |
|| Department of Chemistry, University of San Francisco, San Francisco, CA 94117, USA. |
|
This journal is © The Royal Society of Chemistry 2023 |
Click here to see how this site uses Cookies. View our privacy policy here.