DOI:
10.1039/D4MA90055C
(Editorial)
Mater. Adv., 2024,
5, 5336-5338
Introduction to hybrid pores for CO2 technologies
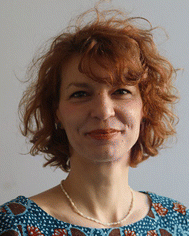
Petra Ágota Szilágyi
| Dr Petra Ágota Szilágyi is a Professor at the University of Oslo (Norway) and a member of the Catalysis Section. She is Fellow of the Royal Society of Chemistry and International Member of its Materials Chemistry Community Council. She received PhD degrees in 2008 in chemistry from the University Eötvös Loránd (Hungary) and in Physics/Nanophysics from the University Paul Sabatier (France). Her research interest is focussed on the green synthesis of metal–organic frameworks, and their design and application for processes of energy and environmental importance. |
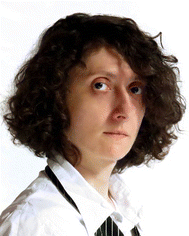
Jenny G. Vitillo
| Jenny G. Vitillo obtained her PhD in Materials Science and Technology from the University of Torino (Italy) in 2005. Following her doctoral studies, she embarked on a journey of academic exploration, holding postdoctoral positions at the University of Torino (2005–2013, 2016–2017), the University of Insubria (2013–2016), and the University of Minnesota (2017–2018). Currently, she serves as an Associate Professor in Physical Chemistry at the University of Insubria, Italy. Her professional pursuits are dedicated to addressing pressing environmental challenges, including CCUS, hydrogen utilization, methane-to-methanol conversion, and light upconversion, by using spectroscopies (IR, UV) and advanced computational methods (DFT, TD-DFT, wave-function-based multireference calculations). |
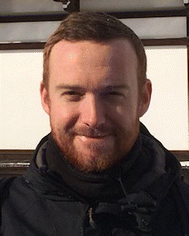
Gavin A. Craig
| Gavin A. Craig obtained his PhD for a thesis on spin crossover materials at the Universitat de Barcelona in 2013, before moving to the University of Glasgow to work as a Post-Doctoral Research Associate studying the effects of pressure on magnetic materials. In 2016 he moved to Prof. Shuhei Furukawa's group in Kyoto University as a JSPS Post-Doctoral fellow, and was promoted to Assistant Professor in 2018. In 2019, he moved to the Department of Pure and Applied Chemistry at the University of Strathclyde, where his research interests are in the design and synthesis of porous cages. |
Rationale for themed issue
Averting a climate catastrophe, the consequence of increasing atmospheric greenhouse gases (GHGs) from anthropogenic sources, has arguably become our most pressing scientific, technological, and socio-economic challenge. Primary among GHGs is CO2, whose management has been proposed to be implemented via either Carbon Capture and Storage (CCS), aiming at long-term storage of CO2 in rocks, for example, or via Carbon Capture and Utilisation (CCU), which capitalises on CO2 as a renewable carbon feedstock for the synthesis of value-added chemicals and fuels, such as methane and methanol.
Porous materials are viewed as key to the development of both approaches, via CO2 capture from flue gases (or more ambitiously directly from the air). Thanks to their tuneable chemistry, inorganic–organic hybrid porous materials such as metal–organic frameworks (MOFs) and metal–organic cages/polyhedra (MOCs/MOPs) have been highlighted as possible solutions not only for CO2 adsorption and separation, but also for its conversion into useful chemicals.
Challenges and recent progress
To employ these materials, however, several challenges need to be overcome. Specifically, challenges persist in simulating open-shell hybrid porous materials, particularly in the context of their composition and structure. Further improvements of both properties and their evaluation are needed to meet the exacting demands for applications. Moreover, for these materials to become commercially viable, synthesis and processing procedures must be advanced.
Modelling
Kohn–Sham density functional theory (KS-DFT) has reached the accuracy to drive experiments and accelerate scientific discovery. An open challenge lies in simulating systems with unpaired electrons because of their multi-configurational nature.1 Open-shell systems are ubiquitous in catalysis, and include transition metal-containing systems, excited states, transition states, etc. The hybrid nature of organic–inorganic compounds makes this challenge even harder, with effects on predicting their photochemical behaviour, such as light absorption and photocatalytic CO2 reduction. Multireference (MR) wave-function-based methods may be required in such cases, but they can be computationally expensive, and they are not user-friendly. Ingham et al. (https://doi.org/10.1039/D3MA00518F) provide a comprehensive overview of the state-of-the-art computational methods for simulating diverse excited states in MOFs, emphasising limitations in molecular, periodic, and embedding approaches. Machine learning (ML) is anticipated to be pivotal in addressing current challenges in modelling excited states. The growing importance of ML for the modelling of CO2 technologies in porous hybrid compounds is also discussed by Yang et al. (https://doi.org/10.1039/D1MA01152A) for adsorbate–adsorbent interactions in MOFs. Their study demonstrates the potential of ab initio (deep) neural network (DNN) force fields for simulating CO2, H2O, and CO in MOFs. DNN models are shown to reduce Monte Carlo simulations’ computational cost by 100 times compared to classical potentials. This work foresees a transition in molecular potentials for gaseous adsorption simulations, impacting various adsorbate and adsorbent systems.
Applications
Currently, potential applications of hybrid porous materials, or indeed the hybrid pores themselves for CO2 technologies, include gas adsorption and separation, addressing both CCU and CCS. CO2 fixation is an inevitable challenge for CCS, and fixation in synthetic materials is an emergent area. Bayati et al. (https://doi.org/10.1039/D3MA00513E) post-synthetically modified MIL-68(In) via cyano-functionalisation of the MOF nodes. The so-obtained materials were not only robust but also displayed appreciable catalytic performances for CO2 cycloaddition into five-membered rings, even without added solvent or co-catalyst.
CCU is based on catalytic CO2 reduction via photo-, (photo)electro-, and thermal catalytic methods. For all purposes ranging from capture, through storage and separation to conversion, pore topology and chemical composition play significant roles. Developing understanding of the structure–composition–property–performance relationships is therefore most pressing. Two approaches of CCU with hybrid pores are exemplified by photocatalytic syngas production, reviewed by Chauhan et al. (https://doi.org/10.1039/D2MA00334A), and direct methanol production, reviewed by Tezel et al. (https://doi.org/10.1039/D3MA00345K), both products of interest being useful platform molecules or synthetic fuel. According to Chauhan et al., Ru(bpy)x moieties may be integrated into MOF structures in various approaches, providing high CO2 to CO conversion and selectivity. On the other hand, Tezel et al. evaluated the thermal catalytic performance of various metal nanoparticles embedded into Zr6-MOF frameworks, pointing out the importance of the MOF defect structure and a lack of shared best practices across the community.
Synthesis and processing
This themed issue highlights three key challenges for the fabrication and performance development of porous materials used in CO2 capture. The first is how materials are processed beyond crystalline phases while retaining their performance. J. Martí-Rujas (https://doi.org/10.1039/D3MA00477E) provides a perspective on a molecular approach through the chemical inter-linking of MOCs/MOPs to create soft matter. MOPs present intrinsic porosity arising from the molecular unit itself, as well as extrinsic porosity originating in the spatial organisation of the MOPs – here, J. Martí-Rujas describes how linking the cages with chemical bonds impacts CO2 capture.
The second challenge is how cutting-edge characterisation techniques are used to understand more fully the structure and adsorption processes of complex porous materials. In an original research paper, Crocellà, Taddei and co-workers (https://doi.org/10.1039/D3MA00356F) describe the application of in situ measurements to elucidate phase transitions in a copper ethylenediphosphonate framework. The work demonstrates the importance of open metal sites generated on Cu(II) ions for the capture of CO2. Beyond this, the in situ spectroscopic data show that changes in coordination geometry at the metal site drive the phase transitions, rather than ligand flexibility.
Thirdly, A. Laybourn and co-workers (https://doi.org/10.1039/D3MA00351E) address the regeneration of materials after gas capture to enable their repeated use. They describe a hybrid material consisting of the MOF UTSA-16(Zn) and magnetite nanoparticles. The incorporation of magnetite enables the application of a magnetic field to produce a heating effect in the material, driving thermal regeneration of the framework.
Future perspectives
Theoretical approaches must address the need for faster scaling methods to handle large models, like those necessary to investigate gate-opening,2 materials composites, or membranes. Efficient screening of large sets is also highly desirable. As shown in this issue, machine learning is expected to be pivotal in future developments of CCUS modelling.
Challenges in the application of hybrid pores may only be overcome when performance testing of this immensely rich class of materials follows best practices for comparability of handling and testing conditions. In the case of MOFs, the evaluation of defects must become standard procedure to establish structure–property–function relationships. Such investigations are key to developing fundamental understanding, enabling rational design and, ultimately, materials optimisation.
References
- J. G. Vitillo,
et al., Multireference Methods are Realistic and Useful Tools for Modeling Catalysis, Isr. J. Chem., 2022, 62(1–2), e202100136 CrossRef CAS
.
- V. Van Speybroeck,
et al., Towards modeling spatiotemporal processes in metal-organic frameworks, Trends Chem., 2021, 3(8), 605–619 CrossRef
.
|
This journal is © The Royal Society of Chemistry 2024 |