DOI:
10.1039/D3NA00342F
(Review Article)
Nanoscale Adv., 2023,
5, 5705-5716
Post COVID-19 complications and follow up biomarkers
Received
19th May 2023
, Accepted 11th September 2023
First published on 15th September 2023
Abstract
Millions of people were infected by the coronavirus disease (COVID-19) epidemic, which left a huge burden on the care of post COVID-19 survivors around the globe. The self-reported COVID-19 symptoms were experienced by an estimated 1.3 million people in the United Kingdom (2% of the population), and these symptoms persisted for about 4 weeks from the beginning of the infection. The symptoms most frequently reported were exhaustion, shortness of breath, muscular discomfort, joint pain, headache, cough, chest pain, cognitive impairment, memory loss, anxiety, sleep difficulties, diarrhea, and a decreased sense of smell and taste in post-COVID-19 affected people. The post COVID-19 complications were frequently related to the respiratory, cardiac, nervous, psychological and musculoskeletal systems. The lungs, liver, kidneys, heart, brain and other organs had been impaired by hypoxia and inflammation in post COVID-19 individuals. The upregulation of substance “P” (SP) and various cytokines such as tumor necrosis factor-alpha (TNF-α), interleukin 6 (IL-6), interleukin 10 (IL-10), interleukin 1 beta (IL-1β), angiotensin-converting enzyme 2 (ACE2) and chemokine C–C motif ligand 3 (CCL3) has muddled respiratory, cardiac, neuropsychiatric, dermatological, endocrine, musculoskeletal, gastrointestinal, renal and genitourinary complications in post COVID-19 people. To prevent these complications from worsening, it was therefore important to study how these biomarkers were upregulated and block their receptors.
1. Introduction and background
The COVID-19 viral infection was first reported in Wuhan, China, in December 2019 and its prevalence caused respiratory distress syndrome worldwide.1–3 It was estimated that 5 million people were facing COVID-19 across the globe.4 It was reported on March 5, 2022, that there were 446
511
318 cases globally recorded with 6
004
421 deaths (WHO, 2022).5 In Pakistan, there were 1
516
150 cases reported and the number of deaths increased to 30
287 (NHSRC, 2022).6 The COVID-19 pandemic infected millions of people around the world, putting a financial burden on the treatment of COVID-19 survivors. An estimated 1.3 million people living in their houses in the United Kingdom, which was 2% of the population, experienced self-reported COVID-19 symptoms and these symptoms persisted for ≥4 weeks after the onset of the COVID-19 infection.7
According to the World Health Organization (WHO), post COVID-19 is defined as symptoms and complications that appeared after COVID-19 recovery, continued for ≥12 weeks and had a worsening impact on the patients' health and quality of life. The most common symptoms are fatigue, shortness of breath and neural dysfunction that may worsen over time.8
The post COVID-19 survivors continued to develop physical, mental and psychological symptoms and complications. It was reported that fatigue, shortness of breath (SOB), myalgia, arthralgia, headache, cough, chest pain, anxiety, memory loss and sleep disorders were present in post COVID-19 people. Post-COVID-19 individuals experienced impaired quality of life, and mental health and employment issues.9
Currently, there is limited literature available that reports possible risk factors, pathophysiology and biomarker expression in post COVID-19. This review focuses on the post COVID-19 symptoms, biomarkers, complications of respiratory, cardiovascular, hematological, neuropsychiatric, dermatological, diabetes mellitus, renal, gastrointestinal and genitourinary diseases. Moreover, we will discuss potential biomarkers that may escalate health impairments in COVID-19 (Fig. 1).
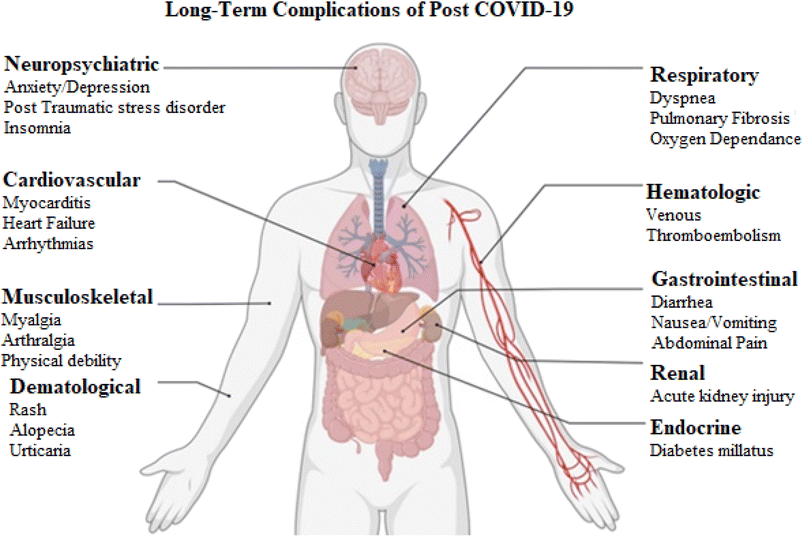 |
| Fig. 1 Schematic representation of COVID-19 terms sequelae observed following the COVID-19 infection. This figure has been reproduced from ref. 30 with permission from American Journal of Physiology-Cell Physiology, copyright 2021. | |
2. Post COVID-19 symptoms
Post COVID-19 caused systemic inflammation and hypoxia which could damage the kidneys, liver, heart, brain and other body organs.10,11 It was reported that dyspnea and reduced exercise tolerance developed in 10–40% of the hospitalized patients within 2–4 months and shortness of breath developed in 65% of patients after discharge from the intensive care unit (ICU). It was documented that 10–35% of COVID-19 patients did not require hospitalization after post-COVID-19 symptoms.12
Hannah et al. (2020) studied responses from 3762 participants with confirmed COVID-19 from 56 countries and the duration of COVID-19 was at least 28 days. It was reported that 96% of patients experienced the symptoms of fatigue, dry cough, shortness of breath, headaches, muscle ache, chest tightness and sore throat apart from the loss of taste and smell beyond 90 days. The post COVID-19 symptoms that continued after 6 months were fatigue, post-exertional malaise and cognitive dysfunction. Most of the people recovered approximately after seven months from COVID-19, but not all the symptoms were relieved.13 The common clinical systemic complications of post COVID-19 were interstitial lung disease (organized pneumonia and pulmonary fibrosis), pulmonary embolism, chronic cough, cavity lesions, lower airway pulmonary hypertension lung fibrosis, arterial thrombosis, venous thromboembolism, cardiac thrombosis, strokes, inflammation, dermatological complications and overall neuropsychiatric dysfunctions14 A meta-analysis of studies involving n = 17
794 patients revealed that troponin and aspartate levels increased with the worsening of COVID-19 conditions. Furthermore, this study described that comorbidities of cerebrovascular diseases, chronic heart diseases, obstructive pulmonary disease, hypertension, chronic kidney disease, metastatic neoplasm and diabetes mellitus increased the risk of mortality and severity in post COVID-19 patients.15
Post COVID-19 affected survivors at all levels of age including adults, children and those who were not hospitalized, but possible risk factors were reported including female sex, early dyspnea and prior psychiatric disorders.28 Older age, severe pneumonia, long ICU duration, mechanical ventilation duration, smoking and chronic alcoholism history were predictors for lung fibrosis development.29 These symptoms may be controlled by overcoming blockage in the expression pathways of systemic biomarkers which contributed to escalate post COVID-19 worsening.
3. Post COVID-19 pathological complications
3.1 Respiratory complications
The most common pulmonary symptom reported following COVID-19 is dyspnea which persisted in 22.9 to 53% of patients 2 months after COVID-19.31,32. One of the most serious complications of the post-acute period is the development of pulmonary fibrosis.33 The post-COVID-19 survivors have the potential to develop coughs or shortness of breath and if symptoms worsen, they can develop extensive fibrosis of the lungs.34 Oxygen dependency was reported in up to 6.6% of survivors. Diffuse alveolar damage, cytokines recruiting lymphocytes, macrophages and neutrophils which recruit fibroblasts result in pulmonary fibrosis.35 Therefore, the risk of developing fibrotic lung changes increased in post COVID-19 survivors.36 After COVID-19 recovery, most of the lungs would heal without complications but minimal fibrosis could develop which can progress to an advanced phase of fibrosis.37
In our opinion, strategies should be developed to control the development of pulmonary fibrosis by looking at which main inflammatory agents contributed to the production of cytokine storms at the alveolar level in order to speed up the healing process and get rid of pulmonary fibrosis (Table 1).
Table 1 Clinical symptoms of COVID-19 survivors
Symptoms |
% Of symptoms observed |
Ref. |
16
|
17
|
18
|
19
|
20
|
21
|
22
|
23
|
24
|
25
|
26
|
27
|
Fatigue |
28 |
53 |
52 |
— |
39 |
— |
98 |
63 |
— |
— |
— |
31 |
Fever |
— |
— |
— |
— |
— |
— |
75 |
— |
— |
— |
— |
— |
Diaphoresis |
24 |
— |
— |
— |
— |
— |
— |
— |
— |
— |
— |
— |
Post-activity tachypnea |
21 |
— |
— |
— |
— |
— |
— |
— |
— |
— |
— |
— |
Palpitation |
— |
— |
— |
11 |
— |
— |
— |
11 |
— |
— |
19 |
— |
Chest pain |
12 |
22 |
— |
13 |
— |
5 |
73 |
— |
— |
— |
10 |
— |
Psychosocial distress |
23 |
— |
— |
— |
— |
17 |
— |
— |
— |
39 |
— |
— |
Anxiety |
7 |
— |
— |
— |
— |
— |
— |
7 |
— |
30 |
16 |
— |
Depression |
— |
— |
— |
— |
— |
— |
— |
23 |
— |
33 |
— |
— |
Malaise |
— |
— |
— |
— |
— |
— |
— |
— |
— |
— |
— |
33 |
Concentration impairment |
— |
— |
— |
— |
— |
— |
— |
— |
— |
— |
— |
26 |
Headache |
— |
— |
— |
— |
— |
— |
83 |
— |
6 |
— |
— |
— |
Weakness |
— |
— |
— |
— |
— |
— |
— |
— |
— |
— |
— |
41 |
Myalgia |
— |
— |
— |
— |
22 |
6 |
88 |
— |
9 |
— |
— |
— |
Arthralgia |
8 |
27 |
— |
16 |
— |
6 |
78 |
9 |
9 |
— |
— |
— |
Inability to walk |
— |
— |
— |
— |
— |
— |
40 |
— |
— |
— |
— |
— |
Cough |
7 |
— |
— |
— |
11 |
— |
73 |
7 |
6 |
— |
— |
— |
Dyspnea |
— |
43 |
— |
8 |
39 |
6 |
87 |
26 |
15 |
— |
— |
25 |
Wheezing |
— |
— |
— |
— |
— |
— |
48 |
— |
— |
— |
— |
— |
Abdominal pain |
— |
— |
— |
— |
5 |
— |
— |
— |
— |
— |
— |
— |
Diarrhea |
— |
— |
— |
— |
— |
59 |
— |
— |
— |
— |
— |
Anorexia |
— |
— |
— |
— |
— |
— |
— |
8 |
— |
— |
— |
— |
Rhinorrhea |
— |
— |
— |
— |
— |
— |
34 |
— |
— |
— |
— |
— |
Ageusia |
— |
— |
— |
— |
— |
— |
— |
7 |
10 |
— |
— |
— |
Sore throat |
— |
— |
— |
— |
— |
— |
71 |
— |
— |
— |
— |
— |
Anosmia |
— |
— |
— |
— |
11 |
5 |
— |
26 |
12 |
— |
— |
— |
Sleep disorders |
17 |
— |
— |
23 |
24 |
— |
— |
— |
— |
— |
17 |
— |
Dizziness |
— |
— |
— |
— |
— |
— |
— |
6 |
5 |
— |
— |
— |
Hair loss |
29 |
|
|
— |
— |
— |
— |
22 |
— |
— |
24 |
— |
Reduced quality of life |
— |
— |
— |
— |
— |
— |
— |
— |
— |
— |
— |
26 |
Number of symptoms observed |
10/30 |
4/30 |
1/30 |
5/30 |
7/30 |
6/30 |
13/30 |
12/30 |
8/30 |
3/30 |
5/30 |
6/30 |
3.2 Cardiovascular complications
The COVID-19 infection caused systematic inflammation, which further caused cardiovascular complications, morbidity and mortality. It was reported that troponin levels increased by 7–17% with the severity of the COVID-19 infection.38 Chest pain was reported in 21% of patients 60 days after discharge from the hospital. The susceptibility to cardiovascular complications such as ischemic and non-ischemic heart disease, cerebrovascular disorders, pericarditis, heart failure, myocarditis, shocks, dysrhythmia and thromboembolic complications increased.39,40 The most serious cardiovascular complication in COVID-19 patients was myocarditis, and deaths were reported in 7% of the patients with myocarditis.41 Eman and Toraih (2020) concluded that an increase in cardiac enzymes could be used as a biomarker for the identification of those patients who are at a higher risk of suffering from post-COVID-19 complications.15
It seems that post-COVID-19 cardiovascular complications occurred due to inflammatory changes caused by the production of cytokines and substance P. So, it may be possible to use systemic anti-inflammatory drugs to defeat the causative agents.
3.3 Hematological complications
It was reported that COVID-19 also invaded vascular endothelial cells and increases pro-inflammatory cytokine release and vascular endothelial injury which resulted in impaired fibrinolysis, thrombosis and hematological complications.42 Endothelial pathway activation increased the risk of thrombotic events in post COVID-19 survivors. The microvascular dysfunction enhanced the expression of tissue factors in response to the inflammatory cytokines as well as the effects of hypoxia that contributed to hematological complications.43,44 So, this revealed to us that certain cytokines caused vascular endothelial injuries which may be reduced by inhibiting the production of cytokines by using medicines.
3.4 Neuropsychiatric complications
COVID-19 infections invade the neural system and are involved in hypoxic brain injury, metabolic imbalances and oxidative stress which lead to worse neurogenic symptoms or complications in survivors.45 The two-month monitoring revealed that loss of smell was 13.11% and taste was 11% post-traumatic stress disorder 28%, depression 31%, anxiety 42% and insomnia 40% in post COVID-19. Sun et al. (2021) reported that plasma cytokines IL-4 and IL-6 increased in post COVID-19 recovering individuals from 1 to 3 months. The peripheral and neuro-inflammation in post COVID-19 infection increased neurological complications. Individuals recovering from COVID-19 experienced concealed neural and neurological symptoms.46 It was reported that depression was 31%, anxiety was 42%, obsessive compulsive disorders were 20% and insomnia was 40%. A record of n = 402 adults with psychiatric symptoms after surviving COVID-19 showed that increased inflammation was related to worsening depression in COVID-19 survivors, and inflammation based on peripheral lymphocytes, neutrophils and platelets can be used as biomarkers for psychiatric disorders.47
Over 30% of post COVID-19 survivors complained of altered memory status.48 The neurological symptoms such as headache, fatigue, brain fog, dizziness, memory loss, confusion, dysautonomia and difficulty focusing, are associated with post COVID-19 infections.49–51 Therefore, early screening and detailed rehabilitation strategies may be necessary to prevent and manage post-COVID-19 complications and their worsening.
3.5 Dermatological complications
The literature reported that dermatological complications developed in COVID-19 due to deposition in dermal capillaries or interstitials.52 Dermal complications such as maculopapular rash, vesicular rash microvascular vasculitis, urticaria, chilblain and petechiae are associated with COVID-19.53,54 A meta-analysis reported that the most common complication reported was maculopapular exanthema in 36.1% of patients with COVID-19. The papulovesicular rash (34.7%), painful red acral purple papules (15.3%) and urticaria (9.7%) were reported in the hands and feet in post-COVID-19 patients.55 It seems that there are certain other biological components which are related to post-COVID-19 and worsening of dermatology. So we should look further to see which more components are involved and deposited in dermal capillaries and interstitials to intensify complications. Proper medications should be taken to cure these dermatological ailments.
3.6 Diabetes mellitus complications
COVID-19 causes increased oxidative stress and the release of pro-inflammatory cytokines in diabetic patients as compared to healthy individuals. COVID-19 damaged β cells and is related to an insulin deficiency which led to hyperglycemia.56 The post COVID-19 patients with diabetes mellitus types I and II developed hyperglycemia, loss of acute compensation for diabetes and diabetic ketoacidosis.57 The data showed that the prevalence of diabetes was 15.4% in post COVID-19 patients. Therefore, it seems that oxidative stress and cytokine production after post COVID-19 and other complex interrelated processes contributed to hyperglycemia and had an impact on β Langerhans cells and the appearance of glucose uptake receptors on the cell surface.58,59 Psychotherapy and medicines that may minimize the secretions of cytokines may be helpful.
3.7 Renal complications
The renal function was reported to be abnormal in 35% of patients at the time of discharge from hospitals, and in those patients 30% required dialysis. During follow-up in the same study, 36% of patients with residual kidney disease had recovered at the time of discharge but 14% of those who had recovered before discharge had recurrent kidney disease. Direct COVID-19 viral damage, systemic hypoxia, abnormal coagulation and effects of inflammatory cytokines were the contributing factors to acute kidney injury.60,61 Renal abnormalities occurred in most patients with COVID-19 pneumonia. Renal complications in COVID-19 were associated with higher mortality.62 Kidney dialysis is required in this situation.
3.8 Gastrointestinal complications
Diarrhea was among the top 10 most common symptoms and its prevalence was reported to be 6%. Other COVID-19 symptoms were nausea, vomiting, abdominal pain and loss of appetite. In post COVID-19 patients with acute liver injury, abnormal liver function may persist but improve over time.63 Assessment of dietary intake and personalized dietary recommendations are the best strategies to recover.
3.9 Musculoskeletal complications
Arthritis was associated with some kind of viral infection. COVID-19 caused myalgia and arthralgia without true inflammatory arthritis in most patients. Post COVID-19 was less related to rheumatological diseases in patients.64,65 Fatigue, myalgia and arthralgia were most commonly reported in post COVID-19 survivors.66,67 It was documented that musculoskeletal complications arise due to high pro-inflammatory cytokines that are related to post COVID-19.68,69 Elevated levels of the pro-inflammatory factor interleukin-6 may be related to myalgia and joint pain.70 Drugs such as siltuximab, sarilumab, and tocilizumab which have antiinflammatory effects, and inhibit the production of IL-6, may be used on the prescription of a doctor for recovery in post COVID-19 patients.
3.10 Genitourinary complications
It was shown by the literature survey that there was a significant reduction in dihydrotestosterone, testosterone, and orchitis in several patients. Viral testicular inflammation was reported in up to 19% of patients. In female patients, luteinizing hormone levels were elevated and preterm delivery took place with no vertical transmission.71 COVID-19 is associated with severe physiological changes in the testes and impairment of spermatogenesis, hormonal imbalances secondary to hypogonadism and sexual dysfunction.72 Hypogonadism correlates with unregulated pro-inflammatory cytokines TNF-α, IL-1β and IL-6.73 A recent study found that 30% of COVID-19 patients still had persistent symptoms or complications after nine months and the majority of non-hospitalized patients (85%) had mild symptoms.74 So, it seemed that production of TNF-α, IL-1β and IL-6 resulted in increased post genitourinary complications and may be stopped by blocking the pathways that cause the production of these inflammatory components. Psychological support and proper nutrition, including micro and macronutrients, are required for recovery, which may upgrade the sexual functioning of patients.
4. Biomarkers of COVID-19
Humans have approximately 20
000–25
000 protein-encoding genes.75 There could be over 1
000
000 proteoforms including splice variants and essential post-translational modifications.76 There were 29 predicted proteins in the COVID-19 virus with four main structural proteins (s, e, m, and n).77 The post translation proteins (PTMs) could provide additional signatures to detect COVID-19 and its effects. The consideration of PTMs such as glycosylation and lipidation could provide more opportunities for the detection of viral proteins. A concentration on COVID-19 protein-derived glyco-conjugate peptides may enable the specificity and sensitivity of detection of the viral protein to be improved.78
Early biomarker identification improved the management of COVID-19 patients and the appropriate allocation of healthcare resources during the pandemic. Anne et al. (2020) systematically reviewed routine laboratory tests of 45 studies for severe COVID-19 disease and described that an increased level of lymphocytes, neutrophil count, C reactive protein (CRP), lactate dehydrogenase, D-dimer, and aspartate aminotransferase and a low level of hemoglobin was observed in severe COVID-19 patients.79 A significant association was presented between lymphopenia, thrombocytopenia, and elevated levels of procalcitonin, CRP, lactate dehydrogenase (LDH), and D-dimer and COVID-19 severity.80 Protein markers of neuronal dysfunction such as amyloid beta, neurofilament light, neurogranin, total tau and p-T181-tau increased in COVID-19 compared to historic controls.81 Raymond's (2020) meta-analysis on n = 967 patients from six studies concluded that N terminal pro B type natriuretic peptide (NTP-BNP) was associated with increased mortality in COVID-19.82 Li et al. (2020) systematically reviewed 4189 patients with COVID-19 from 28 studies, which revealed that higher troponin, creatinine kinase myoglobin binding myoglobin and NT-BNP were associated with higher mortality in COVID-19.83 NTP-BNP can be used as a biomarker in patients without chronic heart failure, but has a poor prognosis in patients with sepsis and acute respiratory distress syndrome (ARDS).84
Jing et al. (2020) conducted a systematic review regarding the blood coagulation biomarkers in patients with severe COVID-19 by searching databases from PubMed, Embase, Web of Science and the Cochrane Library. A record of 13 studies with n = 1341 adult patients showed that low platelets, higher D dimers and higher fibrinogen were associated with severity in patients with COVID-19. No correlation was manifested between activated partial thromboplastin time (APTT) or prothrombin time (PT) and the severity of COVID-19.85 Jiaxi Xu and Eric Lazartigues (2022) studied ACE2 in COVID-19 patients with severe neurological symptoms and the possibility that COVID-19 can infect and injure the central nervous system in humans. It was determined that the expression of ACE2 in human neurons is related to COVID-19 by using human pluripotent stem cell-derived neurons and the possibility of a patient's ability to respire and worsen respiratory failure.86 Interleukin 4 (IL-4) is a cytokine that is a potent regulator of immunity and is secreted primarily by mast cells, T helper 2 cells (Th2), eosinophils and basophils. Interleukin 4 (IL-4) is related to abnormal learning, memory, neurogenesis and neurological disorders. Sachin (2012) conducted extensive research on the role of IL-4 in immunity. The study evidence indicated that it has a critical role in the central nervous system (CNS) for memory and learning functions.87
The data suggested that a systematic and longitudinal assessment of cytokines is required for the COVID-19 disease. A cytokine storm profile associated with severe COVID-19 disease was characterized by increased interleukin 2 (IL-2), interleukin 7 (IL-7), granulocyte-colony stimulating factor, interferon-γ inducible protein 10, monocyte chemoattractant protein 1, macrophage inflammatory protein 1 alpha and tumor necrosis factor alpha.88 Elevated concentrations of immunological interleukin markers were consistent with the “cytokine storm” hypothesis, whereas elevated concentrations of D dimer were related to inappropriate blood coagulation as a possible contributor to pathogenesis.89 Wenjie et al. (2020) reviewed published articles according to preferred reporting items for systematic reviews and meta-analyses (PRISMA) guidelines and evaluated the risk factors associated with mortality in COVID-19. Data (n = 4659) retrieved from 14 studies showed that hypertension, coronary heart disease and diabetes were likely more associated with mortality in COVID-19 patients. The greater levels of troponin, CRP, Interleukin-6 (IL-6), D dimer, creatinine and alanine transaminase and low level of albumin were presented in the mortality group. The increased acute response was related to cardiac inflammation and renal, liver and hematologic organ damage in COVID-19.90 Yujun et al. (2020) provided evidence that interleukin 6 (IL-6) induced the exhaustion of lymphocytes in COVID-19 and that pro-inflammatory IL-6 levels increased in severely ill COVID-19 patients as compared to moderately ill patients.91 Sun et al. (2021) studied neurological sequelae in COVID-19 from 1 to 3 months and found that cytokines (IL-4 and IL-6), antibody levels and neuronal enriched extracellular vesicle protein cargo were increased in COVID-19 patients.81 Piotr Lorkiewicz and Napoleon Waszkiewicz (2021) searched PubMed, Scopus and Google Scholar scientific literature databases to evaluate the development of post-COVID depression. They found that increased levels of IL-6, interleukin 1 β (IL-1β), tumor necrosis factor alpha (TNF-α), interferon-gamma (IFN-γ), interleukin 10 (IL-10), interleukin 2 (IL-2), soluble interleukin 2 receptor (SIL-2R), CRP, monocyte chemoattractant protein 1 (MCP-1), and serum amyloid A1 (SAA1), and a declining level of brain derived neurotrophic factor (BDNF) and tryptophan were associated with the development of depression in post-COVID-19.92
The substance “P” (SP) is a neuropeptide related to the tachykinin peptide family. The SP primary structure consists of 11 amino acids (RPKPQQFFGLM) that have a C terminal α amidated methionine residue. SP is released in the central and peripheral nervous systems and distributed in the human brain (Kast et al., 2015).93 Neurokinin 1 is a receptor for SP which is distributed in the gastrointestinal tract, lung, urinary tract, muscles, peripheral tissues, immune cells and thyroid in mammalians.94,95 SP is involved in the regulation of the cardiovascular system, dilatation of the vascular system, neural response, gastric motility, inflammation, pain, depression and respiratory mechanisms.96–98 SP is released from nerves, macrophages, eosinophils, lymphocytes and dendrites, and binds to NK1. The mast cells produced histamines, chemokines and cytokines in response to the SP stimulus. SP inhibited transforming growth factor beta 1 (TGF-β1), released macrophages and acted as an immune suppressor. Like interleukin 4, interferon gamma (IFN-ϒ) and TNF-α activated upregulation of SP-NK1.99–102 An elevated level of SP is involved in inflammation of the respiratory, gastrointestinal and musculoskeletal systems.103 SP acts as a neuromodulator functioning in an autocrine, endocrine, and paracrine manner. It is also released from non-neural cells like immune cells.104 SP is associated with inflammation in asthma and chronic obstructive lung diseases. Viral infections like respiratory syncytial virus (RSV) caused upregulation of SP expression and were linked with inflammation in rat lungs.105
The COVID-19 virus stimulates the trigeminal ganglion (TG) via trigeminal nerves. The trigeminal nerve has three branches named ophthalmic, maxillary and mandibular nerves and noxious stimulus-activated nociceptor afferents that have cell bodies present in the TG. As a result of nerve injury, neuropeptides such as SP are secreted which are involved in inflammation (Goto et al., 2017).106
Upregulation of SP impairment of mucociliary transport helps to secrete mucus or secretion from the airway. Nuclear factor kappa B (NF-kB) pathways were reported to be involved in the SP overexpression in airways causing inflammation and inhibition of Interlukin10 (Sponchiado et al., 2020).107 Cytokine storms were aroused in the COVID-19 infection and contributed to airway inflammation (Riffat, 2021).108
The cytokine storm syndrome is reported in critically ill COVID-19 patients and presents with high inflammatory mediators, systemic inflammation and multiple organ injury or failure.143 Pro-inflammatory cytokines such as TNF-α, IL-6, IL-10, IL-1β and chemokines increase in COVID-19 patients.144 There are certain biomarkers such as TNF-α, IL-6, IL-10, IL-1β chemokines (CCL2 and CCR2) and neuropeptides (substance P) which are produced systemically in post COVID-19 patients and are responsible for deregulation of organ functions (Table 2).
Table 2 Predictive immune biomarkers of COVID-19
Type |
Family |
Biomarkers |
Amino acid |
Ref. |
Tachykinin peptides |
Neuropeptide family |
SP |
11 |
109 and 110 |
Cytokines111 |
Interleukin family |
IL-6 |
212 |
112 and 113 |
IL-7 |
177 |
114
|
1L-1β |
269 |
115
|
1L-18 |
193 |
114
|
TNF-α |
269 |
116 and 117 |
Interferon family |
IFN-I |
166 |
118
|
Chemokine family |
CCR2 |
374 |
119
|
MCP1/CCL2 |
25 |
120–122
|
Anaphylatoxins |
Glycoproteins |
C3a, C5a |
77, 74 |
123 and 124 |
Metalloenzymes |
Zinc metalloenzyme |
ACE2 |
805 |
86, 125–127 |
Mucine |
Glycosylated proteins |
MUC-1 & SAC |
72 |
128
|
Brain natriuretic peptide |
N-terminal prohormone of brain natriuretic peptide |
NT-proBNP |
76 |
82
|
Protein |
Apolipoproteins family |
SAA1 |
122 |
129 and 130 |
Pentameric protein |
CRP |
187 |
131 and 132 |
Cells |
— |
T cells |
133–137
|
NK cells |
138 and 139 |
Eosinophils, basophils, and mast cell |
140 and 141 |
Immunoglobulin protein |
Antibodies |
Anti-SARS-CoV-2 N/SIgM/IgG ab |
46 and 142 |
Substance P (SP) expression increased with the onset of viral infections. COVID-19 caused the induction of SP which has a counterpart in neurogenic inflammation in the lung. Stress-induced effects on intracerebral SP levels were mainly obtained from brain tissue measurements. Substance P counterparts escalate the cytokine storm in COVID-19 patients. Therefore, it may be a good biomarker to follow up with post COVID-19 patients.
The minimum detectable limit of detection for TNF-α is 10 pg mL−1. TNF-α levels were higher in the SARS-CoV-2 (+) symptomatic group (16 pg mL−1) compared with the SARS-CoV-2 (−) symptomatic individuals (7.6 pg mL−1). TNF- α and IL-6 are counterparts of the cytokine storm.145 Since previous findings suggested the involvement of TNF-α-induced inflammation and the manifestation of long term COVID symptoms,146 as a biomarker for the prognosis of severe COVID-19, targeting TNF-α using antibodies or inhibitors is a good biomarker.
The lower limit for the detection of IL-6 is 1.5 pg mL−1, whereas the upper limit for detection is 5000 pg mL−1 without any prior dilution.147 But it may vary due to different ELISA kits. Some studies reported that it may be increased in sepsis (>1000 pg mL−1 at COVID-19 onset).148 In addition, patients with such complicated forms of COVID-19 had nearly threefold higher serum IL-6 levels than those with non-complicated disease.149 IL-6 is a suitable biomarker to follow up with post COVID-19 patients. Monoclonal antibodies may be effective in reducing IL 6 expression in COVID 19.150
COVID-19 patients had higher IL-18 levels compared to healthy subjects (103 [210] pg mL−1vs. 310 [502] pg mL−1, p = 0.006). Levels of circulating IL-18 were considered increased in different diseases in relation to the disease characteristics and severity.151 High levels of IL-18 have been found in the blood of patients with many different types of allergic diseases. Serum IL-18 concentrations have a significant correlation with COVID-19 severity.152
In normal healthy adults, an IL-1B concentration in the serum ranges from 0.5 to 12 pg mL−1. Interleukin-1β (IL-1β) is a member of the interleukin 1 family of cytokines that are instrumental in inflammatory responses. IL-1β is an important, robust pro-inflammatory cytokine involved in the body's immune response against infection and injury. In the cytokine storm, the levels of several cytokines such as IL-1β, IL-10 and TNF-α increased which correlate with disease severity.153 But the range of minimum and maximum values was also narrower on days 3 and 6 than on day 0. Studies on longitudinal cytokine profiles in patients across all severities of COVID-19 are still limited.154
IFN-I has important roles in protecting the lungs from the spread of respiratory viruses and reducing inflammation in the lung. Integrated immune analysis, including immune cell analysis, whole-blood transcriptomics and cytokine quantification in COVID-19 patients impaired the IFN-I response as a result of low IFN-I levels. The assay range is approximately 0.4–298 pg mL−1. The reference range for a healthy population is less than 2.0.155
Chemokine receptor CCR2 expression causes high monocyte numbers, which contribute to inflammation. During COVID-19 infection, CCR2 stimulates monocytes to enter the lung parenchyma and causes monocyte driven cytokine storm inflammation.156 The critical point in this therapeutic approach is to pay attention to other mechanisms involved in migration of monocytes and macrophages to the lung and which on worsening, cause fibrotic changes.
Monocyte chemoattractant protein-1 (MCP-1/CCL2) is one of the key chemokines that regulate the migration and infiltration of monocytes/macrophages.157 The circulating levels of CCL2 were nearly doubled (264 vs. 134 pg mL−1) in the severe group compared to the non-severe group of COVID-19.158
Airway mucus from patients with COVID-19 had a higher level of MUC5AC, which may be detected only when secretions are taken by bronchoscopy.128 Serum amyloid A (SAA) is the most prominent acute phase reactant as its serum levels in the acute phase response demonstrate the most notable increase. In healthy individuals, SAA is present at a blood concentration below 3 mg L−1. Its level rises during the COVID-19 infection.159 There is less published data on SAA as a biomarker of COVID-19 severity, and future longitudinal studies should be conducted to assess the clinical relevance of SAA with the severity of COVID-19 and follow-up biomarkers.
CRP might be related to the production of inflammatory cytokines, which fight against microbes, but when the immune system becomes hyperactive, it can damage lung tissue.160 CRP is a valuable early marker but CRP levels in patients with COVID-19 who may progress from non-severe to severe cases need to be further studied in large-scale multicenter studies.
Basophils play an important role in promoting antibody response and mast cells may take part in the pro-coagulative status typical of COVID-19 patients. The limitations of this biomarker are the number and timing of serum sampling that affect the results in long COVID-19.161
The detection limitations of biomarkers for COVID-19 are a BSL-3 laboratory, well trained technicians, state of the art calibrated instruments, and high quality standardized kits that have high sensitivity and specificity that may detect threshold value for a biomarker.162 In the longitudinal studies, participants should be involved in the study from the very beginning to the end with their consent for COVID-19 biomarkers. Phlebotomists should be well trained for sample drawing and samples should be handled and stored properly and carefully as prescribed by the kit manufacturer's protocol.163 Some patients who are PCR negative for COVID-19 have biomarkers for COVID-19. These results are false positives and they should be reported clinically in order to retain validity of data. Standardized techniques should be used for biomarker detection. The balance between assay sensitivity and specificity must be weighed to reduce the risk of false positives. The limitations of biomarkers are number and timing of serum sampling that affect result in long COVID-19. The assay should be performed within the given time limit mentioned in kit protocol.164 The aforementioned conditions should be fulfilled properly to avoid detect limitations of biomarkers for COVID-19. These may be possible detection limitations for COVID-19 biomarkers and need further to be explored.
5. Outlook
Although great progress has been made in post COVID-19 complications and biomarkers, there are still several problems to be addressed. The expression pathways for developing complications with respect to biomarkers should be investigated by using inhibitors to reduce and stop the cytokine storm in post-COVID-19 patients. A release of the substance P counterpart for worsening symptoms and complications in post-COVID-19 patients should be sorted out to lessen and cure COVID-19. It is suggested that patients with COVID-19 who experience symptoms should manage by blocking mast cells and their mediators, which could be useful for cure and needed to be investigated. Currently, the major constraint to controlling the symptoms and complications of COVID-19 patients is a lack of drugs that inhibit the production pathways for biomarkers. Neurological and musculoskeletal complications remained for long periods, and other complications were refractory. Drugs should be developed so that the care of these patients can be optimized to cure COVID-19 patients and give them a better, healthier life. Finally, expression pathways of biomarkers related to post-COIVD-19 complications have not been investigated. We must take more serious steps for patients and be more concerned about limiting the expression pathways for the generation of these biomarkers, and there should be nationwide surveillance programmes to improve patients' health and quality of life.
6. Conclusion
Patients' education will be useful in identifying the post COVID-19 syndrome, which is defined by lingering symptoms and complications that occur after 4 weeks. After COVID-19, the expression of SP and several cytokines (TNFα, IL-6, IL-10, IL-1, ACE2 and CCL3) result in complications involving the respiratory system, heart, brain, endocrine system, skin, musculoskeletal system, gastrointestinal tract, kidneys and genitourinary system. Pulmonary fibrosis development is the worst complication because it affects the quality of life. We should rule out and block the SP expression pathway as well as that of other cytokines, which will help to overcome post COVID-19 complications.
Conflicts of interest
There are no conflicts to declare.
Acknowledgements
The Unveiling and Leading Project of XZHMU under (Grant no. JBGS202204) and also Xiao Zhang was supported by the National Key Research and Development Programme of China (No. 2020YFB1711500) and the Deanship of Scientific Research at King Khalid University Saudi Arabia for funding through the research groups programme under grant number (R.G.P. 2/445/44).
References
- J. She, J. Jiang, L. Ye, L. Hu, C. Bai and Y. Song, Clin. Transl. Med., 2020, 9, 1–7 CrossRef PubMed.
- W. Wu, A. Wang and M. Liu, Lancet, 2020, 395, 497–506 CrossRef PubMed.
- D. McGonagle, K. Sharif, A. O'Regan and C. Bridgewood, Autoimmun. Rev., 2020, 19, 102537 CrossRef CAS PubMed.
- D. M. Altmann and R. J. Boyton, BMJ, 2021, 372 Search PubMed.
-
W. H. Organization, World Health Organization 2020 guidelines on physical activity and sedentary behaviour, 2020 Search PubMed.
- S. Shareef, S. Akhtar, N. Tufail and F. Ahmad, J. Univ. Med. Dent. Coll., 2022, 13, 299–303 Search PubMed.
- E. Rezel-Potts, A. Douiri, X. Sun, P. J. Chowienczyk, A. M. Shah and M. C. Gulliford, PLoS Med., 2022, 19, e1004052 CrossRef CAS PubMed.
- E. Wynberg, H. D. van Willigen, M. Dijkstra, A. Boyd, N. A. Kootstra, J. G. van den Aardweg, M. J. van Gils, A. Matser, M. R. de Wit and T. Leenstra, Clin. Infect. Dis., 2022, 75, e482–e490 CrossRef PubMed.
- M. Chutiyami, U. M. Bello, D. Salihu, D. Ndwiga, M. A. Kolo, R. Maharaj, K. Naidoo, L. Devar, P. Pratitha and P. Kannan, Int. J. Nurs. Stud., 2022, 104211 CrossRef PubMed.
- A. Gupta, M. V. Madhavan, K. Sehgal, N. Nair, S. Mahajan, T. S. Sehrawat, B. Bikdeli, N. Ahluwalia, J. C. Ausiello and E. Y. Wan, Nat. Med., 2020, 26, 1017–1032 CrossRef CAS PubMed.
- Y. Cao, A. Hiyoshi and S. Montgomery, BMJ Open, 2020, 10, e043560 CrossRef PubMed.
- H. C. Maltezou, A. Pavli and A. Tsakris, Vaccines, 2021, 9, 497 CrossRef CAS PubMed.
- H. E. Davis, G. S. Assaf, L. McCorkell, H. Wei, R. J. Low, Y. Re'em, S. Redfield, J. P. Austin and A. Akrami, eClinicalMedicine, 2021, 38, 101019 CrossRef PubMed.
- S. SeyedAlinaghi, A. M. Afsahi, M. MohsseniPour, F. Behnezhad, M. A. Salehi, A. Barzegary, P. Mirzapour, E. Mehraeen and O. Dadras, Arch. Acad. Emerg. Med., 2021, 9 CAS.
- E. A. Toraih, R. M. Elshazli, M. H. Hussein, A. Elgaml, M. Amin, M. El-Mowafy, M. El-Mesery, A. Ellythy, J. Duchesne and M. T. Killackey, J. Med. Virol., 2020, 92, 2473–2488 CrossRef CAS PubMed.
- Q. Xiong, M. Xu, J. Li, Y. Liu, J. Zhang, Y. Xu and W. Dong, Clin. Microbiol. Infect., 2021, 27, 89–95 CrossRef CAS PubMed.
- A. Carfi, R. Bernabei, F. Landi and C.-P.-A. C. S. G. Gemelli Against, JAMA, 2020, 324, 603–605 CrossRef CAS PubMed.
- H. Fogarty, L. Townsend, H. Morrin, A. Ahmad, C. Comerford, E. Karampini, H. Englert, M. Byrne, C. Bergin, J. M. O'Sullivan, I. Martin-Loeches, P. Nadarajan, C. Bannan, P. W. Mallon, G. F. Curley, R. J. S. Preston, A. M. Rehill, D. McGonagle, C. Ni Cheallaigh, R. I. Baker, T. Renné, S. E. Ward and J. S. O'Donnell, J. Thromb. Haemostasis, 2021, 19, 2546–2553 CrossRef CAS PubMed.
- C. Carvalho-Schneider, E. Laurent, A. Lemaignen, E. Beaufils, C. Bourbao-Tournois, S. Laribi, T. Flament, N. Ferreira-Maldent, F. Bruyère, K. Stefic, C. Gaudy-Graffin, L. Grammatico-Guillon and L. Bernard, Clin. Microbiol. Infect., 2021, 27, 258–263 CrossRef CAS PubMed.
- D. T. Arnold, F. W. Hamilton, A. Milne, A. J. Morley, J. Viner, M. Attwood, A. Noel, S. Gunning, J. Hatrick, S. Hamilton, K. T. Elvers, C. Hyams, A. Bibby, E. Moran, H. I. Adamali, J. W. Dodd, N. A. Maskell and S. L. Barratt, Thorax, 2021, 76, 399–401 CrossRef PubMed.
- M. Bellan, D. Soddu, P. E. Balbo, A. Baricich, P. Zeppegno, G. C. Avanzi, G. Baldon, G. Bartolomei, M. Battaglia, S. Battistini, V. Binda, M. Borg, V. Cantaluppi, L. M. Castello, E. Clivati, C. Cisari, M. Costanzo, A. Croce, D. Cuneo, C. De Benedittis, S. De Vecchi, A. Feggi, M. Gai, E. Gambaro, E. Gattoni, C. Gramaglia, L. Grisafi, C. Guerriero, E. Hayden, A. Jona, M. Invernizzi, L. Lorenzini, L. Loreti, M. Martelli, P. Marzullo, E. Matino, A. Panero, E. Parachini, F. Patrucco, G. Patti, A. Pirovano, P. Prosperini, R. Quaglino, C. Rigamonti, P. P. Sainaghi, C. Vecchi, E. Zecca and M. Pirisi, JAMA Netw. Open, 2021, 4, e2036142 CrossRef PubMed.
- A. Dennis, M. Wamil, J. Alberts, J. Oben, D. J. Cuthbertson, D. Wootton, M. Crooks, M. Gabbay, M. Brady, L. Hishmeh, E. Attree, M. Heightman, R. Banerjee and A. Banerjee, BMJ Open, 2021, 11, e048391 CrossRef PubMed.
- C. Huang, L. Huang, Y. Wang, X. Li, L. Ren, X. Gu, L. Kang, L. Guo, M. Liu, X. Zhou, J. Luo, Z. Huang, S. Tu, Y. Zhao, L. Chen, D. Xu, Y. Li, C. Li, L. Peng, Y. Li, W. Xie, D. Cui, L. Shang, G. Fan, J. Xu, G. Wang, Y. Wang, J. Zhong, C. Wang, J. Wang, D. Zhang and B. Cao, Lancet, 2021, 397, 220–232 CrossRef CAS PubMed.
- K. Stavem, W. Ghanima, M. K. Olsen, H. M. Gilboe and G. Einvik, Thorax, 2021, 76, 405–407 CrossRef PubMed.
- H. Ahmed, K. Patel, D. C. Greenwood, S. Halpin, P. Lewthwaite, A. Salawu, L. Eyre, A. Breen, R. O'Connor, A. Jones and M. Sivan, J. Rehabil. Med., 2020, 52, jrm00063 Search PubMed.
- A. Parker, E. H. Louw, U. Lalla, C. F. N. Koegelenberg, B. W. Allwood, H. Rabie, S. I. Sibeko, J. J. Taljaard and S. Lahri, S. Afr. Med. J., 2020, 110, 957–958 CrossRef CAS PubMed.
- M. Michelen, L. Manoharan, N. Elkheir, V. Cheng, A. Dagens, C. Hastie, M. O'Hara, J. Suett, D. Dahmash, P. Bugaeva, I. Rigby, D. Munblit, E. Harriss, A. Burls, C. Foote, J. Scott, G. Carson, P. Olliaro, L. Sigfrid and C. Stavropoulou, BMJ Glob Health, 2021, 6 Search PubMed.
- S. J. Yong, Infect. Dis., 2021, 53, 737–754 CrossRef CAS PubMed.
- A. Combrink, N. Irwin, G. Laudin, K. Naidoo, S. Plagerson and A. Mathee, S. Afr. Med. J., 2010, 100, 297–299 CrossRef CAS PubMed.
- A. D. Desai, M. Lavelle, B. C. Boursiquot and E. Y. Wan, Am. J. Physiol. Cell Physiol., 2022, 322, C1–C11 CrossRef CAS PubMed.
- V. Chopra, S. A. Flanders, M. O'Malley, A. N. Malani and H. C. Prescott, Ann. Intern. Med., 2021, 174, 576–578 CrossRef PubMed.
- S. Mandal, J. Barnett, S. E. Brill, J. S. Brown, E. K. Denneny, S. S. Hare, M. Heightman, T. E. Hillman, J. Jacob and H. C. Jarvis, Thorax, 2021, 76, 396–398 CrossRef PubMed.
- H. F. Schwensen, L. K. Borreschmidt, M. Storgaard, S. Redsted, S. Christensen and L. B. Madsen, J. Clin. Pathol., 2021, 74, 400–402 CrossRef CAS PubMed.
- Z. L. Yang, C. Chen, L. Huang, S. C. Zhou, Y. N. Hu, L. M. Xia and Y. Li, Front. Med., 2020, 7, 605088 CrossRef PubMed.
- L. T. McDonald, Am. J. Physiol. Lung Cell. Mol. Physiol., 2021, 320, L257–L265 CrossRef CAS PubMed.
- A.-R. Koczulla, N. Engl. J. Med., 2014, 371, 781 CrossRef CAS PubMed.
- P. M. George, S. L. Barratt, R. Condliffe, S. R. Desai, A. Devaraj, I. Forrest, M. A. Gibbons, N. Hart, R. G. Jenkins and D. F. McAuley, Thorax, 2020, 75, 1009–1016 CrossRef PubMed.
- F. Zhou, Lancet, 2020, 395, 1054–1062 CrossRef CAS PubMed.
- J. H. Tanne, BMJ, 2022, 376–378 Search PubMed.
- F. Farshidfar, N. Koleini and H. Ardehali, JCI Insight, 2021, 6 CrossRef PubMed.
- Q. Ruan, K. Yang, W. Wang, L. Jiang and J. Song, Intensive Care Med., 2020, 46, 846–848 CrossRef CAS PubMed.
- R. Escher, N. Breakey and B. Lämmle, Thromb. Res., 2020, 190, 62 CrossRef CAS PubMed.
- T. Hadid, Z. Kafri and A. Al-Katib, Blood Rev., 2021, 47, 100761 CrossRef CAS PubMed.
- M. G. Lazzaroni, S. Piantoni, S. Masneri, E. Garrafa, G. Martini, A. Tincani, L. Andreoli and F. Franceschini, Blood Rev., 2021, 46, 100745 CrossRef CAS PubMed.
- M. Pennisi, G. Lanza, L. Falzone, F. Fisicaro, R. Ferri and R. Bella, Int. J. Mol. Sci., 2020, 21, 5475 CrossRef CAS PubMed.
- B. Sun, Y. Feng, X. Mo, P. Zheng, Q. Wang, P. Li, P. Peng, X. Liu, Z. Chen and H. Huang, Emerging Microbes Infect., 2020, 9, 940–948 CrossRef CAS PubMed.
- M. Mazza and R. Lorenzo, Anxiety and depression in COVID-19 survivors: role of inflammatory and clinical predictors, Brain Behav. Immun., 2020, 89, 594–600 CrossRef CAS PubMed.
- E. Garrigues, P. Janvier, Y. Kherabi, A. Le Bot, A. Hamon, H. Gouze, L. Doucet, S. Berkani, E. Oliosi and E. Mallart, J. Infect., 2020, 81, e4–e6 CrossRef CAS PubMed.
- A. S. Nordvig, K. T. Fong, J. Z. Willey, K. T. Thakur, A. K. Boehme, W. S. Vargas, C. J. Smith and M. S. Elkind, Neurol. Clin. Pract., 2021, 11, e135–e146 CrossRef PubMed.
- M.-I. Stefanou, L. Palaiodimou, E. Bakola, N. Smyrnis, M. Papadopoulou, G. P. Paraskevas, E. Rizos, E. Boutati, N. Grigoriadis and C. Krogias, Ther. Adv. Chronic Dis., 2022, 13, 20406223221076890 Search PubMed.
- S. Shanbehzadeh, M. Tavahomi, N. Zanjari, I. Ebrahimi-Takamjani and S. Amiri-Arimi, J. Psychosom. Res., 2021, 147, 110525 CrossRef PubMed.
- C. Magro, J. J. Mulvey, D. Berlin, G. Nuovo, S. Salvatore, J. Harp, A. Baxter-Stoltzfus and J. Laurence, Transl. Res., 2020, 220, 1–13 CrossRef CAS PubMed.
- S. Recalcati, J. Eur. Acad. Dermatol. Venereol., 2020, 34(5), e212–e213 CrossRef CAS PubMed.
- C. Galván Casas, A. Catala, G. Carretero Hernández, P. Rodríguez-Jiménez, D. Fernández-Nieto, A. Rodríguez-Villa Lario, I. Navarro Fernández, R. Ruiz-Villaverde, D. Falkenhain-López and M. Llamas Velasco, Br. J. Dermatol., 2020, 183, 71–77 CrossRef PubMed.
- M. Sachdeva, R. Gianotti, M. Shah, L. Bradanini, D. Tosi, S. Veraldi, M. Ziv, E. Leshem and R. P. Dodiuk-Gad, J. Dermatol. Sci., 2020, 98, 75–81 CrossRef CAS PubMed.
- R. Unnikrishnan and A. Misra, Nutr. Diabetes, 2021, 11, 21 CrossRef CAS PubMed.
- A. K. Singh and K. Khunti, Annu. Rev. Med., 2022, 73, 129–147 CrossRef PubMed.
- A. Abdi, M. Jalilian, P. A. Sarbarzeh and Z. Vlaisavljevic, Diabetes Res. Clin. Pract., 2020, 166, 108347 CrossRef CAS PubMed.
- M. Faghir-Gangi, H. Moameri, N. Abdolmohamadi and S. Nematollahi, Clin. Diabetol., 2020, 9, 271–278 CrossRef CAS.
- L. Chan, K. Chaudhary, A. Saha, K. Chauhan, A. Vaid and I. Paranjpe, J. Am. Soc. Nephrol., 2021, 32, 151–160 CrossRef CAS PubMed.
- Y. Peleg, S. Kudose, V. D'Agati, E. Siddall, S. Ahmad, T. Nickolas, S. Kisselev, A. Gharavi and P. Canetta, Kid. Int. Rep., 2020, 5, 940–945 CrossRef PubMed.
- G. Pei, J. Am. Soc. Nephrol., 2020, 31, 1157–1165 CrossRef CAS PubMed.
- Y.-W. An, S. Song, W.-X. Li, Y.-X. Chen, X.-P. Hu, J. Zhao, Z.-W. Li, G.-Y. Jiang, C. Wang and J.-C. Wang, Int. J. Med. Sci., 2021, 18, 176 CrossRef CAS PubMed.
- M. Marks and J. L. Marks, Clin. Med., 2016, 16, 129 CrossRef PubMed.
- H. Zacharias, S. Dubey, G. Koduri and D. D'Cruz, Autoimmun. Rev., 2021, 20, 102883 CrossRef CAS PubMed.
-
E. M. Amenta, A. Spallone, M. C. Rodriguez-Barradas, H. M. El Sahly, R. L. Atmar and P. A. Kulkarni, Postacute COVID-19: an overview and approach to classification, in Open forum infectious diseases, Oxford University Press, US, 2020, vol. 7(12), p. ofaa509 Search PubMed.
- L. Liang, B. Yang, N. Jiang, W. Fu, X. He, Y. Zhou, W.-L. Ma and X. Wang, J. Korean Med. Sci., 2020, 35, 418 CrossRef PubMed.
- F. Salamanna, F. Veronesi, L. Martini, M. P. Landini and M. Fini, Front. Med., 2021, 392 Search PubMed.
- P. K. Dos Santos, E. Sigoli, L. J. Braganca and A. S. Cornachione, Front. Physiol., 2022, 510 Search PubMed.
- K. Nakamura, K. Saito, Y. Hara, T. Aoyagi, K. Kitakawa, Y. Abe, H. Takemura, F. Ikeda, M. Kaku and K. Kanemitsu, BMC Infect. Dis., 2018, 18, 1–5 CrossRef PubMed.
- M. A. Khalili, K. Leisegang, A. Majzoub, R. Finelli, M. K. P. Selvam, R. Henkel, M. Mojgan and A. Agarwal, World J. Men's Health, 2020, 38, 506 CrossRef PubMed.
- B. Ebner, Y. Volz, J.-N. Mumm, C. G. Stief and G. Magistro, Nat. Rev. Urol., 2022, 19, 344–356 CrossRef CAS PubMed.
- L. M. Barton, E. J. Duval, E. Stroberg, S. Ghosh and S. Mukhopadhyay, Am. J. Clin. Pathol., 2020, 153, 725–733 CrossRef CAS PubMed.
- J. K. Logue, N. M. Franko, D. J. McCulloch, D. McDonald, A. Magedson, C. R. Wolf and H. Y. Chu, JAMA Netw. Open, 2021, 4, e210830 CrossRef PubMed.
- H. G. Sequencing, Nature, 2004, 431, 931–945 CrossRef PubMed.
- R. Aebersold, J. N. Agar, I. J. Amster, M. S. Baker, C. R. Bertozzi, E. S. Boja, C. E. Costello, B. F. Cravatt, C. Fenselau and B. A. Garcia, Nat. Chem. Biol., 2018, 14, 206–214 CrossRef CAS PubMed.
- F. Wu, S. Zhao, B. Yu, Y. Chen, W. Wang and Z. Song, Nature, 2020, 579, 265–269 CrossRef CAS PubMed.
-
Y. Watanabe, J. D. Allen, D. Wrapp, J. S. McLellan and M. Crispin, bioRxiv, 2020, preprint, DOI:10.1101/2020.03.26.010322.
- A. Alnor, M. B. Sandberg, C. Gils and P. J. Vinholt, J. Appl. Lab. Med., 2020, 5, 1038–1049 CrossRef PubMed.
- P. Malik, U. Patel and D. Mehta, BMJ Evid. Based Med., 2020, 26, 107–108 CrossRef PubMed.
- B. Sun, N. Tang, M. J. Peluso, N. S. Iyer, L. Torres, J. L. Donatelli, S. E. Munter, C. C. Nixon, R. L. Rutishauser and I. Rodriguez-Barraquer, Cells, 2021, 10, 386 CrossRef CAS PubMed.
- R. Pranata, I. Huang, A. A. Lukito and S. B. Raharjo, Postgrad. Med. J., 2020, 96, 387–391 CrossRef CAS PubMed.
- J.-W. Li, T.-W. Han, M. Woodward, C. S. Anderson, H. Zhou, Y.-D. Chen and B. Neal, Prog. Cardiovasc. Dis., 2020, 63, 518–524 CrossRef PubMed.
- M. Brueckmann, G. Huhle, S. Lang, K. K. Haase, T. Bertsch, C. Weiß, J. J. Kaden, C. Putensen, M. Borggrefe and U. Hoffmann, Circulation, 2005, 112, 527–534 CrossRef CAS PubMed.
- J. Lin, H. Yan, H. Chen, C. He, C. Lin, H. He, S. Zhang, S. Shi and K. Lin, J. Med. Virol., 2021, 93, 934–944 CrossRef CAS PubMed.
- J. Xu and E. Lazartigues, Cell. Mol. Neurobiol., 2022, 42, 305–309 CrossRef CAS PubMed.
- P. Sachin, S. Gadani, J. Cronk, G. Norris and J. Kipnis, J. Immunol., 2012, 189, 4213–4219 CrossRef PubMed.
- C. Huang and L. Ren, Lancet, 2021, 397, 220–232 CrossRef CAS PubMed.
- A. D. Whetton, G. W. Preston, S. Abubeker and N. Geifman, J. Proteome Res., 2020, 19, 4219–4232 CrossRef CAS PubMed.
- W. Tian, W. Jiang, J. Yao, C. J. Nicholson, R. H. Li, H. H. Sigurslid, L. Wooster, J. I. Rotter, X. Guo and R. Malhotra, J. Med. Virol., 2020, 92, 1875–1883 CrossRef CAS PubMed.
- Y. Tang, J. Liu, D. Zhang, Z. Xu, J. Ji and C. Wen, Front. Immunol., 2020, 11, 1708 CrossRef CAS PubMed.
- P. Lorkiewicz and N. Waszkiewicz, J. Clin. Med., 2021, 10, 4142 CrossRef CAS PubMed.
- R. Kast, H. Schirok, S. Figueroa-Pérez, J. Mittendorf, M. Gnoth, H. Apeler, J. Lenz, J. Franz, A. Knorr and J. Hütter, Br. J. Pharmacol., 2007, 152, 1070–1080 CrossRef CAS PubMed.
- K. Ebner, S. B. Sartori and N. Singewald, Curr. Pharm. Des., 2009, 15, 1647–1674 CrossRef CAS PubMed.
- M. Muñoz and R. Coveñas, Amino Acids, 2014, 46, 1727–1750 CrossRef PubMed.
- M. Samsam, R. Coveñas, R. Ahangari, J. Yajeya, J. Narváez and G. Tramu, PAIN®, 2000, 84, 389–395 CrossRef CAS PubMed.
- R. Bang, G. Sass, A. K. Kiemer, A. M. Vollmar, W. L. Neuhuber and G. Tiegs, J. Pharmacol. Exp. Ther., 2003, 305, 31–39 CrossRef CAS PubMed.
- M. Munoz and R. Covenas, Peptides, 2013, 48, 1–9 CrossRef CAS PubMed.
- M. B. Johnson, A. D. Young and I. Marriott, Front. Cell. Neurosci., 2017, 10, 296 Search PubMed.
-
I. Marriott and K. L. Bost, Neuroimmune Circuits, Drugs of Abuse, and Infectious Diseases, 2001, 247–254 Search PubMed.
- M. Covas, L. Pinto and R. Victorino, Clin. Exp. Immunol., 1994, 96, 384–388 CrossRef CAS PubMed.
- I. Marriott and K. L. Bost, J. Immunol., 2000, 165, 182–191 CrossRef CAS PubMed.
- T. M. O'Connor, J. O'Connell, D. I. O'Brien, T. Goode, C. P. Bredin and F. Shanahan, J. Cell. Physiol., 2004, 201, 167–180 CrossRef PubMed.
- I. Chernova, J.-P. Lai, H. Li, L. Schwartz, F. Tuluc, H. M. Korchak, S. D. Douglas and L. E. Kilpatrick, J. Leucocyte Biol., 2009, 85, 154–164 CrossRef CAS PubMed.
- G. Piedimonte, M. M. Rodriguez, K. A. King, S. McLean and X. Jiang, Am. J. Physiol. Lung Cell. Mol. Physiol., 1999, 277, L831–L840 CrossRef CAS PubMed.
- T. Goto, H. Iwai, E. Kuramoto and A. Yamanaka, Jpn. Dent. Sci. Rev., 2017, 53, 117–124 CrossRef PubMed.
- M. Sponchiado, Y. S. Liao, K. R. Atanasova, E. N. Collins, V. Schurmann, L. Bravo and L. R. Reznikov, Physiol. Rep., 2021, 9, e14749 CAS.
-
R. Mehboob, F. J. Ahmad, A. Qayyum, M. A. Rana, S. A. Gilani, M. A. Tariq and J. Akram, MedRxiv, 2020, preprint, DOI:10.1101/2020.08.01.20166678.
- R. Mehboob, M. Kurdi, A. Bamaga, N. Aldardeir, H. Nasief, L. H. Moshref, T. Alsinani, A. O. Rayes and R. H. Jabbad, Front. Med., 2021, 8, 727593 CrossRef PubMed.
- S. Smieszek, Eur. Respir. J., 2021, 58(65), OA4114 Search PubMed.
-
M. Muñoz and R. J. P. Coveñas, 2013, 48, 1–9.
- J. Liu, X. Zheng, Q. Tong, W. Li, B. Wang, K. Sutter, M. Trilling, M. Lu, U. Dittmer and D. Yang, J. Med. Virol., 2020, 92, 491–494 CrossRef CAS PubMed.
-
Z. Feng, B. Diao, R. Wang, G. Wang, C. Wang, Y. Tan, L. Liu, C. Wang, Y. Liu and Y. Liu, MedRxiv, 2020, preprint, DOI:10.1101/2020.03.27.20045427.
- Y. Jamilloux, T. Henry, A. Belot, S. Viel, M. Fauter, T. El Jammal, T. Walzer, B. François and P. Sève, Autoimmun. Rev., 2020, 19, 102567 CrossRef CAS PubMed.
- E. Toniato, R. Ross and S. Kritas, J. Biol. Regul. Homeost Agents, 2020, 34, 11–16 Search PubMed.
- E. Kamphuis, T. Junt, Z. Waibler, R. Forster and U. Kalinke, Blood, 2006, 108, 3253–3261 CrossRef CAS PubMed.
- A. Ivagnès, M. Messaoudene, G. Stoll, B. Routy, A. Fluckiger, T. Yamazaki, K. Iribarren, C. P. Duong, L. Fend and A. Caignard, Oncoimmunology, 2018, 7, e1386826 CrossRef PubMed.
- S. Xia, Y. Tao, L. Cui, Y. Yu and S. Xu, J. Immunol. Res., 2019, 2019, 5370706 Search PubMed.
- F. Leuschner, G. Courties, P. Dutta, L. J. Mortensen, R. Gorbatov, B. Sena, T. I. Novobrantseva, A. Borodovsky, K. Fitzgerald and V. Koteliansky, Eur. Heart J., 2015, 36, 1478–1488 CrossRef CAS PubMed.
- M. S. Abers, O. M. Delmonte, E. E. Ricotta, J. Fintzi, D. L. Fink, A. A. A. de Jesus, K. A. Zarember, S. Alehashemi, V. Oikonomou and J. V. Desai, JCI Insight, 2021, 6, 144455 CrossRef PubMed.
- M. Catanzaro, F. Fagiani, M. Racchi, E. Corsini, S. Govoni and C. Lanni, Signal Transduction Targeted Ther., 2020, 5, 84 CrossRef CAS PubMed.
- Y. Zhao, L. Qin, P. Zhang, K. Li, L. Liang, J. Sun, B. Xu, Y. Dai, X. Li and C. Zhang, JCI Insight, 2020, 5, 139834 CrossRef PubMed.
- L. Gralinski, T. Sheahan, T. Morrison, V. Menachery, K. Jensen, S. Leist, A. Whitmore, M. Heise and R. Baric, mBio, 2018, 9, 10–128 CrossRef PubMed.
-
T. Gao, M. Hu, X. Zhang, H. Li, L. Zhu, H. Liu, Q. Dong, Z. Zhang, Z. Wang and Y. Hu, MedRxiv, 2020, preprint, DOI:10.1101/2020.03.29.20041962.
- R. D. Lopes, A. V. S. Macedo, R. J. Moll-Bernardes, A. Feldman, G. D. A. S. Arruda, A. S. de Souza, D. C. de Albuquerque, L. Mazza, M. F. Santos and N. Z. Salvador, Am. Heart J., 2020, 226, 49–59 CrossRef CAS PubMed.
- W. Tai, L. He, X. Zhang, J. Pu, D. Voronin, S. Jiang, Y. Zhou and L. Du, Cell. Mol. Immunol., 2020, 17, 613–620 CrossRef CAS PubMed.
- H. Kai and M. Kai, Hypertens. Res., 2020, 43, 648–654 CrossRef CAS PubMed.
- Z. Xu, L. Shi, Y. Wang, J. Zhang, L. Huang, C. Zhang, S. Liu, P. Zhao, H. Liu and L. Zhu, Lancet Respir. Med., 2020, 8, 420–422 CrossRef CAS PubMed.
- M. Pieri, M. Ciotti, M. Nuccetelli, M. A. Perrone, M. T. Calio, M. S. Lia, M. Minieri and S. Bernardini, Int. Immunopharmacol., 2021, 95, 107512 CrossRef CAS PubMed.
- A. Zinellu, P. Paliogiannis, C. Carru and A. A. Mangoni, Int. J. Infect. Dis., 2021, 105, 668–674 CrossRef CAS PubMed.
- D. Azoulay, M. Shehadeh, S. Chepa, E. Shaoul, M. Baroum, N. A. Horowitz and E. Kaykov, J. Infect., 2020, 81, e79–e81 CrossRef CAS PubMed.
- A. Bhargava, E. A. Fukushima, M. Levine, W. Zhao, F. Tanveer, S. M. Szpunar and L. Saravolatz, Clin. Infect. Dis., 2020, 71, 1962–1968 CrossRef CAS PubMed.
- F. Wang, J. Nie, H. Wang, Q. Zhao, Y. Xiong, L. Deng, S. Song, Z. Ma, P. Mo and Y. Zhang, J. Infect. Dis., 2020, 221, 1762–1769 CrossRef CAS PubMed.
- C. Guo, B. Li, H. Ma, X. Wang, P. Cai, Q. Yu, L. Zhu, L. Jin, C. Jiang and J. Fang, bioRxiv, 2020, 22(5), bbab085, DOI:10.1101/864488.
- C. Qin, L. Zhou, Z. Hu, S. Zhang, S. Yang and Y. Tao, Clin. Infect. Dis., 2020, 71, 762–768 CrossRef CAS PubMed.
- L. Lei, H. Qian, X. Yang, X. Zhang, D. Zhang, T. Dai, R. Guo, L. Shi, Y. Cheng and B. Zhang, J. Cell. Mol. Med., 2020, 24, 11603–11606 CrossRef CAS PubMed.
-
D. Pinto, Y.-J. Park, M. Beltramello, A. C. Walls, M. A. Tortorici, S. Bianchi, S. Jaconi, K. Culap, F. Zatta and A. De Marco, bioRxiv, 2020, preprint, DOI:10.1101/2020.04.07.023903.
- O. J. McElvaney, N. L. McEvoy, O. F. McElvaney, T. P. Carroll, M. P. Murphy, D. M. Dunlea, O. Ní Choileáin, J. Clarke, E. O'Connor and G. Hogan, Am. J. Respir. Crit. Med., 2020, 202, 812–821 CrossRef CAS PubMed.
-
D. Pinto, Y.-J. Park, M. Beltramello, A. C. Walls, M. A. Tortorici, S. Bianchi, S. Jaconi, K. Culap, F. Zatta and A. De Marco, Structural and functional analysis of a potent sarbecovirus neutralizing antibody, bioRxiv, 2020, preprint, DOI:10.1101/2020.04.07.023903.
- L. M. Buja, D. Wolf, B. Zhao, B. Akkanti, M. Do, L. Lelenwa, N. Do, G. Ottaviani, M. Tarek and D. O. Trujillo, Cardiovasc. Pathol., 2019, 48, 107233 CrossRef PubMed.
- F. Yasui, C. Kai, M. Kitabatake, S. Inoue, M. Yoneda, S. Yokochi, R. Kase, S. Sekiguchi, K. Morita and T. Hishima, J. Immunol., 2008, 181, 6337–6348 CrossRef CAS PubMed.
-
E. Adams, M. Ainsworth, R. Anand, M. I. Andersson, K. Auckland, J. K. Baillie, E. Barnes, S. Beer, J. I. Bell and T. Berry, MedRxiv, 2020, preprint, DOI:10.1101/2020.04.15.20066407.
- W. Zhang, Y. Zhao, F. Zhang, Q. Wang, T. Li, Z. Liu, J. Wang, Y. Qin, X. Zhang and X. Yan, Clin. Immunol., 2020, 214, 108393 CrossRef CAS PubMed.
- B. Silva Andrade, S. Siqueira, W. R. de Assis Soares, F. de Souza Rangel, N. O. Santos, A. dos Santos Freitas, P. Ribeiro da Silveira, S. Tiwari, K. J. Alzahrani and A. Goes-Neto, Viruses, 2021, 13, 700 CrossRef PubMed.
- M. J. Pereson, M. N. Badano, N. Aloisi, R. Chuit, M. E. de Bracco and P. Bare, Microbiol. Spectrum, 2022, 10, e01411–e01421 CAS.
- M. J. Peluso, S. Lu, A. F. Tang, M. S. Durstenfeld, H.-e. Ho, S. A. Goldberg, C. A. Forman, S. E. Munter, R. Hoh and V. Tai, J. Infect. Dis., 2021, 224, 1839–1848 CrossRef CAS PubMed.
- D. Talwar, S. Kumar, S. Acharya, N. Raisinghani, S. Madaan, V. Hulkoti, A. Akhilesh, S. Khanna, D. Shah and S. Nimkar, Ind. J. Crit. Care Med., 2022, 26, 39 CrossRef CAS PubMed.
- D. Zhao, F. Yao, L. Wang, L. Zheng, Y. Gao, J. Ye, F. Guo, H. Zhao and R. Gao, Clin. Infect. Dis., 2020, 71, 756–761 CrossRef CAS PubMed.
- B. K. Patterson, J. Guevara-Coto, R. Yogendra, E. B. Francisco, E. Long, A. Pise, H. Rodrigues, P. Parikh, J. Mora and R. A. Mora-Rodríguez, Front. Immunol., 2021, 12, 2520 Search PubMed.
- F. Zeng, Y. Huang, Y. Guo, M. Yin, X. Chen, L. Xiao and G. Deng, Int. J. Infect. Dis., 2020, 96, 467–474 CrossRef CAS PubMed.
- H. Satış, H. S. Özger, P. A. Yıldız, K. Hızel, Ö. Gulbahar, G. Erbaş, G. Aygencel, O. G. Tunccan, M. A. Öztürk and M. Dizbay, Cytokine, 2021, 137, 155302 CrossRef PubMed.
- S. A. Ihim, S. D. Abubakar, Z. Zian, T. Sasaki, M. Saffarioun, S. Maleknia and G. Azizi, Front. Immunol., 2022, 13, 919973 CrossRef PubMed.
- F. Coperchini, L. Chiovato, G. Ricci, L. Croce, F. Magri and M. Rotondi, Cytokine Growth Factor Rev., 2021, 58, 82–91 CrossRef CAS PubMed.
- Z.-S. Xu, T. Shu, L. Kang, D. Wu, X. Zhou, B.-W. Liao, X.-L. Sun, X. Zhou and Y.-Y. Wang, Signal Transduction Targeted Ther., 2020, 5, 100 CrossRef CAS PubMed.
- J. Hadjadj, N. Yatim, L. Barnabei, A. Corneau, J. Boussier, N. Smith, H. Péré, B. Charbit, V. Bondet and C. Chenevier-Gobeaux, Science, 2020, 369, 718–724 CrossRef CAS PubMed.
- R. A. Grant, L. Morales-Nebreda, N. S. Markov, S. Swaminathan, M. Querrey, E. R. Guzman, D. A. Abbott, H. K. Donnelly, A. Donayre and I. A. Goldberg, Nature, 2021, 590, 635–641 CrossRef CAS PubMed.
- S. Lee, G. Y. Yoon, S. J. Lee, Y.-C. Kwon, H. W. Moon, Y.-J. Kim, H. Kim, W. Lee, G. U. Jeong and C. Kim, Microbiol. Spect., 2022, 10, e02322–e02371 Search PubMed.
- C. Huang, Y. Wang, X. Li, L. Ren, J. Zhao, Y. Hu, L. Zhang, G. Fan, J. Xu and X. Gu, Lancet, 2020, 395, 497–506 CrossRef CAS PubMed.
- I. Sorić Hosman, I. Kos and L. Lamot, Front. Immunol., 2021, 11, 631299 CrossRef PubMed.
-
S. A. B. Garcia and N. Guzman, in StatPearls [Internet], StatPearls Publishing, 2022 Search PubMed.
- A. P. Rathore and A. L. St John, Curr. Opin. Immunol., 2020, 66, 74–81 CrossRef CAS PubMed.
-
G. K. Jena, C. N. Patra and J. Sruti, in Coronavirus Drug Discovery, Elsevier, 2022, pp. 37–48 Search PubMed.
- A. Niraula, B. Gelal and M. Lamsal, J. Nepal Med. Assoc., 2020, 58, 1107 Search PubMed.
- A. N. Masterson, B. B. Muhoberac, A. Gopinadhan, D. J. Wilde, F. T. Deiss, C. C. John and R. Sardar, Anal. Chem., 2021, 93, 8754–8763 CrossRef CAS PubMed.
Footnote |
† These authors contributed equally. |
|
This journal is © The Royal Society of Chemistry 2023 |