DOI:
10.1039/D2TC00850E
(Communication)
J. Mater. Chem. C, 2022,
10, 7443-7448
High-performance ultra-narrow-band green-emitting phosphor LaMgAl11O19:Mn2+ for wide color-gamut WLED backlight displays†
Received
1st March 2022
, Accepted 18th April 2022
First published on 21st April 2022
Abstract
Explorations of narrow-band green-emitting phosphors have attracted much attention due to their great application potential in wide color-gamut wLED backlight displays. Herein, a high-performance narrow-band green-emitting phosphor LaMg0.72Al11O19:0.28Mn2+ (LMAO:0.28Mn2+) was synthesized with a high activator doping content. The LMAO:0.28Mn2+ green phosphor exhibits an ultra-narrow-band green emission peaking at 517 nm with an intriguing full width at half maximum of ∼24 nm. The LMAO:0.28Mn2+ not only has a good internal quantum efficiency of 97.6% but also has an excellent low thermal quenching performance (92.3% peak intensity @423 K), especially an excellent resistance to color drifting (3.82‰ @423 K). A wLED device fabricated using LMAO:0.28Mn2+, K2SiF6:Mn4+ phosphors and a blue chip shows a wide color-gamut of 131% National Television System Committee (NTSC) standard, making LMAO:0.28Mn2+ a highly promising candidate for wide color-gamut wLED backlights. The new strategy can be easily expanded to other systems and boost the development of efficient narrow-band emitting phosphors.
1. Introduction
In recent decades, phosphor-converted white LEDs (pc-wLEDs) have been mainstream candidates for liquid crystal display (LCD) backlights due to their outstanding properties such as low power consumption, high brightness, low heat emission, environment-friendliness and long lifetime.1–3 Compared with other display technologies, narrow-band phosphors have been recognized as key enablers for color gamut backlights in LCDs.4–6 Many high-efficiency narrow-band green emitters have been developed to realize wide color-gamut backlight displays. CdSe/ZnS and perovskite quantum dots (QDs) CsPbBr3 are promising candidates due to the super-narrow FWHM of ∼20 nm, but Cd and Pb are toxic, and CsPbBr3 has limited moisture resistance and poor thermal stability, which inevitably restricts their practical application.7 Furthermore, many lanthanide activated (e.g., Eu2+, Tb3+) efficient narrow-band green emitting phosphors have been developed, such as β-SiAlON:Eu2+ (FWHM ∼55 nm), γ-AlON:Mn2+, Mg2+ (FWHM ∼44 nm), Ba[Li2(Al2Si2)N6]:Eu2+ (FWHM ∼57 nm), Ba2LiSi7AlN12:Eu2+ (FWHM ∼61 nm), SrGa2S4:Eu2+ (FWHM ∼47 nm), RbLi(Li3SiO4)2:Eu2+ (FWHM ∼42 nm) and RbNa(Li3SiO4)2:Eu2+ (FWHM ∼41 nm).4,8–13 However, these materials all suffer from the problems of high cost, harsh synthesis conditions and poor stability. To achieve cheaper and more stable narrow green-emitting phosphors, some transition-metal-activated (e.g., Mn2+) silicate and aluminosilicate phosphors have been investigated, such as Zn2SiO4:Mn2+ (FWHM ∼42 nm), MgAl2O4:Mn2+ (FWHM ∼35 nm), BaZnAl10O17:Mn2+ (FWHM ∼31 nm), BaAl12O19:Mn2+ (FWHM ∼26 nm) and Sr2MgAl22O36:Mn2+ (FWHM ∼26 nm).7,14–17 It is a great possibility to achieve a wide color-gamut (>120% NTSC) wLED device by using a suitable Mn2+ based phosphor as the green emitter. However, the absorption and emission of Mn2+ belong to the spin-forbidden d–d transition and depend strongly on the host lattice.18,19 It is a fundamental challenge to obtain a cheaper and highly efficient Mn2+ based narrow-band green emitting phosphor.
Due to the energy transfer interaction between luminescence centers and luminescence killer centers,8,20–22 there is optimal doping content for an activator in a phosphor. When the activator content exceeds its optimal value, the concentration-quenching effect can occur and the emission intensity can decrease.23,24 While the energy transfer can be reduced between long-distance luminescence centers and the phosphor can achieve efficient emission in spite of high activator content.25 If there is only one site for the activator to occupy, it will contribute to the narrow band emission for the phosphor.26 Thus, designing activator doping at independently remote isolated sites can not only be beneficial to the narrow band emission but also enlarge the optimal doping content of the activator to realize high content activators inducing highly efficient emission for phosphors. LaMgAl11O19 (LMAO) crystallizes in a slightly distorted magnetoplumbite PbFe12O19 (M-type) structure with hexagonal space group P63/mmc.27 There is only one Mg2+ site. In particular, the distances between isolated Mg2+ sites are remote. In this work, a high-performance narrow-band green-emitting phosphor LaMg0.72Al11O19:0.28Mn2+ (LMAO:0.28Mn2+) was synthesized with a high Mn2+ doping content. LMAO:0.28Mn2+ exhibits an impressive ultra-narrow-band emission peaking at 517 nm with a fwhm of ∼24 nm and a high internal quantum efficiency (IQE) up to 97.6%. Furthermore, LMAO:0.28Mn2+ shows an outstanding thermal quenching resistance, maintaining 92.3% peak intensity and 110.1% integrated intensity at 423 K, which is superior to commercial narrow-band green-emitting phosphor β-SiAlON:Eu2+. A pc-wLED device fabricated by LMAO:0.28Mn2+, K2SiF6:Mn4+ (KSF:Mn4+) phosphors and a blue InGaN chip exhibits a wide color gamut up to 131% NTSC and 97.8% Rec. 2020. This work opens a new path to develop high-performance narrow-band green emitting phosphors for wide color-gamut wLED backlight displays.
2. Results and discussion
The crystal structure of LMAO is shown in Fig. 1a. There is one La and one Mg crystallographic site and five distinct Al crystallographic sites. Mg1 and Al3 are co-occupied with a ratio of 1
:
1 and exhibit 4-fold coordination with neighboring O atoms. The independent Mg site exhibits a highly symmetric tetrahedron coordination environment, which is in favor of small Stokes shift and narrow-band green emission.26 The structure framework of LMAO is composed of [Mg1/Al3O4] tetrahedra and [AlO6] octahedra, which connect each other by corner-sharing and edge-sharing to form a three-dimensional structure (Fig. 1a). This rigid structure feature could reduce the structural relaxation of the luminescence center in the excited state and prefer a narrow-band emission.28,29 Furthermore, it could also be of benefit to obtain a phosphor with low thermal quenching. It is worth noting that the [Mg/AlO4] tetrahedra are isolated and they can’t connect each other. Viewing along the c axis direction (Fig. 1b), there are two distances between Mg1/Al3 atoms, the short distance d = 9.7876 Å and the long distance d = 12.1774 Å. These distances far outweigh the usual distances between Mg atoms observed in other known Mg-containing phosphors,7,17 contributing to reducing the energy transfer between activator ions doped at the Mg1 site and boosting the highly efficient narrow-band emission.25 Meanwhile, the nearest distance between isolated Mg1/Al3 atoms is d = 3.4426 Å (Fig. 1b). There is a six-membered ring connected by corner-sharing of six [AlO6] tetrahedra between the nearest [Mg/AlO4] tetrahedra (Fig. 1c), which contributes to reducing the interactions between the activator ions doped at the nearest Mg1 site and induces a positive effect on the narrow-band emission. Considering the valence state and effective ionic radius with different coordination number (cn) (Al3+: cn = 4, r = 0.39 Å, cn = 6, r = 0.535 Å; La3+: cn = 12, r = 1.36 Å; Mg2+: cn = 4, r = 0.57 Å; Mn2+: cn = 4, r = 0.66 Å, cn = 6, r = 0.67 Å) and green emission of LMAO:0.28Mn2+ (Fig. 1e(iii), Fig. 2a),30 it is rational that Mn2+ tends to occupy the Mg2+ site. The as-synthesized sample LMAO:0.28Mn2+ was investigated on XRD Rietveld refinement by Topas 5.31 The observed and calculated X-ray powder diffraction patterns for LMAO:0.28Mn2+, as well as the difference profile of the Rietveld refinement are illustrated in Fig. 1d. The results of Rietveld refinement for LMAO:0.28Mn2+ are summarized in Table S1 (ESI†). All refined crystallographic parameters well satisfy the conditions, and good fits are obtained with Rp = 3.86, Rwp = 5.16 and GOF = 1.60. The refined crystallographic parameters are slightly bigger than the host,27 indicating the substitution of Mg2+ by Mn2+. Furthermore, the LMAO:0.28Mn2+ microcrystal sample exhibits a spindle shape in the optical microscopy (Fig. 1e(i)), which shows a bright shine under the polarized lens (Fig. 1e(ii)), indicating the good crystallization. Under 365 nm excitation, the LMAO:0.28Mn2+ sample shows bright green light in the dark environment (Fig. 1e(iii)). The spindle shape of LMAO:0.28Mn2+ particles is also seen in the SEM image with a size ∼50 μm (Fig. 1e(iv)). The SEM-EDS element mapping results demonstrate that the as-prepared sample contains La, Mg, Al, O and Mn elements (Fig. 1e(v–ix)), which are homogeneously distributed in the phosphor particles. Moreover, the uniform Mn element distribution further supports that Mn2+ penetrates the LMAO structure and the substitution of Mg2+. The typical selected-area electron diffraction (SAED) image and the high resolution TEM (HRTEM) image taken from a fragment of the spindle sample demonstrate that the as-prepared LMAO:0.28Mn2+ is a single crystal, as shown in Fig. S2 (ESI†).
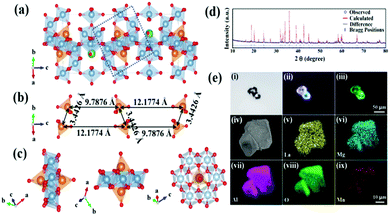 |
| Fig. 1 (a) The crystal structure of LMAO. La atoms (green); Mg atoms (brown); Al atoms (light gray); O atoms (red). (b) The isolated [MgO4] tetrahedra in the crystal structure. (c) The connection of the nearest isolated [Mg1/Al3O4] tetrahedra by a six-membered ring from different directions. (d) The Rietveld refinement XRD pattern of LMAO:0.28Mn2+. (e) i–iii: Optical microscopy images of LMAO:0.28Mn2+ in bright field (i), under the polarized lens in bright field (ii) and excited by a 365 nm UV lamp in dark field (iii), respectively; iv–ix: SEM image and SEM-EDS element mapping images of LMAO:0.28Mn2+. | |
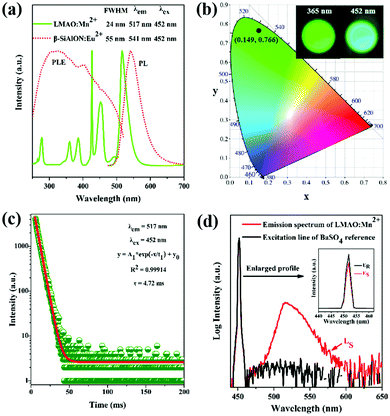 |
| Fig. 2 (a) PLE and PL spectra of LMAO:0.28Mn2+ powder (green solid line) and the commercial green emitting phosphor β-SiAlON:Eu2+ (red dotted line), respectively. (b) The CIE chromaticity coordinate diagram for LMAO:0.28Mn2+ (inset figure: a digital photograph of the LMAO:0.28Mn2+ sample under excitation at 365 nm and 452 nm). (c) The decay curve of LMAO:0.28Mn2+ under excitation at 452 nm, monitored at 517 nm. (d) Excitation line of BaSO4 and the emission spectrum of the LMAO:0.28Mn2+ phosphor collected by using an integrating sphere, the inset shows the magnification for the excitation line of BaSO4 and the emission spectrum of the LMAO:0.28Mn2+. | |
The optimal Mn2+ content is x = 0.28 for LMAO:xMn2+ (x = 0.04–0.68) (Fig. S3, ESI†), which is a considerably higher value than that of usual phosphors. Thanks to the substitution of Mg2+ by Mn2+ at the Mg1 site, LMAO:0.28Mn2+ exhibits an exciting ultra-narrow-band green emission peaking at 517 nm with a FWHM of ∼24 nm under 452 nm excitation (Fig. 2a). The as-prepared LMAO:0.28Mn2+ sample shows an intense green light under 365 nm and 452 nm lamp irradiation (Fig. 2b inset picture). The photoluminescence excitation (PLE) spectrum of LMAO:0.28Mn2+ monitored at 517 nm exhibits a broad band from 275 to 500 nm, indicating that it can be excited by UV and blue light. Compared with the LMAO host, LMAO:0.28Mn2+ shows a characteristic absorption of Mn2+ in the range of 250–500 nm (Fig. S4, ESI†), corresponding to its PLE (Fig. 2a). Surprisingly, the self-reduction phenomenon of Mn is discovered and the emission intensities are equal for LMAO:0.28Mn2+ synthesized in air conditions and a 10%H2/90%N2 atmosphere (Fig. S5, ESI†), indicating that it has an extremely low preparation cost and a very great commercial potential. The commercial β-SiAlON:Eu2+ is chosen as a reference to compare the optical performance. β-SiAlON:Eu2+ shows a narrow-band emission peaking at 541 nm with a FWHM ∼55 nm under 452 nm excitation (Fig. 2a). LMAO:0.28Mn2+ shows a much narrower emission band than β-SiAlON:Eu2+, indicating that LMAO:Mn2+ is more suitable for wide color-gamut display backlights. The Commission Internationale deI’Eclairage (CIE) chromaticity coordinates of LMAO:0.28Mn2+ are determined to be (0.149, 0.766) (Fig. 2b). The color purity of LMAO:0.28Mn2+ is calculated to be 83.0%, which is much higher than that of β-SiAlON:Eu2+ and even Sr2MgAl22O36:Mn2+,17 indicating that LMAO:0.28Mn2+ can help to achieve a larger color gamut for wLED backlights. The lifetime of LMAO:0.28Mn2+ is calculated to be 4.72 ms (Fig. 2c), which is less than 5 ms and could avoid the smear signal in LCD displays. Furthermore, under 452 nm excitation, LMAO:0.28Mn2+ shows a high IQE up to 97.6% at room temperature (Fig. 2d), and the calculated absorption efficiency (AE) and external quantum efficiency (EQE) are 26.4% and 25.8%, respectively. Under 426 nm excitation, the IQE of the LMAO:0.28Mn2+ phosphor is up to 99.6%, and the calculated AE and EQE are 16.1% and 16.0%, respectively (Fig. S6, ESI†).
Thermal stability is a key factor for phosphors in practical wLED applications. The temperature-dependent PL properties of LMAO:0.28Mn2+ are investigated under 452 nm excitation in the temperature range of 298–473 K (Fig. 3a and b). The relative peak intensity of LMAO:0.28Mn2+ drops from the original 100% at 298 K to 92.3% at 423 K (Fig. 3c), while the integrated intensity does not show any decrease when the temperature increases from 298 K to 473 K and can increase to 110.1% at 423 K, which can be ascribed to the temperature-induced emission band broadening (FWHM increasing from 24 to 33 nm, Fig. 3c). The results indicate that LMAO:0.28Mn2+ exhibits an excellent thermal quenching resistance property, which even is better than that of β-SiAlON:Eu2+ (Fig. S7, ESI†).32 Interestingly, the emission peak position of the LMAO:0.28Mn2+ phosphor barely shifts (Fig. 3b). As the temperature increases, the color coordinates of LMAO:0.28Mn2+ show a tiny variation (Fig. 3d). Correspondingly, the chromaticity shifts of LMAO:0.28Mn2+ exhibit a surprisingly and extremely tiny change, 3.82‰ at 423 K and 6.63‰ at 473 K (Fig. 3e), which are much better than that of other reported phosphors.33–36 The results indicate that the LMAO:0.28Mn2+ phosphor exhibits an excellent resistance to temperature-dependent color drifting. The luminescence lifetimes of the phosphors usually shorten when temperature-dependent quenching occurs due to an additional nonradiative contribution to the decay process.26 Interestingly, the lifetime of LMAO:0.28Mn2+ barely changes from 4.72 to 4.55 ms with the temperature increasing from 298 K to 473 K (Fig. 3f), confirming the excellent temperature-dependent PL properties of LMAO:0.28Mn2+.7
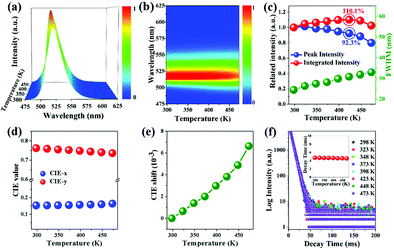 |
| Fig. 3 (a) Temperature-dependent emission spectra of LMAO:0.28Mn2+ recorded at temperatures from 298 to 473 K. (b) The temperature-dependent emission intensities of LMAO:0.28Mn2+ in the wavelength range of 475–625 nm. (c) Normalized integrated intensities, peak intensities, and the variation of the FWHM of LMAO:0.28Mn2+ depending on increasing temperature. (d) Chromaticity coordinate values and (e) chromaticity shift of LMAO:0.28Mn2+ at different temperatures. (f) The decay curves and the decay times (inset figure) of LMAO:0.28Mn2+ at various temperatures. | |
The ultra-narrow-band green emission and excellent temperature-dependent PL properties make LMAO:0.28Mn2+ a desirable candidate for wide color gamut backlight displays. To demonstrate the potential application in backlight displays, a white LED device is fabricated by combining the green phosphor LMAO:0.28Mn2+, the commercial red phosphor KSF:Mn4+ and a blue GaInN chip (λem = 450 nm). At a driven current of 20 mA, the LED device produced white light emission with a correlated color temperature (CCT) of 6667 K and the CIE chromaticity coordinates of (0.312, 0.314) (Fig. 4a). Furthermore, conventional commercial RGB color filters were used to filter the white light. After passing through the color filters, the color coordinates of RGB are (0.6979, 0.3020), (0.1385, 0.7782), and (0.1502, 0.0284), respectively, which exhibits a wide color gamut of 131% NTSC and 97.8% Rec. 2020 in CIE 1931 (Fig. 4b), which is higher than other previous reported pc-wLEDs and even some QLEDs (Table S2, ESI†). Benefiting from the excellent temperature-dependent PL properties of LMAO:0.28Mn2+, the luminescence intensity of the fabricated wLED device continuously increases when the driving current increases from 25 to 300 mA (Fig. 4c and d), indicating that LMAO:0.28Mn2+ can be applied in high power pc-wLEDs. The results indicate that LMAO:Mn2+ is a promising next-generation narrow-band green phosphor for wide color-gamut backlight displays.
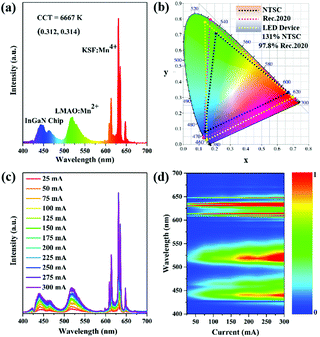 |
| Fig. 4 (a) Electroluminescence spectrum of the wLED fabricated by the as-prepared LMAO:0.28Mn2+, KSiF:Mn4+, and 450 nm blue chip under a current of 20 mA. (b) Color gamut of the NTSC value, and Rec. 2020 value for the fabricated LED device in the 1931 CIE system. (c) Electroluminescence spectra and (d) current-dependent emission intensities of the fabricated LED device in the current range of 25–300 mA. | |
3. Conclusions
In summary, we put forward a new strategy by doping an activator at remote isolated sites with high content to develop a high-performance ultra-narrow-band green emitting phosphor LMAO:0.28Mn2+. LMAO:0.28Mn2+ is successfully synthesized by an easily operated solid-state reaction method, which exhibits an inspiring ultra-narrow-band green emission peaking at 517 nm with a FWHM ∼24 nm under excitation at 452 nm and a high IQE up to 97.6%. The intriguing highly efficient narrow-band emission is related with the high content Mn2+ occupying independently remote isolated Mg2+ sites. As for the LMAO:0.28Mn2+ phosphor, the CIE coordinates are (0.149, 0.766), and the color purity is 83.0%, and the fluorescence lifetime is 4.72 ms. More importantly, the as-prepared LMAO:0.28Mn2+ phosphor exhibits an outstanding thermal quenching resistance property (remaining 92.3% peak intensity and 110.1% integrated intensity at 423 K compared to the corresponding intensity at 298 K) and an excellent resistance to color drifting with chromaticity shifts of 3.82‰ at 423 K and 6.63‰ at 473 K. Exhilaratingly, the emission intensity is equal for LMAO:0.28Mn2+ synthesized in air conditions and in a 10%H2/90%N2 atmosphere, indicating that LMAO:0.28Mn2+ has extremely low preparation cost and very great commercial potential. By combining ultra-narrow-band green emitting LMAO:0.28Mn2+ and narrow-band red emitting KSF:Mn4+ with blue InGaN chips, a wLED device is fabricated with a wide color gamut of 131% NTSC and 97.8% Rec. 2020 in CIE 1931. The luminescence intensity continuously increases for the fabricated wLED device when the driving current increases from 25 to 300 mA, making LMAO:0.28Mn2+ a promising candidate for wide color-gamut and high-power pc-wLED backlight applications. In this work, it is inspiring to develop high-performance narrow-band green emitting phosphors by a new design strategy and accelerate the speed of discovering many new materials for wide color-gamut backlight displays.
Conflicts of interest
There are no conflicts to declare.
Acknowledgements
This work was financially supported by the National Natural Science Foundation of China (Grant No. 52002114 and 12174086).
References
- M. Zhao, Q. Y. Zhang and Z. G. Xia, Narrow-band emitters in LED backlights for liquid-crystal displays, Mater. Today, 2020, 40, 246 CrossRef CAS.
- H. Liao, M. Zhao, M. S. Molokeev, Q. Liu and Z. Xia, Learning from a mineral structure toward an ultra-narrow-band blue-emitting silicate phosphor RbNa3(Li3SiO4)4:Eu2+, Angew. Chem., Int. Ed., 2018, 57, 11728–11731 CrossRef CAS.
- E. F. Schubert and J. K. Kim, Solid-state light sources getting smart, Science, 2005, 308, 1274–1278 CrossRef CAS PubMed.
- M. Zhao, H. Liao, L. Ning, Q. Zhang, Q. Liu and Z. Xia, Next-generation narrow-band green-emitting RbLi(Li3SiO4)2:Eu2+ phosphor for backlight display application, Adv. Mater., 2018, 30, 1802489 CrossRef PubMed.
- X. Zhang, H. C. Wang, A. C. Tang, S. Y. Lin, H. C. Tong, C. Y. Chen, Y. C. Lee, T. L. Tsai and R. S. Liu, Robust and stable narrow-band green emitter: an option for advanced wide-color-gamut backlight display, Chem. Mater., 2016, 28, 8493–8497 CrossRef CAS.
- B. Su, M. S. Molokeev and Z. Xia, Mn2+-based narrow-band green-emitting Cs3MnBr5 phosphor and the performance optimization by Zn2+ alloying, J. Mater. Chem. C, 2019, 7, 11220–11226 RSC.
- E. H. Song, Y. Y. Zhou, Y. Wei, X. X. Han, Z. R. Tao, R. L. Qiu, Z. G. Xia and Q. Y. Zhang, A thermally stable narrow-band green-emitting phosphor MgAl2O4:Mn2+ for wide color gamut backlight display application, J. Mater. Chem. C, 2019, 7, 8192–8198 RSC.
- S. X. Li, L. Wang, D. M. Tang, Y. Cho, X. J. Liu, X. T. Zhou, L. Lu, L. Zhang, T. Takeda, N. Hirosaki and R. J. Xie, Achieving high quantum efficiency narrow-band β-sialon:Eu2+ phosphors for high-brightness LCD backlights by reducing the Eu3+ luminescence killer, Chem. Mater., 2018, 30, 494–505 CrossRef CAS.
- R. J. Xie, N. Hirosaki, X. J. Liu, T. Takeda and H. L. Li, Crystal structure and photoluminescence of Mn2+-Mg2+ codoped gamma aluminum oxynitride (γ-AlON): A promising green phosphor for white light-emitting diodes, Appl. Phys. Lett., 2008, 92, 201905 CrossRef.
- P. Strobel, S. Schmiechen, M. Siegert, A. Tücks, P. J. Schmidt and W. Schnick, Narrow-band green emitting nitridolithoalumosilicate Ba[Li2(Al2Si2)N6]:Eu2+ with framework topology whj for LED/LCD-backlighting applications, Chem. Mater., 2015, 27, 6109–6115 CrossRef CAS.
- T. Takeda, N. Hirosaki, S. Funahshi and R. J. Xie, Narrow-band green-emitting phosphor Ba2LiSi7AlN12:Eu2+ with high thermal stability discovered by a single particle diagnosis approach, Chem. Mater., 2015, 27, 5892–5898 CrossRef CAS.
- Y. Ito, T. Hori, T. Kusunoki, H. Nomura and H. Kondo, A phosphor sheet and a backlight system providing wider color gamut for LCDs, J. Soc. Inf. Disp., 2014, 22, 419 CrossRef CAS.
- H. X. Liao, M. Zhao, Y. Y. Zhou, M. S. Molokeev, Q. L. Liu, Q. Y. Zhang and Z. G. Xia, Polyhedron transformation toward stable narrow-band green phosphors for wide-color-gamut liquid crystal display, Adv. Funct. Mater., 2019, 29, 1901988 CrossRef.
- C. Bertail, S. Maron, V. Buissette, T. L. Mercier, T. Gacoin and J. P. Boilot, Structural and photoluminescent properties of Zn2SiO4:Mn2+ nanoparticles prepared by a protected annealing process, Chem. Mater., 2011, 23, 2961 CrossRef CAS.
- H. R. Li, Y. J. Liang, S. Q. Liu, W. L. Zhang, Y. Y. Bi, Y. M. Gong and W. Lei, Highly efficient green-emitting phosphor BaZnAl10O17:Mn2+ with ultra-narrow band and extremely low thermal quenching for wide color gamut LCD backlights, Adv. Opt. Mater., 2021, 9, 2100799 CrossRef CAS.
- J. Zhou, Y. H. Wang, B. T. Liu and Y. H. Lu, Effect of H3BO3 on structure and photoluminescence of BaAl12O19:Mn2+ phosphor under VUV excitation, J. Alloys Compd., 2009, 484, 439 CrossRef CAS.
- Y. L. Zhu, Y. J. Liang, S. Q. Liu, H. R. Li and J. H. Chen, Narrow-band green-emitting Sr2MgAl22O36:Mn2+ phosphors with superior thermal stability and wide color gamut for backlighting display applications, Adv. Opt. Mater., 2019, 7, 1801419 CrossRef.
- Q. Zhou, L. Dolgov, A. M. Srivastava, L. Zhou, Z. L. Wang, J. X. Shi, M. D. Drami Anin, M. G. Brik and M. M. Wu, Mn2+ and Mn4+ red phosphors: synthesis, luminescence and applications in WLEDs. A review, J. Mater. Chem. C, 2018, 6, 2652 RSC.
- L. Wu, S. J. Sun, Y. X. Bai, Z. G. Xia, L. W. Wu, H. M. Chen, L. R. Zheng, H. Yi, T. Q. Sun, Y. F. Kong, Y. Zhang and J. J. Xu, Defect-induced self-reduction and anti-thermal quenching in NaZn(PO3)3:Mn2+ red phosphor, Adv. Opt. Mater., 2021, 9, 2100870 CrossRef CAS.
- G. Blasse, Luminescence of inorganic solids: from isolated centres to concentrated systems, Prog. Solid State Chem., 1988, 18, 79–171 CrossRef CAS.
- L. Amidani, K. Korthout, J. J. Joos, M. van der Linden, H. F. Sijbom, A. Meijerink, D. Poelman, P. F. Smet and P. Glatzel, Oxidation and luminescence quenching of europium in BaMgAl10O17 blue phosphors, Chem. Mater., 2017, 29, 10122–10129 CrossRef CAS.
- T. Senden, R. J.-A. van Dijk-Moes and A. Meijerink, Quenching of the red Mn4+ luminescence in Mn4+-doped fluoride LED phosphors, Light: Sci. Appl., 2018, 7, 1–13 CrossRef CAS PubMed.
- D. L. Dexter and J. H. Schulman, Theory of concentration quenching in inorganic phosphors, J. Chem. Phys., 1954, 22, 1063 CrossRef CAS.
- H. M. Chen, L. W. Wu, F. Bo, J. K. Jian, L. Wu, H. W. Zhang, L. R. Zheng, Y. F. Kong, Y. Zhang and J. J. Xu, Coexistence of self-reduction from Mn4+ to Mn2+ and elastico-mechanoluminescence in diphase KZn(PO3)3:Mn2+, J. Mater. Chem. C, 2019, 7, 7096–7103 RSC.
- G. J. Zhou, Z. Y. Liu, J. L. Huang, M. S. Molokeev, Z. W. Xiao, C. G. Ma and Z. G. Xia, Unraveling the near-unity narrow-band green emission in zero-dimensional Mn2+-based metal halides: A case study of (C10H16N)2Zn1-xMnxBr4 solid solutions, J. Phys. Chem. Lett., 2020, 11, 5956–5962 CrossRef CAS PubMed.
- C. Li, X. M. Wang, F. F. Chi, Z. P. Yang and H. Jiao, A narrow-band blue emitting phosphor Ca8Mg7Si9N22:Eu2+ for pc-LEDs, J. Mater. Chem. C, 2019, 7, 3730–3734 RSC.
- R. Brandt and B. Müller, Ein beitrag zur kristallchemie der lanthanoidmagnetoplumbite, Z. Anorg. Allg. Chem., 1984, 510, 163–168 CrossRef CAS.
- P. Pust, V. Weiler, C. Hecht, A. Tucks, A. S. Wochnik, A. K. Henss, D. Wiechert, C. Scheu, P. J. Schmidt and W. Schnick, Narrow-band red-emitting Sr[LiAl3N4]:Eu2+ as a next-generation LED-phosphor material, Nat. Mater., 2014, 13, 891–896 CrossRef CAS PubMed.
- J. L. Leaño Jr., M. H. Fang and R. S. Liu, Critical review-narrow-band emission of nitride phosphors for light-emitting diodes: perspectives and opportunities, ECS J. Solid State Sci. Technol., 2017, 7, R3111 CrossRef.
- R. D. Shannon, Revised effective ionic radii and systematic studies of interatomic distances in halides and chalcogenides, Acta Crystallogr., Sect. A: Cryst. Phys., Diffr., Theor. Gen. Crystallogr., 1976, 32, 751–767 CrossRef.
-
A. Coelho, Topas-A: General profile and structure analysis software for powder diffraction data, Bruker-AXS: Madison, WI, 2005 Search PubMed.
- R. J. Xie, N. Hirosaki, H. L. Li, Y. Q. Li and M. Mitomo, Synthesis and photoluminescence properties of β-sialon:Eu2+ (Si6-zAlzOzN8-z:Eu2+): A promising green oxynitride phosphor for white light-emitting diodes, J. Electrochem. Soc., 2007, 154, J314 CrossRef CAS.
- J. Wang, C. C. Tsai, W. C. Cheng, M. H. Chen, C. H. Chung and W. H. Cheng, High thermal stability of phosphor-converted white light-emitting diodes employing Ce:YAG-doped glass, IEEE J. Sel. Top. Quantum Electron., 2011, 17, 741–746 CAS.
- X. J. Zhang, L. Huang, F. J. Pan, M. M. Wu, J. Wang, Y. Chen and Q. Su, Highly thermally stable single-component white-emitting silicate glass for organic-resin-free white-light-emitting diodes, ACS Appl. Mater. Interfaces, 2014, 6, 2709–2717 CrossRef CAS PubMed.
- C. Li, H. Zheng, H. Wei, S. Qiu, L. Xu, X. Wang and H. Jiao, A color tunable and white light emitting Ca2Si5N8:Ce3+, Eu2+ phosphor via efficient energy transfer for near-UV white LEDs, Dalton Trans., 2018, 47, 6860–6867 RSC.
- C. Li, X. M. Wang, Z. P. Yang and H. Jiao, Ca8Mg7Si9N22:Ce3+-a yellow-emitting nitride phosphor for white light emitting diodes, ACS Appl. Electron. Mater., 2020, 2, 936 CrossRef CAS.
|
This journal is © The Royal Society of Chemistry 2022 |