DOI:
10.1039/D4MH00996G
(Communication)
Mater. Horiz., 2024,
11, 5244-5250
Dynamic metal–ligand coordination enables a hydrogel with rewritable dual-mode pattern display†
Received
30th July 2024
, Accepted 5th September 2024
First published on 9th September 2024
Abstract
The realization of dual-mode information display in the same material is of great significance to the expansion of information capacity and the improvement of information security. However, the existing systems lose the ability to re-encode information once they are constructed. Here, dynamic metal–ligand coordination is introduced into a novel hydrogel-based optical platform that allows rewritable dual-mode information display. The hydrogel system consists of a hard lamellar structure of poly(dodecylglyceryl itaconate) (pDGI) and soft double networks of poly(acrylamide)/poly(acrylic acid) (PAAm/PAAc) containing fluorescent carbon dots (CDs). As the carboxylic acid groups can coordinate with metal ions such as Al3+, the layer spacing of the lamellar structure is reduced while CDs aggregate, leading to the blue shift of the structural color and the red shift of the fluorescent color. Additionally, the metal chelating agent, ethylenediaminetetraacetic acid (EDTA), is able to strip away Al3+ ions and restore the two colors, realizing an erasable dual-mode information display. This study opens up a path for the development of new materials and technologies for rewritable dual-mode information protection.
New concepts
With technological advancements, there is an increasing demand for materials capable of securely storing and dynamically displaying information in multiple modes, including the ability to modify content post-deployment. This paper introduces a novel dynamic dual-mode optical hydrogel material utilizing dynamic metal ion coordination, combining a rigid pDGI multilayer structure with flexible PAAm/PAAc polymeric networks incorporating solvatochromic fluorescent CDs. Adding metal ions like Al3+ tightens the pDGI multilayer structure and promotes CD aggregation, enabling a shift between structural color and fluorescence. Post-coordination, EDTA interacts with Al3+ ions within the hydrogel, removing them to restore the material's original color, facilitating information erasure and modification. This study introduces an innovative optical display platform that can read information under both visible and ultraviolet light. It also supports loading, erasing, and reloading of the programmed patterns. This system pioneers dual-mode reading and rewritable optical technology, opening new avenues for research and practical applications.
|
Introduction
Establishing robust information protection platforms is crucial in combating the growing issues of false information and information leaks etc.1–5 These platforms have evolved significantly from static, single-mode information displays to dynamic, multi-channel information decryption systems, which have been driven by advancements in both new materials and techniques.6–9 In particular, dual-mode optical information display refers to a technology that combines two distinct display modes within a single system, offering enhanced versatility and functionality.10–12
One typical dual-mode optical display involves the integration of reflective and emissive display technologies, such as fluorescence and structural color.13–16 For example, Yu's group17 developed a “two-color” photonic ink with adjustable reflection color and stable fluorescence, which is controlled by the liquid crystal's spiral superstructure and the molecular structure of the fluorescent material. This dual-label system exhibits distinct patterns in reflection and fluorescence modes. Yang's team18 proposed a polymer-stabilized cholesteric liquid crystal system with highly temperature-sensitive structural color, photosensitive pigment, and fluorescence color. The structural color changes from red to blue and the fluorescent color varies from blue to red by temperature transition, realizing the transmission of encrypted information with high security. These elegant attempts prove that the dual optical mode of structural color and fluorescent color is feasible in improving visibility, dynamic content display, and versatility.
Owing to the dynamic and adaptive nature, stimuli-responsive gels19,20 can change their properties such as color, transparency, or shape in response to a specific stimulus like temperature, pH, light, electric fields, etc., hiding information under normal circumstances and display information under certain stimuli.21–26 Previously, a dual-mode hydrogel with structural and fluorescent colors was reported for the first time by our group.11 By changing the layer spacing of the lamellar structure to adjust the structural color and changing the type of coordination lanthanide ions to regulate fluorescence color, this kind of soft material can be used for multistage information encryption. However, once the hydrogels have been fabricated, the corresponding two colors are determined.
Herein, a hydrogel with reversible structural and fluorescent color changes was constructed for rewritable dual-mode patterns via the two-step interpenetrating technique. Monomers including dodecylglyceryl itaconate (DGI) and acrylamide (AAm) were firstly photopolymerized to form a rigid lamellar structure and soft hydrogel network. Furthermore, acrylic acid (AAc) bonding CDs penetrated into the PAAm hydrogel network and polymerized to form the second network (Scheme 1A). Metal ions such as Al3+ ions could coordinate with carboxylic acid groups, leading to the variation of the structural color ascribed to the change of the lamellar layer spacing as well as the variation of the fluorescent color caused by the aggregation states of CDs (Scheme 1B). Both the structural color and fluorescent color of the hydrogel could be adjusted on-demand by changing the concentration of Al3+ ions, and further erased by adding EDTA. As shown in Scheme 1C, original information “SMART” could be encoded as information (MART) as well as “ART” by selectively treating the corresponding letters with Al3+ ions, which can be further erased with EDTA solution. These rewritable dual-mode platforms allowed for real-time updates and modifications of displayed content, fostering interactive user experiences and ensuring timely dissemination of information.
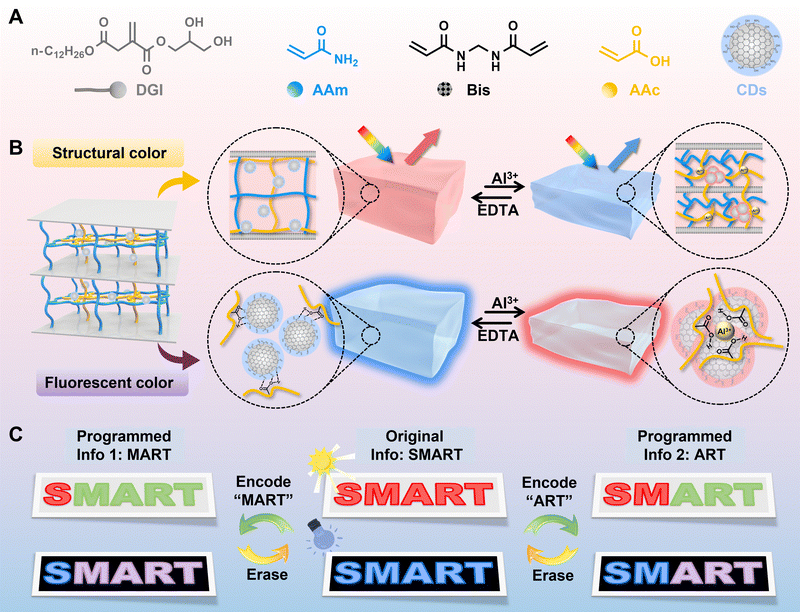 |
| Scheme 1 Dual-mode hydrogel for writable information display. (A) The chemical components used in the construction of the dual-mode hydrogel. (B) Structure diagram of the dual-mode hydrogel, and the coordination of Al3+ and the hydrogel towards the variations of structural color and fluorescent color. (C) Schematic of dual-mode hydrogel for rewritable information display from original information (SMART) to programmed information (MART and ART), in which Al3+ was used for encoding and EDTA for erasing. | |
Results and discussion
Synthesis and characterization of double-mode hydrogels (DMHs)
The amphipathic monomer dodecylglyceryl itaconate (DGI) and solvatochromic carbon dots (CDs) (Fig. S1, ESI†) were synthesized according to our previous reports.11,27 The structure of the synthesized CDs was confirmed by FTIR spectroscopy (Fig. S2, ESI†) and the size of the CDs ranged from 2 to 4 nm, which was characterized by TEM (Fig. S3, ESI†). The former (DGI) is capable of self-assembly into layered structures for achieving structural colors (Fig. S4, ESI†), and the latter can emit different fluorescence in different solvents due to the changes of aggregation states (Fig. S5 and S6, ESI†). Based on the synthesized components, pDGI-PAAm/P(AAc-CDs) hydrogels were fabricated via a two-step interpenetrating method, which can further coordinate with Al3+ to regulate the structural color as well as the fluorescent color (Fig. 1A). The colors were determined by the concentration of Al3+ solution (0 M, 0.05 M, 0.1 M, 0.2 M, 0.4 M, 0.6 M, 0.8 M, 1 M, 1.2 M), and for convenience, the corresponding hydrogels were named DMH0, DMH0.05, DMH0.1, DMH0.2, DMH0.4, DMH0.6, DMH0.8, DMH1.0, and DMH1.2.
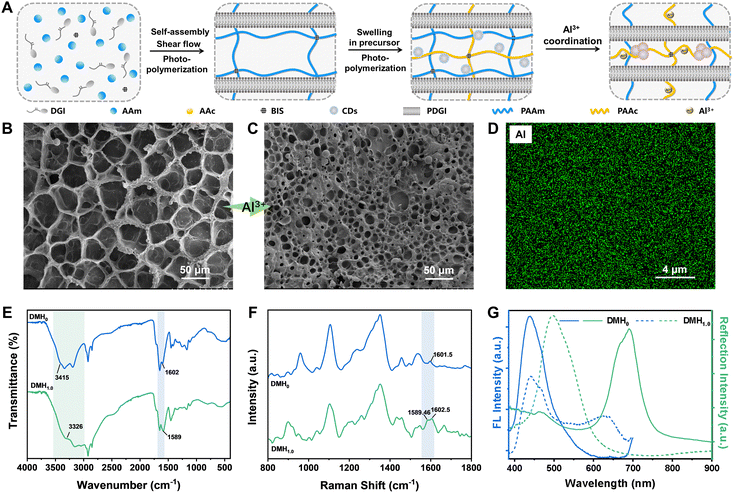 |
| Fig. 1 Preparation and characterization of dual-mode hydrogels with or without Al3+ ions. (A) The flow chart of preparing dual-mode hydrogels and the coordination process with Al3+ ions. (B) and (C) SEM images of pDGI-PAAm/P(AAc-CDs) hydrogel before and after coordinating with Al3+ ions. (D) EDS mapping of Al in pDGI-PAAm/P(AAc-CDs) after being treated with Al3+ solution. (E) ATR-FTIR spectra and (F) Raman spectra of pDGI-PAAm/P(AAc-CDs) hydrogel before and after coordinating with Al3+. (G) Fluorescence spectra (λex = 365 nm) and reflection spectra of the dual-mode hydrogel before and after coordinating with Al3+. | |
The introduction of Al3+ could coordinate with carboxyl groups on PAAc polymer chains and increase the crosslinking density of the hydrogel network, which can be observed by scanning electron microscopy (SEM). As illustrated in Fig. 1B and C, the initial gel pore size of ∼50 μm was reduced to ∼10 μm after being treated with Al3+ ions, which were uniformly distributed in the hydrogel (Fig. 1D). To verify the coordination between carboxylic acid groups and Al3+ ions, ATR-FTIR and Raman were conducted. As shown in Fig. 1E, the hydroxyl peak originating from the carboxyl group at 3415 cm−1 disappears and the stretching vibration peak of –C
O– undergoes a blue shift from 1602 cm−1 to 1589 cm−1 upon coordination with Al3+ ions, confirming the interaction. In the meantime, the vibration peak of the C
O bond in acrylic acid (1601.5 cm−1) splits into two distinct peaks, including a blue-shifted peak at 1589.46 cm−1 and an uncoordinated peak at 1602.5 cm−1 (Fig. 1F), which also proves the coordination between Al3+ ions and carboxylic acid groups.
In addition, the mechanical properties of DMHs also dramatically changed after the introduction of Al3+ ions. The storage modulus of the hydrogel increased tenfold and the tensile strength rose from 76 kPa to 102 kPa (Fig. S7 and S8, ESI†). One interesting phenomenon worth mentioning was that the structural color and fluorescent color of the DMHs would change after the introduction of Al3+ ions, which was also the focus of our follow-up studies. As demonstrated in Fig. 1G, the structural color varied from purple-red (700 nm) to green (510 nm) and the superimposed fluorescent color changed from blue to pink-red with a reduced blue emission (440 nm) and an enhanced red emission (640 nm) upon Al3+ coordination.
Controllable adjusting of the structural colors of DMHs
It is well known that the structural colors arise from the diffraction of light on the periodic nanostructure. Thus, the periodic arrangement of the lamellar structure endows DMHs with bright structural colors. According to Bragg's diffraction law (2d
sin(α) = nλ), the structural colors of the DMHs were determined by the layer spacing, which can be adjusted by Al3+ ions that coordinate carboxylic acid groups on the PAAc polymer chains (Fig. 2A). As illustrated in Fig. S9 (ESI†), the distance of the lamellar was narrowed with the introduction of Al3+ ions, resulting in a blue-shift in the structural color.28–30 During our experiments, we noticed that DMHs treated with Al3+ solutions for different times showed different colors (Fig. S10, ESI†), and the color became stable when the processing time was 5 min. Thus, we chose the treatment time of 5 min for the following experiment and demonstrations. As shown in Fig. 2B, the initial DMH was red and gradually changed to yellow and green with the increase of the Al3+ concentration. We found that there is no noticeable change in the planar view of the hydrogel before and after treatment with Al3+, but there is significant shrinkage in the vertical direction (Fig. S11, ESI†). Correspondingly, the layer spacing of pDGI reduced from 78 nm to 25 nm, which could be directly confirmed by the SEM images (Fig. 2C). Moreover, small-angle X-ray scattering (SAXS) was further utilized to characterize the decrease of the layer spacing (Fig. S12, ESI†), and the results show that the scattering vector q increases with the increase of the Al3+ concentration, which exactly proves this. Additionally, the reflection peaks of DMHs on the reflection spectra had a blue shift of 300 nm and the related CIE chromaticity coordinates also changed as the concentration of the coordination Al3+ increases (Fig. 2D and E). More importantly, the Al3+ ions could be removed with the action of ion chelating agent EDTA to achieve complete restoration of the structural color, which exhibited excellent repeatability and stability during 10 cycles (Fig. 1F and Fig. S13–S15, ESI†).
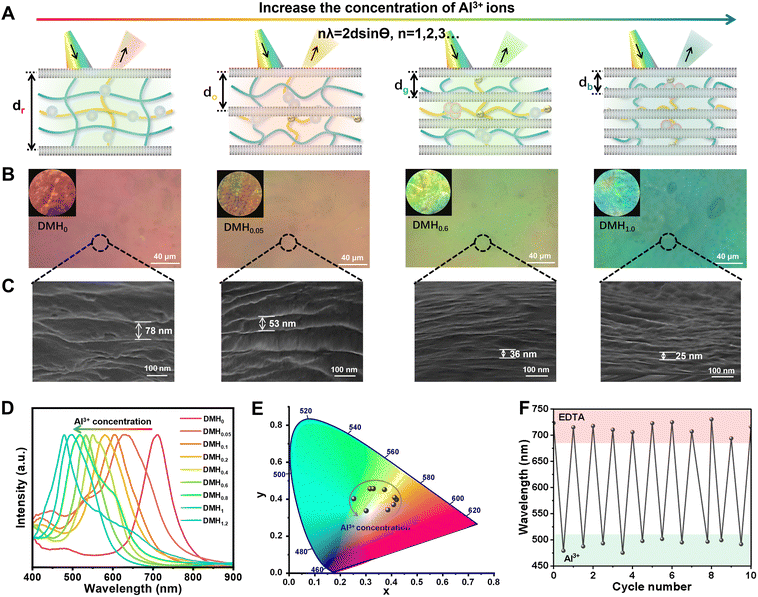 |
| Fig. 2 Structural color of pDGI-PAAm/P(AAc-CDs)-Al3+ hydrogels adjusted by the concentration of Al3+ ions. (A) Schematic of the structural colors and internal structures of the hydrogels with the increase of Al3+ ion concentration. (B) Photographs (inset), reflection optical micrographs and SEM images (C) of DMH0, DMH0.05, DMH0.6 and DMH1.0. Reflection spectra (D) and CIE chromaticity coordinates (E) of DMH0, DMH0.05, DMH0.1, DMH0.2, DMH0.4, DMH0.6, DMH0.8, DMH1.0, and DMH1.2. (F) The changes of the reflection peaks during the process of cyclic Al3+ ion coordination and uncoordination. | |
Controllable regulation of the fluorescent colors of DMHs
In the DMH system, CDs emitting fluorescent color that varied with the aggregation state were synthesized and bonded to the PAAc polymer chains by hydrogen bonding. The introduction of Al3+ ions would change the crosslinking density of the hydrogel network, thus we hypothesized that the aggregation state of the CDs changes. To prove this, the luminous centers of the DMHs before and after coordinating with Al3+ were characterized. Initially, the luminous center of DMH was mainly concentrated at 420–480 nm (DMH0), which red-shifted to 500–600 nm (DMH1.0) after the introduction of Al3+ ions (Fig. 3A and B and Fig. S16, ESI†). It was found that the intensity of the blue emission peak (440 nm) for the dispersed CDs gradually decreased, while the intensity of the red emission peak (640 nm) for the aggregated CDs increased, leading to the fluorescence intensity ratio I440nm/I640nm gradually decreasing from 4.76 to 1.34 and the fluorescence color of DMH changing from blue to pink (Fig. 3C and D). Moreover, the fluorescence lifetime of DMHs showed a gradual weakening trend from 7.54 ns to 3.94 ns (Fig. 3E) and the CIE chromaticity coordinates moved from blue to pink (Fig. 3F) as the concentration of Al3+ ions increased. Notably, the DMHs also have good cyclic properties similar to structural colors (Fig. 3G and Fig. S17, ESI†). As demonstrated in Fig. 3H, the Al3+ coordination brought the PAAc polymer chains closer together, thereby promoting the aggregation of CDs and resulting in a fluorescence color shift from blue to pink.
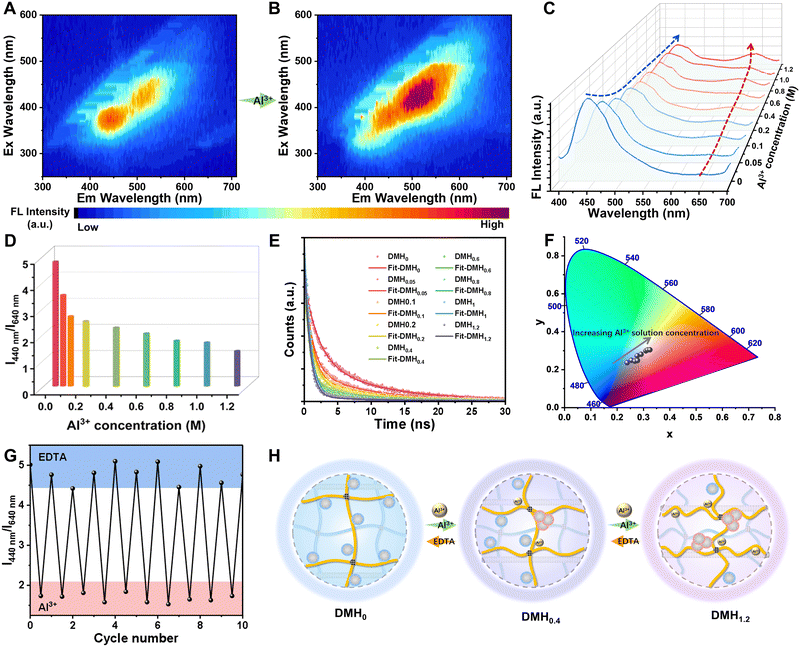 |
| Fig. 3 Fluorescent color of pDGI-PAAm/P(AAc-CDs)-Al3+ hydrogels adjusted by the concentration of Al3+ ions. (A) and (B) PL mapping spectra of DMHs before and after coordinating with Al3+. (C) Fluorescence spectra (λex = 365 nm) of DMHs varying with Al3+ ion concentration. (D) The fluorescence intensity ratio I440nm/I640nm varying with Al3+ ion concentration. The fluorescence lifetime (E) and CIE chromaticity coordinates (F) of the DMHs (DMH0, DMH0.05, DMH0.1, DMH0.2, DMH0.4, DMH0.6, DMH0.8, DMH1.0, and DMH1.2) at 440 nm. (G) The cyclic fluorescent changes of DMH1.0 during the process of cyclic Al3+ ion coordination and uncoordination. (H) The mechanism schematic of fluorescence color variation. | |
Rewritable dual-mode pattern display based on DMHs
As demonstrations of rewritable dual-mode patterns based on DMHs, numbers, patterns and letters were designed. For instance, the “SMART” string (Fig. 4A) could be encoded into different messages such as “MART” and “ART” when treating the corresponding letters with Al3+ solution (1.0 M). Since the introduction of Al3+ ions could change both the structural color and the fluorescent color, the information would be seen in both visible light and ultraviolet light. Similarly, a red flower (Fig. 4B) changed into a colorful flower when treating different parts with distinct concentrations of Al3+ solutions (DMH0.2: yellow, DMH1.0: green), shifting from blue to blue-purple under UV light. When the whole flower was treated with a highly concentrated Al3+ solution (1.0 M), the pattern turned to green. All the above patterns could recover to red (structural color)/blue (fluorescent color) when treated with EDTA solution due to the removal of Al3+ ions, achieving a rewritable dual-mode pattern display. Besides, encoded numbers “2024” and “0831” could be presented successively in dual modes, and the fireworks can change colors by processing the related DMHs with Al3+ solution and EDTA solution (Fig. S18 and S19, ESI†).
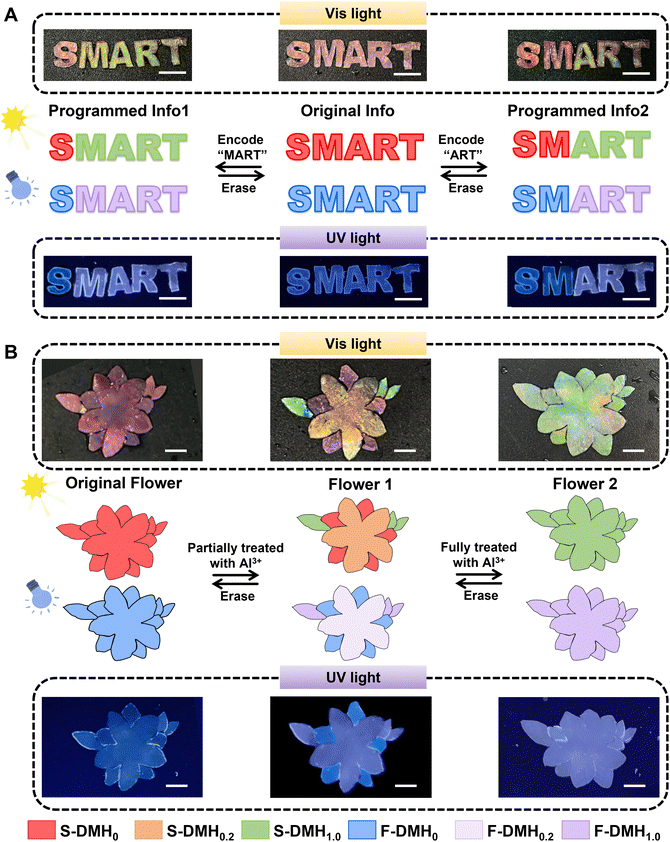 |
| Fig. 4 DMHs for rewritable dual-mode pattern display. (A) A string of letters “SMART” can be encoded into different messages (“MART”, “ART”) by treating different letters with Al3+ ions. (B) A flower that can be transformed into a different color by a designable treatment of aluminum ions with various concentrations. | |
Conclusion
In conclusion, we introduce dynamic metal–ligand coordination into a novel hydrogel-based optical platform, which facilitates rewritable dual-mode information display. The hydrogel system comprises a robust lamellar structure of pDGI and flexible dual networks of PAAm/PAAc embedded with fluorescent CDs. The carboxylic acid groups within the hydrogel can coordinate with metal ions such as Al3+, causing the lamellar structure to contract and CDs to aggregate. This results in a blue shift in structural color and a red shift in fluorescence emission. Furthermore, EDTA is capable of removing Al3+ ions, thereby restoring the initial colors and enabling an erasable dual-mode information display. This innovative approach not only demonstrates the feasibility of creating dynamically responsive optical materials but also opens avenues for developing advanced technologies in rewritable dual-mode information systems.
Author contributions
Hui Shang: methodology, data curation, formal analysis, investigation, writing – original draft. Yu Sun: data curation, supervision. Xiaoxia Le: conceptualization, formal analysis, writing – review & editing, supervision. Ying Shen: data curation, supervision. Tao Chen: supervision, conceptualization.
Data availability
The data supporting this article have been included as part of the ESI.†
Conflicts of interest
There are no conflicts to declare.
Acknowledgements
The authors thanked Prof. Patrick Theato from the Karlsruhe Institute of Technology for his thoughtful suggestions. This work was supported by the National Key R&D Program of China (2022YFB3204300), the National Natural Science Foundation of China (52103246), the Zhejiang Provincial Natural Science Foundation of China (LQ22E030015), the Natural Science Foundation of Ningbo (2023J408, 20221JCGY010301), the Ningbo International Cooperation Project (2023H019), and the Sino-German Mobility Program (M-0424).
Notes and references
- R. Arppe and T. J. Sørensen, Nat. Rev. Chem., 2017, 1, 0031 CrossRef CAS.
- Y. Shen, X. Le, Y. Wu and T. Chen, Chem. Soc. Rev., 2024, 53, 606–623 RSC.
- Y. Sun, X. Le, S. Zhou and T. Chen, Adv. Mater., 2022, 34, 2201262 CrossRef CAS.
- X. Wei, S. X. A. Zhang and L. Sheng, Adv. Mater., 2022, 35, 2208261 CrossRef.
- X. Yu, H. Zhang and J. Yu, Aggregate, 2021, 2, 20–34 CrossRef CAS.
- Y. Ai, Y. Fei, Z. Shu, Y. Zhu, J. Liu and Y. Li, Chem. Eng. J., 2022, 450, 138390 CrossRef CAS.
- H. Deng, H. Wang, Y. Tian, Z. Lin, J. Cui and J. Chen, Mater. Horiz., 2023, 10, 5256–5262 RSC.
- L. Ding and X.-d Wang, J. Am. Chem. Soc., 2020, 142, 13558–13564 CrossRef CAS.
- G. Yin, G. Huo, M. Qi, D. Liu, L. Li, J. Zhou, X. Le, Y. Wang and T. Chen, Adv. Funct. Mater., 2023, 34, 2310043 CrossRef.
- Y. Liu, D. Cheng, B. Wang, J. Yang, Y. Hao, J. Tan, Q. Li and S. Qu, Adv. Mater., 2024, 2403775, DOI:10.1002/adma.202403775.
- Y. Sun, X. Le, H. Shang, Y. Shen, Y. Wu, Q. Liu, P. Théato and T. Chen, Adv. Mater., 2024, 36, 2401589 CrossRef CAS.
- W. C. Xu, C. Liu, S. Liang, D. Zhang, Y. Liu and S. Wu, Adv. Mater., 2022, 34, 2202150 CrossRef CAS PubMed.
- Y. Gao, K. Ge, Z. Zhang, Z. Li, S. Hu, H. Ji, M. Li and H. Feng, Adv. Sci., 2024, 11, 2305876 CrossRef CAS.
- Y. Zhao, X. Zhao, M. D. Li, Z. A. Li, H. Peng and X. Xie, Angew. Chem., Int. Ed., 2020, 59, 10066–10072 CrossRef CAS PubMed.
- Z.-H. Zuo, Z.-W. Feng, Y.-Y. Peng, Y. Su, Z.-Q. Liu, G. Li, Y. Yin and Y. Chen, ACS Nano, 2024, 18, 4456–4466 CrossRef CAS PubMed.
- J.-H. Tian, H. Xu, X.-Y. Hu and D.-S. Guo, Supramol. Mater., 2024, 3, 100063 Search PubMed.
- L. Qin, X. Liu, K. He, G. Yu, H. Yuan, M. Xu, F. Li and Y. Yu, Nat. Commun., 2021, 12, 699 CrossRef CAS.
- J. Zhang, S. Qin, S. Zhang, C. Sun, Y. Ren, L. Zhang, J. Liu, J. Xiao, W. Hu, H. Yang and D. Yang, Adv. Mater., 2023, 2305872, DOI:10.1002/adma.202305872.
- S. Chen, K. Zhang, Z. Li, Y. Wu, B. Zhu and J. Zhu, Supramol. Mater., 2023, 2, 100032 Search PubMed.
- M. Wu, L. Han, B. Yan and H. Zeng, Supramol. Mater., 2023, 2, 100045 Search PubMed.
- S. Lai, Y. Jin, L. Shi, R. Zhou and Y. Li, ACS Appl. Mater. Interfaces, 2022, 14, 47113–47125 CrossRef CAS.
- D. Lou, Y. Sun, J. Li, Y. Zheng, Z. Zhou, J. Yang, C. Pan, Z. Zheng, X. Chen and W. Liu, Angew. Chem., Int. Ed., 2022, 61, e202117066 CrossRef CAS PubMed.
- H. Shang, X. Le, Y. Sun, S. Wu, Y. Wang, P. Théato and T. Chen, Mater. Horiz., 2024, 11, 2856–2864 RSC.
- Q. Wang, Z. Qi, Q. M. Wang, M. Chen, B. Lin and D. H. Qu, Adv. Funct. Mater., 2022, 32, 2208865 CrossRef CAS.
- J. Xue, Y. Wang, T. Zhang, K. Li, F. Vogelbacher, Y. Zhang, X. Hou, Z. Zhu, Y. Tian, Y. Song and M. Li, Sci. China: Chem., 2023, 66, 3567–3575 CrossRef CAS.
- W. Zhao, B. Wu, Z. Lei and P. Wu, Angew. Chem., Int. Ed., 2024, 63, e202400531 CrossRef CAS PubMed.
- S. Wu, W. Li, Y. Sun, X. Zhang, J. Zhuang, H. Hu, B. Lei, C. Hu and Y. Liu, J. Colloid Interface Sci., 2019, 555, 607–614 CrossRef CAS.
- S. Deng, R. Deng, X. Mao, B. Xiong, M. Wang, S. Chen, W. H. Binder, J. Zhu and Z. Yang, CCS Chem., 2023, 5, 2301–2311 CrossRef CAS.
- P. H. Michels-Brito, V. Dudko, D. Wagner, P. Markus, G. Papastavrou, L. Michels, J. Breu and J. O. Fossum, Sci. Adv., 2022, eabl8147 CrossRef.
- Z. Zhang, Z. Chen, Y. Wang, Y. Zhao and L. Shang, Adv. Funct. Mater., 2021, 32, 2107242 CrossRef.
|
This journal is © The Royal Society of Chemistry 2024 |
Click here to see how this site uses Cookies. View our privacy policy here.