DOI:
10.1039/D3MA01150J
(Review Article)
Mater. Adv., 2024,
5, 3094-3112
Application of biodegradable microsphere injections: an anticancer perspective
Received
20th December 2023
, Accepted 22nd February 2024
First published on 26th February 2024
Abstract
Long-acting injections have outstanding contributions in prolonging the release period of drugs, reducing the frequency of administration, and improving the compliance of patients. Various long-acting schemes have been extensively studied, such as oil-based formulations, drug crystal suspensions, in situ forming implants, and microspheres. Among them, microspheres can load drug molecules with different properties through a variety of pathways, which have excellent prospects in drug delivery, especially as anticarcinogens. We have briefly summarized common biodegradable carrier materials for microsphere injections and their utilization in biological medicine, including natural, synthetic, and inorganic materials. Next, we have focused on deliberating the application of biodegradable microsphere injections in cancer therapy through marketed microspheres, clinical research, and experimental studies. At present, the use of microspheres in the treatment of cancers, such as prostate cancer, breast cancer, uterine fibroids, neuroendocrine neoplasm, and liver cancer, has been well-established. Finally, technical challenges and process difficulties in developing injectable microsphere products and viable solutions are briefly discussed. Microspheres have shown enormous potential in delivering anticancer drugs, and we believe that more types of microspheres will be used for cancer therapy in the future.
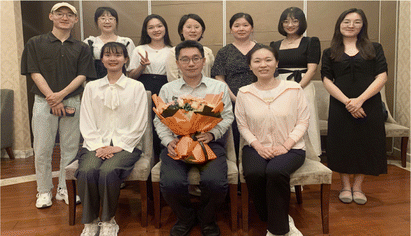 Jiyong Liu research group | Our supervisor is Professor Jiyong Liu, current director of the Department of Pharmacy and doctoral supervisor of Fudan University Shanghai Cancer Center. Professor Liu is a Shanghai Leading Talent and Shanghai Academic Research Leader. Our main research directions are new drug delivery systems based on clinical demand orientation and clinical pharmacy, novel anti-tumor nano drug preparations and technologies, and clinical rational drug use. As a project leader, Professor Liu has presided over 6 projects funded by the National Natural Science Foundation of China and more than 20 research projects at the provincial and ministerial levels. As the main investigator, Professor Liu has won the second prize in the National Science and Technology Progress Award and the first prize in the Science and Technology Progress Award for Higher Education Institutions of the Ministry of Education. We published a total of 156 research papers, including 77 SCI papers. In addition, Professor Liu has served as an associate editor of Cancer Screening and Prevention and an associate editor of the Chinese edition of Nature Review Drug Discovery. |
1 Introduction
Injections are widely used in clinical practice because of the advantages of rapid onset and reliable action, but their rapid degradation in vivo causes drug concentration to quickly drop below the threshold, requiring repeated injections to maintain the therapeutic level. This can seriously affect the compliance of patients, especially in chronic diseases; thus, more long-acting injections are urgently needed. Several types of long-acting injections have been used in clinical practice, such as oil-based long-acting parenteral formulations, drug crystal suspensions with the highest drug-loaded carrier system, in situ forming implants with easy scale-up, and microspheres with low risk of mechanical tissue irritation. The comparison of four popular long-acting injections is shown in Table 1. Among them, we believe that long-acting microsphere injections deserve more attention. Although microspheres are expensive, they can maximize the release of active pharmaceutical ingredients (APIs). Encapsulated APIs can be slowly released along with the biodegradation of microsphere carriers to achieve long-term and continuous treatment ranging from weeks to months. The rate of API release is mainly determined by biodegradable carrier materials that have high biocompatibility and safety. Biodegradable carrier materials can be slowly degraded via normal metabolism; thus, they will not cause damage to the body. Biodegradable microsphere injections can eliminate the peak and trough phenomena of drug concentration produced by multiple administrations and constantly release drugs to obtain the effective concentration of stabilization for a long time. In addition, microsphere preparations have an affinity for tissues and selectivity for special sites, which enhances drug targeting and reduces toxic side effects. Biodegradable microsphere injections could mainly load small molecule drugs in the past but research has been extended now to the delivery of nucleic acids, peptides, proteins, and other drugs, including hydrophobic and hydrophilic drugs.1
Table 1 The comparison of four popular long-acting injections
Type |
Release principle |
Advantages |
Disadvantages |
Representative drug |
Cycle/months |
Safety |
Ref. |
Oil-based long-acting parenteral formulations |
Prodrug containing fatty acid chains is released slowly by the gradual hydrolysis of ester bonds or by the slow entry from adipose tissue into the blood circulation. |
Low cost |
Some drugs are not suitable for linking fatty acid chains. |
Faslodex® |
1 |
Lower |
2 and 3
|
Suspensions |
The suspension is mainly controlled by the dissolution kinetic of local interstitial fluid and drug crystal surface area. |
High drug load |
API particles have rough surfaces; the size of API particles is not easy to control; strong organizational stimulation. |
Invega Hafyera ™ |
6 |
Low |
2–4
|
In situ forming implants |
The preparation is injected as an aqueous solution, solidifies at the injection site to form a gel, and API is slowly released as the gel degrades. |
Drug release can be regulated to some extent; easy industrial scale-up. |
Limitations on the use of organic solvents; API release options are limited; low drug loading. |
Leuprone Hexal® |
3 |
Higher |
2 and 3
|
Microspheres |
The skeleton material gradually hydrolyzed and dissolved, and the API was slowly released from the microsphere matrix. |
API release can be adjusted; hydrophilic and hydrophobic drugs can be included; smooth and soft surfaces allow for low risk of mechanical tissue irritation. |
High cost; low drug loading. |
Lupron® depot |
6 |
Highest |
2 and 3
|
Microspheres were used in the 1990s and now have been widely used in the treatment of a number of diseases (Table 2), including digestive system diseases, metabolic diseases,5 nervous system diseases,6 and cancer. For cancer, microspheres have more applications, especially for delivering peptides. Microsphere preparations of leuprolide, triptorelin, octreotide, lanreotide and pasireotide have shown good efficacy in the treatment of hormone-dependent tumors, including prostate cancer, breast cancer, uterine fibroids, and neuroendocrine neoplasm.
Table 2 Commercial availability of microsphere injections
Trade name |
API |
Materials |
Formulations/months |
Route |
Firm |
Indications |
Data were obtained from Drugs@FDA search. Abbreviation: PLGA, poly(lactic-co-glycolic acid); PLA, polylactic acid; CMC, carboxymethyl cellulose; I.M, intramuscular; S.C, subcutaneous; IAT, intra articular injection. |
Lupron®Depot |
Leuprolide |
PLGA/PLA |
1,3,4,6 |
I.M |
Takeda, abbott |
Prostate cancer, breast cancer, uterine fibroid, etc. |
Decapepty®Depot |
Triptorelin |
PLGA/PLA |
1,3,6 |
I.M |
Ferring |
Prostate cancer, breast cancer, endometriosis, pubertas praecox, etc. |
Decapepty®SR |
Triptorelin |
PLGA |
1,3,6 |
I.M |
Ipsen |
Prostate cancer, breast cancer, endometriosis, pubertas praecox, etc. |
Trelstar® |
Triptorelin |
PLGA |
1,3,6 |
I.M |
Debiopharm |
Prostate cancer, uterine fibroid, etc. |
Sandostati® |
Octreotide |
PLGA |
1 |
I.M |
Novartis |
Acromegaly, neuroendocrine neoplasm, carcinoid syndrome, etc. |
Somatuline®Depot |
Lanreotide |
PLGA |
1 |
S.C |
Ipsen |
Acromegaly, neuroendocrine neoplasm, carcinoid syndrome, etc. |
Signifor®LAR |
Pasireotide |
PLGA |
1 |
I.M |
Novartis |
Acromegaly, hypophysoma, cushing's syndrome, etc. |
Plenaxis® |
Abarelix |
CMC |
1 |
I.M |
Praecis |
Prostate cancer |
Nutropin®Depot |
Somatropin |
PLGA |
1 |
S.C |
Alkermes, genentech |
Growth retardation |
Risperdal®Consta™ |
Risperidone |
PLGA |
0.5 |
I.M |
Johnson |
Schizophrenia, bipolar disorder, schizophrenia, etc. |
Bydureon® |
Exenatide |
PLGA |
0.25 |
S.C |
AstraZeneca |
Diabetes |
Vivitrol® |
Naltrexone |
PLGA |
1 |
I.M |
Alkermes, Cephalon |
Alcohol or opioid dependence |
Zilretta® |
Triamcinolone |
PLGA |
3 |
IAT |
FLXN |
Knee osteoarthritis |
This review presents the biodegradable carrier materials of microsphere injections and their applications in drug delivery. The specific application of microsphere injections in the delivery of anticancer drugs was analyzed from marketed microspheres, clinical research, and experimental studies, including common malignant tumors such as prostate cancer, breast cancer, liver cancer, lung cancer, glioblastoma multiform and pancreatic adenocarcinoma, as well as some benign tumors such as neuroendocrine neoplasm and uterine fibroids. The difficulties to be overcome in the preparation and production of microsphere injections (such as initial burst release, the stability of peptide and protein, and the expansion of production) as well as possible solutions are discussed at the end. It is hoped that this review can provide a reference to the researchers who want to develop new drugs for microsphere injection.
2 Biodegradable carrier materials for microsphere injections
The biodegradable carrier materials for preparing microsphere injections are mainly polymer materials with good biocompatibility properties, including natural, synthetic, and inorganic. Synthetic materials are the most used, and their structures are easily modified and suitable for the preparation of multifunctional microspheres with complex structures. Natural materials are widely available, inexpensive, and most of them have adhesive properties, which are suitable for the preparation of hydrogel microspheres. Many researchers have applied it to embolization therapy and osteosarcoma. The utilization of inorganic materials can enhance the targeting and delivery efficiency of growth factors, making them suitable for the preparation of composite microspheres with other carrier materials. The characteristics and application examples of biodegradable carrier materials for microsphere injections are shown in Table 3.
Table 3 Characteristics and application examples of biodegradable carrier materials for microsphere injections
Carrier material |
Feature |
Application |
Biological function |
Ref. |
PLA |
Sustained release, surface modification |
Malignant melanoma |
They can inhibit tumor growth and prolong the survival time of tumor-bearing mouse, which has no obvious toxic and side effects on major organs. |
7
|
PLGA |
Sustained release, structural modification |
Hepatocellular carcinoma |
They are designed to participate in HCC immunoregulatory therapy and tumor starvation therapy. They can reverse the immunosuppressive tumor microenvironment, which can be combined with PD-L1 checkpoint inhibition by co-delivery. |
8
|
Gelatin |
Adhesiveness, cell delivery and culture embolization |
Breast cancer |
The porous gelatin hydrogel microspheres exhibited increased cell loading and viability, resulting in significant promotion of adipose tissue formation and simultaneously inhibited tumor recurrence. |
9
|
Alginate |
Adhesiveness, embolization, pH-responsive, anionic material |
Hepatocellular carcinoma |
IDO1 inhibitor-loaded alginate microspheres have been shown to be effective in the treatment of HCC by radioactive seed implantation in mice and transarterial radiation therapy embolization in rabbits. |
10
|
Chitosan |
Adhesiveness, pH-responsive, cationic material |
Colorectal carcinoma |
They displayed enhanced cytotoxicity and proapoptotic activity against HCT-116 colon cancer cells as well as improved the colon targeting efficiency. |
11
|
Starch |
Embolization |
Hepatocellular carcinoma |
Degradable starch microspheres provide relatively transient embolization before being degraded by serum amylases. |
12
|
Fe3O4 |
Magnetic targeting, magnetic resonance imaging |
Laryngeal carcinoma |
The Fe3O4 can not only increase the stimulation of mechanical force to cells but also improve the photothermal conversion ability under near-infrared light because of surface plasmon resonance. |
13
|
CaCO3 |
pH-responsive |
Cervical carcinoma |
Because of the inherently good biocompatibility, pH sensitivity, and biodegradability of CaCO3, they can release the DOX in a controlled and sustained manner in response to the weak acidic microenvironment of cancer cells. |
14
|
Calcium phosphate-based biomaterials |
pH-responsive, promote bone defect repair |
Osteosarcoma |
They show excellent properties for promoting the osteogenic differentiation of human bone mesenchymal stem cell |
15
|
2.1 Synthetic polymer carrier material
2.1.1 PLA.
Polylactic acid (PLA) is a high molecular polymer obtained by the polymerization of lactic acid fermented by plant starch. In vivo, PLA is eventually hydrolyzed into CO2 and H2O; thus, it is not toxic to humans. As early as the 1960s, PLA was used in clinical practice as a surgical suture due to its good biodegradable properties. PLA microspheres can not only prolong the duration of drug release but also significantly improve the drug encapsulation efficiency. Compared with the micellar group (about 200 h), the PLA microsphere group (about 750 h) loaded with rapamycin for treating breast cancer shows a significant improvement in drug release time, and the encapsulation efficiency is about 83%.16
Moreover, the PLA microsphere surface can be modified to improve its performance.17–19 For example, a study20 showed that acidic-cleavable acetal bonds can be introduced into the PLA microsphere skeleton to achieve pH-sensitivity and targeting (Fig. 1). According to Fig. 1c, the modified PLA microspheres have the most cumulative release under acidic conditions. According to Fig. 1d, the modified PLA microspheres were injected intratumorally into the mouse model of breast cancer, and the tumor volume was significantly lower than that in the control group measured after 21 days of administration (3.4 V vs. 10.5 V, V is the average tumor volume before treatment). The above results fully demonstrated that the modified pH-sensitive PLA microspheres could improve the anticancer effect. Neda Rostami et al.21 synthesized (PLA–PEG–PLA) and (PEG–PLA–PEG) and showed by molecular dynamics simulations and free energy analysis that PLA–PEG–PLA has a higher tendency to interact with the cell membrane and more negative free energy, indicating that PLA–PEG–PLA is a better carrier for cell membrane penetration. Furthermore, PLA–PEG–PLA exhibited higher drug loading and more controlled release rates, leading to greater cell death in MCF-7 breast cancer cells, probably because of the stronger hydrophobicity of PLA–PEG–PLA than PEG–PLA–PEG.
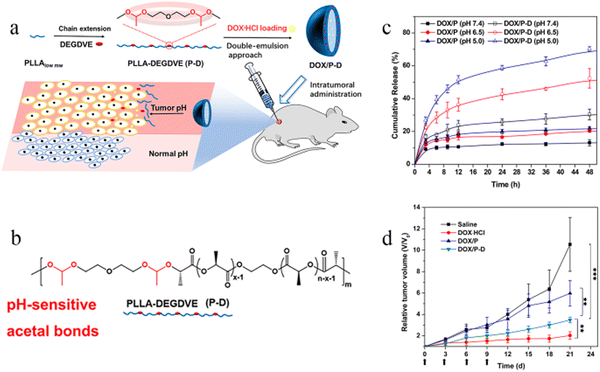 |
| Fig. 1 Modification and antitumor efficacy of pH-responsive PLA microspheres. (a) pH-sensitive DOX-loaded polymer microspheres prepared by the double-emulsion method and intratumorally administrated for localized chemotherapy. (b) Synthesis of PLLA-DEGDVE (P-D) with pH-sensitive acetal bonds (red color). (c) In vitro drug release of DOX-loaded microspheres (n = 3). (d) Relative tumor volume changes in cancer therapy (n = 12). Reproduced from ref. 20 with permission from American Chemical Society, copyright 2018. | |
2.1.2 PLGA.
PLGA is a polymeric compound obtained from the two monomers of lactic acid and glycolic acid according to a certain proportion.22 Unlike PLA aggregated by a monomer, PLGA not only has the rigidity, hydrophobicity and slow degradation of LA but also has the ductility, relative hydrophilicity, and faster degradation of GA.23 The significant advantage of PLGA as a microsphere carrier material is that it can modify the polymer by adjusting the ratio of two monomers, polymer molecular weight, concentration, and end groups during the synthesis process, which can regulate the encapsulation efficiency and drug release.24 There are many methods to prepare PLGA microspheres, including emulsification, microfluidic,25 electrospray26 and spray drying method.27
The extensive application of PLGA in biomedical fields includes drug delivery, tissue engineering, artificial catheter, and wound suturing. More than 32 PLGA microspheres have been approved by the FDA for small molecule drug delivery, and the number of biological macromolecule drugs is also on the rise.28 In the last decade, PLGA combined with nanotechnology have been widely explored.29 Published articles have shown that PLGA microspheres have become ideal materials for multiple drugs due to their various advantages, including small molecule drugs, vaccines,30 protein,31 metal,32 and magnetic nanoparticles.33
The main purpose of drug delivery with PLGA microsphere is to prolong the duration of drug action. For example, Birgitta et al.34 prepared bupivacaine into PLGA microsphere injection and achieved the sustained release of API in vivo. The results showed that the analgesia time in the microsphere group was significantly longer than that in the solution group, which contributed to long-term postoperative analgesia.
In addition to long-acting, PLGA microspheres can also improve the safety of drugs. The PLGA microspheres loaded with arteannuin B can effectively inhibit the growth of the tumor.35 The relative tumor proliferation rate is less than 40%, and only weekly subcutaneous injection achieved the continuous release of API for 21 days. Furthermore, the index of organ, organ staining, and staining of tumor cells indicated that this microsphere formulation was safer than cis-platinum diamine–dichloride injection; thus, it might be the first microsphere to deliver small molecule antitumor drugs.
2.2 Natural polymer carrier materials
2.2.1 Gelatin.
Gelatin, which is produced by the degradation of collagen in connective tissues such as animal skin or bone, does not express antigens in the body and is therefore safe. The preparation methods of gelatin microspheres include emulsification method,36 condensation method37 and spray drying method.38 Gelatin microspheres can be used for tumor embolization therapy,39 which can be injected into the designated blood vessels to block the nutrient supply of the tumor.
Gelatin has certain adhesive properties, which forms a clear and transparent solution in hot water and becomes a gel after cooling. Therefore, gelatin can be used to prepare biodegradable hydrogel microspheres. For example, Kaili Chen et al.40 have constructed a gelatin microsphere loaded with ebselen liposomes with excellent viscosity and coupled to the polydopamine layer (GM@PDA@Lipo-Ebselen), which adheres tightly to the target tissue even after 180° inversion and 360° rotation. Yu et al.41 prepared the gelatin microsphere carrying losartan by the emulsification method and successfully demonstrated that it could inhibit the synthesis of tumor collagen. Collagen fibers in tumors can reduce the permeability of drugs to the tumor tissue, which can hinder the anti-tumor effects of drugs. The results provide important guidelines for a long-acting local delivery system of collagen inhibitors.
2.2.2 Alginate.
Alginate is a natural polyuronic acid in the cytoderm of alga. Alginate microspheres not only have the characteristics of good water-solubility, easy gel formation, simple preparation, and low price, but also have high safety because they can be degraded into simple sugars for reuse in vivo. Alginate microspheres can be prepared by emulsification, extrusion, spray drying and microfluidic technology,42 W/O single emulsification is the most generally used method.43
Alginate microspheres have excellent pH sensitivity, which can be targeted to the special microenvironment of tumors. Studies designated that alginate microspheres showed the pH-responsive swelling behavior at pH values of 2.0, 4.5, 7.4, and 8.5, with percentages of 10.60%, 352.65%, 690.03%, and 1211.46%, respectively.44 The release rate of the microspheres showed an increasing trend proportional to pH.
Alginate microspheres also have better therapeutic effects in tumor embolization therapy. A vascular embolic agent made of sodium alginate microspheres was approved by the China Food and Drug Administration in 2019. This product is suitable for interventional embolization therapy of various solid tumors, and it is the only natural, degradable, safe, non-toxic, and environment-friendly vascular embolization agent commercially available in the world.
In addition, the content of alginate could determine the size of the microspheres. Jia Man et al.45 used microfluidic methods to prepare calcium alginate microspheres as histone deacetylase inhibitor carriers. The study showed that when the flow rate ratio was fixed, the size of the CAM decreased as the concentration of the alginate decreased.
2.2.3 Chitosan.
Chitosan is a kind of natural polymer polysaccharide extracted from marine crustaceans, which is insoluble in water and most organic solvents.46 Due to the positive charge of chitosan, chitosan microspheres can be formed by ionic crosslinking.47,48 The advantages of chitosan are its biological adhesion and strong permeability,49 which can be used for embolization therapy. The injection of 131I-labeled chitosan–collagen composite microspheres through hepatic arteries into hepatocellular carcinoma rats which effectively increased the overall survival of the intervening sites from 19 days to 44 days.50
It has been reported that chitosan microspheres can deliver traditional Chinese medicine.51 They prepared chitosan microspheres by the spray drying method using panax notoginseng, Dangshen and atractylodes extracts as API. The release behavior in vitro of API occurs in two phases, with 41% API being released within the first hour to quickly reach the therapeutic concentration and the remaining API being sustainedly released within 12 hours to maintain the plasma concentration.
2.2.4 Starch.
Starch microspheres can be made from natural starch under the action of initiator and crosslinker and are safe carrier materials because they can be degraded by amylase in vivo.52 Starch microspheres have a good slow-release effect. Yashar Hassanpouraghdam et al. compared the release rate of starch microspheres (St@5-Fu@GQDs@Bio-MOF) with that of non-starch microspheres (5-Fu@GQDs@Bio-MOF) and found that the release of API in starch microspheres was more sustained. This behavior can be explained by the entrapping of the API in the cross-linked starch gel-like network.53
Degradable starch microspheres are often used in tumor embolization therapy.54 Results from a European multicenter survey55 showed that 121 patients with HCC had a median survival time of 15.5 months, a median time to tumor progression of 9.5 months and a disease control rate of 83.2%. Starch microsphere embolization is a good option when other standard treatments are ineffective.
2.3 Inorganic materials
2.3.1 Fe3O4.
Fe3O4 is usually combined with other polymer carrier materials to make magnetic microspheres. Some researchers have conducted detailed studies on the biodegradation process of Fe3O4 nanoparticles in vivo.56 Partial results are shown in Fig. 2, where Fe3O4 nanoparticles are first adsorbed to the cell membrane (Fig. 2a) and subsequently fused with lysosomes and then slowly degraded (Fig. 2c and d) by vesicular transport (Fig. 2b). Finally, the Fe3O4 nanoparticles clearly segregate into membrane and protein-rich regions, suggesting that their biotransformation occurs in these regions (Fig. 2e and f). The remarkable feature of magnetic microspheres is targeting, which can be enriched in target organs and tissues according to the action of an external magnetic field. The work of Ayanaar et al.57 described the formation of iron nanoparticles using an extract of watermelon rind as a reductant/surfactant. They fabricated multifunctional magnetically-targeted microspheres for cancer treatment using a double-emulsion (W1/O/W2) method with Fe3O4@lecithin nanoparticles (Fe3O4@LEC NP) as the inner aqueous phase (W1), curcumin (CUR), which an antioxidant drug and PLGA as the middle oil phase (O), and PEG as the outer aqueous phase (W2) (Fe3O4@LEC-CUR-PLGA-MMS). The cytotoxicity test proved its cytotoxicity against A549 and HeLa S3 cells with IC50 values after 24 h of 10 and 12 μg mL−1 and 10 and 20 μg mL−1, respectively. This study was a well-established evidence of the important role of Fe3O4 microspheres in the delivery of anticancer drugs by magnetic targeting.
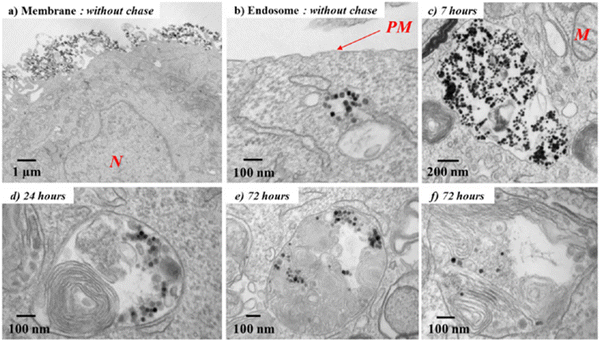 |
| Fig. 2 TEM micrographs of the biodegradation process of Fe3O4 nanoparticles after entry into cancer cells. (a) After the incubation (without chase), the nanotubes are still present on the plasma membrane or (b) already internalized into early endosomes with a clear background. (c) On increasing the chase period, nanotubes are confined in the endosomes in the aggregated form and then (d) localize within heterolysosomes, which are electron dense and are characterized by numerous multilamellar bodies and high-density proteins. (e) and (f) Nanoparticles appear increasingly dispersed within the lysosomes and therefore start degrading (N, nucleus; M, mitochondria; PM, plasma membrane). Reproduced from ref. 56 with permission from American Chemical Society, copyright 2013. | |
In the other study,58 bovine serum albumin (BSA) was successfully loaded into the magnetic chitosan microsphere through ion electrostatic adsorption, which obtained high drug loading and drug release rates of 947.01 mg g−1 and 87.8%, respectively. At present, there is no protein-loaded microsphere for sale, and a recombinant human growth hormone (rhGH) microsphere has been withdrawn from the market due to commercial problems. Thus, this study is a good example of the feasibility of Fe3O4 microspheres to load protein drugs.
2.3.2 CaCO3.
CaCO3 is well known as the main component of bone and is used as delivery carrier due to its good bioactivity.59 CaCO3 mainly exists in three crystal forms, namely, calcite, vaterite, and aragonite, among which calcite is the most stable, while vaterite is extremely unstable. Therefore, we need to ensure the stability of CaCO3 microspheres in the preparation process, and gelatin can solve this problem very well. Researchers added gelatin to the porous CaCO3 microsphere loaded with doxorubicin (DOX), and then FTIR absorption spectroscopy, differential scanning calorimetry and X-ray diffraction analysis confirmed that all the vaterite in the microspheres changed into a stable calcite.60
CaCO3 microspheres can target the tumor microenvironment due to the pH sensitivity. Yan et al.61 showed that the final cumulative release rate of DOX in the CaCO3 microsphere was 95% at pH = 4.8, significantly higher than the 52% at pH = 7. A study dedicated to the development of dual delivered microspheres showed that CaCO3 microspheres can also deliver growth factors.62 CaCO3 microspheres were first co-functionalized by casein and heparin before loading with BMP-2 and finally injected in the hydrogel form into the rabbit model of tibial fracture to study the drug delivery capacity (Fig. 3). In this drug-delivery system, the presence of CaCO3 significantly increased the tensile strength. Casein has a strong affinity to calcium ions to stabilize the structure of microspheres. Heparin can efficiently bind positively charged growth factors through negatively charged sulfate groups, thereby improving the drug delivery ability. In vitro results from Micro-CT analysis showed that more new generated bone tissue was present at day 60 than at day 30, especially in the FC-B group.
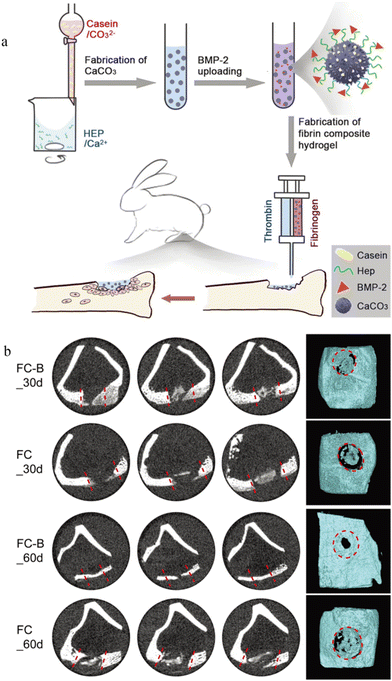 |
| Fig. 3 Application of CaCO3 microspheres in bone repair. (a) Application of the BMP-2 loaded CaCO3/fibrin-glue composite hydrogel to repair tibia defects in rabbits. Casein and heparin co-functionalized CaCO3 microspheres were prepared; BMP-2 was loaded onto microspheres; the CaCO3/fibrin-glue composite hydrogel was fabricated and can be injected into defect sites; after implantation, cells migrated from the surrounding tissues to repair bone defects. (b) Micro-CT analyses of bone tissues with defects after implantation with CaCO3/fibrin-glue composite hydrogels (FC-B and FC) for 30 and 60 days. The dotted red line/circles indicate the defect area. Reproduced from ref. 62 with permission from the Royal Society of Chemistry, copyright 2019. | |
2.3.3 Calcium phosphate-based biomaterials.
Calcium phosphate-based biomaterials (CPBMs) mainly refer to compounds containing calcium and phosphorus, including hydroxyapatite, tricalcium phosphate and bipolar calcium phosphate (BCP). The latter is composed of the former two and exhibits better biological activity and osteoconductivity; thus, BCP is the most widely used. CPBMs can be used for bone repair and bone regeneration in the later stage of bone cancer treatment because of their excellent mobility and injectability. Microspheres can fill into complex and irregular defective bones and increase the synthesis of osteopontin and alkaline phosphatase to enhance bone induction and bone formation.63 It has been shown that composite porous microspheres prepared from bidirectional calcium phosphate-collagen can be used to deliver the growth factor.64 After injecting the microsphere to the bone defect of the rabbit model for 8 weeks, the μ-CT imaging clearly showed that almost the entire defect part is filled by the new bone. Quantitative analysis revealed that this composite microsphere showed the highest coverage of the new bone of about 76% compared to the other groups. This study demonstrated that BCP microspheres could be successfully used for the delivery of bone morphogenic protein-2, which a human growth factor.
2.4 Composite materials
When preparing the microsphere injections, there are many alternative carrier materials. The most selected is the artificial synthetic material PLA and PLGA. Because each carrier material has its own limitations, many researchers now like to mix various types of carrier materials. Composite microspheres can make full use of the strengths of various carrier materials and avoid shortcomings to achieve multi-functionalization. Researchers have proposed that calcium alginate can significantly reduce the problem of initial burst release of PLGA microspheres.65 In another study, Chen et al. designed a hydrogel microsphere multifunctional drug delivery system (DDS) loaded with tetracycline hydrochloride and flamazine and using Fe3O4, hydroxyapatite, gelatin, chitosan, and hydrogel as carrier materials. In this injectable microsphere DDS, Fe3O4 plays the role of magnetic targeting, hydroxyapatite is involved in bone conduction, gelatin provides better solubility, and chitosan and hydrogel provide gelatinization. The combination of various carrier materials makes the delivery system injectable, targeting and self-healing.66
3 Application of microsphere injections in anticancer drug delivery
3.1 Preclinical study on the application of microspheres in cancer
3.1.1 Glioblastoma multiforme.
Glioblastoma multiforme (GMB) accounts for almost 80% of all malignant brain tumors, which is the most aggressive primary brain tumor.67 With the extremely poor therapeutic prognosis and low survival rate of GBM, one of the important reasons for this outcome may be the presence of the blood brain barrier (BBB). An effective solution is the targeted local injection of drug bearing microspheres, which can not only avoid BBB but also achieve the long-term continuous drug release to the brain tumor site. For example, some researchers68 encapsulated vincristine (VCR) in magnetic albumin (MAMbs) and then conjugated anti-CD133 monoclonal antibody (CD133mAb) on the surface (CD133mAb/TMAMbs). The results showed that this DDS could specifically bind to CD133 on the cell membrane of U251 glioblastoma cells and that VCR could exert a strong anti-tumor proliferation effect after being delivered into the cancer cells (Fig. 4).
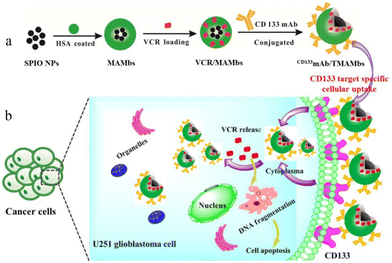 |
| Fig. 4 Schematic illustration of the preparation and effect of CD133mAb/TMAMbs for treating glioblastoma. (a) Process of preparation. (b) Mechanisms that act within cancer cells. (SPIO NPs: superparamagnetic iron oxide nanoparticles; HSA: human serum albumin; MAMbs: magnetic albumin microbeads; VCR: vincristine; CD133mAb: anti-CD133 monoclonal antibody). Reproduced from ref. 68 with permission from American Chemical Society, copyright 2019. | |
Mitoxantrone has a strong glioblastoma multiforme cells activity in vitro, but the poor penetration of the central system causes it to be limited. It can be wrapped into microspheres and repeatedly injected into the tumor area to provide an effective and sustained local drug concentration. The investigators have experimentally demonstrated that this method can significantly inhibit the growth of malignant glioblastoma.69 The tumor volume was about 17 mm3 after intratumoral injection a week, which was significantly lower than the 76 mm3 found in the blank microsphere group. Later, researchers conducted more detailed studies on PLGA microspheres loaded with imatinib mesylate and injected them subcutaneously into human (U87-MG) and murine (GL261) glioma tumors, which reduced the tumor volume by 88% and 79%, respectively. They also conducted a further study of the intracranial injection of the drug-loaded microspheres, which showed that a single injection reduced the tumor volume by 79%.70 This study further demonstrates the feasibility of using microspheres to deliver antibrain tumor drugs. Another study demonstrated that radioactive microspheres can also be used to treat brain tumors. A case71 reported the study of the treatment of canine pituitary tumor in the treatment of radioactive Ho166 microspheres. The results showed that the total amount of tumor volume was reduced by 40% after treatment. This is the first time that intracranial tumor treatment is performed through the acupuncture injection of radioactive microspheres.
3.1.2 Pancreatic adenocarcinoma.
Since gemcitabine was approved by the FDA in 1996, various chemotherapy regimens have been proposed,72 but these regimens have problems of rapid systemic clearance, chemoresistance and poor survival.73 To address this issue, chemotherapeutic drugs can be encapsulated in microspheres and then be delivered to the designated parts.74 In recent years, studies have shown that drug-loaded microspheres coupled to tumor antigen-related antibodies can improve the targeting of the microsphere delivery system, prevent the toxic effects of anticancer drugs on normal cells, and improve the therapeutic index of tumors.75 For example, the incorporation of anti-EGFR antibody and chitosan antibody on gemcitabine chitosan microspheres can form a nanoscale targeted microsphere delivery system for pancreatic cancer.76 Compared with the control group, the attached antibody drug bearing microspheres group had a significant inhibitory on pancreatic cancer cells (SW1990), and the inhibition rate was higher than 60%. This trial embodies the potential of a multifunctional microsphere in the treatment of pancreatic cancer. In this DDS, anti-EGFR antibodies can deliver microspheres to the surface of pancreatic adenocarcinoma cells because EGFR is highly expressed among pancreatic cancer cells. The physiological pH of the human is above 7, and chitosan is degraded at the pH below 6.5. Therefore, the drug concentration in the tumor cells is very high and the impact on normal cells is small, which will help reduce the side effects of the drug. In addition, the cell membrane is negatively charged, but the chitosan belt is positive, which helps the microspheres to penetrate the cancer cell membrane and improve the efficacy.
Another study77 demonstrated the feasibility of microspheres, which loaded immunotherapy agents for pancreatic ductal adenocarcinoma. The experiments proved that the intratumoral injection of the PLA microsphere encapsulated with intratumoral interleukin-12 (IL-12) in KCKO tumor-bearing mice after stereotactic body radiotherapy (SBRT) can effectively modulate the tumor-draining lymph node (TDLN) cytokine profile (by upregulating factors related to Th1 immune responses), thus enhancing the antitumor effect through immunotherapy.
3.1.3 Lung cancer.
Lung cancer is currently the most deadly cancer in the world, with about 18% cancer patients dying from it.78 The drugs used to treat lung cancer are mainly divided into targeted therapy, immunotherapy, and anti-angiogenic therapy, and microspheres are mainly used for targeted therapy. Several studies have shown that smaller particle size microspheres can achieve passive lung targeting by intravenous injection. For example, Monica et al.79 demonstrated that the intravenous injection of 12 μm PLGA microsphere is suitable for lung targeting in passive and pulmonary treatment. Another study80 also demonstrated the lung-targeting ability of lysozyme-loaded PLGA microspheres (LyIR@MS) in the methicillin-resistant Staphylococcus aureus (MRSA)-infected mice (Fig. 5). Besides, the PLGA microspheres DDS may potentially improve the efficacy of sophoradin to treat lung cancer.81 Sophoradin-loaded PLGA microspheres prepared by emulsification showed high drug levels (220.10 mg g−1) in the lung after 30 min, which was nearly 33 times higher than that in the solution group (6.77 μg g−1).
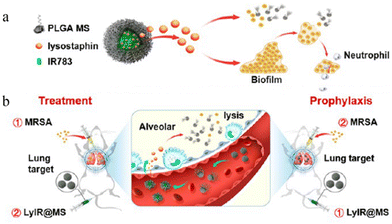 |
| Fig. 5 Lung-targeting studies of microspheres. (a) The structure of LyIR@MS. (b) The action of LyIR@MS. Reproduced from ref. 76 with permission from American Chemical Society, copyright 2021. | |
3.2 The application of commercially available microspheres in cancer
3.2.1 Prostate cancer.
Prostate cancer is a typical hormone-dependent tumor, and its occurrence and development is closely related to sex hormones.82 The main treatment methods for prostate cancer are radiotherapy, chemotherapy and androgen blocking therapy, and microspheres are mainly used for hormone blocking therapy. Leuprolide acetate for depot suspension (Lupron®Depot) was the first microsphere for prostate cancer. It is a gonadotrophin-releasing hormone analogue (GnRHa) (Fig. 6),83 which can control hormone levels through negative feedback regulatory mechanisms in vivo.84 It uses PLGA as the carrier material and adopts the traditional emulsion solvent evaporation method to prepare and realize the continuous release of leuprolide for a month, creating a precedent for such medicines. It has now been developed in 4 dosage forms with a single intramuscular injection every 1, 3, 4 and 6 months, respectively. All of them inevitably have an initial burst of API because the current 4 dosage forms are prepared by the conventional emulsification method, which will lead to patients having to take other drugs as adjuvant treatment and will increase side effects. Recently, investigators have reported a new preparation method for leuprolide microspheres based on micro-electromechanical systems and the IVL-DrugFluidic™ microsphere technology of the microfluidic system.85 PLGA-based 1 month formulation and PLA 3-based formulation prepared based on this technique was compared with commercially available microspheres. The results showed that the microspheres produced by the IVL-DrugFluidic™ system have the advantages of reducing the initial burst release, increasing the level of testosterone inhibition, and stabilizing the concentrations of API in the plasma.
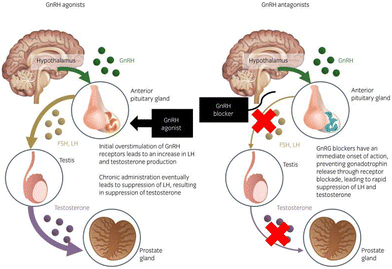 |
| Fig. 6 Mechanism of the action of GnRH agonists and antagonists in the hypothalamic–pituitary–gonadal axis of prostate cancer treatment. Reproduced from ref. 84 with permission from John Wiley and Sons, copyright 2020. | |
Triptorelin pamoate for injectable suspension (Trelstar®) is another microsphere formulation commonly used in the palliative treatment of advanced prostate cancer, and now 1, 3, and 6 month formulations have been developed for patients to choose instead of taking daily subcutaneous injections.86 Compared to Lupron® Depot, Trelstar® showed a stronger castration where triptorelin sustained-release microsphere kept the level of testosterone below 20 ng dL−1, whereas the level of testosterone less than 50 ng dL−1 (1.7 nmol L−1) has been identified as the standard of castration threshold.87
Recently, some researchers have combined microspheres with immunotherapy and have also achieved good results. Hydrogel microspheres covalently conjugated with IL-15 prepared by John A Hangasky et al.88 showed potent and prolonged antitumor activity when administered in combination with other immunotherapeutic agents in a CD8+ T cell-driven bilateral prostate cancer model.
3.2.2 Breast cancer.
Global cancer data posted by the International Agency for Research on Cancer (IARC) show that female breast cancer became the largest cancer in the world in 2020.78 The systemic therapy of breast cancer has now initially formed a mature system including chemotherapy, endocrine therapy, targeted therapy, and immunotherapy. The FDA has approved 24 drugs for the treatment of breast cancer between 1991 and 2021. Hormone-dependent breast cancer is common in women, and GnRHa (leuprolide acetate and triptorelin pamoate for injectable microsphere) have been approved for the treatment of premenopausal breast cancer. The results of several studies indicated the outstanding contribution of GnRHa to endocrine adjuvant therapy in breast cancer.89,90 They can effectively reduce the sex hormone levels in patients and promote the recovery of ovarian function.91
A phase II randomized clinical trial indicated that GnRHa worked best on premenopausal as well as postmenopausal women with hormone receptor-positive, HER2-negative metastatic breast cancer. Microspheres can also be used in combination therapy. Compared with capecitabine, the combination treatment group with palbociclib plus exemestane with GnRHa showed clinical benefits in the treatment of non-progressive survival in the treatment of patients.92 (Median progression-free survival: 14.2 months in the combination group and 1.17 months in the capecitabine group).
In addition, microspheres have been used in uterine fibroids. As early as 1999, leuprorelin acetate microspheres were approved by the FDA for the treatment of uterine fibroids as an important measure of preoperative tumor reduction. It acts primarily by hindering the extracellular matrix and TGF-β pathways.93 It reduces the volume of uterine fibroids by inhibiting the estradiol and progesterone signaling pathways, which is completely reversible after treatment termination;94 thus, it is safe. Leuprolide is considered the most effective drug in the treatment of uterine fibroids so far.95 Several studies have shown that leuprolide has good results in patients with uterine fibroids in anemia,96 reducing inflammatory response and angiogenic,97 and inhibiting tumor cell proliferation.98 But some researchers have pointed out that they could lead to some side effects, such as hot flashes.99
3.2.3 Neuroendocrine neoplasm.
Neuroendocrine neoplasms can be classified as functional and non-functional based on whether hormones can be secreted and produce hormone-related symptoms. Microsphere preparations currently available for functional neuroendocrine neoplasms-related therapy include octreotide, lanreotide, and pasireotide,100 all of which are somatostatin analogue (SSA) and encapsulated in the PLGA skeleton. They can reduce the levels of growth hormone in the body and play an antiproliferative effects.101–103 Octreotide microspheres and lanreotide microspheres belong to the first generation of first-line therapeutic drugs, which has similar therapeutic effects, but lanreotide has less side effects and is more convenient to use (low volume prefilled syringes) than octreotide.104 Pasireotide microspheres are the second generation of therapeutic agents and are used for hormone-related symptoms, such as Cushing's syndrome resulting from adreno corticotropic hormone tumors and pituitary neuroendocrine tumors. Studies showed that pasireotide is better than octreotide and lanreotide.105,106
3.2.4 Liver cancer.
Embolization therapy with microspheres have been successfully applied in the treatment of liver cancer.107,108 According to the different treatment methods, it is mainly divided into three types, namely, transarterial embolization (TAE), TACE, and transarterial radioembolization (TARE).109 The marketing of biodegradable embolic microspheres for treating HCC is shown in Table 4.110
Table 4 Biodegradable microsphere embolisms marketed for the treatment of HCC
Type |
Trade name |
Material |
Size (μm) |
Degradation time |
Firm |
Ref. |
The literature search was performed in PubMed, using as descriptors “microsphere” and “Biodegradable”. |
TAE |
Embocept® |
Starch |
50 |
35–40 min |
Pharmacept |
109
|
Spherex® |
Starch |
50 |
35–40 min |
Magle Life Sciences |
109
|
Gel-Bead® |
Gelatin |
100–300, 300–500, 500–700, 700–1000 |
4–12 weeks |
Teleflex |
109
|
Occlusin500® |
Collagen-coated PLGA |
150–210 |
12–24 weeks |
IMBiotechnologies |
109
|
TACE |
BioPearl® |
n.d. |
200 |
n.d. |
Terumo |
111
|
TARE |
QuiremSpheres® |
PLA |
15–60 |
>1 year |
Terumo |
112
|
TAE technology has been used in clinical practice since 1980s, which was initially mostly non-biodegradable microspheres. Although they can well block the nutrient supply of tumor cells, they are prepared from non-biodegradable materials, which will cause non-target vascular obstruction in the body for a long time. There are four biodegradable TAE microspheres (including Embocept®, Spherex®, Gel-Bead® and Occlusin500®) on the market, which can help to alleviate the side effects of permanent embolized microspheres.
TACE is a technique for injecting microsphere embolic agents loaded with compounds into an arterial catheter to block the blood supply of cancer cells in the liver. Biodegradable drug-eluting microspheres are used to temporarily occlude blood vessels to allow repeated manipulation or effort to limit the duration of tissue hypoxia.111 BioPearl® is the first biodegradable drug-eluting microsphere that was introduced to the European Union market in 2020. It can load the three different chemotherapy drugs, DOX, idarubicin, and epirubicin, according to the manufacturer's information.113 Due to the flexibility and versatility of TACE, many investigators have proposed therapeutic strategies for combining first-line anticancer drugs with TACE. For example, Song Yi Lee et al.114 loaded DOX encapsulated by hyaluronic acid-ceramide (HACE) into PLGA microspheres (DOX/HACE MS) as TACE for liver cancer treatment (Fig. 7). The antitumor efficacy assay in vivo showed that the tumor volume of the DOX/HACE MS treatment group was significantly lower than that of the control group at day 3 and 7, which was only 7% of that of the control group at day 24. The results of a phase IV trial involving 101 patients with advanced liver cancer showed that the camrelizumab plus TACE plan was effective and safe, indicating its potential as a promising treatment option for patients with advanced HCC.115 HCC patients with portal vein tumor thrombosis have a poor prognosis; thus, most drugs for them are incurable. A phase IV trial involving 32 patients with such disease suggested that the treatment strategy for TACE with lenvatinib is safe, effective and well tolerated.116
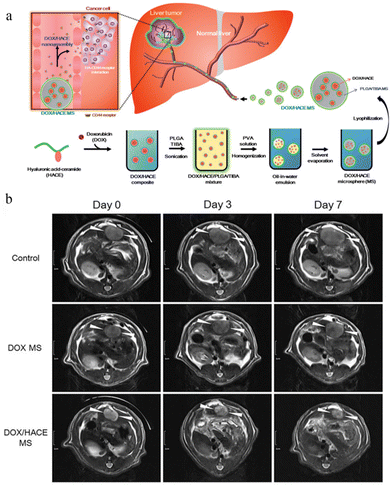 |
| Fig. 7 Microspheres as TACE for liver cancer treatment. (a) The preparation and effect of DOX/HACE MS. (b) In vivo anticancer activities in the McA-RH7777 tumor-implanted rat model. Serial MR images of liver tumors in the control (sham operation), DOX MS, and DOX/HACE MS groups on day 0 (pre-treatment status), 3, and 7 after IA administration. White arrowheads in the MR images indicate liver tumor. The length of the scale bar at the left side is 1 cm. Reproduced from ref. 110 with permission from Drug Delivery, copyright 2018. | |
Injectable degradable microspheres for TARE QuiremSpheres®, which is a PLA microsphere labeled by radioactive Holmium-166, was marketed in 2015.112 Unlike the previously marketed TheraSpheres® with a Y90 glass microsphere and SIR-Spheres® with a Y90 resin microsphere, Ho166 can emit γ radiation (81 keV (6.7%)) while emitting 1.77 MeV (48.7%), and 1.85 MeV (50.0%) β radiation. Moreover, it has paramagnetic properties, which allows it to image by both SPECT and MRI, thus having higher accuracy.117 The relatively short half-life of Ho166 in QuiremSpheres® enables the delivery of 90% of the radiation dose to the tumor site within 4 days, ensuring a high dose rate delivery118 (Ho166: 26.8 h vs. Y90: 64.1 h).
4 Difficulties and challenges in the development of microspheres
Microsphere injections can be widely used in the treatment of tumors and other diseases due to its strong syringeability (the degree of difficulties in transferring the medicated liquid into a syringe), injectability (the degree of difficulties in injecting the medicated liquid from a syringe to the injection site) and biodegradability. However, it is not difficult to see from the data of less than 20 microsphere injections on the market in the past 30 years that the development of this preparation still faces many difficulties and challenges, for example, the initial burst release of API, the stability of peptide and protein API when microsphere is degraded, and the expansion of production.
4.1 Initial burst release
The process of degradation and drug release in microspheres can be summarized into 3 categories, including initial burst, diffusion and erosion119 (some researchers have divided it into 4 categories into four phases: monophasic, burst biphasic, delayed biphasic, and triphasic). As shown in Fig. 8b, the ideal release curve of the API should be close to zero-order release in vitro. Unfortunately, most of the current formulations have the behavior of initial burst release. Particle size, particle porosity, polymer molecular weight, type of drug (hydrophobic and hydrophilic, or small molecular entities and biopharmaceuticals), aqueous boundary layer (dissolution and diffusion) may affect the release behavior of drug-loaded microspheres (Fig. 8a).120 Other reason for this may result from the adsorption of the API on the surface of the microspheres during the preparation, which may lead to a local drug concentration beyond the safety range.121 Some possible solutions are presented from 5 aspects, including drug−polymer interaction (such as linking the primary amino group of DOX to the terminal hydroxyl group of PLGA by a slowly hydrolyzable carbamate bond122), surface permeability (coating of the surface of microspheres with grafted polymers, cross-linked polymers or inorganic materials), monomer sequence distribution (adjusting the arrangement order of GA and LA), the adjustment of spatial distribution of encapsulated drugs and the manufacturing process (such as spray-drying, microfluidics, electrospray, phase separation)123 (Fig. 8c). For example, Richard Schutzman et al.124 showed that most of the microspheres prepared by the spray drying method displayed lower initial burst values than the commercially available Lupron Depot®.
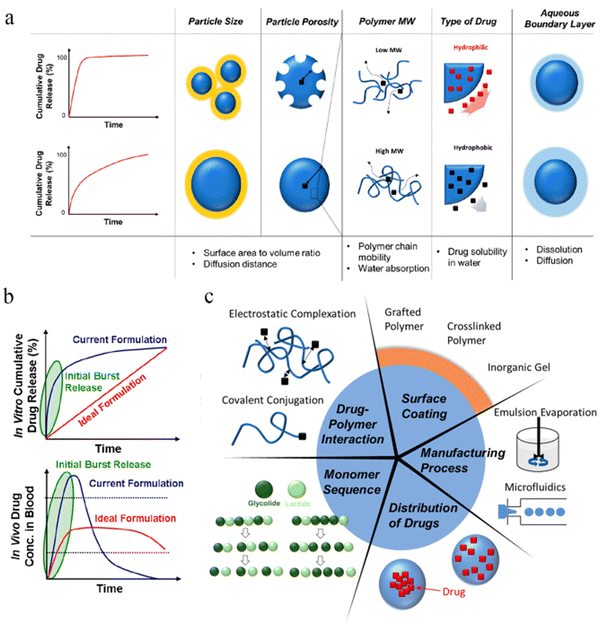 |
| Fig. 8 Burst release and resolutions of PLGA microspheres. (a) Factors affecting the initial burst release of the drug from PLGA microparticles. (b) Drug release curves in vivo and in vitro for current and ideal formulations. (c) Strategies for suppressing initial drug burst from PLGA microparticles. Reproduced from ref. 123 with permission from American Chemical Society, copyright 2020. | |
4.2 Stability
As shown in Table 1, the API of the microsphere injections used in the tumor therapy were all peptides. It is known that peptides and proteins are extremely unstable, and their activity is often influenced by factors such as preparation, storage, and environment in vivo.125 One of the most intractable questions is how to address the effects of the complex environment in vivo on peptides and proteins degradation. Although microspheres can well wrap the peptides to prevent them from decomposition and denaturation, the surrounding environment will change during the gradual degradation.126 The body temperature at a relative humidity 100% can degrade the exposed peptides and proteins. The subacidity environment generated by the degradation of microspheres can also pose a threat to the stability of peptides. One possible approach is to prepare mixed microspheres that neutralize the acid by interactions in the carrier materials. Researchers connected the poly(cyclohexane-1,4-diylacetonedimethylene ketal) (PCADK) to the rhGH-loaded PLGA microspheres and demonstrated its effectiveness.127 The results of confocal imaging of the microclimate pH inside microspheres showed that the internal environment of the control group with PLA microspheres became more acidic over time, while that of the experimental group with PCADK/PLGA microspheres was neutral. This provides a good idea to address the problem of in vivo stability of polypeptide and proteins. Another viable option is to add stabilizers. Heejun Park et al.128 investigated the effect of different additives (including carbohydrates: sucrose; amino acids: proline, lysine and phenylalanine; surfactant: poloxamer 188, phospholipids and DMPC, etc.) on the stability of polypeptide microspheres. The experimental results show that different stabilizers can adjust the stability of peptide microspheres from different aspects of aqueous solutions, at water/DCM interfaces, and during freeze-thawing and freeze-drying procedures.
4.3 Production
The production of microsphere injections is extremely complex, and there have been cases of their withdrawal from the market due to production problems. For example, Plenaxis® (a gonadotropin-releasing factor antagonist, which is an injectable microsphere with abarelix as the API and carboxymethyl cellulose (CMC) as the carrier material) is withdrawn from the market due to severe allergic reactions and production difficulties. A similar case is also the Nutropin®Depot, which is a long-acting human growth hormone. Nutropin®Depot was approved by the FDA in 1999 for the growth problems caused by the lack of growth hormone. Compared to the classic daily subcutaneous injection regimen, Nutropin®Depot only requires injections once a month and is loved by patients. However, the drug left the market in 2004 due to the difficulties of production and high production costs.129 Therefore, we should pay attention to the factors affecting the industrial production of pharmaceutical preparations in the research and development process. There is no universal PLGA microsphere formulation, which brings difficulties for qualitative and quantitative products. Small changes in manufacturing methods and raw materials can also lead to a great difference.130 In general, the development of the PLGA microsphere process usually takes 5–10 years from feasibility studies to the approval of new drug application. It can be said that the development of microsphere preparations has not only cost a lot of time but also money. But its high requirements of technology leading to non-replication and high market value characteristics still attract researchers and businessmen. A great challenge in the production of microspheres is sterility. Traditional terminal sterilization methods (such as autoclaving, filtration, gamma radiation and ionizing radiation) are not applicable because the microspheres contain biodegradable materials or proteins or peptides.131 Manufacturers can only set up many sterile clean rooms to ensure that the entire preparation process is free from contamination, which adds to the production burden.132 Morgan B. Giles et al.133 designed a simple, low-cost encapsulation process that does not require organic solvents and allows simple and cost-effective sterilization of blank microspheres prior to drug loading. It's an efficient organic-solvent-free remote encapsulation based on the capacity of common uncapped PLGA to bind and absorb into the polymer phase net positively charged peptides from aqueous solution after short exposure at modest temperature. According to the results of the animal experiments in Fig. 9c, the plasma testosterone levels of the animals with microspheres prepared by this method were not significantly different from those of the commercial 1 month Lupron® depot (p > 0.05). These results are helpful for the encapsulation and sterilization research in the production of microspheres.
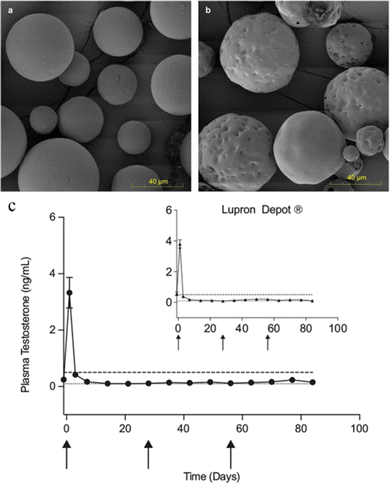 |
| Fig. 9 Scanning electron micrography images of PLGA microspheres and in vivo performance of remote-loaded microspheres compared to commercial gold standard. Representative micrography images of (a) blank PLGA microspheres and (b) the blank microspheres remote-loaded with octreotide (n = 2 independent experiments). (c) In vivo plasma testosterone levels of male Sprague-Dawley rats administered 3 monthly doses of remote-loaded leuprolide PLGA microspheres compared to equivalent 1 month Lupron® Depot administration (inset), NS. Dashed line indicates castration level (0.5 ng mL−1). Dotted line indicates analysis limit of quantitation (0.1 ng mL−1). Data are presented as mean values ± SEM (n = 6 rats). Arrow indicates days of dosing. Remote-loaded microspheres were from 240 mg mL−1 leuprolide in PLGA microspheres previously exposed to gamma irradiation. Reproduced from ref. 133 with permission from Nature Communications, copyright 2022. | |
To accelerate the development of microspheres, Jiayin Deng et al.134 built a prediction model through machine learning techniques. This model can help researchers to design and screen the formulation of microspheres, saving not only the cost but also time. Now, the manufacturing conditions of microspheres have also improved with the development of preparation technology, which has achieved in the manufacturing technology platforms of microspheres production and novel upstream and downstream facilities.135 Moreover, some researchers have proposed developing long-acting injectable PLGA/PLA-based microspheres through quality by design thinking and taking the approved Bydureon® as a typical example to prove its feasibility.125 It is believed that with the deepening of research and the improvement of production and preparation technology, more microsphere injections will enter the market and bring good news to patients suffering from pain.
5 Conclusions and discussion
From the listed data, clinical research and literature, the effect of microsphere injections is outstanding in anticancer drug delivery and has been clinically used in prostate cancer, breast cancer, uterine fibroids, neuroendocrine tumor, and liver cancer. Studies showed that the microspheres also have a good curative effect in other cancers, such as glioma, pancreatic cancer, and osteosarcoma.
As a long-acting DDS, microspheres have numerous advantages. In terms of drug encapsulation, microspheres can form a variety of core–shell structures or porous structures to meet different encapsulation requirements. In terms of controlled drug release, the drug can be slowly released with the degradation of carrier materials to prolong the drug action time. In terms of safety, biodegradable materials are highly biocompatible and harmless to humans. At present, there are a very large number of materials available for the preparation of microspheres, which can meet various therapeutic needs. Natural materials with certain adhesive properties such as starch, gelatin, chitosan, and alginate can be used to prepare hydrogel microspheres for injection into irregular structures. Alginate, chitosan, CaCO3 and calcium phosphate-based bio-materials are pH-responsive and can be used for targeted therapy. Magnetic microspheres can be combined with physical therapy to improve the treatment effect. PLGA and PLA are the most frequently used carrier materials because they are synthetic, controllable, and modifiable.
However, microspheres also have imitations, such as burst release, stability, and production problems. The burst release may lead to a surge of drug in the early stages of drug administration. In addition to the preparation of core–shell microspheres with different structures, the microsphere–hydrogel composite drug delivery system seems to be able to solve the problem. For the stability of drugs, in addition to adding a stabilizer, one can also modify the carrier materials and preparation method of improvement. No generic long-acting microsphere injections are currently available. Because of the high complexity of the manufacturing process and the lack of standards and guidelines, there are some difficulties in production. Scientists are solving the problem from different angles, including the improvement of the preparation process and the update of the equipment. In addition, to better facilitate microsphere development, scientists are also working to explore the in vitro–in vivo correlation (IVIVC) of biodegradable microsphere injections and develop modeling tools to facilitate the development of universal injectable microspheres bioequivalence guidelines. In my opinion, there are still many challenges for long-acting injectable microspheres from the laboratory to the production workshop. Relying on unilateral forces is far from enough, and close cooperation between researchers, the pharmaceutical industry and drug regulatory authorities is needed to overcome these problems. With the promotion of various aspects, it is believed that in the future, more long-acting injectable microspheres will be applied to the treatment of various cancers.
Author contributions
Huanhuan Cai: Conceptualization, methodology, data curation and writing – original draft preparation. Aixue Li and Fu Qi: visualization and investigation. Rongmei Liu, Xiaomeng Tang, Dan Li: validation and formal analysis. Yongwei Gu and Jiyong Liu: writing review & editing, methodology and supervision.
Conflicts of interest
The authors declare that they have no known competing financial interests or personal relationships that could have appeared to influence the work reported in this paper.
Acknowledgements
This work was supported by the projects of the National Natural Science Foundation of China (82074272, 82274103), the Science and Technology Commission of Shanghai Municipality (21XD1403400), and the Science and Technology Commission of Shanghai Municipality (20S21900300).
References
- K. Park, S. Skidmore, J. Hadar, J. Garner, H. Park and A. Otte,
et al., Injectable, long-acting PLGA formulations: analyzing PLGA and understanding microparticle formation, J. Controlled Release, 2019, 304, 125–134 CrossRef CAS PubMed.
- Y. Shi, A. Lu, X. Wang, Z. Belhadj, J. Wang and Q. Zhang, A review of existing strategies for designing long-acting parenteral formulations: focus on underlying mechanisms, and future perspectives, Acta Pharm. Sin. B, 2021, 11, 2396–2415 CrossRef CAS PubMed.
- C. I. Nkanga, A. Fisch, M. Rad-Malekshahi, M. D. Romic, B. Kittel and T. Ullrich,
et al., Clinically established biodegradable long acting injectables: an industry perspective, Adv. Drug Delivery Rev., 2020, 167, 19–46 CrossRef CAS PubMed.
- Q. Bao, X. Wang, Y. Zou, Y. Wang and D. J. Burgess, In vitro release testing method development for long-acting injectable suspensions, Int. J. Pharm., 2022, 622, 121840 CrossRef CAS PubMed.
- T. Li, A. Chandrashekar, A. Beig, J. Walker, J. K. Y. Hong and A. Benet,
et al., Characterization of attributes and in vitro performance of exenatide-loaded PLGA long-acting release microspheres, Eur. J. Pharm. Biopharm., 2021, 158, 401–409 CrossRef CAS PubMed.
- K. L. Subotnik, L. R. Casaus, J. Ventura, J. S. Luo, G. S. Hellemann and D. Gretchen-Doorly,
et al., Long-Acting Injectable Risperidone for Relapse Prevention and Control of Breakthrough Symptoms After a Recent First Episode of Schizophrenia. A Randomized Clinical Trial, JAMA Psychiatry, 2015, 72, 822–829 CrossRef PubMed.
- Y. Liu, W. Ma, P. Zhou, Q. Wen, Q. Wen and Y. Lu,
et al., In situ administration of temperature-sensitive hydrogel composite loading paclitaxel microspheres and cisplatin for the treatment of melanoma, Biomed. Pharmacother., 2023, 160, 114380 CrossRef CAS PubMed.
- H. Wu, J.-Q. Zhu, X.-F. Xu, H. Xing, M.-D. Wang and L. Liang,
et al., Biointerfacing Antagonizing T-Cell Inhibitory Nanoparticles Potentiate Hepatocellular Carcinoma Checkpoint Blockade Therapy, Small, 2021, 17, 2105237 CrossRef CAS PubMed.
- W. Xu, Y. Huang, H. Yuen, L. Shi, H. Qian and L. Cui,
et al., Living prosthetic breast for promoting tissue regeneration and inhibiting tumor recurrence, Bioeng. Transl. Med., 2022, 8, e10409 CrossRef PubMed.
- S. Yang, C. Mu, T. Liu, P. Pei, W. Shen and Y. Zhang,
et al., Radionuclide-Labeled Microspheres for Radio-Immunotherapy of Hepatocellular Carcinoma, Adv. Healthcare Mater., 2023, 12, 2300944 CrossRef CAS PubMed.
- N. A. Alhakamy, U. A. Fahmy, O. A. A. Ahmed, G. Caruso, F. Caraci and H. Z. Asfour,
et al., Chitosan Coated Microparticles Enhance Simvastatin Colon Targeting and Pro-Apoptotic Activity, Mar. Drugs, 2020, 18, 226 CrossRef CAS PubMed.
- J. Altomonte, R. Braren, S. Schulz, S. Marozin, E. J. Rummeny and R. M. Schmid,
et al., Synergistic antitumor effects of transarterial viroembolization for multifocal hepatocellular carcinoma in rats, Hepatology, 2008, 48, 1864–1873 CrossRef PubMed.
- K. Wu, A. Mohsin, W. Q. Zaman, Z. Zhang, W. Guan and M. Chu,
et al., Urchin-like magnetic microspheres for cancer therapy through synergistic effect of mechanical force, photothermal and photodynamic effects, J. Nanobiotechnol., 2022, 20, 224 CrossRef CAS PubMed.
- Y. Guo, H. Li, W. Shi, J. Zhang, J. Feng and X. Yang,
et al., Targeted delivery and pH-responsive release of doxorubicin to cancer cells using calcium carbonate/hyaluronate/glutamate mesoporous hollow spheres, J. Colloid Interface Sci., 2017, 502, 59–66 CrossRef CAS PubMed.
- Z.-F. Zhou, T.-W. Sun, F. Chen, D.-Q. Zuo, H.-S. Wang and Y.-Q. Hua,
et al., Calcium
phosphate-phosphorylated adenosine hybrid microspheres for anti-osteosarcoma drug delivery and osteogenic differentiation, Biomaterials, 2017, 121, 1–14 CrossRef CAS PubMed.
- K. Jelonek, A. Zajdel, A. Wilczok, B. Kaczmarczyk, M. Musiał-Kulik and A. Hercog,
et al., Comparison of PLA-Based Micelles and Microspheres as Carriers of Epothilone B and Rapamycin. The Effect of Delivery System and Polymer Composition on Drug Release and Cytotoxicity against MDA-MB-231, Breast Cancer Cells Pharm., 2021, 13, 1881 CAS.
-
Y. Wang, J. Yuan, L. Ma, X. Yin, Z. Zhu and P. Song, Fabrication of anti-dripping and flame-retardant polylactide modified with chitosan derivative/aluminum hypophosphite. Carbohydr Polym 10723Q1 [Internet]. 2022 [cited 2023 Feb 9];298. Available from: https://pubmed.ncbi.nlm.nih.gov/36241306/.
- K. Zhang, Z. Chen, M. Boukhir, W. Song and S. Zhang, Bioinspired polydopamine deposition and silane grafting modification of bamboo fiber for improved interface compatibility of poly(lactic acid) composites, Int. J. Biol. Macromol., 2022, 201, 121–132 CrossRef CAS PubMed.
- Z. Zhu, J. Hu and Z. Zhong, Preparation and characterization of long-term antibacterial and pH-responsive Polylactic acid/Octenyl succinic anhydride-chitosan @ tea tree oil microcapsules, Int. J. Biol. Macromol., 2022, 220, 1318–1328 CrossRef CAS PubMed.
- J. Li, X. Zhang, M. Zhao, L. Wu, K. Luo and Y. Pu,
et al., Tumor-pH-Sensitive PLLA-Based Microsphere with Acid Cleavable Acetal Bonds on the Backbone for Efficient Localized Chemotherapy, Biomacromolecules, 2018, 19, 3140–3148 CrossRef CAS PubMed.
- N. Rostami, F. Faridghiasi, A. Ghebleh, H. Noei, M. Samadzadeh and M. M. Gomari,
et al., Design, Synthesis, and Comparison of PLA–PEG–PLA and PEG–PLA–PEG Copolymers for Curcumin Delivery to Cancer Cells, Polymers, 2023, 15, 3133 CrossRef CAS PubMed.
- Y. Su, B. Zhang, R. Sun, W. Liu, Q. Zhu and X. Zhang,
et al., PLGA-based biodegradable microspheres in drug delivery: recent advances in research and application, Drug Delivery, 2021, 28, 1397–1418 CrossRef CAS PubMed.
- D. Essa, P. P. D. Kondiah, Y. E. Choonara and V. Pillay, The Design of Poly(lactide-co-glycolide) Nanocarriers for Medical Applications, Front. Bioeng. Biotechnol., 2020, 8, 48 CrossRef PubMed.
- X. Li, Y. Wei, K. Wen, Q. Han, K. Ogino and G. Ma, Novel insights on the encapsulation mechanism of PLGA terminal groups on ropivacaine, Eur. J. Pharm. Biopharm., 2021, 160, 143–151 CrossRef CAS PubMed.
- X. Zeng, W. Tao, L. Mei, L. Huang, C. Tan and S.-S. Feng, Cholic acid-functionalized nanoparticles of star-shaped PLGA-vitamin E TPGS copolymer for docetaxel delivery to cervical cancer, Biomaterials, 2013, 34, 6058–6067 CrossRef CAS PubMed.
- J. Wang, L. Helder, J. Shao, J. A. Jansen, M. Yang and F. Yang, Encapsulation and release of doxycycline from electrospray-generated PLGA microspheres: effect of polymer end groups, Int. J. Pharm., 2019, 564, 1–9 CrossRef CAS PubMed.
- N.-Q. Shi, J. Zhou, J. Walker, L. Li, J. K. Y. Hong and K. F. Olsen,
et al., Microencapsulation of luteinizing hormone-releasing hormone agonist in poly(lactic-co-glycolic acid) microspheres by spray-drying, J. Controlled Release, 2020, 321, 756–772 CrossRef CAS PubMed.
- D. Ding and Q. Zhu, Recent advances of PLGA micro/nanoparticles for the delivery of biomacromolecular therapeutics, Mater. Sci. Eng., C, 2018, 92, 1041–1060 CrossRef CAS PubMed.
- C. V. Rocha, V. Gonçalves, M. C. da Silva, M. Bañobre-López and J. Gallo, PLGA-Based Composites for Various Biomedical Applications, Int. J. Mol. Sci., 2022, 23, 2034 CrossRef CAS PubMed.
- S. Jilek, H. Zurkaulen, J. Pavlovic, H. P. Merkle and E. Walter., Transfection of a mouse dendritic cell line by plasmid DNA-loaded PLGA microparticles in vitro, Eur. J. Pharm. Biopharm., 2004, 58, 491–499 CrossRef CAS PubMed.
- H. Park, D.-H. Ha, E.-S. Ha, J.-S. Kim, M.-S. Kim and S.-J. Hwang, Effect of Stabilizers on Encapsulation Efficiency and Release Behavior of Exenatide-Loaded PLGA Microsphere Prepared by the W/O/W Solvent Evaporation Method, Pharmaceutics, 2019, 11, 627 CrossRef CAS PubMed.
- W. Zhou, Y. Zhang, S. Meng, C. Xing, M. Ma and Z. Liu,
et al., Micro-/Nano-Structures on Biodegradable Magnesium@PLGA and Their Cytotoxicity, Photothermal, and Anti-Tumor Effects. Small, Methods, 2021, 5, 2000920 CAS.
- C. He, W. Zeng, Y. Su, R. Sun, Y. Xiao and B. Zhang,
et al., Microfluidic-based fabrication and characterization of drug-loaded PLGA magnetic microspheres with tunable shell thickness, Drug Delivery, 2021, 28, 692–699 CrossRef CAS PubMed.
- B. Schmidt, R. Ohri, J. C.-F. Wang, P. Blaskovich, A. Kesselring and N. Scarborough,
et al., Local Pathology and Systemic Serum Bupivacaine After Subcutaneous Delivery of Slow-Releasing Bupivacaine Microspheres, Anesth. Analg., 2015, 120, 36 CrossRef CAS PubMed.
- Y. Wang, W. Huang, N. Wang, D. Ouyang, L. Xiao and S. Zhang,
et al., Development of Arteannuin B Sustained-Release Microspheres for Anti-Tumor Therapy by Integrated Experimental and Molecular Modeling Approaches, Pharm, 2021, 13, 1236 CAS.
- L. Li, Y. Du, Z. Yin, L. Li, H. Peng and H. Zheng,
et al., Preparation and the hemostatic property study of porous gelatin microspheres both in vitro and in vivo, Colloids Surf., B, 2020, 187, 110641 CrossRef CAS PubMed.
- S. Al-Nimry, A. A. Dayah, I. Hasan and R. Daghmash, Cosmetic, Biomedical and Pharmaceutical Applications of Fish Gelatin/Hydrolysates, Mar. Drugs, 2021, 19, 145 CrossRef CAS PubMed.
- D. M. Silva, H. K. N. Vyas, M. L. Sanderson-Smith and V. Sencadas, Development and optimization of ciprofloxacin-loaded gelatin microparticles by single-step spray-drying technique, Powder Technol., 2018, 330, 201–209 CrossRef CAS.
- D. J. Tischfield, A. Gurevich, O. Johnson, I. Gatmaytan, G. J. Nadolski and M. C. Soulen,
et al., Transarterial Embolization Modulates the Immune Response within Target and Nontarget Hepatocellular Carcinomas in a Rat Model, Radiology, 2022, 303, 215–225 CrossRef PubMed.
- K. Chen, F. Wang, R. Ding, Z. Cai, T. Zou and A. Zhang,
et al., Adhesive and Injectable Hydrogel Microspheres for Inner Ear Treatment, Small, 2022, 18, 2106591 CrossRef CAS PubMed.
- M. Yu, C. Zhang, Z. Tang, X. Tang and H. Xu, Intratumoral injection of gels containing losartan microspheres and (PLG-g-mPEG)-cisplatin nanoparticles improves drug penetration, retention and anti-tumor activity, Cancer Lett., 2019, 442, 396–408 CrossRef CAS PubMed.
- L. M. Caballero Aguilar, S. Duchi, C. Onofrillo, C. D. O’Connell, C. Di Bella and S. E. Moulton, Formation of alginate microspheres prepared by optimized microfluidics parameters for high encapsulation of bioactive molecules, J. Colloid Interface Sci., 2021, 587, 240–251 CrossRef CAS PubMed.
- D. Dhamecha, R. Movsas, U. Sano and J. U. Menon, Applications of alginate microspheres in therapeutics delivery and cell culture: past, present and future, Int. J. Pharm., 2019, 569, 118627 CrossRef CAS PubMed.
- D. Sadeghi, A. Solouk, A. Samadikuchaksaraei and A. M. Seifalian, Preparation of internally-crosslinked alginate microspheres: optimization of process parameters and study of pH-responsive behaviors, Carbohydr. Polym., 2021, 255, 117336 CrossRef CAS PubMed.
- J. Man, X. Wang, J. Li, X. Cui, Z. Hua and J. Li,
et al., Intravenous Calcium Alginate Microspheres as Drug Delivery Vehicles in Acute Kidney Injury Treatment, Micromachines, 2022, 13, 538 CrossRef PubMed.
- W. Wang, Q. Meng, Q. Li, J. Liu, M. Zhou and Z. Jin,
et al., Chitosan Derivatives and Their Application in Biomedicine, Int. J. Mol. Sci., 2020, 21, 487 CrossRef CAS PubMed.
- T. He, W. Wang, B. Chen, J. Wang, Q. Liang and B. Chen, 5-Fluorouracil monodispersed chitosan microspheres: microfluidic chip fabrication with crosslinking, characterization, drug release and anticancer activity, Carbohydr. Polym., 2020, 236, 116094 CrossRef CAS PubMed.
- L. Bugnicourt and C. Ladavière, Interests of chitosan nanoparticles ionically cross-linked with tripolyphosphate for biomedical applications, Prog. Polym. Sci., 2016, 60, 1–17 CrossRef CAS.
- P. Maleki Dana, J. Hallajzadeh, Z. Asemi, M. A. Mansournia and B. Yousefi, Chitosan applications in studying and managing osteosarcoma, Int. J. Biol. Macromol., 2021, 169, 321–329 CrossRef CAS PubMed.
- F. Pang, Y. Li, W. Zhang, C. Xia, Q. He and Z. Li,
et al., Biodegradable 131Iodine-Labeled Microspheres: Potential Transarterial Radioembolization Biomaterial for Primary Hepatocellular Carcinoma Treatment, Adv. Healthcare Mater., 2020, 9, 2000028 CrossRef CAS PubMed.
- Z.-L. Zhang, L.-J. Li, Dan Sun, Min Wang, J.-R. Shi and Di Yang,
et al., Preparation and properties of chitosan-based microspheres by spray drying, Food Sci. Nutr., 2020, 8, 1933–1941 CrossRef CAS PubMed.
- J. Chen, S. Chen, W. Cheng, J. Lin, S. Hou and H. Liu,
et al., Fabrication of porous starch microspheres by electrostatic spray and supercritical CO2 and its hemostatic performance, Int. J. Biol. Macromol., 2019, 123, 1–9 CrossRef CAS PubMed.
- Y. Hassanpouraghdam, M. Pooresmaeil and H. Namazi, In vitro evaluation of the 5-fluorouracil loaded GQDs@Bio-MOF capped with starch biopolymer for improved colon-specific delivery, Int. J. Biol. Macromol., 2022, 221, 256–267 CrossRef CAS PubMed.
- R. Minici, M. Ammendola, F. Manti, M. A. Siciliano, M. Minici and I. Komaei,
et al., Safety and Efficacy of Degradable Starch Microspheres Transcatheter Arterial Chemoembolization (DSM-TACE) in the Downstaging of Intermediate-Stage Hepatocellular Carcinoma (HCC) in Patients With a Child-Pugh Score of 8–9, Front. Pharmacol., 2021, 12, 634087 CrossRef CAS PubMed.
- J. M. Ludwig, R. Iezzi, J. M. Theysohn, T. Albrecht, A. Posa and A. Gross, European Multicenter Study on Degradable Starch Microsphere TACE: The Digestible Way to Conquer HCC in Patients with High Tumor Burden, Cancers, 2021, 13, 5122 CrossRef CAS PubMed.
- L. Lartigue, D. Alloyeau, J. Kolosnjaj-Tabi, Y. Javed, P. Guardia and A. Riedinger,
et al., Biodegradation of Iron Oxide Nanocubes: High-Resolution In Situ Monitoring, ACS Nano, 2013, 7, 3939–3952 CrossRef CAS PubMed.
- S. Ayyanaar, M. P. Kesavan, C. Balachandran, S. Rasala, P. Rameshkumar and S. Aoki,
et al., Iron oxide nanoparticle core-shell magnetic microspheres: applications toward targeted drug delivery, Nanomed. Nanotechnol. Biol. Med., 2020, 24, 102134 CrossRef CAS PubMed.
- X. Li, D. Zeng, P. Ke, G. Wang and D. Zhang, Synthesis and characterization of magnetic chitosan microspheres for drug delivery, RSC Adv., 2020, 10, 7163–7169 RSC.
- F. Mo, Q. Chen and X. Zhang, Synthesis of Hollow Calcium Carbonate Microspheres by a Template Method for DOX Loading and Release with Carbon Dots Photosensitivity, Mater. Basel Switz, 2022, 15, 8768 CAS.
- L. Li, Y. Yang, Y. Lv, P. Yin and T. Lei, Porous calcite CaCO3 microspheres: preparation, characterization and release behavior as doxorubicin carrier, Colloids Surf., B, 2020, 186, 110720 CrossRef CAS PubMed.
- Y. Wei, R. Sun, H. Su, H. Xu, L. Zhang and D. Huang,
et al., Synthesis and characterization of porous CaCO3 microspheres templated by yeast cells and the application as pH value-sensitive anticancer drug carrier, Colloids Surf., B, 2021, 199, 111545 CrossRef CAS PubMed.
- Y. Gong, Y. Zhang, Z. Cao, F. Ye, Z. Lin and Y. Li, Development of CaCO3 microsphere-based composite hydrogel for dual delivery of growth factor and Ca to enhance bone regeneration, Biomater. Sci., 2019, 7, 3614–3626 RSC.
- S. Mofakhami and E. Salahinejad, Biphasic calcium phosphate microspheres in biomedical applications, J. Controlled Release, 2021, 338, 527–536 CrossRef CAS PubMed.
- Y.-J. Seong, E.-H. Song, C. Park, H. Lee, I.-G. Kang and H.-E. Kim,
et al., Porous calcium phosphate–collagen composite microspheres for effective growth factor delivery and bone tissue regeneration, Mater. Sci. Eng., C, 2020, 109, 110480 CrossRef CAS PubMed.
- D. Kim, T. H. Han, S.-C. Hong, S. J. Park, Y. H. Lee and H. Kim,
et al., PLGA Microspheres with Alginate-Coated Large Pores for the Formulation of an Injectable Depot of Donepezil Hydrochloride, Pharmaceutics, 2020, 12, 311 CrossRef CAS PubMed.
- M. Chen, H. Tan, W. Xu, Z. Wang, J. Zhang and S. Li,
et al., A self-healing, magnetic and injectable biopolymer hydrogel generated by dual cross-linking for drug delivery and bone repair, Acta Biomater., 2022, 153, 159–177 CrossRef CAS PubMed.
- L. Rong, N. Li and Z. Zhang, Emerging therapies for glioblastoma: current state and future directions, J. Exp. Clin. Cancer Res., 2022, 41, 142 CrossRef CAS PubMed.
- X. Wang, P. Lu, L. Zhu, L. Qin, Y. Zhu and G. Yan,
et al., Anti-CD133 Antibody-Targeted Therapeutic Immunomagnetic Albumin Microbeads Loaded with Vincristine-Assisted to Enhance Anti-Glioblastoma Treatment, Mol. Pharm., 2019, 16, 4582–4593 CrossRef CAS PubMed.
- M. Yemisci, S. Bozdag, M. Çetin, F. Söylemezoglu, Y. Çapan and T. Dalkara,
et al., Treatment of malignant gliomas with mitoxantrone-loaded poly(lactide-co-glycolide) microspheres, Neurosurg, 2006, 59, 1296 CrossRef PubMed.
- O. Benny, L. G. Menon, G. Ariel, E. Goren, S.-K. Kim and C. Stewman,
et al., Local Delivery of Poly Lactic-co-glycolic Acid Microspheres Containing Imatinib Mesylate Inhibits Intracranial Xenograft Glioma Growth, Clin. Cancer Res., 2009, 15, 1222–1231 CrossRef CAS PubMed.
- N. C. Morsink, N. J. M. Klaassen, B. P. Meij, J. Kirpensteijn, G. C. M. Grinwis and I. A. Schaafsma,
et al., Case Report: Radioactive Holmium-166 Microspheres for the Intratumoral Treatment of a Canine Pituitary Tumor, Front. Vet. Sci., 2021, 8, 748247 CrossRef PubMed.
- T. Ma, X. Bai, Q. Wei, Y. Shui, M. Lao and W. Chen,
et al., Adjuvant therapy with gemcitabine and stereotactic body radiation therapy versus gemcitabine alone for resected stage II pancreatic cancer: a prospective, randomized, open-label, single center trial, BMC Cancer, 2022, 22, 865 CrossRef CAS PubMed.
- B. Pandit and M. Royzen, Recent Development of Prodrugs of Gemcitabine, Genes, 2022, 13, 466 CrossRef CAS PubMed.
- C. A. Ventura, C. Cannavà, R. Stancanelli, D. Paolino, D. Cosco and A. La Mantia,
et al., Gemcitabine-loaded chitosan microspheres. Characterization and biological in vitro evaluation, Biomed. Microdevices, 2011, 13, 799–807 CrossRef CAS PubMed.
-
A. Hawryłkiewicz and N. Ptaszyńska, Gemcitabine Peptide-Based Conjugates and Their Application in Targeted Tumor Therapy. Mol 4927Q2 [Internet]. 2021 [cited 2023 Feb 4];26. Available from: https://www.ncbi.nlm.nih.gov/pmc/articles/PMC7828243/.
- J. Xiao and H. Yu, Gemcitabine Conjugated Chitosan and Double Antibodies (Abc-GC-Gemcitabine Nanoparticles) Enhanced Cytoplasmic Uptake of Gemcitabine and Inhibit Proliferation and Metastasis In Human SW1990 Pancreatic Cancer Cells, Med. Sci. Monit., 2017, 23, 1613–1620 CrossRef CAS PubMed.
- B. J. Han, J. D. Murphy, S. Qin, J. Ye, T. P. Uccello and J. Garrett-Larsen,
et al., Microspheres Encapsulating Immunotherapy Agents Target the Tumor-Draining Lymph Node in Pancreatic Ductal Adenocarcinoma, Immunol. Investig., 2020, 49, 808–823 CrossRef CAS PubMed.
- H. Sung, J. Ferlay, R. L. Siegel, M. Laversanne, I. Soerjomataram and A. Jemal,
et al., Global Cancer Statistics 2020: GLOBOCAN Estimates of Incidence and Mortality Worldwide for 36 Cancers in 185 Countries, CA-Cancer J. Clin., 2021, 71, 209–249 CrossRef PubMed.
- M. Agnoletti, C. Rodríguez-Rodríguez, S. N. Kłodzińska, T. V. F. Esposito, K. Saatchi and H. Mørck Nielsen,
et al., Monosized Polymeric Microspheres Designed for Passive Lung Targeting: Biodistribution and Pharmacokinetics after Intravenous Administration, ACS Nano, 2020, 14, 6693–6706 CrossRef CAS PubMed.
- X. Lin, J. He, W. Li, Y. Qi, H. Hu and D. Zhang,
et al., Lung-Targeting Lysostaphin Microspheres for Methicillin-Resistant Staphylococcus aureus Pneumonia Treatment and Prevention, ACS Nano, 2021, 15, 16625–16641 CrossRef CAS PubMed.
- W. Wang, Y. Cai, G. Zhang, Y. Liu, H. Sui and K. Park,
et al., Sophoridine-loaded PLGA microspheres for lung targeting: preparation, in vitro, and in vivo evaluation, Drug Delivery, 2016, 23, 3674–3680 CrossRef CAS PubMed.
- V. N. Giri, T. M. Morgan, D. S. Morris, J. E. Berchuck, C. Hyatt and E. Taplin, Genetic testing in prostate cancer management: considerations informing primary care, CA-Cancer J. Clin., 2022, 2–12 Search PubMed.
- K. Desai, J. M. McManus and N. Sharifi, Hormonal Therapy for Prostate Cancer, Endocr. Rev., 2021, 42, 354–373 CrossRef PubMed.
- H. Van Poppel and P.-A. Abrahamsson, Considerations for the use of gonadotropin-releasing hormone agonists and antagonists in patients with prostate cancer, Int. J. Urol., 2020, 27, 830–837 CrossRef CAS PubMed.
- M. Kim, J. H. Kim, S. Kim, R. Maharjan, N. A. Kim and S. H. Jeong, New long-acting injectable microspheres prepared by IVL-DrugFluidicTM system: 1 month and 3 month in vivo drug delivery of leuprolide, Int. J. Pharm., 2022, 622, 121875 CrossRef CAS PubMed.
- G. Ploussard and P. Mongiat-Artus, Triptorelin in the management of prostate cancer, Future Oncol. Lond. Engl., 2013, 9, 93–102 CrossRef CAS PubMed.
- J. Breul, E. Lundström, D. Purcea, W. P. Venetz, P. Cabri and P. Dutailly,
et al., Efficacy of Testosterone Suppression with Sustained-Release Triptorelin in Advanced Prostate Cancer, Adv. Ther., 2017, 34, 513 CrossRef CAS PubMed.
- J. A. Hangasky, W. Chen, S. P. Dubois, A. Daenthanasanmak, J. R. Müller and R. Reid,
et al., A very long-acting IL-15: implications for the immunotherapy of cancer, J. Immunother Cancer, 2022, 10, e004104 CrossRef PubMed.
- M. Lambertini, L. Boni, A. Michelotti, E. Magnolfi, A. A. Cogoni and A. M. Mosconi,
et al., Long-Term Outcomes With Pharmacological Ovarian Suppression During Chemotherapy in Premenopausal Early Breast Cancer Patients, JNCI, J. Natl. Cancer Inst., 2021, 114, 400–408 CrossRef PubMed.
- M. Lambertini, H. C. F. Moore, R. C. F. Leonard, S. Loibl, P. Munster and M. Bruzzone,
et al., Gonadotropin-Releasing Hormone Agonists During Chemotherapy for Preservation of Ovarian Function and Fertility in Premenopausal Patients With Early Breast Cancer: A Systematic Review and Meta-Analysis of Individual Patient–Level Data, J. Clin. Oncol., 2018, 36, 1981–1990 CrossRef CAS PubMed.
- X. Zong, Y. Yu, H. Yang, W. Chen, X. Ding and S. Liu,
et al., Effects of Gonadotropin-Releasing Hormone Analogs on Ovarian Function Against Chemotherapy-Induced Gonadotoxic Effects in Premenopausal Women With Breast Cancer in China, JAMA Oncol., 2022, 8, 1–7 Search PubMed.
-
P. Yh, K. Ty, K. Gm, K. Sy, P. Ih and K. Jh, et al., Palbociclib plus exemestane with gonadotropin-releasing hormone agonist versus capecitabine in premenopausal women with hormone receptor-positive, HER2-negative metastatic breast cancer (KCSG-BR15-10): a multicentre, open-label, randomised, phase 2 trial. Lancet Oncol [Internet]. 2019 [cited 2023 Mar 2];20. Available from: https://pubmed.ncbi.nlm.nih.gov/31668850/.
- Q. Yang, M. Ciebiera, M. V. Bariani, M. Ali, H. Elkafas and T. G. Boyer,
et al., Comprehensive Review of Uterine Fibroids: Developmental Origin, Pathogenesis, and Treatment, Endocr. Rev., 2022, 43, 678 CrossRef PubMed.
- A. C. Wilson, S. V. Meethal, R. L. Bowen and C. S. Atwood, Leuprolide acetate: a drug of diverse clinical applications, Expert Opin. Investig Drugs, 2007, 16, 1851–1863 CrossRef CAS PubMed.
- M. Nisolle, S. Gillerot, F. Casanas-Roux, J. Squifflet, M. Berliere and J. Donnez, Immunohistochemical study of the proliferation index, oestrogen receptors and progesterone receptors A and B in leiomyomata and normal myometrium during the menstrual cycle and under gonadotrophin-releasing hormone agonist therapy, Hum Reprod Oxf Engl., 1999, 14, 2844–2850 CrossRef CAS PubMed.
- T. G. Stovall, O. Muneyyirci-Delale, R. L. Summitt and A. R. Scialli, GnRH agonist and iron versus placebo and iron in the anemic patient before surgery for leiomyomas: a randomized controlled trial. Leuprolide Acetate Study Group, Obstet Gynecol., 1995, 86, 65–71 CrossRef CAS PubMed.
- K. N. Khan, M. Kitajima, K. Hiraki, A. Fujishita, I. Sekine and T. Ishimaru,
et al., Changes in tissue inflammation, angiogenesis and apoptosis in endometriosis, adenomyosis and uterine myoma after GnRH agonist therapy, Hum Reprod Oxf Engl., 2010, 25, 642–653 CrossRef CAS PubMed.
- K. Vu, D. L. Greenspan, T.-C. Wu, H. A. Zacur and R. J. Kurman, Cellular proliferation, estrogen receptor, progesterone receptor, and bcl-2 expression in GnRH agonist-treated uterine leiomyomas, Hum. Pathol., 1998, 29, 359–363 CrossRef CAS PubMed.
- J. Donnez, J. Tomaszewski, F. Vázquez, P. Bouchard, B. Lemieszczuk and F. Baró,
et al., Ulipristal acetate versus leuprolide acetate for uterine fibroids, New Engl. J. Med., 2012, 366, 421–432 CrossRef CAS PubMed.
- A. K. Stueven, A. Kayser, C. Wetz, H. Amthauer, A. Wree and F. Tacke,
et al., Somatostatin Analogues in the Treatment of Neuroendocrine Tumors: Past, Present and Future, Int. J. Mol. Sci., 2019, 20, 3049 CrossRef CAS PubMed.
- M. Theodoropoulou and G. K. Stalla, Somatostatin receptors: from signaling to clinical practice, Front. Neuroendocrinol., 2013, 34, 228–252 CrossRef CAS PubMed.
- M. E. Caplin, M. Pavel, J. B. Ćwikła, A. T. Phan, M. Raderer and E. Sedláčková,
et al., Lanreotide in Metastatic Enteropancreatic Neuroendocrine Tumors, N. Engl. J. Med., 2014, 371, 224–233 CrossRef PubMed.
- A. Rinke, H.-H. Müller, C. Schade-Brittinger, K.-J. Klose, P. Barth and M. Wied,
et al., Placebo-controlled, double-blind, prospective, randomized study on the effect of octreotide LAR in the control of tumor growth in patients with metastatic neuroendocrine midgut tumors: a report from the PROMID Study Group, J. Clin. Oncol., 2009, 27, 4656–4663 CrossRef CAS PubMed.
- V. K. Pokuri, M. K. Fong and R. Iyer, Octreotide and Lanreotide in Gastroenteropancreatic Neuroendocrine Tumors, Curr. Oncol. Rep., 2016, 18, 7 CrossRef PubMed.
- M. R. Gadelha, M. D. Bronstein, T. Brue, M. Coculescu, M. Fleseriu and M. Guitelman,
et al., Pasireotide versus continued treatment with octreotide or lanreotide in patients with inadequately controlled acromegaly (PAOLA): a randomised, phase 3 trial, Lancet Diabetes Endocrinol., 2014, 2, 875–884 CrossRef CAS PubMed.
- A. Colao, M. D. Bronstein, P. Freda, F. Gu, C.-C. Shen and M. Gadelha,
et al., Pasireotide versus octreotide in acromegaly: a head-to-head superiority study, J. Clin. Endocrinol. Metab., 2014, 99, 791–799 CrossRef CAS PubMed.
- P. R. Galle, A. Forner, J. M. Llovet, V. Mazzaferro, F. Piscaglia and J.-L. Raoul,
et al., EASL Clinical Practice Guidelines: management of hepatocellular carcinoma, J. Hepatol., 2018, 69, 182–236 CrossRef PubMed.
- J. M. Llovet, R. K. Kelley, A. Villanueva, A. G. Singal, E. Pikarsky and S. Roayaie,
et al., Hepatocellular carcinoma, Nat. Rev. Dis. Primer, 2021, 7, 1–28 CrossRef PubMed.
- A. Pérez-López, C. Martín-Sabroso, L. Gómez-Lázaro, A. I. Torres-Suárez and J. Aparicio-Blanco, Embolization therapy with microspheres for the treatment of liver cancer: state-of-the-art of clinical translation, Acta Biomater, 2022, 149, 1–15 CrossRef PubMed.
- X. Li, P. Ramadori, D. Pfister, M. Seehawer, L. Zender and M. Heikenwalder, The immunological and metabolic landscape in primary and metastatic liver cancer, Nat. Rev. Cancer, 2021, 21, 541–557 CrossRef CAS PubMed.
- A. S. Mikhail, A. H. Negussie, M. Mauda-Havakuk, J. W. Owen, W. F. Pritchard and A. L. Lewis,
et al., Drug-eluting embolic microspheres: state-of-the-art and emerging clinical applications, Expert Opin Drug Delivery, 2021, 18, 383–398 CrossRef CAS PubMed.
- R. Bastiaannet, C. van Roekel, M. L. J. Smits, S. G. Elias, W. A. C. van Amsterdam and D. Doan,
et al., First Evidence for a Dose-Response Relationship in Patients Treated with 166Ho Radioembolization: A Prospective Study, J. Nucl. Med., 2020, 61, 608–612 CrossRef CAS PubMed.
- Terumo's BioPearl, the First Biodegradable Drug Eluting Microspheres Received CE mark | Terumo [Internet]. [cited 2023 Apr 6]. Available from: https://www.terumo.com/newsrelease/detail/20200428/530.
- S. Y. Lee, J. W. Choi, J.-Y. Lee, D.-D. Kim, H.-C. Kim and H.-J. Cho, Hyaluronic acid/doxorubicin nanoassembly-releasing microspheres for the transarterial chemoembolization of a liver tumor, Drug Delivery, 2018, 25, 1472–1483 CrossRef CAS PubMed.
-
R. You, Q. Xu, Q. Wang, Q. Zhang, W. Zhou, C. Cao, et al., Efficacy and safety of camrelizumab plus transarterial chemoembolization in intermediate to advanced hepatocellular carcinoma patients: a prospective, multi-center, real-world study. Front Oncol [Internet]. 2022 [cited 2023 Feb 3];12. Available from: https://pubmed.ncbi.nlm.nih.gov/35982962/.
- X. Ding, W. Sun, W. Li, Y. Shen, X. Guo and Y. Teng,
et al., Transarterial chemoembolization plus lenvatinib versus transarterial chemoembolization plus sorafenib as first-line treatment for hepatocellular carcinoma with portal vein tumor thrombus: a prospective randomized study, Cancer, 2021, 127, 3782–3793 CrossRef CAS PubMed.
- M. Weber, M. Lam, C. Chiesa, M. Konijnenberg, M. Cremonesi and P. Flamen,
et al., EANM procedure guideline for the treatment of liver cancer and liver metastases with intra-arterial radioactive compounds, Eur. J. Nucl. Med. Mol. Imaging, 2022, 49, 1682–1699 CrossRef CAS PubMed.
- M. A. D. Vente, J. F. W. Nijsen, T. C. de Wit, J. H. Seppenwoolde, G. C. Krijger and P. R. Seevinck,
et al., Clinical effects of transcatheter hepatic arterial embolization with holmium-166 poly(L-lactic acid) microspheres in healthy pigs, Eur. J. Nucl. Med. Mol. Imaging, 2008, 35, 1259–1271 CrossRef CAS PubMed.
- Y. Hua, Y. Su, H. Zhang, N. Liu, Z. Wang and X. Gao,
et al., Poly(lactic-co-glycolic acid) microsphere production based on quality by design: a review, Drug Delivery, 2021, 28, 1342–1355 CrossRef CAS PubMed.
- S. P. Schwendeman, R. B. Shah, B. A. Bailey and A. S. Schwendeman, Injectable controlled release depots for large molecules, J. Controlled Release, 2014, 190, 240–253 CrossRef CAS PubMed.
- O. S. Muddineti and A. Omri, Current trends in PLGA based long-acting injectable products: the industry perspective, Expert Opin. Drug Delivery, 2022, 19, 559–576 CrossRef CAS PubMed.
- H. S. Yoo and T. G. Park, Biodegradable polymeric micelles composed of doxorubicin conjugated PLGA–PEG block copolymer, J. Controlled Release, 2001, 70, 63–70 CrossRef CAS PubMed.
- J. Yoo and Y.-Y. Won, Phenomenology of the Initial Burst Release of Drugs from PLGA Microparticles, ACS Biomater. Sci. Eng., 2020, 6, 6053–6062 CrossRef CAS PubMed.
- R. Schutzman, N.-Q. Shi, K. F. Olsen, R. Ackermann, J. Tang and Y.-Y. Liu,
et al., Mechanistic evaluation of the initial burst release of leuprolide from spray-dried PLGA microspheres, J. Controlled Release, 2023, 361, 297–313 CrossRef CAS PubMed.
- C. Zhang, L. Yang, F. Wan, H. Bera, D. Cun and J. Rantanen,
et al., Quality by design thinking in the development of long-acting injectable PLGA/PLA-based microspheres for peptide and protein drug delivery, Int. J. Pharm., 2020, 585, 119441 CrossRef CAS PubMed.
- B. Yang, A. Gomes Dos Santos, S. Puri, A. Bak and L. Zhou, The industrial design, translation, and development strategies for long-acting peptide delivery, Expert Opin Drug Delivery, 2022, 19, 1233–1245 CrossRef CAS PubMed.
- C. Wang, Preparation and in vivo evaluation of PCADK/PLGA microspheres for improving stability and efficacy of rhGH, Int. J. Pharm., 2015, 495, 924–931 CrossRef CAS PubMed.
- H. Park, D.-H. Ha, E.-S. Ha, J.-S. Kim, M.-S. Kim and S.-J. Hwang, Effect of Stabilizers on Encapsulation Efficiency and Release Behavior of Exenatide-Loaded PLGA Microsphere Prepared by the W/O/W Solvent Evaporation Method, Pharmaceutics, 2019, 11, 627 CrossRef CAS PubMed.
- J. Hrkach and R. Langer, From micro to nano: evolution and impact of drug delivery in treating disease, Drug Delivery Transl. Res., 2020, 10, 567–570 CrossRef PubMed.
- Y. W. Lim, W. S. Tan, K. L. Ho, A. R. Mariatulqabtiah, N. H. Abu Kasim and N. Abd. Rahman,
et al., Challenges and Complications of Poly(lactic-co-glycolic acid)-Based Long-Acting Drug Product Development, Pharm., 2022, 14, 614 CAS.
- K. Panchal, S. Katke, S. K. Dash, A. Gaur, A. Shinde and N. Saha,
et al., An expanding horizon of complex injectable products: development and regulatory considerations, Drug Delivery Transl. Res., 2023, 13, 433 CrossRef PubMed.
- S. Mitragotri, P. A. Burke and R. Langer, Overcoming the challenges in administering biopharmaceuticals: formulation and delivery strategies, Nat. Rev. Drug Discovery, 2014, 13, 655–672 CrossRef CAS PubMed.
- M. B. Giles, J. K. Y. Hong, Y. Liu, J. Tang, T. Li and A. Beig,
et al., Efficient aqueous remote loading of peptides in poly(lactic-co-glycolic acid), Nat. Commun., 2022, 13, 3282 CrossRef CAS PubMed.
- J. Deng, Z. Ye, W. Zheng, J. Chen, H. Gao and Z. Wu,
et al., Machine learning in accelerating microsphere formulation development, Drug Delivery Transl. Res., 2023, 13, 966–982 CrossRef CAS PubMed.
- X. Li, Z. Zhang, A. Harris and L. Yang, Bridging the gap between fundamental research and product development of long acting injectable PLGA microspheres, Expert Opin. Drug Delivery, 2022, 19, 1247–1264 CrossRef CAS PubMed.
Footnote |
† These authors made equal contributions to this work. |
|
This journal is © The Royal Society of Chemistry 2024 |
Click here to see how this site uses Cookies. View our privacy policy here.