DOI:
10.1039/D2MA00642A
(Review Article)
Mater. Adv., 2022,
3, 7463-7483
Cellulose composites containing active constituents of coffee and tea: a prospective novel wound dressing
Received
5th June 2022
, Accepted 6th August 2022
First published on 14th September 2022
Abstract
Cellulose-based wound dressings are increasingly in demand due to their biocompatibility and extracellular matrix (ECM) mimicking properties. Although they contain no active constituents, pure cellulose-based scaffolds have promoted tissue regeneration. On the other hand, the absence of active ingredients opens the opportunity for the wound to become infected through microbial contamination and reactive oxygen, which will prolong the wound healing time. Microbial resistance to antibiotics has also become a problem that needs to be solved by replacing them using bioactive constituents of coffee and tea, which will be incorporated in a cellulose-based biopolymer matrix. Here, we discuss the potential development of a prospective wound dressing using cellulose composites with active ingredients found in coffee and tea. The mechanisms of coffee and tea wound healing properties are discussed, including their in vitro and in vivo characterization.
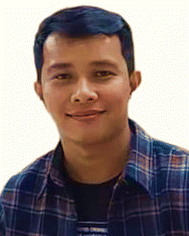
Afrinal Firmanda
| Afrinal Firmanda is a Master's student in Agroindustrial Engineering at IPB University, Indonesia. His research interest is the development of agricultural biomass-based nanomaterials under the supervision of Dr Farah Fahma and Prof. Khaswar Syamsu. Currently, he is focusing on studying the development of various nanocellulose-based products from microbes and plants. |
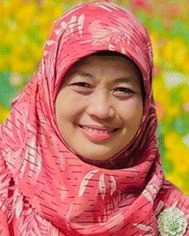
Farah Fahma
| Farah Fahma is an Associate Professor in the Department of Agroindustrial Technology at IPB University, Indonesia. She received her PhD degree from the Department of Biomaterial Sciences, The University of Tokyo, Japan. She did her post-Doctoral research at the Laboratory of Plant Material Science, Department of Global Agricultural Sciences, The University of Tokyo, Japan. Her research interests are cellulose and nanocellulose based products and value-added utilization of agricultural biomass. |
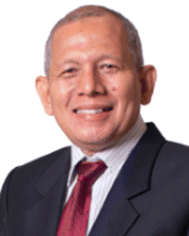
Khaswar Syamsu
| Khaswar Syamsu is a Professor in Bioprocess Engineering at the Department of Agroindustrial Technology, IPB University in which he is also the head of Bioindustry Lab and Division. He received his PhD in Chemical Engineering (Biochemical Engineering) from the University of Queensland. His research interest is bioproduct development. |
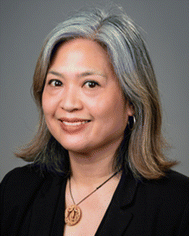
Jaydee Cabral
| Jaydee Cabral is a senior research fellow in the Department of Microbiology & Immunology at the University of Otago, Dunedin, New Zealand. She received her PhD in Biomedical Sciences from Eastern Virginia Medical School and Old Dominion University, USA. She is an expert in biomaterials, bioengineering, and chemical biology which combines 3D bioprinting and regenerative medicine to guide cells to make more complex living structures. She played a key role in the development of a commercialized, post-surgical, wound healing hydrogel, Chitogel®. Her research resides at the interface of chemistry, microbiology, and bioengineering with the goal of developing novel medical devices for various biomedical applications. |
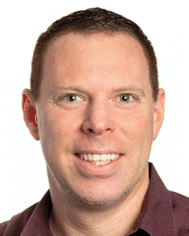
Daniel Pletzer
| Daniel Pletzer is a senior lecturer in the Department of Microbiology & Immunology at the University of Otago, Dunedin, New Zealand. He received his PhD from the Jacobs University Bremen, Germany. His research focuses on intractable high-density infections such as typified by skin abscesses. His research interests are centred around antimicrobial resistance, host-pathogen interaction, and nanomedicines. Currently, his team is working on novel approaches to combat hard-to-treat clinical bacterial isolates involved in multidrug resistance, biofilm infections, and persistence (specifically in skin infections). |
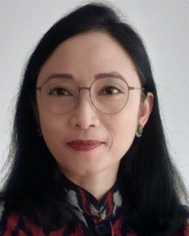
Marsia Gustiananda
| Marsia Gustiananda is an assistant professor in the Biomedicine Department at the Indonesia International Institute for Life Sciences, Indonesia. She received her PhD in Medical Sciences from John Curtin School of Medical Research (JCSMR)-Australian National University. Her research spans the field of virology, immunology, cancer immunology, and cell signalling with main interest in the identification of T-cell epitopes from pathogenic agents and cancer antigens using combine methods of immunoinformatics and immunology assay. |
1. Introduction
The wound healing mechanism is a complex series of events involving various cellular and biochemical processes to reconstruct and regenerate damaged tissue.1 The wound healing process involves three distinct phases: inflammation, proliferation, and maturation.2 Wound dressings are essential to cover and protect open wounds; an ideal wound dressing should be non-toxic, inexpensive and sterile, not release non-biodegradable particles, and be flexible and easy to use. It also maintains moisture in the wound, and is rarely changed, produces minimal pain during replacement, maintains wound at optimal temperature and pH, provides an antimicrobial barrier and activity, absorbs excess exudate, inactivates proteolytic enzymes in chronic wound fluid, provides hemostatic activity and effective wound debriding activity, and is permeable to oxygen, CO2, and water vapor.3,4 Wound dressings are generally widely available in the form of traditional cotton gauze. However, cotton gauze has no active healing ability.5 Synthetic polymers have been extensively modified as biomedical materials such as in drug delivery systems (DDSs), tissue engineering, wound dressings, and other medical applications. However, these polymers are controversial in their biocompatibility and bioaccumulation and are limited in their efficiency and versatility.6 Textile wound dressings also impact wound maceration and often cause severe pain upon removal. This limitation can be overcome by utilizing natural polymer-based wound dressings, such as cellulose hydrogel scaffolds to maintain environmental humidity and drug delivery.7
The availability of abundant natural biopolymers leads to the optimization of cellulose hydrogels' application in DDSs and tissue engineering.8 Several other natural polymers have also shown biocompatibility in tissue engineering and DDS applications, for example, chitosan,9 hyaluronic acid,10 collagen,11 alginates,12 and biopolymers. In modern wound care, wound dressings are expected to be able to maintain wound moisture while being able to absorb excess exudate. Even though they have shown their biocompatibility, these natural biopolymers have limitations in absorbing wound fluid (e.g., chitosan, alginates, and collagen) and are relatively expensive (collagen).13 Compared to these biopolymers, the use of cellulose in wound dressing applications is superior in terms of:
a. Low cost due to abundant availability which depends also on process technology and modifications
b. Having a nanostructure, especially bacterial cellulose (BC)
c. High hydrophilicity, especially after being modified, so it has a high exudate absorption capacity
d. Tending to be easy to modify
Of the many types of cellulose available, BC has been widely studied in medical biomaterial applications, is biocompatible with living tissue, and could support tissue growth due to its nanopore structure, good mechanical properties, and features that mimic the skin extracellular matrix (ECM). The 3D structural property of BC is supported by its superior physicochemistry, as its nano-size allows it to store the drug before it is released at the target site. In addition, the nanometer pore size and high hydrophilicity allow the added benefit of absorbing excess wound exudate.14
Biofilms and antibiotic resistance are often the main problems in choosing drugs to heal chronically infected wounds. In the long-term, topical antibiotics alter the microbiological environment attached to the skin and alter the bacteriology of the anterior nares and oropharynx, resulting in the emergence of resistant pathogens.15 Bacteria can wrap themselves in a matrix of hydrated polysaccharides and proteins and form a slimy layer called a biofilm.16 Biofilm formation increases bacterial tolerance to antibiotics and chemical disinfectants and protects bacteria against phagocytosis and other components of the body's defense system, resulting in chronic infection.17 Bacteria in chronic wounds that form biofilms will be protected from host defenses and develop resistance to antibiotic treatment.18 Of course, chronic infection by bacteria is very detrimental to wound healing. Therefore, the topical route offers several advantages in avoiding systemic toxicity and side effects, decreased induction of bacterial resistance, and high concentrations of antibacterial agents at the site of infection.15
Cellulose on its own does not contain active constituents that would act as antimicrobials or antioxidants. Antimicrobial activity, growth factor delivery, exogenous cell therapy, and biocompatible and biodegradable matrix construction play a role in designing high-tech dressings.19 Hence, adding active pharmaceutical ingredients (APIs) or drugs to the scaffold matrix based on cellulose is an attractive way to augment their activity. Some stimulant plants, such as coffee and tea, contain naturally active ingredients for wound healing and are available in abundance in natural ingredients or herbal products sold commercially. They provide a stimulant effect when consumed, but these plants can also be helpful as cheap topical drugs to accelerate wound healing.
Active constituents such as alkaloids, flavonoids, phenolics, essential oils, saponins, and other bioactive phytochemicals are the key to the potential of stimulant plants to repair damaged skin tissue. The active phytochemicals of plant stimulants are very complex, making it difficult to determine the specific constituents that contribute to wound healing. However, some specific constituents were found to contribute to the acceleration of wound healing activity, such as chlorogenic acid in coffee and green tea polyphenols. On the other hand, caffeine has shown contradictory characteristics, namely accelerating and inhibiting wound healing simultaneously.20 Furthermore, caffeine may contribute to antioxidant, anti-inflammatory, and antibacterial mechanisms, but caffeine can also inhibit cell proliferation.20–22 The natural active constituents of stimulant plant-based drugs are an attractive alternative to reduce the excessive use of antibiotics.
The combination of cellulose-based wound dressings as a natural, sustainable biopolymer and medicinal medium with natural active ingredients from stimulant plants as a source of antimicrobials and antioxidants could enhance wound healing. This article reviews the potential combination of cellulose biopolymers and plant stimulants as natural, bioactive wound dressings.
2. Cellulose wound dressing
The unique structural, hydrophilic, and physicochemical properties make cellulose an exciting option as a potential wound dressing. Cellulose can be found in the lignocellulosic biomass of plants and hemicellulose, lignin, and other components; and is also produced by bacteria, algae, and marine animals. Surface modification of nanocellulose can improve dispersion ability in solvents of different polarities, making it useful in DDS, tissue engineering, protein immobilization, and inorganic reaction templates.23 In addition, based on the fiber size, nanometer-structured biopolymers can significantly improve wound healing compared to conventional fibrous dressings.24
BC is superior to vegetable cellulose due to its high porosity, purity, permeability to gases and liquids, water absorption, mechanical resistance, and biocompatibility. It allows modification to obtain local drug delivery features and an antibacterial response.25–27 Furthermore, BC hydrogels have improved the nonhealing lower extremities, including diabetic ulcers, by accelerating epithelialization in human studies.28,29 In addition, chemically functionalized cellulose, like carboxymethyl cellulose (CMC)-based wound dressings, is also an attractive source for the development of wound dressings because of its biocompatibility, biodegradability, resemblance to extracellular tissue, low cost, and non-toxicity.30
Cellulose-based wound dressings have been widely developed by forming composite dressings with other polymers. For example, Harkins et al.31 developed a composite wound dressing of cellulose and chitosan that exhibited biocompatible, non-toxic, and biodegradable properties. It effectively inhibited the growth of vancomycin-resistant E. faecalis (ATCC 51299) and E. coli (ATCC 8739). BC soaked in chitosan, followed by a freeze-drying process, showed no cytotoxicity, inhibited S. aureus and E. coli, and accelerated epithelialization and regeneration of wound tissue in a rat model.32 The hydrogel composite of carboxymethyl chitosan and dialdehyde-modified nanocellulose crystals is also biocompatible and supports the growth of partial-thickness burn cells.33
BC and montmorillonite nanocomposites, which have antimicrobial activity against S. aureus and E. coli, have been developed and applied as a dressing to accelerate tissue regeneration for wound healing.34 Adding zein, a maize protein, to the nanocellulose acetate fiber membrane increased the hydrophilicity and bioactivity and created a moist wound environment.35 Cellulose/gelatin composite sponges were influential in wound healing and exhibited micro-and macro-porous architectures.5 Cellulose–collagen composites were found to support the healing of moderate depth donor site wounds with little or no postoperative pain.36 Nanoelectrospores of poly(ε-caprolactone), cellulose acetate, dextran, and tetracycline hydrochloride were developed for wound dressing applications.37 The incorporation of dextran and the antibiotic enhanced the adhesion and cell proliferation of the nanocomposite fibers and antimicrobial activity. Using crosslinking and freeze-drying methods, Yin et al.38 combined gelatin, hyaluronic acid, and cellulose nanocrystals to produce a hydrogel wound dressing. Nanocellulose significantly improved the hydrogel properties and played a vital role in displaying the appropriate rheological and swelling responses. NIH-3T3 cells were able to adhere, proliferate, and grow in these hydrogel composites. These studies show that cellulose composites increase the multifunctionality of the developed biomaterial and increase their potential use as bioactive wound dressings.
Commercialized, modern wound dressings are made of biopolymers containing active compounds.24 Several methods can incorporate drug models (such as natural active constituents, antibiotics, and metal dioxides) into cellulose polymers, such as crosslinking, suspension and solution mixing, synthesis, impregnation, immersion, UV irradiation, and immobilization (Table 1). Nanocellulose fibers containing chemicals, biochemically APIs, or even stem cells can be used as a delivery vehicle for wound dressings.39 For example, silver nanoparticles added in the adsorbed cellulose and chitosan composites were biocompatible with NIH3T3 fibroblastic cells and displayed antibacterial efficacy against Gram-positive and Gram-negative bacteria.40Table 1 summarizes some biocompatibility studies incorporating cellulose and its derivatives with active ingredients (model drugs) for wound dressing applications.
Table 1 Study of cellulose as a wound dressing
Type of cellulose |
Active compound |
Cell culture model |
Microbial target |
Methods |
Results |
Ref. |
Hydroxyethylcellulose |
Tungsten oxide |
Human dermal fibroblast cell |
Salmonella sp., P. aeruginosa |
Crosslink with citric acid |
The hydrogel membrane was anti-inflammatory and antibacterial, and tungsten was safe against normal human cells (white blood cells and dermal fibroblasts). |
41
|
|
Cellulose |
Diclofenac |
— |
— |
Mixing trimethylsilyl cellulose (DSSi:2.5) solutions with diclofenac dissolved in tetrahydrofuran |
The kinetics of drug release from cellulose films had potential as a model platform for viscose-based wound dressings. |
42
|
|
BC |
Nanosilver |
Rat fibroblasts |
S. aureus
|
Self-assembly of silver nanoparticles on the BC surface |
BC-containing silver nanoparticles reduce inflammation, inhibit bacterial growth and low cytotoxicity, and accelerate the healing of blisters. |
43
|
|
BC |
Nanosilver |
Epidermal cells |
E. coli, S. aureus, P. aeruginosa |
Synthesize and impregnate silver nanoparticles onto the BC |
The composite had significant antibacterial properties and allowed epidermal cell attachment and growth without cytotoxicity, reduced inflammation, and accelerated wound healing. |
44
|
|
BC |
Nanosilver |
— |
E. coli, S. aureus |
Immersion of BC in silver nitrate solution and reducing it using sodium borohydride |
The membrane had a potent effect against Gram-negative (E. coli) and Gram-positive (S. aureus) bacteria |
45
|
|
Cellulose |
Lysostaphin |
Keratinocytes |
S. aureus
|
Immobilization of lysostaphin enzymes and cellulose-chitosan and polymethylmethacrylate cellulose |
Bandage preparations showed activity against S. aureus based on in vitro skin models, low keratinocyte toxicity, and good biocompatibility in wound healing applications. |
46
|
|
BC |
Vaccarin |
Testing in a rat model |
— |
Immersion of BC in vaccarin solution |
BC and bacterial-vaccarin cellulose membranes were non-cytotoxic and suitable for cell growth. Wounds treated with BC and vaccarin resulted in faster epithelialization and regeneration than those treated with BC. |
47
|
|
BC |
Silver nanoparticles |
— |
E. coli
|
Silver nanoparticle synthesis in BC by UV irradiation. |
Composites killed bacteria and supported wound healing. |
48
|
|
BC |
Chitosan, copper sulfate |
Living cell L929 mouse fibroblasts, human dermal fibroblasts |
E. coli, MRSA |
— |
After undergoing antimicrobial and live-cell tests, BC/sodium alginate/chitosan/copper sulfa composites were promised to be applied as wound dressings. |
49
|
|
BC |
Silver sulfadiazine |
Epidermal cell |
P. aeruginosa, E. coli, S. aureus |
Immersing BC into silver sulfadiazine suspension using ultrasonication |
The BC and silver sulfadiazine membrane composites showed potential as antimicrobial and biocompatible wound dressings. |
50
|
|
BC |
Amoxicillin |
Red blood cells |
Fungus, Gram-positive and -negative bacteria |
Amoxicillin and BC grafting |
Sponges were non-toxic in vitro and in vivo, showed antimicrobial activity, and accelerated wound healing in vivo. |
51
|
|
BC |
Ampicillin |
— |
E. coli, C. albicans, S. aureus |
Synthesized using glutaraldehyde |
The BC and gelatin composite sponge showed excellent antibacterial activity and potential as a wound dressing. |
52
|
3. Cellulose as a medium for drug delivery systems (DDSs))
In general, wound dressings consist of a matrix/medium and a drug model, in which the delivery efficiency affecting the drug efficacy is discussed in DDS. The DDS must be considered in designing and developing cellulose-based wound dressings to prevent infection or increase the drug efficacy. A DDS determines the active compound's concentration in the blood with minimum fluctuations and predictability. The DDS is also helpful in protecting bioactive compounds with very short half-lives, eliminating their side effects due to drug wastage and the need for frequent dosing, thereby optimizing their therapeutic effect by solving drug stability problems.53 Hydrogels, as a DDS, have been shown to demonstrate good biocompatibility, a flexible network structure to control drug diffusion, an adaptable affinity for drugs, an ability to encapsulate water-soluble compounds, and sustained local bioactive release.53,54 The hydrogel formation mechanism as a drug delivery medium can be carried out through:55 (1) physical crosslinking, achieved by using various environmental triggers (ionic strength, temperature, and pH) and various physicochemical interactions (charge condensation, hydrophobic interactions, stereocomplexation, hydrogen bonding, or supramolecular chemistry), and (2) covalent crosslinking via small-molecule or polymer–polymer crosslinking initiated via redox polymerization, esterification with an anhydride, and imine linkage formation.
Cellulose might be an excellent DDS due to its biodegradability, biocompatibility, abundance, and unique encapsulation properties. Hydrogels, based on cellulose, can be easily prepared through physical interactions (van der Waals, hydrogen bonds, mechanical chain entanglement, electronic associations, or hydrophobic) or chemical crosslinking (using cross-linker agents).56 Cellulose and its derivatives can change the solubility or gelling of drugs to control the drug release profile sustainably.57 Because of its high surface area and polymerization, nanocellulose provides high loading and binding capacities for APIs, allowing drug-release control.58 Cellulose nanofiber-based media enable controlled drug release by dispersing the drug in the fiber, changing processing conditions, and exploiting the unique chemical properties of cellulose nanofibers.59 As a medium for controlling drug release, nanocellulose forms a dense fiber network around the incorporated drug entity.60 Electrospun cellulose nanofibers have been studied as a DDS due to their antioxidant, anti-inflammatory, antimicrobial, anti-cancer, vitamin, and amino acid characteristics. However, these fibers are not fully biodegradable in the human body because of the absence of cellulase enzymes.61 The routes that could be used to control the rate of drug release include:55
(1) Drug–hydrogel interactions (Fig. 1)
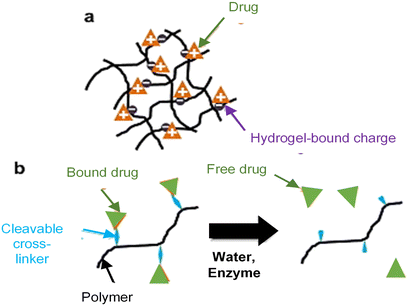 |
| Fig. 1 Drug–hydrogel interaction strategy, (a) physical, for example, the opposite charge interaction between cellulose and drugs, and (b) chemistry, for example, crosslinking agents facilitate drug interactions with cellulose.55 Adopted with permission from ref. 55. | |
a. Physical interactions: through charge interactions between ionic polymers and charged drugs.
b. Covalent bonding: drugs are covalently conjugated to the hydrogel matrix, the release of which is controlled by the chemical or enzymatic cleavage rate of the polymer–drug bond.
(2) Gel Tissue Engineering (Fig. 2)
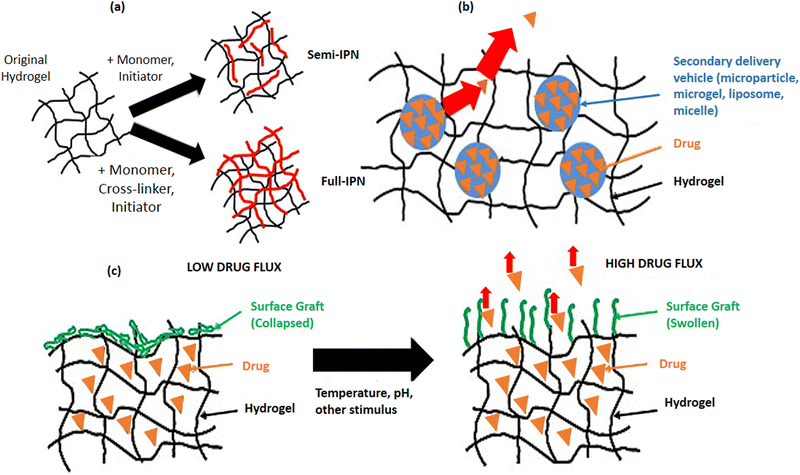 |
| Fig. 2 Engineering gel network via (a) semi and full interpenetration (IPN), (b) composite hydrogels containing a drug, and (c) surface diffusion control.55 Adopted with permission from ref. 55. | |
a. Interpenetrating Polymer Networks (IPNs): the second hydrogel network is polymerized in the pre-polymer hydrogel by immersing the pre-polymerized hydrogel into the monomer solution and polymerization initiator.
b. Surface Diffusion Control: this reduces the permeability “film” layer on the hydrogel surface, often with a thermosensitive switch for on–off drug release.
c. Composite Hydrogels: drug encapsulated microparticles are added to the hydrogel network.
The difference in the drug solubility impacts the ease of dispersion in the cellulose matrix. BC is one type of cellulose utilized for topical drug delivery.62 The surface of the BC matrix can be modified before drug loading to inhibit drug release. Modification before and after the drug loading changes the physicochemical properties of the BC matrix. It is influenced by the chemical structure, loaded drug concentration, and surface modifier concentration. This surface modification has been studied in drug models in the form of famotidine which is poorly soluble in water, and tizanidine which is very soluble in water.63 The studies showed that most of the drug was released in 0.5–3 h from famotidine loaded matrices and 0.25–0.5 h from tizanidine loaded matrices, depending on the chemical structure, loaded concentration drug, the surface modifier concentration, and pre- and post-drug loading modifications. Glutaraldehyde as a binding agent for the BC hydrogel formation and gelatin are also considered good candidates for DDSs.64
Cellulose esters also play an essential role in modern drug delivery technology due to their very low toxicity, having endogenous dietary components as a byproduct of their decomposition process, stability, film strength, high water permeability, compatibility with various active ingredients, high Tg, and the ability to form micro-and nanoparticles.65 For example, ultrasound-assisted preparation of hydroxypropylmethylcellulose-based nanocomposites through the blending of cellulose nanofibrils has been explored to generate biopolymers for a transdermal DDS.66 Meanwhile, sodium CMC wafers containing neomycin trisulfate showed advantageous wound dressing characteristics (flexibility, sponge-like properties, uniform wafer texture, and high drug content uniformity) and Gram-positive and Gram-negative bacterial inhibition.67 Other studies on cellulose as a drug delivery vehicle are summarized in Table 2.
Table 2 Cellulose application as a medium of DDSs
Type of cellulose |
Drug model |
Characteristics of drug delivery vehicles |
Ref. |
Cellulose nanofiber |
Bendamustine hydrochloride |
The porous and woven matrix had good mechanical properties with the release of approximately 69.205% ± 2.5% drug (for 24 hours, pH = 1.2) and ∼78% ± 2.28% drug (pH = 7.4) with floating behavior for ∼7.5 hours. Cellulose nanofiber gels are helpful for a gastroretentive DDS. |
68
|
|
Hydroxyethyl cellulose |
Isoliquiritigenin |
The hydrogel synthesized by crosslinking hyaluronic acid and hydroxyethylcellulose can be a transdermal delivery system whose properties are influenced by the swelling medium and crosslink density. |
69
|
|
Cellulose |
Galangin |
The crosslinked cyclosophorose/cellulose hydrogel inhibited the S. aureus growth, which was maintained for up to 72 hours, making it potentially efficient antibacterial dressing material for a long time without frequent replacement. |
70
|
|
Ethylcellulose microspheres |
Ceftazidime |
The hydroxyapatite/polyurethane composite containing microspheres (ceftazidime encapsulated in ethylcellulose) reduced initial blast release with the sustained release for up to 60 days. This composite proved to be an effective DDS with good cytocompatibility and antibacterial properties. |
71
|
|
Cellulose acetate nanofiber |
Tetracycline hydrochloride |
Tetracycline hydrochloride bonded in spun cellulose acetate nanofiber composites showed effectiveness in reducing E. coli up to 77–88% (after 10 minutes to 1 hour) and S. aureus around 83–85% (after 10 minutes to 1 hour). |
72
|
|
CMC |
Doxorubicin |
The combination of graphene quantum dots in the CMC hydrogel and doxorubicin increased in vitro swelling, degradation, pH-sensitive drug delivery properties, and water vapor permeability, although with little toxicity to blood cancer cells (K562). |
73
|
|
Mucoadhesive hydrophobic cationic amino cellulose |
Camptothecin |
Cellulose derivatives can reduce the rate of release of camptothecin, making it promising for DDS applications that are difficult to dissolve in water. |
74
|
|
Ethylcellulose hydroxypropyl methylcellulose |
Hydrochlorothiazide |
Hydrochlorothiazide release from patches formulated with ethylcellulose and hydroxypropyl methylcellulose (1 : 1 and 2 : 1 in ratio) occurs via diffusion. Therefore, the process can be an effective alternative delivery approach for hydrochlorothiazide. |
75
|
|
Cellulose nanocrystals |
Curcumin |
Incorporating curcumin into nanocellulose in surfactant media provides a promising approach for delivering to the stomach and upper intestinal tract. |
76
|
Based on studies in Tables 1 and 2, cellulose can be inserted or bound with various drug models such as active ingredients in oils and extracts, metal oxides, and commercially available antibiotics. When using metal oxides as model drugs, paying attention to their effects they accumulate in tissues is essential. Future studies expect to focus on dispersing and binding the drug in the cellulose matrix so that the release time is slow and follows the rate of tissue regeneration needed. The future challenge is to produce drug release kinetics using compatible raw materials that offer cheap and reliable process technology.
4. Wound healing activity of coffee and tea
Bioresource-based wound healing is a growing field in modern biomedical science.77 Natural active ingredients derived from plants can modulate wound healing by promoting angiogenesis, stimulating collagen production, antimicrobial and antioxidant activity, and other mechanisms.78 Plant-based medicine for wound healing is cheap, affordable, and safe because hypersensitivity reactions are rare.79 Efficient downstream processing of APIs is highly dependent on their particulate properties, such as size and shape distribution.80 In promoting wound healing mechanisms, the potency of natural active ingredients is assessed through microbial, animal, and human studies.
4.1 Coffee
Coffee has long been known as a traditional wound healing treatment modality. Coffee is the most efficient and inexpensive medicine for treating acutely infected wounds.81 Winata et al.82 showed that wound treatment with coffee grounds and hydrocolloid wound dressings was better for lowering MMP-1 levels and accelerating epithelialization than saline gauze dressings. Saline-gauze could not reduce MMP-1 levels, which resulted in delayed epithelialization.
Yuwono et al.81 studied the effect of coffee on healing acute and chronic wounds in 130 patients. All wounds were treated using topical coffee grounds as wound dressings which were changed every four weeks until they healed. In this study, coffee grounds were used as a new paradigm in wound management due to their antimicrobial and antioxidant activity, pleasing odor, increased wound dressing longevity, absorption capacity to maintain a moist wound environment, autolytic debridement capacity, cost-effectiveness, and minimal adverse reactions.
The coffee's pharmacological properties are due to its high polyphenol content, such as chlorogenic and caffeic acid, diterpenes (kahweol and cafestol), melanoidin, and trigonelline. The constituents contribute to anti-inflammatory, antioxidant, anti-angiogenic, anti-cancer, chemoprotective, and hepatoprotective effects.83 In addition, the chlorogenic acid hydrogel significantly reduced the wound area in the inflammatory phase, related to its anti-inflammatory and antioxidant action.84 The content of coffee oil has been studied to provide skin wound healing effects. Lania et al.85 showed that coffee oil from green coffee and roasted coffee produced systemic effects observed in serum cytokine levels and second harmonics generation (SHG) analysis through rat tests. However, more work is still needed to identify the most active molecule and the mechanism of action of coffee oil.
On the other hand, coffee has an anti-angiogenesis effect due to cafestol and kahweol. Therefore, anti-angiogenic compounds in coffee are promising for treating cancer.86 Cafestol and kahweol are diterpenes commonly found in unfiltered coffee. Cafestol provides benefits through various biological activities, including antitumorigenic, antioxidant, and anti-inflammatory effects. Cafestol inhibits human umbilical vascular endothelial cell angiogenesis by influencing proliferation, migration, and tube formation.86 Kahweol is an anti-angiogenic and anti-inflammatory compound tested in angiogenesis in vivo and ex vivo, and has potential for use in antitumor therapy.87
Caffeine is an alkaloid found in coffee that serves as an antioxidant and anti-inflammatory agent in wound healing mechanisms.88 Ojeh et al.20 showed that coffee can also act as an antagonist and negates the adenosine receptors' effect that promotes wound healing. Furthermore, the study confirmed that caffeine could limit keratinocyte cell proliferation depending on the dose. Moreover, differentiation and cell adhesion remained unaffected in single layer cultures treated with different caffeine doses. Caffeine has also been shown to inhibit epithelialization in human ex vivo studies.20
Several studies in animal models showed the biocompatibility of the active ingredients of coffee in providing wound-healing effects. Humaryanto and Ave89 studied green coffee bean extracts extracted with 70% ethanol for wound healing in rats. The percentage of total wound closure on day 16 in the rat group smeared with the coffee extract was 83% higher than that in the control group (only 66%). Setyawan et al.90 assessed the healing of S. aureus infected wounds using macroscopic indicators (dry wounds, non-hyperemic wound edges, and average leukocyte count) in male Wistar rats. There was a significant difference in healing infected wounds using coffee grounds compared to honey. The healing time of infected wounds using coffee grounds is faster than using honey (3.4 weeks). Coffee plus honey was the most effective treatment modality for persistent post-infectious cough (PPC).91,92 Another study showed that wound healing using coffee grounds or honey was not significantly different.93 Although it shows a positive performance, future research is vital to determine the effective coffee powder dose and honey in providing a wound-healing effect.
Histopathological studies on thirty-six New Zealand white rabbits supported the wound healing activity of green coffee bean extracts. Shahriari et al.94 studied the green coffee bean extract in a full-thickness wound model. Coffee (10%) increased the wound closure rate, exhibited a shorter epithelialization period, increased hydroxyproline content, and suppressed lipid peroxidation compared with eucerin treatment. The therapeutic effect of 10% green coffee beans was significantly superior to phenytoin in increasing the speed of wound closure, decreasing the epithelialization period, increasing the hydroxyproline content, and suppressing lipid peroxidation.
The effectiveness of coffee in healing acute and chronic wounds has been proven by Yuwono et al.81 (Fig. 3). They investigated wound healing properties using topical coffee grounds (n = 82) and compared this to those obtained using saline (n = 78 with gauze dipped in 0.9% NaCl) in diabetic patients. A total of 130 wound patients suffering from type-2 diabetes mellitus (90 cases), autoimmune disorder (1 case juvenile rheumatoid arthritis), burns (6 cases), post amputation wounds in Buerger's disease (15 cases), cellulitis (6 cases), venous malformations (10 cases), and deep femoral soft tissue injuries (2 cases) were studied. They have identified that the healing of diabetic wounds by coffee grounds depends on the size and depth of the wound. Wound drying occurs at week 8, and skin epithelial closure occurs at week 12–16, depending on the size of the wound. In juvenile rheumatoid arthritis patients, soft tissue and skin covered the wound with a typical scar by the eighth week. In the case of venous malformations (Klippel–Trenaunay syndrome), ground coffee could stop bleeding from the subcutaneous layer at week 8, which was difficult to stop with tight sutures only.82
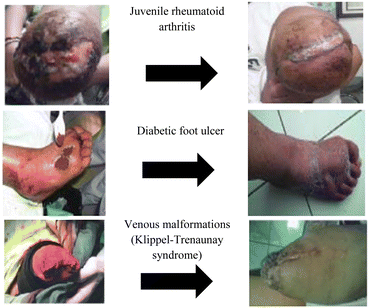 |
| Fig. 3 Wound healing by coffee through human study.81 Adopted with permission from ref. 81. | |
4.1.1. Deodorization.
The aspect that distinguishes coffee from other wound remedies is the coffee aroma. Coffee contains distinctive aromatic compounds. Therefore, the use of coffee as a wound medicine can disguise the smell of wounds or other unpleasant drugs and increase enthusiasm for the wound healing process.95
4.1.2. Acidity aspects.
During the wound healing process, the increase in proteases and the release of oxygen will affect the pH level on the wound surface to become acidic. This condition will reduce the toxicity of bacteria as ammonia products increase collagen breakdown in wounds, trigger angiogenesis, and increase macrophage, fibroblast, and enzyme activity. If the pH of the wound exudate is around 7.3, the growth of bacteria in the wound can result in inflammation or infection.96
A low pH environment can suppress bacterial growth, control infection, release oxygen, alter protease activity, reduce the bacterial end product toxicity, and promote epithelialization and angiogenesis. Acids for topical application, including citric acid, acetic acid, ascorbic acid, boric acid, and alginic acid, have been reported to control infection and promote wound healing.97 The level of acidity also plays an essential role in optimizing the inflammatory phase in preventing the timing of these phases, which can be caused by microbial contamination.96
Coffee has a pH ranging from 4.5–5.0, almost the same as the skin's natural pH (4.5–5.5), and reported to be the optimum pH to accelerate wound healing.81,98 In this case, coffee can maintain the pH of the wound to be slightly acidic, reducing the effect of increasing ammonia (alkaline) as a microbial product. Although a wound pH of 4–6 creates an unfavorable environment for bacterial growth, this will reduce the chances of inflammatory complications. Arimbi and Yuwono96 have reported the clinical effect of coffee acidity on wound healing mechanisms.
Besides that, the pH profiles of healthy skin and acute and chronic wounds differ significantly. Chronic wounds have an alkaline pH, whereas healthy skin has a slightly acidic pH.99,100 It has also been reported that open wounds typically have a neutral to alkaline pH in the pH range of 6.5 to 8.5, while chronic wounds have a pH range of 7.2 to 8.9.101 It has been shown that wounds with a more alkaline pH have a lower healing rate than wounds with a pH close to 7. Although pH affects protease production and bacterial proliferation in wounds, there is little evidence to suggest an effect on ECM synthesis and degradation.99 The reduction of wound pH during the healing stage could help to identify wounds that do not heal early and advise on the most appropriate treatment.99 On the other hand, alkaline pH is not always detrimental; for example, it has been reported to play an essential role in restoring skin grafts that require an alkaline environment.98
4.1.3. Antioxidant activity.
ROS radical formation plays a vital role in delayed wound healing. Coffee contains many polyphenol constituents as antioxidants in controlling ROS radicals. Oxidative stress occurs following the pro-oxidant/antioxidant balance decrease in chronic wounds, causing a delay in healing. A good balance between the endogenous antioxidant defense system and oxidative stress is beneficial for wound healing under redox control.102 Caffeinated coffee and decaffeinated coffee act as antioxidant and anti-inflammatory agents, which can be beneficial during the therapeutic processes or be used as preventive agents in pathological situations. The therapeutic potential of coffee is derived from its bioactive compounds, especially chlorogenic acid, caffeine, caffeic acid, cafestol, and kahweol. Together, the compounds have been shown to reduce inflammation, accelerate wound healing, and modulate inflammatory and neuropathic pain in animal models.103
The function of topical antioxidants in coffee grounds is critical in diabetic or autoimmune ulcers (e.g., juvenile rheumatoid arthritis).81 Pergolizzi et al.104 evaluated the topical anti-inflammatory activity of an ointment prepared using methanol extracts of green robusta coffee beans via histology of carrageenan-induced leg edema. The methanol extract from coffee was proven to scavenge the increase in free radicals by the DPPH test, thereby contributing to anti-inflammatory activity. The antioxidant and radical scavenging activities of coffee ground biomass and chlorogenic acid can accelerate wound healing by controlling overexposure to wound oxidative bed stress.84 In addition, the systemic antioxidant activity of chlorogenic acid in the wound healing process was also studied by Bagdas et al.102 This study confirmed that chlorogenic acid improved wound healing, increased hydroxyproline content, reduced glutathione, decreased nitric oxide/malondialdehyde levels, and did not affect the superoxide dismutase/catalase levels of the wound bed. Chlorogenic acid also reduced the level of lipid peroxidation. Therefore, chlorogenic acid, one of the most extensive constituents of coffee, is a good candidate for diabetic wound management both for topical application and dietary intake.
4.1.4. Antimicrobial activity.
Daglia et al.105 confirmed that coffee contains antimicrobial activity due to the formation of other compounds produced during roasting, involving the Maillard reaction, caramelization of carbohydrates, and product decomposition due to heat. For example, robusta coffee grounds inhibited methicillin-resistant S. aureus (MRSA)81 due to phenolic acidity and hyperosmolarity when mixed with wound fluid. Plain caffeine, trigonelline, chlorogenic acid, caffeic acid, and protocatechuic acid at 2.0 mg mL−1 exerted a similar effect against S. mutans.106
Caffeine or caffeic acid provides an antibacterial effect against S. aureus.107–109 Caffeic acid anilides with an electron donor group at the p position of the benzene ring have better bacterial inhibitory activity against B. subtilis.110 Caffeine also inhibits the bacterium E. coli O157:H7.111 Caffeine–isophthalic acid, theophylline–trimesic acid, and caffeine–trimesic acid cocrystals were reported to inhibit pathogenic bacteria, namely K. pneumonia, A. baumannii, P. aeruginosa, and E. coli.112 Caffeic acid, gallic acid, and pyrogallol also show potential as antimicrobial agents, although they have no direct antibacterial and antifungal action.113 Protocatechuic acid, chlorogenic acid, and caffeine showed powerful effects on E. cloacae and S. marcescens. Trigonelline, caffeine, and protocatechuic acid were natural antimicrobial agents against S. enterica.114 The alkaloid compounds yohimbine and vincamine (indole-type), scopolamine and atropine (tropane-type), colchicine (tropolone-type), allantoin (imidazolidine-type), trigonelline (pyridine-type) as well as synephrine, octopamine, and capsaicin (exocyclic amine-type); the flavonoid derivatives genistein, quercetin, apigenin, silymarin, naringin, and silibinin; and phenolic acids, namely gallic acid, chlorogenic acid, caffeic acid, and quinic acid, have been reported to have strong antibacterial effects against E. coli, P. mirabilis, P. aeruginosa, K. pneumoniae, A. baumannii, E. faecalis, S. aureus, and B. subtilis.115
In addition, the coffee extract is known to inhibit the growth of C. albicans,116,117 possibly due to the effect of caffeine.118 Caffeine prevents biofilm formation in a concentration-dependent manner.119 Chlorogenic acid exhibits antifungal activity in an energy-independent manner, without hemolytic effects on human erythrocytes or disrupting the cell membrane structure.120 Ma121 synthesized chlorogenic acid derivatives with a combination of protected chlorogenic acid or p-octyloxyaniline and selected amino acids. Most of the compounds showed significant potency against C. neoformans and Candida species. The 4,5-dihydroxyl group in the quinic acid group is required for activity, and introducing a free amino group increases activity against A. fumigatus. da Silva122 tested the antifungal activity of chlorogenic acid against fluconazole-resistant strains of Candida spp. Chlorogenic acid can decrease cell viability, increase mitochondrial depolarization potential and reactive oxygen species production, DNA fragmentation, and phosphatidylserine externalization, which indicate apoptotic processes. In addition, chlorogenic acid showed a significant interaction with the ALS3 active site residue of C. albicans, which is vital in the process of adhesion and resistance to fluconazole. In addition, it was also reported that the lower concentration of coffee ground extracts (0.5 mg mL−1) showed stronger inhibition against C. krusei and C. parapsilosis.123
4.2. Tea
A green tea extract (GTE), Camellia sinensis, contains antioxidant, anti-inflammatory, antimicrobial, anticarcinogenic, and antimutagenic properties, reducing adhesion formation, and improving wound healing.124–130 Based on the in vitro study on LPS-stimulated human oral gingival epithelial keratinocytes, GTE produces an anti-inflammatory response activating the nuclear factor erythroid 2-related factor 2 (Nrf2) pathway increasing levels of the antioxidant protein heme oxygenase-1 (HO-1).131 In addition, GTE exhibited anti-diabetic activity and was also crucial in promoting diabetic wound healing by stimulating angiogenesis.132
Tea polyphenols can support the mechanism of wound healing. Green tea contains catechins, polyphenolic or flavonoid compounds, polysaccharide conjugates, amino acids, caffeine, and vitamins.133 Catechins are the primary green tea polyphenolic compounds, including epigallocatechin, epigallocatechin-3-gallate, epicatechin-3-gallate, epicatechin, gallocatechin, and gallocatechin gallate. Theaflavins and thearubigins are commonly found in black tea.134 A green tea polyphenol complex accelerates advanced tissue granulation, epithelialization formation, and activation of transglutaminase expression epidermal morphogenesis to repair skin injuries.128
Epigallocatechin-3-gallate is the most common green tea catechin. Collagen sponges containing epigallocatechin-3-gallate at low concentrations improved diabetic rats' wound healing by accelerating angiogenesis and re-epithelialization and increased cellular reorganization of granulation tissue by triggering cellular reorganization by triggering myofibroblast activity.135 Epigallocatechin-3-gallate acted as an anti-inflammatory agent and antioxidant to improve wound healing and reduce scar tissue formation. Epigallocatechin-3-gallate affects transforming growth factor -β1 in the collagen gel contraction inhabited by fibroblast cells through myofibroblast differentiation and expression of connective tissue growth factor genes; and reduces the expression of collagen type I gene regulation.136 In addition, epigallocatechin-3-gallate regulates cytokine secretion and skin cell activation during wound healing.137 Epigallocatechin-3-gallate also induces differential effects between normal and tumor cells, making it helpful in treating wounds or certain skin conditions characterized by altered metabolism or cellular activity.138 On the other hand, catechins have clinically mild side effects on laparotomy wound healing in mice.139
Several composite products from tea constituents have been investigated for wound healing. Shahrahmani et al.140 developed an ointment based on GTE. An electrospinning technique by Sadri et al.141 produced an environmentally friendly composite GTE wound dressing made from chitosan and polyethylene oxide. When tested in a mouse model, a composite based on polyethylene oxide, green tea, and chitosan showed the best healing effect compared to other wound dressings. In this composite, GTE helped reduce inflammation, keep the wound surface moist, and increase the rate of wound healing. Qin et al.130 combined green tea polyphenols and chitosan to heal male Sherman rat wounds by accelerating epithelialization. The combination of epigallocatechin gallate with gold nanoparticles and lipoic acid was shown to significantly accelerate wound healing in rat skin through its anti-inflammatory and antioxidant effects.142 Chen et al.143 conducted studies using green tea polyphenols encapsulated in hydrogels made from polyvinyl alcohol and alginate. The hydrogel enhanced diabetic wound healing in a mouse model by regulating the PI3K/AKT signalling pathway. Kim et al.137 showed that adding one wt% epigallocatechin-3-gallate in an electrospun dressing consisting of poly(lactic-co-glycolic acid) could promote wound healing full-thickness accelerating cell infiltration, re-epithelialization, and angiogenesis. Several studies utilizing tea in wound healing mechanisms are summarized in Table 3.
Table 3 Wound healing activity by utilizing the active constituents of tea
Preparation |
Test model |
Results |
Ref. |
The mixture of Vaseline and GTE |
36 male Wistar rats |
The duration of healing in the GTE group was significantly reduced (P = 0.05) in the first two weeks than the Vaseline, so GTE helped in the wound healing process. |
127
|
|
Tea plant methanol extract |
NIH3T3 fibroblast cells |
Extract exhibits important apoptotic properties and wound healing potential. |
129
|
|
Green tea drink |
Male Wistar rats |
Drinking green tea can accelerate epithelial reform on the third day after surgery. |
133
|
|
Green tea ointment |
99 primiparous women |
Green tea showed better wound healing properties and effectively relieved episiotomy pain through the Redness, Edema, Ecchymosis, Discharge, Approximation (REEDA) test. |
142
|
|
GTE and hyaluronic acid |
Rats |
GTE microneedles and hyaluronic acid were not cytotoxic to human embryonic kidney cells (293T), mouse muscle cells (C2C12), and Chinese hamster ovary cells (CHO-K1). The material reduces the growth of bacteria at the site of the infected wound on the skin and enhances the wound healing process. |
144
|
|
GTE |
34 male Wistar rats |
GTE significantly reduces the healing time of surgical wounds and burns. |
145
|
|
Tea extract |
Sprague Dawley rat male |
Wounds treated with extracts contained less inflammatory cells and more collagen and angiogenesis, so they healed faster, with a smaller scar width than controls. |
146
|
|
Green tea ethanolic extract |
36 male Wistar rats |
GTE can help in the wound healing process, especially for burns |
147
|
|
Green tea |
Rats |
Green tea significantly reduces the size of the burn, making it practical for the healing process of second-degree burns. |
148
|
4.2.1. Antioxidant activity.
Tea contains high antioxidants due to various polyphenols that can modulate oxidative stress in vivo, especially epigallocatechin-3-gallate, epicatechin-3-gallate, theaflavins, and thearubigins.149–151 Tea polyphenols can scavenge ROS and nitrogen species and chelate redox-active transition metal ions indirectly through:149 (a) inhibition of nuclear factor-κB, redox-sensitive transcription factors, and activator protein-1, (b) inhibition of pro-oxidant enzymes such as inducible nitric oxide synthase, lipoxygenase, cyclooxygenase, and xanthine oxidase, and (c) induction of phase II and antioxidant enzymes such as glutathione S-transferases and superoxide dismutase. Chinese green tea has been shown to have antioxidant activity against H2O2, and O2−.152 Black tea, green tea, brown rice green tea, Malva tea, and herbal tea showed relatively high antioxidant activity in scavenging the ABTS cation radical and DPPH free radical.153
The total antioxidant capacity of tea is not related to the specific type of polyphenol but rather to the combined activity of various antioxidants. Green tea has higher antioxidants than black tea.154 When consuming green tea as a capsule supplement, the absorption and plasma antioxidant activity of flavanols are increased compared to just drinking black or green tea.155 Consuming 300 to 450 mL green tea can significantly increase the total antioxidant capacity of plasma, depending on the dose.156 In animal model studies of skin, colon, lung, liver, and pancreatic cancers, tea polyphenols inhibited the carcinogen-induced increase in the oxidized DNA base, 8-hydroxy-2′-deoxyguanosine. Green and black teas in animal models of atherosclerosis improved lipoprotein resistance to oxidation ex vivo.149 Having demonstrated beneficial effects under those pathological conditions suggests that high antioxidant content in tea could also be very beneficial for healing chronic wounds.
4.2.2. Antimicrobial activity.
Theaflavin is one of the constituents that play a role in the antimicrobial mechanism of tea.157 Theaflavins can suppress the activity of 1-deoxy-D-xylulose 5-phosphate reductoisomerase, which contributes to the terpenoid MEP biosynthetic pathway and is a validated antimicrobial target.157 Park et al.144 reported that GTE and hyaluronic acid showed a ∼95% reduction in Gram-negative (S. typhimurium, E. coli, and P. putida) and Gram-positive (B. subtilis and S. aureus) bacteria. Tea polyphenols also inhibited the growth of violin production in C. violaceum 12472 (nearly 98% at 3125 mg mL−1) and P. aeruginosa by reducing total proteolytic activity and swarming motility, elastase, and biofilm formation, depending on concentration. The authors explained that they could develop new non-antibiotic quorum sensing inhibitors from tea polyphenols, which would act as antivirulence compounds to control bacterial infections without killing the bacteria.158 The Nigerian black tea inhibits E. coli (sensitive between 6%, 8% and 10% aqueous extract and 2% to 10% methanol extract), S. aureus (sensitive between 4% to 10% aqueous extract and 2% to 10% methanol extract sensitive between 4% to 10% aqueous extract and 2% to 10% methanol extract sensitive between 4% to 10% aqueous extract and 2% to 10% methanol extract sensitive between 4% to 10% aqueous extract and 2% to 10% methanol extract sensitive between 4% to 10% aqueous extract and 2% to 10% methanol extract sensitive between 4% to 10% aqueous extract and 2% to 10% methanol extract), P. aeruginosa (sensitive to 2% to 10% aqueous extracts and between 6%, 8% and 10% methanol extracts), and B. subtilis (sensitive to 4%, 6% and 8% aqueous extracts and 4% to 10% methanol extracts but sensitive to 10% aqueous extracts).159
Epigallocatechin-3-gallate has been reported to inhibit S. maltophilia, MRSA, and multidrug-resistant P. aeruginosa.160,161 Green tea leaf decoction inhibited S. aureus (inhibition zone of 19 mm) and V. parahaemolyticus (inhibition zone of 17.5 mm). GTE in methanol showed the same inhibition zone against Pseudomonas spp. (clear zone of 18 mm).162 The combination of silver nanoparticles with tea extracts was also reported to inhibit S. aureus (ATCC 29213), P. aeruginosa (ATCC 27853), E. coli (ATCC 25922), K. pneumoniae (ATCC 700603), and S. enterica (ATCC 14028).163 The tea constituents' antimicrobial properties are summarized in Table 4.
Table 4 Antimicrobial properties of compounds contained in tea
Constituents |
Effect of antimicrobials |
Ref. |
Epicatechin-(4β → 8)-epicatechin-(4β → 8, 2β → O → 7)-catechin |
These compounds have strong properties against P. intermedia and P. gingivalis |
164
|
|
Catechin, chlorogenic acid, dan phloridzin |
All phenolics inhibited E. coli O157:H7, L. innocua, and P. chrysogenum at 25 mM. |
165
|
|
(−)-epicatechin gallate (ECg) |
Relatively low concentrations of (−)-epicatechin gallate sensitized clinical isolates of MRSA to oxacillin levels. (−)-Epicatechin gallate intercalates into a phospholipid bilayer affecting virulence and antibiotic resistance by interfering with the function of critical processes associated with the bacterial cytoplasmic membrane. |
166
|
|
Catechin |
Catechin has antibacterial activity against S. aureus. |
167
|
|
Catechin-lysozyme |
Depending on the concentration, the film has inhibitory activity against E. coli and S. aureus |
168
|
|
Epigallocatechin gallate |
Epigallocatechin gallate inhibits the growth and biofilm formation of S. mutans and does not interact with streptococcal lipoteichoic acid. |
169
|
|
(−)-Epigallocatechin-3-gallate, (−)-epicatechin-3-gallate, (−)-gallocatechin-3-gallate, (−)-catechin-3-gallate, theaflavin-3′-gallate, theaflavin-3, 3′-digallate, and theaflavin-3-gallate |
These compounds have antimicrobial activity at the nanomolar level in B. Cereus and are mostly more active than tetracycline or vancomycin. |
170
|
|
Epigallocatechin-3-O-gallate, epigallocatechin, castalagin, punicalagin, tannic acid, prodelphinidin, procyanidins, geraniin, black tea theaflavin, and green tea polyphenols treated with loquat polyphenol oxidase |
The mean minimum inhibitory concentrations of all polyphenols against S. aureus (192 ± 91 g mL) and the genus Vibrio (162 ± 165 g mL−1) were much lower than those against genus Salmonella (795 ± 590 g mL−1) and E. coli (1519 ± 949 g mL−1). |
171
|
|
Black tea processing waste containing catechins and theaflavins |
The extracts showed antimicrobial activity against S. flexneri (1.33–3.89 mm), S. aureus (1.26–3.65 mm), and B. cereus (1.87–3, 90 mm) |
172
|
|
Epigallocatechin gallate |
Epigallocatechin gallate enhances the curcumin's antibacterial activity against multidrug-resistant A. baumannii. |
173
|
|
Pu-erh tea constituents |
The tea constituents significantly inhibited the growth of S. typhimurium (0.18 mg mL−1), L. monocytogenes (0.07 mg mL−1), S. faecalis (0.50 mg mL−1), B. anthracis (0.48 mg mL−1), and E. coli (0.42 mg mL−1) |
174
|
|
|
|
Green and black tea extract |
Tea extract is an antimicrobial agent for S. aureus ATCC 25923 and E. coli ATCC 25922, which are resistant to antibiotics. Also, it can use the combination of GTE with penicillin to manage resistant pathogenic bacteria. |
175
|
5. Future directions and prospects
5.1. Coffee and tea constituents for wound healing
Coffee and tea contain many natural active ingredients that help the wound healing process and are inexpensive and easy to find. There are still many other active constituents that have not been identified; it is exciting and challenging at the same time because raw coffee extracts can have wound healing activity, but pure compounds such as caffeine harm the healing process.20 This effect can be minimized by reducing the amount of caffeine content of coffee through the coffee decaffeination process, which is the choice of the decaffeination method. It need to be considered to keep the other active constituents. Likewise, other minor compounds are reported to be anti-angiogenic, such as cafestol and kahweol.86,87 In order to avoid the effect of this minor constituent, it is imperative to study the dose and whether the effect at that dose is significant when combined with other active constituents such as chlorogenic acid. A combination of various constituents is required for adequate healing activity, and it remains to be studied to find the ideal combination.
Generally, complex active compounds in coffee and tea can be polar and non-polar. The selection of the correct type of solvent and extraction method will impact the effectiveness of the wound healing mechanism. For example, when selecting a target compound, chlorogenic acid, the researcher must identify the solubility properties and characteristics of the compound. If the chlorogenic acid is polar, it can be extracted using a solvent of suitable polarities, such as methanol. Both polar and non-polar fractions need to be investigated in vivo and in vitro. It should be noted that some solvents may be toxic to skin cells, so the extraction process must ensure that the final isolate is solvent-free. Knowledge of the characteristics of the extracted active ingredients will also determine the appropriate extraction method. Some compounds may be sensitive to high temperatures. Therefore, controlling the temperature during the extraction process is very important to avoid damage to the active compounds.
There are exciting things about the active compounds that have been reported to have performance as antibiofilms.63,144 Although it is not known precisely how the antibiofilm mechanism is, it is crucial to explore the contribution and dosage of these compounds in inhibiting the formation of various types of biofilms formed by resistant microbes. In the future, this knowledge will be advantageous in reducing the use of antibiotics that cause microbial resistance.
5.2. Wound dressing novel synthesis
A number of methods have been used to synthesize wound dressing hydrogels based on cellulose biopolymers.55,176 When synthesizing new wound dressings, it is also necessary to pay attention to how they interact with the supporting components (e.g. addition of polymers, drugs, and other composite materials) and their changes. For example, collagen has been tested to accelerate wound healing, but this protein is expensive, and has no antimicrobial properties, and limited exudate absorption. It is also possible to consider synergistic composites with other types of support materials to produce an ideal novel by considering these weaknesses. Chitosan has also demonstrated its biocompatibility in tissue engineering. However, its insolubility in water poses a challenge for bonding with cellulose and tea and coffee compounds. Learning how to develop novel strategies by using simple methods and selecting appropriate materials is vital. Therefore, knowledge of the characteristics of raw materials is important for producing ideal wound dressing composites.
Cellulose is a hydrophilic biopolymer that does not readily bind to hydrophobic active constituents. Therefore, it is necessary to add an emulsifier or modify the cellulose to be more hydrophobic, and an understanding of the hydrophilicity of other biopolymers that need to be considered as cellulose composites to be explored. The nature of the active ingredients will also impact the drug release rate. One of the conditions for an ideal wound dressing is to absorb excess exudate. This aspect should be considered in selecting and studying the active ingredients and biopolymers and their release profile. This is where the importance of modifying the properties of cellulose to be highly hydrophilic is. The dosage of active ingredients and their level of toxicity also need to be studied in formulating wound dressing biomaterials.
6. Conclusions
Cellulose is a natural biopolymer that has demonstrated its biocompatibility in tissue engineering applications. On the other hand, the problem of antimicrobial, reactive oxygen, and antibiotic resistance has also become a focus for biomaterials synthesis for biomedical applications. In general, the bioactive constituents of coffee and tea have demonstrated their activity in wound healing through clinical studies (Table 5), including antimicrobial and antioxidant studies. Considering the perspectives and challenges ahead, the combination of cellulose with the active ingredients of coffee and tea shows its potential as a promising novel wound dressing.
Table 5 Summary of characteristics of coffee, tea, and cellulose as wound dressing materials
Materials |
Reported compounds |
Properties |
Coffee |
Chlorogenic, caffeine, caffeic acid, diterpenes (kahweol and cafestol), melanoidin, caffeine–isophthalic acid, theophylline–trimesic acid, caffeine–trimesic acid cocrystals protocatechuic acid, gallic acid, pyrogallol, trigonelline, and formation of other compounds produced during roasting, such as the Maillard reaction, caramelization of carbohydrates, and product decomposition |
• Lowering MMP-1 levels |
• Anti-inflammatory |
• Accelerating epithelialization |
• Antioxidant |
• A pleasing odor (especially roasted coffee) |
• Increased wound dressing longevity |
• Absorption capacity to maintain a moist wound environment (coffee powder) |
• Autolytic debridement capacity |
• Minimal adverse reactions |
• Acidity effect |
• Antagonist negates the adenosine receptors, limits keratinocyte cell proliferation, and inhibits epithelialization depending on the dose (caffeine) |
• Antitumorigenic (cafestol) |
• Anti-angiogenesis (cafestol and kahweol) |
• Antimicrobial |
|
Tea |
Polyphenolic, flavonoid, catechins, theaflavins, thearubigins, epigallocatechin, epigallocatechin-3-gallate, epicatechin-3-gallate, epicatechin, gallocatechin, gallocatechin gallate, caffeine, polysaccharide conjugates, amino acids, and vitamins |
• Antioxidant |
• Anti-inflammatory |
• Antimicrobial |
• Anticarcinogenic |
• Anti-diabetic |
• Antimutagenic |
• Reducing adhesion formation |
• Promote angiogenesis |
• Accelerate advanced tissue granulation, epithelialization formation, and activation of transglutaminase expression epidermal morphogenesis (green tea polyphenol) |
|
Cellulose |
Structure and functional groups |
• Biocompatible |
• Biodegradable |
• Low toxicity (depending on preparation) |
• Easy to modify |
• Hydrophilic |
• High absorption capacity |
• Nanostructure (especially BC) |
• High porosity |
• Purity (especially BC) |
• Permeability to gases and liquids |
• Mechanical resistance |
Abbreviation
BC | Bacterial cellulose |
CMC | Carboxymethyl cellulose |
DDS | Drug delivery system |
APIs | Active pharmaceutical ingredients |
MRSA | Methicillin-resistant S. aureus (MRSA) |
GTE | Green tea extract |
ROS | Reactive oxygen species |
ABTS | 2,2′-Azino-bis(3-ethylbenzothiazoline-6-sulfonic acid) |
DPPH | 1,1-diphenyl-2-picrylhydrazyl |
MEP | 2-methyl-D-erythritol 4-phosphate |
Conflicts of interest
There are no conflicts to declare.
Acknowledgements
This work was supported by Direktorat Jenderal Pendidikan Tinggi, Riset, dan Teknologi, Kementrian Pendidikan, Kebudayaan, Riset, dan Teknologi, Republic of Indonesia.
References
- M. H. Farzaei, Z. Abbasabadi, M. R. Shams-Ardekani, M. Abdollahi and R. Rahimi, A comprehensive review of plants and their active constituents with wound healing activity in traditional Iranian medicine, Wounds, 2014, 26(7), 197–206 Search PubMed , https://europepmc.org/article/med/25856320.
- E. R. Ghomi, S. Khalili, S. N. Khorasani, R. E. Neisiany and S. Ramakrishna, Wound dressings: Current advances and future directions, J. Appl. Polym. Sci., 2019, 136(27), 47738, DOI:10.1002/app.47738.
-
S. Thomas, The role of dressings in the treatment of moisture-related skin damage, World Wide Wounds 2008. https://www.worldwidewounds.com/2008/march/Thomas/Maceration-and-the-role-of-dressings.html#:~:text=Local%20treatment%<?pdb_no 20of?>20of<?pdb END?>%20moisture%2Drelated,provide%20protection%<?pdb_no 20to?>20to<?pdb END?>%20periwound%20skin.
- J. Dissemond, M. Augustin, S. A. Eming, T. Goerge, T. Horn, S. Karrer, H. Schumann and M. Stücker, Modern wound care – practical aspects of non-interventional topical treatment of patients with chronic wounds, Ger. Hist., 2014, 12, 541–554, DOI:10.1111/ddg.12351.
- Y. Pei, D. Ye, Q. Zhao, X. Wang, C. Zhang, W. Huang, N. Zhang, S. Liu and L. Zhang, Effectively promoting wound healing with cellulose/gelatin sponges constructed directly from a cellulose solution, J. Mater. Chem. B, 2015, 3(38), 7518–7528, 10.1039/C5TB00477B.
- B. George and T. V. Suchithra, Plant-derived bioadhesives for wound dressing and drug delivery system, Fitoterapia, 2019, 137, 104241, DOI:10.1016/j.fitote.2019.104241.
- E. Pinho and G. Soares, Functionalization of cotton cellulose for improved wound healing, J. Mater. Chem. B, 2018, 6(13), 1887–1898, 10.1039/C8TB00052B.
- N. T. Laçin, Development of biodegradable antibacterial cellulose based hydrogel membranes for wound healing, Int. J. Biol. Macromol., 2014, 67, 22–27, DOI:10.1016/j.ijbiomac.2014.03.003.
- J. Li, C. Cai, J. Li, J. Li, J. Li, T. Sun, L. Wang, H. Wu and G. Yu, Chitosan-based nanomaterials for drug delivery, Molecules, 2018, 23(10), 2661, DOI:10.3390/molecules23102661.
- G. Huang and H. Huang, Application of hyaluronic acid as carriers in drug delivery, Drug Deliv., 2018, 25(1), 766–772, DOI:10.1080/10717544.2018.1450910.
- H. H. Tønnesen and J. Karlsen, Alginate in drug delivery systems, Drug Dev. Ind. Pharm., 2022, 28(6), 621–630, DOI:10.1081/DDC-120003853.
- D. Jain and D. Bar-Shalom, Alginate drug delivery systems: Application in context of pharmaceutical and biomedical research, Drug Dev. Ind. Pharm., 2014, 40(12), 1576–1584, DOI:10.3109/03639045.2014.917657.
-
G. Serafica, R. Mormino, G. A. Oster, K. E. Lentz and K. P. Koehler, Microbial cellulose wound dressing for treating chronic wounds, US7704523B2, 2010, https://patentimages.storage.googleapis.com/1e/c2/b2/2462031421d165/US7704523.pdf.
- J. Kucińska-Lipka, I. Gubanska and H. Janik, Bacterial cellulose in the field of wound healing and regenerative medicine of skin: recent trends and future prospectives, Polym. Bull., 2015, 72, 2399–2419, DOI:10.1007/s00289-015-1407-3.
- R. Schwartz and N. Al-Mutairi, Topical Antibiotics in Dermatology: An Update, J. Eur. Acad. Dermatol. Venereol., 2010, 17(1), 1–19 Search PubMed , http://www.gulfdermajournal.net/pdf/2010-04/1.pdf.
- P. S. Stewart and J. W. Costerton, Antibiotic resistance of bacteria in biofilms, Lancet, 2001, 358(9276), 135–138, DOI:10.1016/S0140-6736(01)05321-1.
- N. Høiby, T. Bjarnsholt, M. Givskov and O. Ciofu, Antibiotic resistance of bacterial biofilms, Int. J. Antimicrob. Agents, 2010, 35(4), 322–332, DOI:10.1016/j.ijantimicag.2009.12.011.
- R. Edwards and K. G. Harding, Bacteria and wound healing, Curr. Opin. Infect. Dis., 2004, 17(2), 91–96 CrossRef PubMed . https://journals.lww.com/co-infectiousdiseases/Abstract/2004/04000/Bacteria_and_wound_healing.4.aspx.
- P. Zarrintaj, A. S. Moghaddam, S. Manouchehri, Z. Atoufi, A. Amiri, M. A. Amirkhani, M. A. Nilforoushzadeh, M. R. Saeb, M. R. Hamblin and M. Mozafari, Can regenerative medicine and nanotechnology combine to heal wounds? The search for the ideal wound dressing, Nanomedicine, 2017, 12(19), 2403–2422, DOI:10.2217/nnm-2017-0173.
- N. Ojeh, O. Stojadinovic, I. Pastar, A. Sawaya, N. Yin and M. Tomic-Canic, The effects of caffeine on wound healing, Int. Wound J., 2014, 13(5), 605–613, DOI:10.1111/iwj.12327.
- E. N. Hosny, H. G. Sawie, M. E. Elhadidy and Y. A. Khadrawy, Evaluation of antioxidant and anti-inflammatory efficacy of caffeine in rat model of neurotoxicity, Nutr. Neurosci., 2019, 22(11), 789–796, DOI:10.1080/1028415X.2018.1446812.
- C. V. S. Raj and S. Dhala, Effect of naturally occurring xanthines on bacteria, Appl. Environ. Microbiol., 1965, 13(3), 432–436 CrossRef CAS , https://aem.asm.org/content/13/3/432.short.
- M. Alavi, Modifications of microcrystalline cellulose (MCC), nanofibrillated cellulose (NFC), and nanocrystalline cellulose (NCC) for antimicrobial and wound healing applications, e-Polymers, 2019, 19(1) DOI:10.1515/epoly-2019-0013.
- P. Zahedi, I. Rezaeian, S.-O. Ranaei-Siadat, S.-H. Jafari and P. Supaphol, A review on wound dressings with an emphasis on electrospun nanofibrous polymeric bandages, Polym. Adv. Technol., 2010, 21(2), 77–95, DOI:10.1002/pat.1625.
- T. Carvalho, G. Guedes, F. L. Sousa, C. S. R. Freire and H. A. Santos, Latest advances on bacterial cellulose-based materials for wound healing, delivery systems, and tissue engineering, Plant Biotechnol. J., 2019, 14(12), 1900059, DOI:10.1002/biot.201900059.
- W. Czaja, A. Krystynowicz, S. Bielecki and R. M. Brown Jr, Microbial cellulose—the natural power to heal wounds, Biomaterials, 2006, 27(2), 145–151, DOI:10.1016/j.biomaterials.2005.07.035.
- R. Portela, C. R. Leal, P. L. Almeida and R. G. Sobral, Bacterial cellulose: a versatile biopolymer for wound dressing applications, Microb. Biotechnol., 2019, 12(4), 586–610, DOI:10.1111/1751-7915.13392.
- O. Portal, W. A. Clark and D. J. Levinson, Microbial cellulose wound dressing in the treatment of nonhealing lower extremity ulcers, Wound, 2009, 21(1), 1–3 Search PubMed . https://europepmc.org/article/med/25904579.
- D. R. Solway, W. A. Clark and D. J. Levinson, A parallel open-label trial to evaluate microbial cellulose wound dressing in the treatment of diabetic foot ulcers, Int. Wound J., 2011, 8(1), 69–73, DOI:10.1111/j.1742-481X.2010.00750.x.
- V. Kanikireddy, K. Varaprasad, T. Jayaramudu, C. Karthikeyan and R. Sadiku, Carboxymethyl cellulose-based materials for infection control and wound healing: A review, Int. J. Biol. Macromol., 2020, 164, 963–975, DOI:10.1016/j.ijbiomac.2020.07.160.
- A. L. Harkins, S. Duri, L. C. Kloth and C. D. Tran, Chitosan–cellulose composite for wound dressing material. Part 2. Antimicrobial activity, blood absorption ability, and biocompatibility, J. Biomed. Mater. Res. Part B Appl. Biomater., 2014, 102(6), 1199–1206, DOI:10.1002/jbm.b.33103.
- W.-C. Lin, C.-C. Lien, H.-J. Yeh, C.-M. Yu and S.-H. Hsu, Bacterial cellulose and bacterial cellulose–chitosan membranes for wound dressing applications, Carbohydr. Polym., 2013, 94(1), 603–611, DOI:10.1016/j.carbpol.2013.01.076.
- W. Huang, Y. Wang, Z. Huang, X. Wang, L. Chen, Y. Zhang and L. Zhang, On-demand dissolvable self-healing hydrogel based on carboxymethyl chitosan and cellulose nanocrystal for deep partial thickness burn wound healing, ACS Appl. Mater. Interfaces, 2018, 10(48), 41076–41088, DOI:10.1021/acsami.8b14526.
- M. Ul-Islam, T. Khan, W. A. Khattak and J. K. Park, Bacterial cellulose-MMTs nanoreinforced composite films: novel wound dressing material with antibacterial properties, Cellulose, 2013, 20, 589–596, DOI:10.1007/s10570-012-9849-3.
- A. R. Unnithan, G. Gnanasekaran, Y. Sathishkumar, Y. S. Lee and C. S. Kim, Electrospun antibacterial polyurethane–cellulose acetate–zein composite mats for wound dressing, Carbohydr. Polym., 2014, 102, 884–892, DOI:10.1016/j.carbpol.2013.10.070.
- A. Konstantinow, T. V. Fischer, J. R. A. Konstantinow, T. V. Fischer and J. Ring, Effectiveness of collagen/oxidised regenerated cellulose/silver-containing composite wound dressing for the treatment of medium-depth split-thickness skin graft donor site wounds in multi-morbid patients: a prospective, non-comparative, single-centre study, Int. Wound J., 2017, 14(5), 791–800, DOI:10.1111/iwj.12698.
- N. Liao, A. R. Unnithan, M. K. Joshi, A. P. Tiwari, S. T. Hong, C.-H. Park and C. S. Kim, Electrospun bioactive poly (ε-caprolactone)–cellulose acetate–dextran antibacterial composite mats for wound dressing applications, Colloids Surf., A, 2015, 469, 194–201, DOI:10.1016/j.colsurfa.2015.01.022.
- F. Yin, L. Lin and S. Zhan, Preparation and properties of cellulose nanocrystals, gelatin, hyaluronic acid composite hydrogel as wound dressing, J. Biomater. Sci. Polym. Ed., 2018, 30(3), 190–201, DOI:10.1080/09205063.2018.1558933.
- Y. Liu, S. Zhou, Y. Gao and Y. Zhai, Electrospun nanofibers as a wound dressing for treating diabetic foot ulcer, Asian J. Pharm., 2019, 14(2), 130–143, DOI:10.1016/j.ajps.2018.04.004.
- A. Haider, S. Haider, I.-K. Kang, A. Kumar, M. R. Kummara, T. Kamal and S. S. Han, A novel use of cellulose based filter paper containing silver nanoparticles for its potential application as wound dressing agent, Int. J. Biol. Macromol., 2018, 108, 455–461, DOI:10.1016/j.ijbiomac.2017.12.022.
- G. F. El Fawal, M. M. Abu-Serie, M. A. Hassan and M. S. Elnouby, Hydroxyethyl cellulose hydrogel for wound dressing: Fabrication, characterization and in vitro evaluation, Int. J. Biol. Macromol., 2018, 111, 649–659, DOI:10.1016/j.ijbiomac.2018.01.040.
- T. Maver, U. Maver, F. Mostegel, T. Griesser, S. Spirk, D. M. Smrke and K. Stana-Kleinschek, Cellulose based thin films as a platform for drug release studies to mimick wound dressing materials, Cellulose, 2015, 22, 749–761, DOI:10.1007/s10570-014-0515-9.
- J. Wu, Y. Zheng, X. Wen, Q. Lin, X. Chen and Z. Wu, Silver nanoparticle/bacterial cellulose gel membranes for antibacterial wound dressing: investigation in vitro and in vivo, Biomed. Mater., 2014, 9(3), 35005, DOI:10.1088/1748-6041/9/3/035005/meta.
- J. Wu, Y. Zheng, W. Song, J. Luan, X. Wen, Z. Wu, X. Chen, Q. Wang and S. Guo, In situ synthesis of silver-nanoparticles/bacterial cellulose composites for slow-released antimicrobial wound dressing, Carbohydr. Polym., 2014, 102, 762–771, DOI:10.1016/j.carbpol.2013.10.093.
- T. Maneerung, S. Tokura and R. Rujiravanit, Impregnation of silver nanoparticles into bacterial cellulose for antimicrobial wound dressing, Carbohydr. Polym., 2008, 72(1), 43–51, DOI:10.1016/j.carbpol.2007.07.025.
- J. Miao, R. C. Pangule, E. E. Paskaleva, E. E. Hwang, R. S. Kane, R. J. Linhardt and J. S. Dordick, Lysostaphin-functionalized cellulose fibers with antistaphylococcal activity for wound healing applications, Biomaterials, 2008, 32(36), 9557–9567, DOI:10.1016/j.biomaterials.2011.08.080.
- Y. Qiu, L. Qiu, J. Cui and Q. Wei, Bacterial cellulose and bacterial cellulose-vaccarin membranes for wound healing, Mater. Sci. Eng. C, 2016, 59, 303–309, DOI:10.1016/j.msec.2015.10.016.
- S. Pal, R. Nisi, M. Stoppa and A. Licciulli, Silver-Functionalized Bacterial Cellulose as Antibacterial Membrane for Wound-Healing Applications, ACS Omega, 2017, 2(7), 3632–3639, DOI:10.1021/acsomega.7b00442.
- S. Wichai, P. Chuysinuan, S. Chaiarwut, P. Ekabutr and P. Supaphol, Development of bacterial cellulose/alginate/chitosan composites incorporating copper (II) sulfate as an antibacterial wound dressing, J. Drug Deliv. Sci. Technol., 2019, 51, 662–671, DOI:10.1016/j.jddst.2019.03.043.
- J. Luan, J. Wu, Y. Zheng, W. Song, G. Wang, J. Guo and X. Ding, Impregnation of silver sulfadiazine into bacterial cellulose for antimicrobial and biocompatible wound dressing, Biomed. Mater., 2012, 7(6), 65006, DOI:10.1088/1748-6041/7/6/065006/meta.
- S. Ye, L. Jiang, J. Wu, C. Su, C. Huang, X. Liu and W. Shao, Flexible amoxicillin-grafted bacterial cellulose sponges for wound dressing: in vitro and in vivo evaluation, ACS Appl. Mater. Interfaces, 2018, 10(6), 5862–5870, DOI:10.1021/acsami.7b16680.
- S. Ye, L. Jiang, C. Su, Z. Zhu, Y. Wen and W. Shao, Development of gelatin/bacterial cellulose composite sponges as potential natural wound dressings, Int. J. Biol. Macromol., 2019, 133, 148–155, DOI:10.1016/j.ijbiomac.2019.04.095.
- S. Simões, A. Figueiras and F. Veiga, Modular hydrogels for drug delivery, J. Biomater. Nanobiotechnol., 2012, 3, 185–199, DOI:10.4236/jbnb.2012.32025.
- D. E. Ciolacu, R. Nicu and F. Ciolacu, Cellulose-based hydrogels as sustained drug-delivery systems, Materials, 2020, 13(22), 2–37, DOI:10.3390/ma13225270.
- T. R. Hoare and D. S. Kohane, Hydrogels in drug delivery: Progress and challenges, Polymer, 2008, 49(8), 1993–2007, DOI:10.1016/j.polymer.2008.01.027.
-
D. E. Ciolacu and D. M. Suflet, Cellulose-based hydrogels for medical/pharmaceutical applications, Biomass as Renewable Raw Material to Obtain Bioproducts of High-Tech Value, 2008, pp. 401–439 DOI:10.1016/B978-0-444-63774-1.00011-9.
- B. Sun, M. Zhang, J. Shen, Z. He, P. Fatehi and Y. Ni, Applications of cellulose-based materials in sustained drug delivery systems, Curr. Med. Chem., 2019, 26(14), 2485–2501, DOI:10.2174/0929867324666170705143308.
- N. Hasan, L. Rahman, S.-H. Kim, J. Cao, A. Arjuna, S. Lallo, B. H. Jhun and J.-W. Yoo, Recent advances of nanocellulose in drug delivery systems, J. Pharm. Investig., 2002, 50, 553–572, DOI:10.1007/s40005-020-00499-4.
- K. Löbmann, J. Wohlert, A. Müllertz, L. Wågberg and A. J. Svagan, Cellulose nanopaper and nanofoam for patient-tailored drug delivery, Adv. Mater. Interfaces, 2017, 4(9), 1600655, DOI:10.1002/admi.201600655.
- R. Kolakovic, L. Peltonen, A. Laukkanen, J. Hirvonen and T. Laaksonen, Nanofibrillar cellulose films for controlled drug delivery, Eur. J. Pharm. Biopharm., 2012, 82(2), 308–315, DOI:10.1016/j.ejpb.2012.06.011.
- M. A. Wsoo, S. Shahir, S. P. M. Bohari, N. H. M. Nayan and S. I. A. Razak, A review on the properties of electrospun cellulose acetate and its application in drug delivery systems: A new perspective, Carbohydr. Res., 2020, 491, 107978, DOI:10.1016/j.carres.2020.107978.
- P. Weyell, U. Beekmann, C. Küpper, M. Dederichs, J. Thamm, D. Fischer and D. Kralisch, Tailor-made material characteristics of bacterial cellulose for drug delivery applications in dentistry, Carbohydr. Polym., 2019, 207, 1–10, DOI:10.1016/j.carbpol.2018.11.061.
- M. Badshah, H. Ullah, A. R. Khan, S. Khan, J. K. Park and T. Khan, Surface modification and evaluation of bacterial cellulose for drug delivery, Int. J. Biol. Macromol., 2018, 113, 526–533, DOI:10.1016/j.ijbiomac.2018.02.135.
- W. Treesuppharat, P. Rojanapanthu, C. Siangsanoh, H. Manuspiya and S. Ummartyotin, Synthesis and characterization of bacterial cellulose and gelatin-based hydrogel composites for drug-delivery systems, Biotechnol. Rep., 2017, 15, 84–91, DOI:10.1016/j.btre.2017.07.002.
- K. J. Edgar, Cellulose esters in drug delivery, Cellulose, 2007, 14, 49–64, DOI:10.1007/s10570-006-9087-7.
- J. T. Orasugh, N. R. Saha, D. Rana, G. Sarkar, M. M. R. Mollick, A. Chattoapadhyay, B. C. Mitra, D. Mondal, S. K. Ghosh and D. Chattopadhyay, Jute cellulose nano-fibrils/hydroxypropylmethylcellulose nanocomposite: A novel material with potential for application in packaging and transdermal drug delivery system, Ind. Crops Prod., 2018, 112, 633–643, DOI:10.1016/j.indcrop.2017.12.069.
- S.-F. Ng and N. Jumaat, Carboxymethyl cellulose wafers containing antimicrobials: A modern drug delivery system for wound infections, Eur. J. Pharm. Sci., 2014, 51, 173–179, DOI:10.1016/j.ejps.2013.09.015.
- J. Bhandari, H. Mishra, P. K. Mishra, R. Wimmer, F. J. Ahmad and S. Talegaonkar, Cellulose nanofiber aerogel as a promising biomaterial for customized oral drug delivery, Int. J. Nanomedicine, 2017, 12, 2021–2031, DOI:10.2147/IJN.S124318.
- B. J. Kong, A. Kim and S. N. Park, Properties and in vitro drug release of hyaluronic acid-hydroxyethyl cellulose hydrogels for transdermal delivery of isoliquiritigenin, Carbohydr. Polym., 2016, 147, 473–481, DOI:10.1016/j.carbpol.2016.04.021.
- D. Jeong, H. K. Kim, J.-P. Jeong, S. D. Dindulkar, E. Cho, Y.-H. Yang and S. Jung, Cyclosophoraose/cellulose hydrogels as an efficient delivery system for galangin, a hydrophobic antibacterial drug, Cellulose, 2016, 23, 2609–2625, DOI:10.1007/s10570-016-0975-1.
- H. Liu, L. Zhang, P. Shi, Q. Zou, Y. Zuo and Y. Li, Hydroxyapatite/polyurethane scaffold incorporated with drug-loaded ethyl cellulose microspheres for bone regeneration, J. Biomed. Mater. Res., 2010, 95B(1), 36–46, DOI:10.1002/jbm.b.31680.
- M. Gouda, A. A. Hebeish and A. Aljafari, Synthesis and characterization of novel drug delivery system based on cellulose acetate electrospun nanofiber mats, J. Ind. Text., 2014, 43(3), 319–329, DOI:10.1177/1528083713495250.
- S. Javanbakht and H. Namazi, Doxorubicin loaded carboxymethyl cellulose/graphene quantum dot nanocomposite hydrogel films as a potential anticancer drug delivery system, Mater. Sci. Eng. C, 2018, 87, 50–59, DOI:10.1016/j.msec.2018.02.010.
- K. Songsurang, K. Siraleartmukul and N. Muangsin, Mucoadhesive drug carrier based on functional-modified cellulose as poorly water-soluble drug delivery system, J. Microencapsul., 2018, 32(5), 450–459, DOI:10.3109/02652048.2015.1046516.
- A. A. Attama, P. A. Akpa, L. E. Onugwu and G. Igwilo, Novel buccoadhesive delivery system of hydrochlorothiazide formulated with ethyl cellulose-hydroxypropyl methylcellulose interpolymer complex, J. Sci. Res. Essay, 2008, 3(6), 343–347, DOI:10.5897/SRE.9000682.
- Y. C. Ching, T. M. S. U. Gunathilake, C. H. Chuah, K. Y. Ching, R. Singh and N.-S. Liou, Curcumin/tween 20-incorporated cellulose nanoparticles with enhanced curcumin solubility for nano-drug delivery: characterization and in vitro evaluation, Cellulose, 2019, 26, 5467–5481, DOI:10.1007/s10570-019-02445-6.
- T. K. Biswas and B. Mukherjee, Plant medicines of indian origin for wound healing activity: A review, Int. J. Low Extrem. Wounds, 2003, 2(1), 25–39, DOI:10.1177/1534734603002001006.
- P. K. Ghosh and A. Gaba, Phyto-extracts in wound healing, J. Pharm. Pharm. Sci., 2013, 16(5), 760–820, DOI:10.18433/J3831V.
- G. Alam, M. P. Singh and A. Sin, Wound healing potential of some medicinal plants, Int. J. Pharm. Sci. Rev. Res., 2011, 9(1), 136–145 Search PubMed . https://www.researchgate.net/publication/258920339_Wound_healing_potential_of_some_medicinal_plants#fullTextFileContent.
- N. Pudasaini, P. P. Upadhyay, C. R. Parker, S. U. Hagen, A. D. Bond and J. Rantanen, Downstream processability of crystal habit-modified active pharmaceutical ingredient, Org. Process Res. Dev., 2017, 21(4), 571–577, DOI:10.1021/acs.oprd.6b00434.
- H. S. Yuwono, The new paradigm of wound management using coffee powder, J. Surg., 2014, 2(2), 25–29 Search PubMed . http://pubs.sciepub.com/js/2/2/2.
- A. A. Winata, H. S. Yuwono and P. Hapsari, Correlation between mmp-1 responses and epithelialization of acute Staphylococcus aureus infected wounds treated by coffee powder, saline gauze, and hydrocolloid, Int. j. gen. med. pharm., 2017, 6(5), 31–38 Search PubMed.
- C. S. Yoon, M. K. Kim, Y. S. Kim and S. K. Lee, In vivo protein expression changes in mouse livers treated with dialyzed coffee extract as determined by IP-HPLC, Maxillofac Plast. Reconstr. Surg., 2018, 40(44), 1–17, DOI:10.1186/s40902-018-0183-z.
- R. C. L. Affonso, A. P. L. Voytena, S. Fanan, H. Pitz, D. S. Coelho, A. L. Horstmann, A. Pereira, V. G. Uarrota, M. C. Hillmann, L. A. C. Varela, R. M. Ribeiro-do-Valle and M. Maraschin, Phytochemical composition, antioxidant activity, and the effect of the aqueous extract of coffee (Coffea arabica L.) bean residual press cake on the skin wound healing, Oxid. Med. Cell. Longev., 2016, 2016, 1923754, DOI:10.1155/2016/1923754.
- B. G. Lania, J. Morari, A. L. de Souza, M. N. da Silva, A. R. de Almeida, G. Veira-Damiani, S. M. Alegre, C. L. César, L. A. Velloso, M. L. Cintra, N. B. Maia and P. E. N. F. Velho, Topical use and systemic action of green and roasted coffee oils and ground oils in a cutaneous incision model in rats (Rattus norvegicus albinus), PLoS One, 2017, 12(12), 1–17, DOI:10.1371/journal.pone.0188779.
- S. Wang, Y. C. Yoon, M.-J. Sung, H.-J. Hur and J.-H. Park, Antiangiogenic properties of cafestol, a coffee diterpene, in human umbilical vein endothelial cells, Biochem. Biophys. Res. Commun., 2012, 421(3), 567–571, DOI:10.1016/j.bbrc.2012.04.046.
- C. Cárdenas, A. R. Quesada and M. A. Medina, Anti-angiogenic and anti-inflammatory properties of kahweol, a coffee diterpene, PLoS One, 2011, 6(8), 1–9, DOI:10.1371/annotation/38262cc6-07cc-4074-8ce7-2181d4d0fbdc.
- E. J. Welsh, A. Bara, E. Barley and C. J. Cates, Caffeine for asthma, Cochrane Database Syst. Rev., 2010, 1, DOI:10.1002/14651858.CD001112.pub2.
- Humaryanto and O. R. Ave, Exploring the potential of green coffee extract for wound healing treatment, IOP Conference Series: Earth and Environmental Science, 2019, 39, https://iopscience.iop.org/article/10.1088/1755-1315/391/1/012057/meta.
- Y. Setyawan, H. S. Yuwono and R. Andean, Comparison on infected wound healing time, using coffee powder and honey in Wistar Rat, Int. J. Gen. Med. Pharm., 2017, 6(6), 1–6 Search PubMed . https://papers.ssrn.com/sol3/papers.cfm?abstract_id=3091621.
- M. A. Raeessi, J. Aslani, N. Raeessi, H. Gharaie, A. A. K. Zarchi and F. Raeessi, Honey plus coffee versus systemic steroid in the treatment of persistent post-infectious cough: a randomised controlled trial, Prim Care Respir J., 2013, 22, 325–330, DOI:10.4104/pcrj.2013.00072.
- M. A. Raeessi, N. Raeessi, Y. Panahi, H. Gharaie, S. M. Davoudi, A. Saadat, A. A. K. Zarchi, F. Raeessi, S. M. Ahmadi and H. Jalalian, “Coffee plus honey” versus “topical steroid” in the treatment of chemotherapy-induced oral mucositis: a randomised controlled trial, BMC Complement Altern. Med., 2014, 14, 293, DOI:10.1186/1472-6882-14-293.
- T. Herliani, F. A. Yusyahadi, H. S. Yuwono, I. M. Noor, K. Sakinah and T. Djajakusumah, The experimental wound healing using coffee powder and honey compared to NPWT, IJMPS, 2019, 8(6), 19–22 Search PubMed . http://paper.researchbib.com/view/paper/200410.
- R. Shahriari, P. Tamri, A. L. Harchegani and A. Nourian, Green coffee bean hydroalcoholic extract accelerates wound healing in full-thickness wounds in rabbits, Tradit. Med. Res., 2020, 5(6), 433–441, DOI:10.53388/TMR20200603191.
- H. S. Yuwono, A case of diabetic wound: the coffee powder protects the growth of cells on the wound bed, Am, J. Med. Case Rep., 2019, 7(5), 94–96 Search PubMed . http://article.americanmedicalcasereports.com/pdf/AJMCR-7-5-5.pdf.
- D. Arimbi and H. S. Yuwono, pH of wound fluids treated using coffee powder and bacitracin-neomycin powder, Glob. J. Surg., 2016, 4(1), 9–11 Search PubMed . http://pubs.sciepub.com/js/4/1/3/.
- B. S. Nagoba, N. M. Suryawanshi, B. Wadher and S. Selkar, Acidic environment and wound healing: A review, Wounds, 2015, 27(1), 5–11 Search PubMed . https://www.hmpgloballearningnetwork.com/site/wounds/article/acidic-environment-and-wound-healing-review.
- L. A. Schneider, A. Körber, S. Grabbe and J. Dissemond, Influence of pH on wound-healing: A new perspective for wound-therapy, Arch. Dermatol. Res., 2007, 298(9), 413–420, DOI:10.1007/s00403-006-0713-x.
- E. M. Jones, C. A. Cochrane and S. L. Percival, The effect of pH on the extracellular matrix and biofilms, Adv. Wound Caref., 2015, 4(7), 431–439, DOI:10.1089/wound.2014.0538.
-
G. Gethin, The significance of surface pH in chronic wounds, Wounds UK, 2007, 3(3), https://www.researchgate.net/publication/265530186_The_significance_of_surface_pH_in_chronic_wounds.
- S. L. Percival, S. McCarty, J. A. Hunt and E. J. Woods, The effects of pH on wound healing, biofilms, and antimicrobial efficacy, Wound Repair Regen., 2014, 22(2), 174–186, DOI:10.1111/wrr.12125.
- D. Bagdas, B. C. Etoz, Z. Gul, S. Ziyanok, S. Inan, O. Turacozen, N. Y. Gul, A. Topal, N. Cinkilic, S. Tas, M. O. Ozyigit and M. SibelGurun, In vivo systemic chlorogenic acid therapy under diabetic conditions: Wound healing effects and cytotoxicity/genotoxicity profile, Food Chem. Toxicol., 2015, 81, 54–61, DOI:10.1016/j.fct.2015.04.001.
-
D. León, S. Medina, J. Londoño-Londoño, C. Jiménez-Cartagena, F. Ferreres and A. Gil-Izquierdo, Anti-inflammatory activity of coffee, Coffee: Consumption and health implications, Royal Society of Chemistry, 2019 10.1039/9781788015028-00057.
- S. Pergolizzi, V. D’Angelo, M. Aragona, P. Dugo, F. Cacciola, G. Capillo, G. Dugo and E. Rita Lauriano, Evaluation of antioxidant and anti-inflammatory activity of green coffee beans methanolic extract in rat skin, Nat. Prod. Res., 2018, 34(11), 1535–1541, DOI:10.1080/14786419.2018.1523161.
- M. Daglia, M. T. Cuzzoni and C. Dacarro, Antibacterial activity of coffee, J. Agric. Food Chem., 1994, 42(10), 2270–2272, DOI:10.1021/jf00046a035.
- A. A. P. Almeida, C. C. Naghetini, V. R. Santos, A. G. Antonio, A. Farah and M. B. A. Glória, Influence of natural coffee compounds, coffee extracts and increased levels of caffeine on the inhibition of Streptococcus mutans, Food Res. Int., 2012, 49(1), 459–461, DOI:10.1016/j.foodres.2012.07.026.
- C. O. Esimone, F. B. Okoye, C. S. Nworu and C. O. Agubata, In vitro interaction between caffeine and some penicillin antibiotics against Staphylococcus aureus, Trop. J. Pharm. Res., 2008, 7(2), 969–974, DOI:10.4314/tjpr.v7i2.14675.
- M. Kępa, M. Miklasińska-Majdanik, R. D. Wojtyczka, D. Idzik, K. Korzeniowski, J. Smoleń-Dzirba and T. J. Wąsik, Antimicrobial potential of caffeic acid against Staphylococcus aureus clinical strains, BioMed Res. Int., 2018, 2018, 7413504, DOI:10.1155/2018/7413504.
- D. Stojković, J. Petrović, M. Soković, J. Glamočlija, J. Kukić-Marković and S. Petrović,
In situ antioxidant and antimicrobial activities of naturally occurring caffeic acid, p-coumaric acid and rutin, using food systems, J. Sci. Food Agric., 2012, 93(13), 3205–3208, DOI:10.1002/jsfa.6156.
- J. Fu, K. Cheng, Z.-M. Zhang, R.-Q. Fang and H.-L. Zhu, Synthesis, structure and structure–activity relationship analysis of caffeic acid amides as potential antimicrobials, Eur. J. Med. Chem., 2010, 45(6), 2638–2643, DOI:10.1016/j.ejmech.2010.01.066.
- S. A. Ibrahim, M. M. Salameh, S. Phetsomphou, H. Yang and C. W. Seo, Application of caffeine, 1,3,7-trimethylxanthine, to control Escherichia coli O157:H7, Food Chem., 2006, 99(4), 645–650, DOI:10.1016/j.foodchem.2005.08.026.
- O. O. Abosede, A. T. Gordon, T. O. Dembaremba, C. M. A. Lorentino, H. F. Frota, A. L. S. Santos, E. C. Hosten and A. S. Ogunlaja, Trimesic acid–theophylline and isopthalic acid–caffeine cocrystals: Synthesis, characterization, solubility, molecular docking, and antimicrobial activity, Cryst. Growth Des., 2020, 20(5), 3510–3522, DOI:10.1021/acs.cgd.0c00301.
- V. N. Lima, C. D. M. Oliveira-Tintino, E. S. Santos, L. P. Morais, S. R. Tintino, T. S. Freitas, Y. S. Geraldo, R. L. S. Pereira, R. P. Cruz, I. R. A. Menezes and H. D. M. Coutinho, Antimicrobial and enhancement of the antibiotic activity by phenolic compounds: Gallic acid, caffeic acid and pyrogallol, Microb. Pathog., 2016, 99, 56–61, DOI:10.1016/j.micpath.2016.08.004.
- A. A. P. Almeida, A. Farah, D. A. M. Silva, E. A. Nunan and M. B. A. Glória, Antibacterial activity of coffee extracts and selected coffee chemical compounds against Enterobacteria, J. Agric. Food Chem., 2006, 54(23), 8738–8743, DOI:10.1021/jf0617317.
- B. Özçelik, M. Kartal and I. Orhan, Cytotoxicity, antiviral and antimicrobial activities of alkaloids, flavonoids, and phenolic acids, Pharm Biol., 2011, 49(4), 396–402, DOI:10.3109/13880209.2010.519390.
- A. S. Rakatama, A. Pramono and R. Yulianti, The antifungal inhibitory concentration effectiveness test from ethanol seed arabica coffee (Coffea arabica) extract against the growth of Candida albicans patient isolate with in vitro method, J. Phys.: Conf. Ser., 2018, 970, 012023, DOI:10.1088/1742-6596/970/1/012023.
- I. Mathur, S. Shruthi, K. Gandrakota and K. J. Nisha, Comparative evaluation of antifungal activity of green coffee and green tea extract against Candida albicans: An In Vitro Study, World J. Dent., 2021, 12(4), 265–270, DOI:10.5005/jp-journals-10015-1839.
- Z. Nasrollahi and M. H. Yadegari, Antifungal activity of caffeine in combination with fluconazole against Candida albicans, Infect. Epidemiol. Med., 2016, 2(2), 18–21 CrossRef . https://www.researchgate.net/publication/317698548_Antifungal_Activity_of_Caffeine_in_Combination_with_Fluconazole_against_Candida_albicans.
- J. S. Raut, N. M. Chauhan, R. B. Shinde and S. M. Karuppayil, Inhibition of planktonic and biofilm growth of Candida albicans reveals novel antifungal activity of caffeine, J. Med. Plant. Res., 2013, 7(13), 777–782 Search PubMed . https://academicjournals.org/journal/JMPR/article-full-text-pdf/5D3CFDC22235.
- W. S. Sung and D. G. Lee, Antifungal action of chlorogenic acid against pathogenic fungi, mediated by membrane disruption, Pure Appl. Chem., 2010, 82(1), 219–222, DOI:10.1351/PAC-CON-09-01-08.
- C.-M. Ma, M. Kully and J. K. Khan, Synthesis of chlorogenic acid derivatives with promising antifungal activity, Bioorg. Med. Chem., 2007, 15(21), 6830–6833, DOI:10.1016/j.bmc.2007.07.038.
- C. R. d. Silva, L. G. d. A. V. Sá, E. V. d. Santos, T. L. Ferreira, T. d. N. P. Coutinho, L. E. A. Moreira, R. d. S. Campos, C. R. d. Andrade, W. M. B. d. Silva, I. d. S. Carneiro, J. Silva, H. S. d. Santos, E. S. Marinho, B. C. Cavalcanti, M. O. d. Moraes, H. V. N. Júnior and J. B. A. Neto, Evaluation of the antifungal effect of chlorogenic acid against strains of Candida spp. resistant to fluconazole: Apoptosis induction and in silico analysis of the possible mechanisms of action, J. Med. Microbiol., 2022, 71(5) DOI:10.1099/jmm.0.001526.
-
C. S. e Silva, C. Gabriel, F. Cerqueira, M. C. Manso and A. F. Vinha, Technological Advances and Educational Programs, The Battle Against Microbial Pathogens: Basic Science, Badajoz, Formatex Research Center, 2015. https://bdigital.ufp.pt/handle/10284/8043.
- P. Parsaei, M. Karimi, S. Y. Asadi and M. Rafieian-kopaei, Bioactive components and preventive effect of green tea (Camellia sinensis) extract on post-laparotomy intra-abdominal adhesion in rats, Int. J. Surg., 2013, 11(9), 811–815, DOI:10.1016/j.ijsu.2013.08.014.
- A. Forouzanfar, H. R. Arab, H. Shafaee, M. R. Mokhtari and S. Golestani, The effect of green tea mouthwash (Camellia sinensis) on wound healing following periodontal crown lengthening surgery; a double blind randomized controlled trial, Sci. Res. J., 2012, 2(4), 369–372, DOI:10.4236/ojst.2012.24064.
- A. Zink and C. Traidl-Hoffmann, Green tea in dermatology – myths and facts, J. Dtsch. Dermatol. Ges., 2015, 13(8), 768–775, DOI:10.1111/ddg.12737.
- S. Y. Asadi, P. Parsaei, M. Karimi, S. Ezzati, A. Zamiri, F. Mohammadizadeh and M. Rafieian-kopaei, Effect of green tea (Camellia sinensis) extract on healing process of surgical wounds in rat, Int. J. Surg., 2013, 11(4), 332–337, DOI:10.1016/j.ijsu.2013.02.014.
- Y. Qin, H.-w Wang, T. Karuppanapandian and W. Kim, Chitosan green tea polyphenol complex as a released control compound for wound healing, Chin. J. Traumatol., 2010, 13(2), 91–95 CAS . https://www.sciencedirect.com/science/article/pii/S1008127510600181.
- Y. Qin, X. W. Guo, L. Li, H. W. Wang and W. Kim, The antioxidant property of chitosan green tea polyphenols complex induces transglutaminase activation in wound healing, J. Med. Food, 2013, 16(6), 487–498, DOI:10.1089/jmf.2012.2623.
- S. Er and M. Dikmen,
Camellia sinensis increased apoptosis on U2OS osteosarcoma cells and wound healing potential on NIH3T3 fibroblast cells, Cytotechnology, 2017, 69, 901–914, DOI:10.1007/s10616-017-0105-4.
- A. Hagiu, T. Attin, P. R. Schmidlin and L. L. Ramenzoni, Dose-dependent green tea effect on decrease of inflammation in human oral gingival epithelial keratinocytes: In vitro study, Clin. Oral. Investig., 2020, 24, 2375–2383, DOI:10.1007/s00784-019-03096-4.
- H. A. Al-Rawaf, S. A. Gabr and A. H. Alghadir, Circulating hypoxia responsive microRNAs (HRMs) and wound healing potentials of green tea in diabetic and nondiabetic rat models, J. Evidence-Based Complementary Altern. Med., 2019, 2019, 9019253, DOI:10.1155/2019/9019253.
- A. L. de, A. Neves, M. C. Komesu and M. A. S. D. Matteo, Int J. Morphol., 2010, 28(3), 905–910, DOI:10.4067/S0717-95022010000300039.
- N. Khan and H. Mukhtar, Tea polyphenols in promotion of human health, Nutrients, 2019, 11(1), 39, DOI:10.3390/nu11010039.
- H. H. Kim, T. Kawazoe, D.-W. Han, K. Matsumara, S. Suzuki, S. Tsutsumi and S.-H. Hyon, Enhanced wound healing by an epigallocatechin gallate-incorporated collagen sponge in diabetic mice, J. Tissue. Eng. Regen. Med., 2008, 16(5), 714–720, DOI:10.1111/j.1524-475X.2008.00422.x.
- B. R. Klass, O. A. Branford, A. O. Grobbelaar and K. J. Rolfe, The effect of epigallocatechin-3-gallate, a constituent of green tea, on transforming growth factor-β1–stimulated wound contraction, J. Tissue Eng. Regen. Med., 2010, 18(1), 80–88, DOI:10.1111/j.1524-475X.2009.00552.x.
- H.-L. Kim, J.-H. Lee, B. J. Kwon, M. H. Lee, D.-W. Han, S.-H. Hyon and J.-C. Park, Promotion of Full-thickness wound healing using epigallocatechin-3-o-gallate/poly (lactic-co-glycolic acid) membrane as temporary wound dressing, Artif. Organs, 2013, 38(5), 411–417, DOI:10.1111/aor.12190.
- S. Hsu, W. B. Bollag, J. Lewis, Q. Huang, B. Singh, M. Sharawy, T. Yamamoto and G. Schuster, Green tea polyphenols induce differentiation and proliferation in epidermal keratinocytes, J. Pharmacol. Exp. Ther., 2003, 306(1), 29–34, DOI:10.1124/jpet.103.049734.
- A. Hoffman, R. Baxter, A. Nasar, T. R. Gardner, S. Kumara, C. Cordon-Cardo, A. Ahmed, R. A. Newman, O. Zmora and R. L. Whelan, Perioperative polyphenon E, a green tea extract, does not affect the wound complication rate in mice after sham laparotomy yet has an inhibitory effect on wound healing, Surg. Innov., 2012, 19(4) DOI:10.1177/1553350612436565.
- H. Shahrahmani, N. Kariman, S. Jannesari, M. Rafieian-Kopaei, M. Mirzaei, S. Ghalandari, N. Shahrahmani and G. Mardani, The effect of green tea ointment on episiotomy pain and wound healing in primiparous women: A randomized, double-blind, placebo-controlled clinical trial, Phytother. Res., 2017, 32(3), 522–530, DOI:10.1002/ptr.5999.
- M. Sadri, S. Arab-Sorkhi, H. Vatani and A. Bagheri-Pebdeni, New wound dressing polymeric nanofiber containing green tea extract prepared by electrospinning method, Fibers Polym., 2015, 16, 1742–1750, DOI:10.1007/s12221-015-5297-7.
- J.-G. Leu, S.-A. Chen, H.-M. Chen, W.-M. Wu, C.-F. Hung, Y.-D. Yao, C.-S. Tu and Y.-J. Liang, The effects of gold nanoparticles in wound healing with antioxidant epigallocatechin gallate and α-lipoic acid, Nanomedicine, 2012, 8(5), 767–775, DOI:10.1016/j.nano.2011.08.013.
- G. Chen, L. He, P. Zhang, J. Zhang, X. Mei, D. Wang, Y. Zhang and Z. Chen, Encapsulation of green tea polyphenol nanospheres in PVA/alginate hydrogel for promoting wound healing of diabetic rats by regulating PI3K/AKT pathway, Mater. Sci. Eng. C, 2020, 110, 110686, DOI:10.1016/j.msec.2020.110686.
- S. Y. Park, H. U. Lee, Y.-C. Lee, G. H. Kim, E. C. Park, S. H. Han, J. G. Lee, S. Choi, N. S. Heo, D. L. Kim, Y. S. Huh and J. Lee, Wound healing potential of antibacterial microneedles loaded with green tea extracts, Mater. Sci. Eng. C, 2014, 42, 757–762, DOI:10.1016/j.msec.2014.06.021.
- S. Y. Asadi, A. Zamiri, S. Ezzati, P. Parsaei, M. Rafieian-Kopaei and H. Shirzad, Effect of alcoholic extract of green tea (Camellia sinensis) on the healing process in surgical and burn wounds in rats, J. Birjand Univ. Med. Sci., 2011, 18(1), 1–9 Search PubMed . http://eprints.skums.ac.ir/6721/.
- F. Hajiaghaalipour, M. S. Kanthimathi, M. A. Abdulla and J. Sanusi, The effect of Camellia sinensis on wound healing potential in an animal model, J. Evidence-Based Complementary Altern. Med., 2013, 2013, 386734, DOI:10.1155/2013/386734.
- M. Karimi, P. Parsaei, S. Y. Asadi, S. Ezzati, R. K. Boroujeni, A. Zamiri and M. Rafieian-Kopaei, Effects of Camellia sinensis ethanolic extract on histometric and histopathological healing process of burn wound in rat, Middle East, J. Sci. Res., 2013, 13(1), 14–19 Search PubMed . http://eprints.skums.ac.ir/2700/.
- M. J. Fatemi, B. Nikoomaram, A. A. K. Rahimi, D. Talayi, S. Taghavi and Y. Ghavami, Effect of green tea on the second degree burn wounds in rats, In. J. Plast. Surg., 2014, 47(3), 370–374, DOI:10.4103/0970-0358.146593.
- B. Frei and J. V. Higdon, Antioxidant activity of tea polyphenols in vivo: Evidence from animal studies, J. Nutr., 2003, 133(10), 3275S–3284S, DOI:10.1093/jn/133.10.3275S.
- M. Serafini, A. Ghiselli and S. Ferro-Luzzi, In vivo antioxidant effect of green and black tea in man, Eur. J. Clin. Nutr., 1996, 50(1), 28–32 CAS . https://europepmc.org/article/med/8617188?utm_medium=74593.
- S. P. J. N. Senanayake, Green tea extract: Chemistry, antioxidant properties and food applications – A review, J. Funct. Foods, 2013, 5(4), 1529–1541, DOI:10.1016/j.jff.2013.08.011.
- R. J. Ruch, S.-j Cheng and J. E. Klaunig, Prevention of cytotoxicity and inhibition of intercellular communication by antioxidant catechins isolated from Chinese green tea, Carcinogenesis, 1989, 10(6), 1003–1008, DOI:10.1093/carcin/10.6.1003.
- Y.-M. Choi, M.-H. Kim, J.-J. Shin, J.-M. Park and J.-S. Lee, The antioxidant activities of the some commercial teas, J. Korean Soc. Food Sci. Nutr., 2003, 32(5), 723–727 CrossRef CAS . https://www.koreascience.or.kr/article/JAKO200311921773097.page.
- K. W. Lee, H. J. Lee and C. Y. Lee, Antioxidant activity of black tea vs. green tea, J. Nutr., 2002, 132(4), 785, DOI:10.1093/jn/132.4.785.
- S. M. Henning, Y. Niu, N. H. Lee, G. D. Thames, R. R. Minutti, H. Wang, V. L. W. Go and D. Heber, Bioavailability and antioxidant activity of tea flavanols after consumption of green tea, black tea, or a green tea extract supplement, Am. J. Clin. Nutr., 2004, 80(6), 1558–1564, DOI:10.1093/ajcn/80.6.1558.
- H. Sung, J. Nah, S. Chun, H. Park, S. E. Yang and W. K. Min, In vivo antioxidant effect of green tea, Eur. J. Clin. Nutr., 2000, 54, 527–529, DOI:10.1038/sj.ejcn.1600994.
- X. Hui, Q. Yue, D.-D. Zhang, H. Li, S.-Q. Yang and W.-Y. Gao, Antimicrobial mechanism of theaflavins: They target 1-deoxy-D-xylulose 5-phosphate reductoisomerase, the key enzyme of the MEP terpenoid biosynthetic pathway, Sci. Rep., 2016, 6, 38945, DOI:10.1038/srep38945.
- H. Yin, Y. Deng, H. Wang, W. Liu, X. Zhuang and W. Chu, Tea polyphenols as an antivirulence compound disrupt quorum-sensing regulated pathogenicity of Pseudomonas aeruginosa, Sci. Rep., 2015, 5, 16158, DOI:10.1038/srep16158.
- O. F. Olosunde, K. Abu-Saeed and M. B. Abu-Saeed, Phytochemical screening and antimicrobial properties of a common brand of black tea (Camellia sinensis) marketed in nigerian environment, Adv. Pharm. Bull., 2012, 2(2), 259–263, DOI:10.5681/apb.2012.040.
- N. C. Gordon and D. W. Wareham, Antimicrobial activity of the green tea polyphenol (−)-epigallocatechin-3-gallate (EGCG) against clinical isolates of Stenotrophomonas maltophilia, Int. J. Antimicrob. Agents, 2010, 36(2), 129–131, DOI:10.1016/j.ijantimicag.2010.03.025.
- M. Radji, R. A. Agustama, B. Elya and C. R. Tjampakasari, Antimicrobial activity of green tea extract against isolates of methicillin–resistant Staphylococcus aureus and multi–drug resistant Pseudomonas aeruginosa, Asian Pac. J. Trop. Biomed., 2013, 3(8), 663–667, DOI:10.1016/S2221-1691(13)60133-1.
- A. Farjana, N. Zerin and M. S. Kabir, Antimicrobial activity of medicinal plant leaf extracts against pathogenic bacteria, Asian Pacific J. Trop. Dis., 2014, 4(2), S920–S923, DOI:10.1016/S2222-1808(14)60758-1.
- W. R. Rolim, M. T. Pelegrino, B. A. Lima, L. S. Ferraz, F. N. Costa, J. S. Bernardes, T. Rodigues, M. Brocchi and A. B. Seabra, Green tea extract mediated biogenic synthesis of silver nanoparticles: Characterization, cytotoxicity evaluation and antibacterial activity, Appl. Surf. Sci., 2019, 463, 66–74, DOI:10.1016/j.apsusc.2018.08.203.
- K. Y. Ho, C. C. Tsai, J. S. Huang, C. P. Chen, T. C. Lin and C. C. Lin, Antimicrobial activity of tannin components from Vaccinium vitis-idaea L, J. Pharm. Pharmacol., 2010, 53(2), 187–191, DOI:10.1211/0022357011775389.
- S. Muthuswamy and H. P. V. Rupasinghe, Fruit phenolics as natural antimicrobial agents: Selective antimicrobial activity of catechin, chlorogenic acid and phloridzin, J. Food, Agric. Environ., 2007, 5(3-4), 81–85 CAS . https://pubag.nal.usda.gov/catalog/723473.
- P. W. Taylor, J. M. T. Hamilton-Miller and P. D. Stapleton, Antimicrobial properties of green tea catechins, Food. Sci. Technol. Bull., 2005, 2, 71–81 Search PubMed . https://www.ncbi.nlm.nih.gov/pmc/articles/PMC2763290/.
- S. Kasai, S. Watanabe, J. Kawabata, S. Tahara and J. Mizutani, Antimicrobial catechin derivatives of Agrimonia pilosa, Phytochemistry, 1992, 31(3), 787–789, DOI:10.1016/0031-9422(92)80015-7.
- S. Rawdkuen, P. Suthiluk, D. Kamhangwong and S. Benjakul, Mechanical, physico-chemical, and antimicrobial properties of gelatin-based film incorporated with catechin-lysozyme, Chem. Cent. J., 2012, 6, 131, DOI:10.1186/1752-153X-6-131.
- L. Bai, S. Takagi, T. Ando, H. Yoneyama, K. Ito, H. Mizugai and E. Isogai, Antimicrobial activity of tea catechin against canine oral bacteria and the functional mechanisms, J. Vet. Med. Sci., 2016, 78(9), 1439–1445, DOI:10.1292/jvms.16-0198.
- M. Friedman, P. R. Henika, C. E. Levin, R. E. Mandrell and N. Kozukue, Antimicrobial activities of tea catechins and theaflavins and tea extracts against Bacillus cereus, J. Food Prot., 2006, 69(2), 354–361, DOI:10.4315/0362-028X-69.2.354.
- T. Taguri, T. Tanaka and I. Kouno, Antimicrobial activity of 10 different plant polyphenols against bacteria causing food-borne disease, Biol. Pharm. Bull., 2004, 27(2), 1965–1969, DOI:10.1248/bpb.27.1965.
- Ö. G. Üstündağ, S. Erşan, E. Özcan, G. Özan, N. Kayra and F. Y. Ekinci, Black tea processing waste as a source of antioxidant and antimicrobial phenolic compounds, Eur. Food Res. Technol., 2016, 242, 1523–1532, DOI:10.1007/s00217-016-2653-9.
- J. W. Betts and D. W. Wareham, In vitro activity of curcumin in combination with epigallocatechin gallate (EGCG) versus multidrug-resistant Acinetobacter baumannii, BMC Microbiol., 2014, 14, 172, DOI:10.1186/1471-2180-14-172.
- Y. Hu, J. Jia, J. Qiao, C. Ge and Z. Cao, Antimicrobial activity of pu-erh tea extracts in vitro and its effects on the preservation of cooled mutton, J. Food Saf., 2010, 30(1), 177–195, DOI:10.1111/j.1745-4565.2009.00199.x.
- S. K. Mbuthia, F. N. Wachira and R. K. Koech, In-vitro antimicrobial and synergistic properties of water soluble green and black tea extracts, Afr. J. Microbiol. Res., 2014, 8(14), 1527–1534, DOI:10.5897/AJMR2014.6655.
- L. Zili and L. Zhiqun, Recent advances in polysaccharide-based hydrogels for synthesis and applications, Aggregate, 2021, 2(2), 1–26, DOI:10.1002/agt2.21.
|
This journal is © The Royal Society of Chemistry 2022 |
Click here to see how this site uses Cookies. View our privacy policy here.