Environmental significance of trace elements in the Athabasca Bituminous Sands: facts and misconceptions†
Received
7th February 2022
, Accepted 20th June 2022
First published on 6th July 2022
Abstract
The bituminous sands of Alberta, Canada, represent the second largest reserves of hydrocarbons on earth. Open pit bitumen mining and upgrading of the Athabasca Bituminous Sands (ABS), the largest of the deposits, began in 1967. Concerns about fugitive release of trace elements (TEs) to the environment began with studies of V, as this is the most abundant trace metal in bitumen. Recent studies, however, have extended to Ag, As, Be, Cd, Cr, Cu, Pb, Sb, and Tl, and this has led to considerable confusion about which TEs are relevant to ecosystem health. While V along with Ni, Mo, Se and Re are enriched in bitumen, Ag, As, Be, Cd, Cr, Cu, Pb, Sb and Tl are found almost exclusively in the mineral (i.e. sand) fraction of the ABS, with limited opportunity to become bioaccessible, much less bioavailable. Here, a summary is given of ten misunderstandings that have arisen regarding TEs in the environment of the ABS region. To help illustrate the significance of the misconceptions that have arisen regarding (a) air and (b) water resources, published and unpublished TE data obtained from the metal-free, ultraclean SWAMP lab is presented for: (a) snow, moss, and peat cores from bogs, and (b) the dissolved, particulate, and colloidal fractions of water from the Athabasca River (AR), as well as pristine groundwater. Natural enrichments of Ni in plants such as Rat Root (Acorus calamus) and pine (Pinus banksiana), Tl in fish (Percopsis omiscomaycus) and Cd in cranberries (Vaccinium oxycoccus), Labrador Tea (Rhododendron groenlandicum) and beaver (Castor canadensis), are also presented.
| William Shotyk William Shotyk received his B.Sc. (Agr.) in Soil Science and Chemistry from the University of Guelph and a Ph.D. in Geology from the University of Western Ontario. Following postdoctoral research at the University of California, Riverside and UWO, he worked at the University of Berne in Switzerland where he completed a Habilitation in Geochemistry, in 1995. He became Professor at the University of Heidelberg in Germany, and Director of the Institute of Environmental Geochemistry, in October of 2000. He joined the Department of Renewable Resources at the University of Alberta in October of 2011, as the Bocock Chair for Agriculture and the Environment. He has 265 publications to his credit, including more than 200 journal articles. He was awarded the Philippe Duchaufour Medal for Soil System Science by the European Geoscience Union (2013), elected a Fellow of the Royal Society of Canada (2018), and a Fellow of the Geochemical Society and the European Association of Geochemistry (2019). |
Environmental significance
In the Athabasca Bituminous Sands, Ag, As, Be, Cr, Cd, Pb, Sb and Tl are all depleted, relative to the Upper Continental Crust, and found almost exclusively in the mineral fraction. This helps to explain why atmospheric deposition of trace elements (TEs) in the region has been in decline for decades, why dust particles in the area have such low concentrations of TEs, and why TE concentrations in the dissolved fraction of the Athabasca River (AR) are at natural, background levels.
|
Introduction
The bituminous sands of Alberta cover an area of approximately 140
000 km2 and are estimated to contain hydrocarbon reserves equivalent to 27 billion m3: these oil reserves1 are second only to those of Saudi Arabia. The Athabasca Bituminous Sands (ABS) are by far the largest of the four main deposits, with commercial exploitation having begun in 1967 via open pit mining and upgrading. Since that time, there have been many environmental studies of trace elements (TEs) in the vicinity of these open-pit bitumen mines and upgraders. These include studies of wet2 and dry deposition,3 snow,4–6 surface waters,7–9 fish,10,11 mussels,12 soil,13 sediments,14–16 lichens,17,18 higher plants,19,20 and wildlife.21–23 Implicit in all of these studies is the assumption that mining and upgrading of bitumen is a significant source of TEs to the environment. From a geochemical perspective, there is no obvious reason why this would be true for elements other than V, Ni and Mo which are well known for their enrichments in bitumen.24,25 More recently, it became known that Re is also enriched in bitumen,26 as well as Se.27 In the absence of an understanding of the geochemistry of bitumen, it seems that many, if not most environmental studies simply assume that all of the potentially toxic TEs of environmental concern, e.g. Ag, As, Be, Cd, Cr, Cu, Pb, Sb, and Tl, occur in the ABS at elevated concentrations. Mining and upgrading is further assumed to release these elements to the environment at rates and in forms that present threats to the health of aquatic and terrestrial ecosystems. So, from a lack of familiarity with the geochemistry of bitumen deposits, other concepts have arisen which cloud our knowledge of the sources, transport, behaviour and fate of TEs in northern Alberta. Taken together, these hinder our progress in understanding the true environmental impacts of one of the largest hydrocarbon extractive industries in the world.
In an attempt to help bring clarity regarding the ecological significance of TEs in this region, ten of the most common misunderstandings are presented and discussed. To help illustrate the extent of the problems that have arisen, published and unpublished results are presented from research undertaken at the metal-free, ultraclean SWAMP laboratory. The SWAMP laboratory was conceived and created at the University of Alberta to study TEs in soil, water, air, manure and plants, but designed and constructed using the strategies developed for trace element analysis,28 at the levels occurring in polar ice29 and pristine natural waters.30 One of the main objectives of the laboratory is to clearly distinguish between natural and anthropogenic sources of TEs to the environment. Related to this is the second goal of the laboratory which is to distinguish between bioaccessible and bioavailable forms of TEs. While the open pit bitumen mines generate tremendous volumes of dust, the dusts themselves are depleted in TEs, relative to their corresponding values in crustal rocks. Further, we have found that most TEs in the dissolved fraction (i.e. capable of passing through a 0.45 μm membrane filter) of surface waters, occur at natural, “background” concentrations. Thus, it is hoped that the results presented here will be of broad interest to scientists studying the environmental geochemistry of TEs elsewhere in the Boreal Zone of the northern hemisphere.
To help orient the reader, many of the sampling locations mentioned in the text are shown in Fig. 1. For the benefit of students new to the topic, some background information on the geochemical classification of the elements is provided in Appendix A. For further reading, a bibliography of chemistry and geochemistry texts is included in Appendix B. Both Appendices can be found in the ESI.†
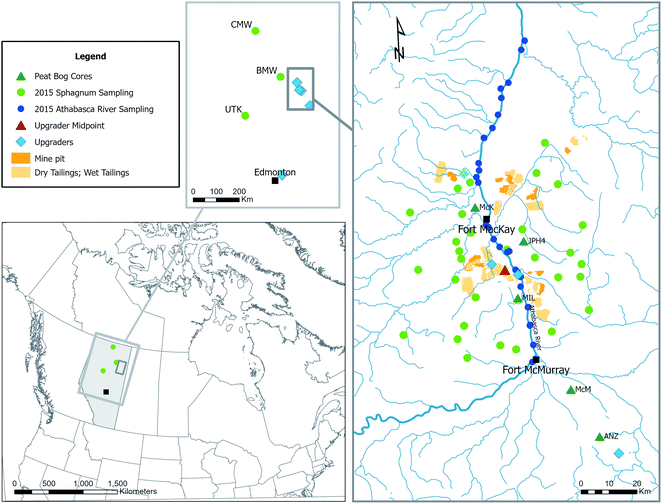 |
| Fig. 1 Locations of some of the sampling sites mentioned in the text: peat cores from bogs (green triangles), Sphagnum moss collected in 2015 (green circles), and AR surface waters collected in 2015 (blue circles). The small, inset map shows the approximate locations of moss and peat samples collected at control locations: Birch Mountain Wildlands (BMW), Caribou Mountain Wildlands (CMW), and Utikuma (UTK) which are approximately 103, 320 and 264 km, respectively, from the mid-point between the two central bitumen upgraders. The four bitumen upgraders in the area are indicated by blue diamonds, and the mid-point between the two central upgraders by red triangle. | |
Misconception 1. The ABS contain elevated concentrations of potentially toxic TEs including Ag, As, Be, Cd, Cr, Cu, Pb, Sb, Tl, and Zn
Until recently, there was very little quantitative data for TEs in the ABS.31 Early work using emission spectrographs was only semi-quantitative.32 Some data was obtained using instrumental neutron activation analysis (INAA) which is an established method providing excellent data for lithophile elements such as Al, Cr, Sc, Th, and V, as well some chalcophile TEs of environmental concern including As and Sb.33 However, while this method provides excellent sensitivity (e.g. μg kg−1) for the elements listed above,34,35 it cannot be used to determine some of the chalcophile elements of greatest concern such as Cd, Pb or Tl. X-ray fluorescence spectroscopy (XRF) was also used in early work on bitumen,36 but yielded very little data for TEs of environmental relevance because of the relatively high (mg kg−1) limits of detection.37 Both INAA and XRF share the great advantage of providing data for solid materials, thereby eliminating the need for sample digestion. So, for elements such as Ti and Zr which are abundant in the ABS and occur in stable mineral phases which are difficult to dissolve, XRF is the perfect analytical tool for their determination. Hydride-generation atomic fluorescence spectroscopy (HG-AFS) is a very sensitive method for the determination of hydride forming elements including As, Sb, and Se,38 but, until recently,27,39 has found little application to bitumen. Despite the introduction in recent decades of far more sensitive methods such as inductively-coupled plasma mass spectrometry (ICP-MS), there have been remarkably few applications of this technique to the study of TEs in bitumen. Recently, however, sector-field ICP-MS was used to determine the abundance of almost all of the TEs of environmental relevance, in both bulk samples (i.e. ores) and the bitumen itself.39
Comparing the abundance of TEs in bulk samples (i.e. ores) of ABS with the corresponding abundance of TEs in the Upper Continental Crust (UCC) shows that only two elements are more abundant in the ABS, compared to the crust: Mo and Re (Fig. 2). When the concentrations of TEs in ABS are normalized to the corresponding abundance of TEs in the UCC, it becomes clear that only two elements are actually enriched in the ABS, compared to the crust: Mo and Re (Fig. 3). Neither Mo nor Re is of general environmental concern. Molybdenum, for example, is a micronutrient, essential for the health of all plants and animals.41 Rhenium, one of the platinum group elements (PGE), is one of the most rare elements in the Earth's crust, being less abundant that either Pt or Au.40 In summary, the concentrations of the potentially toxic, so-called “heavy metals”‡ of environmental concern, namely Ag, As, Be, Cd, Cr, Cu, Pb, Sb, Tl and Zn, are all less abundant in the bulk ABS than typical rocks at the surface of the earth.
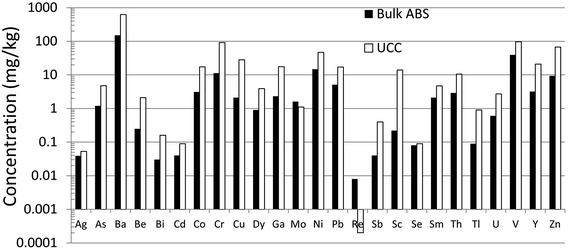 |
| Fig. 2 Abundance of TEs in bulk ABS and UCC. Concentration data for ABS from Bicalho et al.39 except for Se.27 Data for the UCC from Rudnick and Gao.40 | |
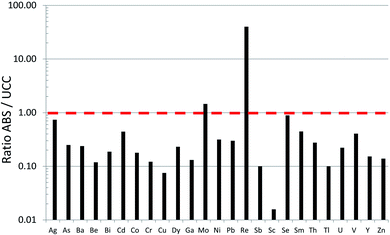 |
| Fig. 3 Ratios of TE concentrations in bulk ABS39 to the UCC.40 The horizontal dashed line (ABS/UCC = 1) represents values that are neither enriched nor depleted, in the ABS relative to the UCC. Notice that Mo and Re are the only elements enriched in the ABS, relative to the UCC. | |
Misconception 2. Potentially toxic TEs in the ABS are enriched in the bitumen fraction of the ABS
Bitumen was carefully extracted from the ABS using metal-free protocols (e.g. employing high purity quartz instead of glassware when using organic solvents), digested, and analyzed for the full suite of TEs using sector-field ICP-MS.39 However, only five elements are found predominantly in the bitumen fraction. Listed in order of decreasing abundance, they are V, Ni, Mo, Se and Re (Table 1). The extent of enrichment of these elements in bitumen, relative to crustal abundance, increases in the order Ni (1.8×), V (2.4×), Se (4.4×), Mo (9.1×) and Re (250×). So, even though V and Ni are the most abundant trace metals in bitumen, their enrichment, relative to crustal abundance, is modest i.e. within a factor of two of their abundance in the UCC. Rhenium, one of the most rare elements on earth, is by far the most highly enriched TE in bitumen. Of the five TEs enriched in bitumen, one is a lithophile (V), two are siderophiles (Ni and Re), one is chalcophile (Se) and one (Mo) has recently been considered “variably chalcophile”.52 Thus, unlike the enrichments of TEs in coal which are almost exclusively restricted to the chalcophile elements,53,54 there is no simple relationship between the enrichment of these TEs in bitumen, and their geochemical classification.
Table 1 Abundance of V, Ni, Mo, Se and Re in bitumen, listed in order abundance. Data for the UCC from Rudnick and Gao (2014)40
Element |
Bitumen (mg kg−1) |
UCC (mg kg−1) |
Ratio bitumen/UCC |
From Bicalho et al. (2017).39
From Donner et al. (2018).27
|
V |
237a |
97 |
2.4 |
Ni |
83a |
47 |
1.8 |
Mo |
10a |
1.1 |
9.1 |
Se |
0.4b |
0.09 |
4.4 |
Re |
0.05a |
0.0002 |
250 |
The mechanism of enrichment of V and Ni in petroleum has been studied for decades.55 The enrichment of V in bitumen was first noted by Kyle in 1891,56 the role of marine organisms in its accumulation was discovered by Vernadsky in 1924,57 and Treibs in 1935,58 recognized that this metal was present in the form of porphyrins. Champlin and Dunning59 identified both Ni- and V-containing porphyrin compounds in samples of ABS from Abasand and Bitumount in northern Alberta. With an average of 180 ppm V and 80 ppm Ni, approximately 10% of the V and Ni in the bitumen was found to be in porphyrins. Subsequent studies of V and Ni in crude oils have suggested that the remainder of the V and Ni may also be in the form of porphyrins which cannot be readily extracted, or in the form of metal naphthenates.60 Given that V and Ni are by far the most abundant trace metals found in bitumen, and that they are in predominantly organic forms, these are the two elements that could reasonably be expected to be enriched in environmental media from fugitive atmospheric and aquatic releases by industry.
Regarding the other TEs of environmental interest, namely Ag, As, Be, Cd, Cr. Cu, Pb, Sb, Tl and Zn, these are found predominantly and almost exclusively in the mineral fraction of the ABS.39 On average, the ABS are approximately 85% mineral matter, predominantly dune and beach aeolian sands,58 with most particles >100 μm.61 The mineral composition is dominated by light minerals (99%), mainly quartz (60–90%) and clays (kaolinite and illite), with minor amounts of feldspars (including orthoclase), mica (biotite and muscovite), carbonates, and chalcedony.62 Quartz is not a host for TEs63 and its predominance in the mineral fraction of the ABS explains the depletions of nearly all the TEs, relative to the composition of the UCC (Fig. 3). Heavy minerals (density > 2.96 g cm−3) account for 1% of the mineral fraction, and includes Ti, Y, and Zr-bearing minerals such as ilmenite, tourmaline and zircon.62 As noted in the detailed work by Bichard (1987),62 the bulk of the minerals are relatively inert materials similar to clastic sediments that have undergone intensive weathering. The principal mineral hosts of TEs in the mineral fraction of ABS are likely the clays, feldspars, and micas. For example, Pb occurs in the lattice structure of silicates: Pb2+ is known to substitute for Ca2+ in plagioclase feldspar, and for K+ in orthoclase feldspar and mica.64 Similarly, Tl occurs in orthoclase feldspars and mica, where Tl+ can easily replace K+,64 given that they have the same ionic radius and charge.51
In contrast to the “sand” fraction, within the bitumen fraction of the ABS, concentrations of the TEs of concern are generally very low. Zinc is most abundant, at 5 mg kg−1, followed by Cu at 1 mg kg−1. Lead and As in bitumen are <1 mg kg−1, and Ag, Bi, Cd, Sb and Tl are all below 0.05 mg kg−1.39 The abundance of Tl in bitumen, for example, is only 0.005 mg kg−1. Thus, most of the TEs of environmental concern are hosted by relatively stable, predominantly silicate minerals which make up the bulk of the ABS ore. The presence of the low and variable concentrations of these elements in the bitumen itself may simply reflect the abundance of fine grained mineral particles which remain, even after extraction and filtration through a 0.45 μm membrane filter.39 Thus, even within the bitumen fraction, many of the elements of environmental relevance may be hosted by silicate minerals with sub-micron dimensions.
Misconception 3. TEs are released to the atmospheric environment from bitumen mining and upgrading by combustion, generating sub-micron aerosols which are respirable and capable of long-range atmospheric transport
The combustion of fossil fuels is widely recognized as a globally significant source of TEs to the atmosphere.65 Again, however, the suite of TEs which are naturally enriched in coal and those which are enriched in petroleum, are very different. Coal is commonly enriched in virtually all of the chalcophile elements53,54 whereas bitumen is enriched in V, Ni, Mo, Re and Se (Table 1). According to Pacyna and Pacyna (2001),66 the emission factors (g of metal emitted per tonne of fuel burned) of Ni to the global atmosphere from oil combustion are 10× greater than the corresponding emissions from coal combustion; emissions of V from oil combustion are 60× those of coal combustion. And again, these emissions are based upon fuel combustion. During the combustion of fossil fuels, very high temperatures are achieved (>1000 °C) which create sub-micron aerosols67,68 enriched in TEs.69–73 With atmospheric residence times on the order of one week, these aerosols are subject to long-range atmospheric transport because they remain in the air until they are removed by dry deposition or precipitation scavenging.65
In contrast, upgrading of bitumen in Alberta takes place at temperatures of below 500 °C (ref. 1) i.e. bitumen is heated, but not combusted. During upgrading, V and Ni are both residually enriched in the coke which is generated as a by-product, with typical concentrations being 1680 and 500 mg kg−1, respectively.1 The International Agency for Research on Cancer (IARC) report on vanadium and its compounds is clear on this point: “during refining and distillation, the vanadium remains in the residual oil because of its low volatility, and as a result becomes more concentrated than the original crude”.74 This point has also been made in the report on vanadium by Environment Canada (2010, p. 11).75 An analysis of coke samples from two firms operating in the ABS region shows that V, Ni and Mo are by far the most enriched of all the TEs measured to date, relative to the UCC (Fig. 4). In contrast to bitumen upgrading, the combustion of petroleum is altogether different, with most of the metal in residual oils released to the atmosphere. Not only will oil combustion contribute substantially to atmospheric emissions of these metals, both V74 and Ni76 are emitted in the form of oxides which are carcinogenic.74
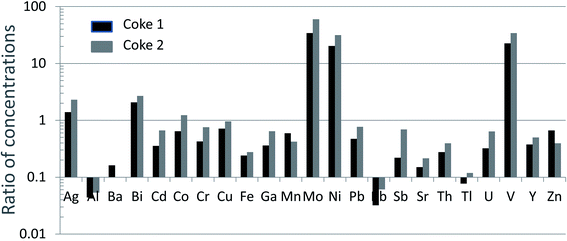 |
| Fig. 4 Ratios of TE concentrations in two coke samples (unpublished data) to the UCC.40 | |
In respect of energy required for upgrading the ABS, most is derived from the combustion of natural gas, process gas, or electricity from the existing grid,77 but some is supplied from the combustion of coke.77 Although the upgraders that are currently using coke for fuel are transitioning to natural gas, emissions of TEs such as V and Ni from coke combustion, and wind-dispersion of dust from coke stockpiles, are legitimate concerns. Several studies of V in coke have recently been published, with a view to understanding its forms and properties,78 as well as potential environmental significance.79,80
Misconception 4. TEs in the Lower Athabasca River, downstream of industry, occur in elevated concentrations, and occur mainly in the form of soluble, ionic species
In water samples collected from the main stem of the Athabasca River (AR), upstream, midstream and downstream of industry, most TEs are found predominantly in particulate form i.e. the size fraction >0.45 μm (Fig. 5). Of the elements found predominantly in dissolved forms i.e. <0.45 μm, they are either soluble cations (e.g. Li+, Sr2+) or elements which form anionic species such as Mo, U, Sb and Re (Fig. 5). Regarding the two most abundant metals in bitumen, in the autumn of 2014 and 2015, 50–81% of total Ni and 75–86% of total V was found in particulate form.82
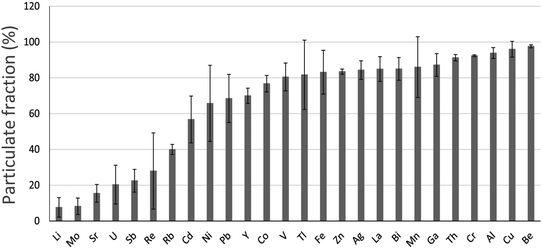 |
| Fig. 5 Relative abundance of TEs in the particulate fraction (>0.45 μm) of the Athabasca River (from Javed et al.,82 and reproduced with the permission of the publisher). These are average values (with standard deviations indicated) of samples collected in duplicate upstream and downstream of industry, during the autumn of 2014 (13 locations) and 2015 (19 locations). | |
In regard to the dissolved fraction of TEs, those of greatest perceived concern (Ag, Cd, Pb, Sb and Tl) may be found at very low concentrations in the main stem of the AR. In consequence, extreme precautions need to be taken in regard to acid cleaning of bottles, filters, and syringes before sampling, and great efforts are needed to minimize the risk of contamination during sample processing and analysis: the absolute necessity for these extreme precautions is described in detail elsewhere.30,83–89 As noted by Tölg and Garten (1985):90 “since it is primarily the blank value that limits the power of detection, it must be remembered that it is the ratio of the concentration of the element to be determined in the sample to its concentration in the environment of the analytical system which is decisive rather than the sensitivity of the detector system itself. Thus, in present day ultratrace analysis, it is more meaningful to keep the blank concentration as low as possible than to search for new and more sensitive detectors” (p. 489).90 In 2014, for example, the average concentrations (ng L−1) in the dissolved fraction of the AR, collected at 14 stations, were 1.7 ± 0.3 Ag, 3.2 ± 0.4 Tl, 13 ± 2 Cd, 20 ± 7 Pb, 48 ± 7 Sb.81 It should be added that these water samples were collected in the autumn, when the flow rate of the river is at its lowest, to maximize the chance to detect anthropogenic inputs. Similar concentrations were found in the autumn of 2015 when water samples were collected in duplicate at 19 stations.82 When water samples were collected at various locations and depths from the main stem of the river in the autumn of 2017 (n = 51) and the spring of 2018 (n = 39),91 remarkably similar concentrations for Cd, Pb, Sb and Tl were obtained for the dissolved fraction (Fig. 6). Thus, the data obtained for TEs such as Cd, Pb, Sb and Tl, in the dissolved fraction of the river, when collected and analyzed using metal-free, ultraclean procedures and protocols, even in the ng L−1 concentration range, are very reproducible.
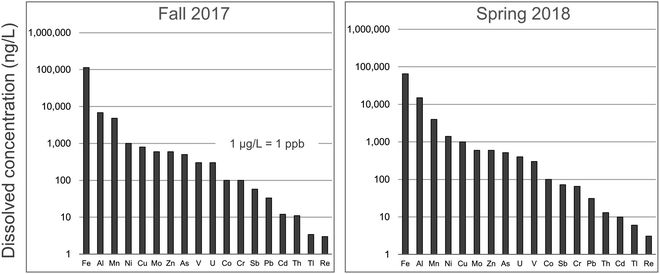 |
| Fig. 6 Concentrations of TEs in the dissolved fraction (<0.45 μm) of the Athabasca River. These are average values of samples collected upstream and downstream of industry, during the autumn of 2017 (52 locations) and spring of 2018 (39 locations). Data is from Ghotbizadeh.91 | |
Arsenic and Se require special consideration, as these two elements can be very challenging to determine using ICP-MS employing a single quadrupole mass analyzer, and an independent analytical method is recommended to ensure high quality data is obtained. In the AR water samples collected in 2014 and 2015, these elements were studied using a combination of ICP-MS and HG-AFS. Dissolved concentrations of As and Se were low, very similar between years, and not significantly different downstream versus upstream.92,93 Specifically, dissolved As averaged 0.37 ± 0.01 and 0.34 ± 0.01 μg L−1 in 2014 and 2015, respectively: these were dwarfed by total concentrations which averaged 12.7 ± 2.8 μg L−1 in 2014 and 3.3 ± 0.13 μg L−1 in 2015. The corresponding values for dissolved Se were 0.11 ± 0.02 μg L−1 (n = 14) in 2014 and 0.16 ± 0.02 μg L−1 (n = 21) in 2015. Using ion chromatography coupled to ICP-MS, the redox pairs As(V) and As(III) as well as Se(VI) and Se(VI) were determined, with the oxidized species dominating in both cases.92,93
It was suggested that bitumen mining and upgrading results in environmental contamination by Ag, Be, and Tl.4 Silver and Tl are rare elements: abundance in the UCC is only 53 and 90 μg kg−1, respectively; the abundance of Be in the UCC is only 2.1 mg kg−1.40 The reliable determination of Ag at the level found in the AR (at or below 1 ng L−1) is a considerable challenge. As noted elsewhere, similar Ag concentrations are found in open ocean seawater in the South Pacific and ancient arctic ice.81 Very few laboratories are capable of reliable measurements at these extremely low levels. Silver is unlikely to have any ecological relevance under these conditions, and for this reason efforts to measure this metal in AR water samples was discontinued by our laboratory soon after the 2015 field campaign. In regard to Be, the average concentration (ng L−1) in the dissolved fraction of the AR was 5.6 ± 1.4 (n = 13) and 5.1 ± 2.0 (n = 15) in the autumn of 2014 and 2015, respectively.82 These values are probably one-half the values for uncontaminated river water globally.94 Finally, in respect of Tl, as noted elsewhere, the values for the AR in 2014 were slightly below the reported value for global average uncontaminated river water.81
Misconception 5. All of the TEs of environmental significance can be easily and reliably measured in environmental samples using modern methods of instrumental analysis
The reliable determination of TEs in the dissolved fraction of surface and groundwaters of the Lower AR watershed, the filterable fraction of snowpack samples, and in the tissues of biota, remains a considerable analytical challenge. This is true, despite the impressive advances in sensitivity of modern methods of instrumental analysis. But even in regard to soil and sediment samples, where TE concentrations may be orders of magnitude greater, there are issues related to the challenges caused by incomplete digestion of refractory mineral phases.
Aqueous samples
Snowpack samples.
Based on snowpack sampling in the vicinity of open pit bitumen mines and upgraders, Kelly et al. (2010)4 claimed that industry was releasing to the AR 13 elements listed as priority pollutants by the U.S. Environmental Protection Agency (EPA): Ag, As, Be, Cd, Cr, Cu, Hg, Ni, Pb, Sb, Se, Tl, and Zn. In snow samples collected near development (ND), however, in the dissolved (i.e. <0.45 μm or “filterable” fraction), Ag, As, Be, Se were below the limits of detection (LOD) at all sites.4 The LODs in that study were comparatively high: in ng L−1, these LODs were 20, 250, 50, and 500. For perspective, the average concentrations of these elements in the dissolved fraction of the AR are well below these LODs: by approximately 50× in the case of Be,82 20 × for Ag,81 2× regarding As,92 and 3–4× in respect of Se.93
Trace elements have been measured by our laboratory in snow collected from five peat bogs in the vicinity of bitumen mines and upgraders.95,96 Lead is almost entirely found in the particulate fraction, with the concentrations in the filterable fraction on the order of 5 ng L−1.95 Within this fraction the average concentrations (ng L−1) of other elements of concern are Cd 1.9 ± 1.5, Tl 3.6 ± 1.2, Sb 19.8 ± 9.6 and As 40.8 ± 23.3 (Fig. 7a). Again, in an absolute sense, these are very low concentrations. For comparison with the dissolved fraction of the AR upstream of industry, Cd in the filterable fraction of snow is approximately 5 times lower, while Sb and As are approximately one-half of the concentrations found in the river. Thallium in the filterable fraction of snow is similar in concentration to the dissolved fraction of the river. Thus, the filterable fraction of snow near industry is an unlikely source of dissolved TEs to the river, simply because the elements are found almost exclusively in the particulate fraction (Fig. 7b).
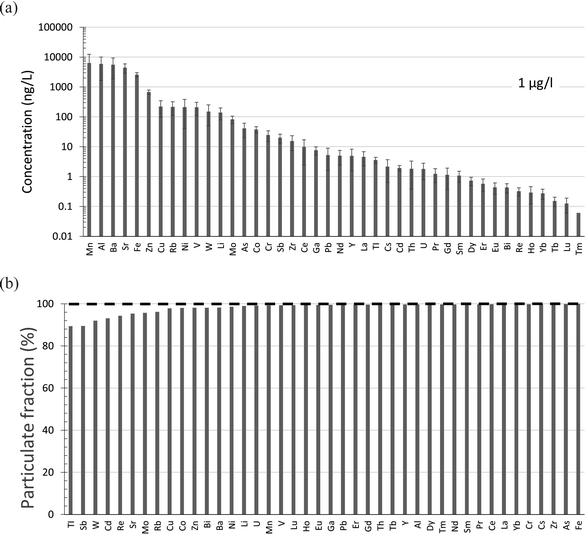 |
| Fig. 7 (a) Average concentrations (with standard deviations indicated) of TEs in the “dissolved” or filterable (<0.45 μm fraction) of snow collected from the same 5 peat bogs where peat cores were collected in the ABS region.97,98 The distances (km) from these sites to the mid-point between the two central bitumen upgraders are MIL 11, JPH4 12, McK 25, McM 49, and ANZ 68. (b) Percent of each TE found in the particulate fraction. Data from Javed et al.96 | |
Groundwaters.
The attempt by Savard et al.99 to determine TEs in groundwaters in the vicinity of tailings ponds failed, largely because the measured concentrations were either below or near the LODs. However, it should be noted that the LODs in this study were rather high (100 ng L−1) which renders them unsuitable for studies of TEs in groundwater. For perspective, the natural abundance of TEs in pristine groundwater from a carbonate terrain is summarized in Fig. 8. Notice that Cr, Ni and V are in the concentration range of 10 ng L−1 which is a factor of 10 below the LOD reported by Savard et al.99 Moreover Ag, Cd, Pb, Sb and Tl in pristine groundwater are all at or below 1 ng L−1 (Fig. 8) which is a factor of 100 below their LODs.99 The attempt to use stable Pb isotope ratios to trace the migration of oil sands leachate was poorly conceived, for many reasons. First, as noted above, the abundance of Pb in the ABS is below its abundance in the UCC. Second, Pb in the ABS occurs predominantly in the mineral fraction where it is hosted by comparatively insoluble minerals. Third, it is well known Pb is particle-reactive and readily scavenged in natural waters.100 Finally, as seen here (Fig. 8), Pb concentrations in groundwaters of carbonate terrains are extremely low: in fact, they may be at or well below the concentrations found in ancient Arctic ice.101 Thus, the determination of the isotopic composition of Pb in groundwaters would require the extreme “clean” lab methods used to study polar snow and ice,102–104 and most likely require sample preconcentration in order to obtain sufficient Pb for accurate measurements.
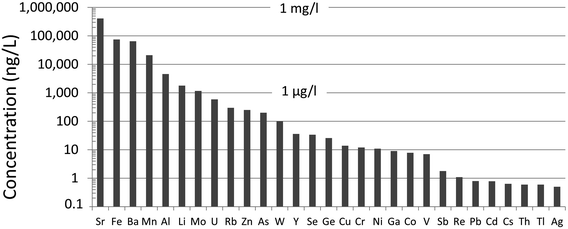 |
| Fig. 8 Abundance of TEs in pristine groundwater from a flowing, artesian well in a carbonate terrain (Elmvale Groundwater Observatory, Elmvale, Ontario). The water is sampled from an aquifer at a depth of 60 m, using a purpose-built well which is constructed entirely of surgical (316) stainless steel, and collected within a laminar flow, clean air cabinet. The concentrations are average values obtained from 10 (unfiltered) samples. The waters were collected in acid-cleaned polypropylene (PP) bottles containing 5 mL L−1 of HNO3 to acidify them; the acid had been purified twice by sub-boiling distillation in quartz. Unpublished data. | |
Solid samples
Acid digests of soils and sediments.
A recent paper on TEs in soils of the ABS region illustrates some of the technical challenges that remain in obtaining robust data for source assessments.13 The interpretation of their findings was evaluated critically and discussed in detailed elsewhere.105 Here, it is essential to draw attention to the quality of their analytical data. Based on their analyses of certified, standard reference materials (SRMs), the recoveries of Al were only one-third of the expected values. Arsenic was 245% of the certified value, and Ni 399 to 612% of the certified values. Aluminum is the most abundant metal in soils, and Ni is one of the more abundant TEs.106 When major and common TEs present analytical challenges, data on rare elements such as Ag, Be, Cd, Sb and Tl, often lacking certified values in SRMs, must be viewed with skepticism. The poor quality of the analytical data noted above may be partly problems associated with the instrumental method of analysis (e.g. interferences in the mass spectrum of ICP-MS instruments with a single quadrupole analyzer), but could also be due to the well known challenge associated with the complete dissolution of soil. Nitric acid alone is sufficient to completely liberate Cd, Co, Cr, Fe, Mn, Ni, Pb, V, and Zn in both soil and sediments, with recoveries ranging from 87%–120%.107 However, the use of HF or HFB4, in addition to HNO3, is necessary for nearly all other elements, to destroy the more recalcitrant minerals in soils. However, the blank contributions from HBF4 for Ag, Cd, and Tl are problematic,107 making it very challenging to measure these elements when they occur in soils at low concentrations. In the suspended solids from the AR, for example, the concentrations of Ag, Bi, Cd, Sb and Tl could not be determined with acceptable accuracy because of their low abundances combined with the challenge of blank contributions.82 For critical work requiring lower blank values for these elements, HF that has been distilled twice in Teflon is a better choice for mineral dissolution compared to HBF4 purchased commercially.39
Trace elements in biota.
A recent study in the ABS region used Deer Mouse (Peromyscus maniculatus) and Meadow Vole (Microtus pennsylvanicus) as biomonitors of TEs in the environment.23 However, Al along with Sr, Ba, Co and Ni were below the LOD in some of the samples. Again, when major elements such as Al and the more common TEs present problems to the analyst, the quality of the data for trace and ultratrace elements has to be carefully scrutinized. In the case of this study of small mammals, the data was obtained from a commercial laboratory.23 However, even for academic research laboratories using state-of-the-art laser ablation ICP-MS and ultraclean facilities, it can be very challenging to determine the concentrations of TEs in biota from the ABS region, simply because their concentrations may be very low. For example, otoliths (i.e. calcium carbonate ear bones) were analyzed from Trout-perch (Percopsis omiscomaycus), a non-migratory fish species collected along the AR, upstream and downstream of industry. However, 17 of 29 elements analyzed using state-of-the-art laser ablation (LA) ICP-MS of solid samples, in a clean lab environment, did not meet the threshold for data quality because >50% of samples were <LOD.108
Misconception 6. Old data on TEs in the environment are unreliable and no longer valid
Trace element concentration data from older publications certainly must be assessed carefully, given the number of analytical challenges that existed in the past. However, there are many examples of high quality data sets from early studies of TEs in the environment of the ABS region. These studies can be very helpful in establishing trends in TE concentrations as they often provide quantitative information from the past, from periods of time that are known, against which modern findings may be compared.
Sediments
Trace elements were investigated in sediments of the Lower AR watershed by Environment Canada.109 They collected bulk sediment samples at various locations along the AR, downstream of industry, as well as in the Peace-Athabasca Delta, and in Lake Athabasca. Samples were analyzed using a variety of techniques after full acid digestion at four separate laboratories. One of the laboratories also carried out selective dissolution to determine metal concentrations in distinct chemical fractions such as the organic matter fraction, the Mn and Fe oxyhydroxide fraction, and the insoluble silicate fraction. This report represents an excellent example of a well planned and carefully undertaken study to assess the extent of environmental contamination by TEs when bitumen mining and upgrading was in its early stages. Twenty-one samples were selected to represent locations in the major units of the main stem of the AR. Duplicate sets of the 21 samples, along with five blind duplicates, were submitted to each of four laboratories for determination of total element concentrations. Each lab used full acid digestion, either (a) HF (to destroy mineral matter) and HClO4 (to decompose organic matter), followed by evaporation and dissolution in HNO3 or (b) H2SO4, HF, and HCl, or (c) aqua regia (HNO3 + HCl). The analytical methods employed for V, Ni, Cu, Co, Zn, Mn and Fe included atomic absorption spectroscopy (AAS), and hydride generation AAS to determine As. While AAS is slow compared to the multielement analytical techniques we have today, it provided accurate and precision measurements of TE in the mg kg−1 concentration range38 which is similar to ICP-OES in regard to sensitivity.110
The results of the interlaboratory comparison proved successful: “on the basis of comparing the concentrations obtained by each laboratory for the blind duplicates, plus a comparison of the values for individual elements obtained by each laboratory for the sample suite, we concluded that the results for V, Ni, Cu, Zn, Mn, Fe and Hg provided by laboratory 3 appeared to be the most reliable, followed by those of laboratory 1. In the discussion section, we rely primarily on the results of these two laboratories. However, the concentration levels and variations are such that the results from any of the four laboratories could have equally well been used to arrive at the main conclusions viz. detection of anthropogenic inputs of heavy metals”.109 The authors ensured the quality of their analytical data by using four laboratories, four digestion procedures, a number of analytical methods to determine TE concentrations, duplicates and blind duplicates. In contrast to this effort, there is a disturbing number of contemporary studies on TE in the environment of the ABS region that pay no attention to the quality of sampling, sample preparation, or analysis.
Their main findings can be summarized as follows: “none of the heavy metal concentrations in any of the sediment samples were unusual. It is extremely unlikely that anthropogenic activities have enhanced concentrations in any of the analysed samples. Heavy metal concentrations were much lower than in sediments from contaminated drainage systems elsewhere in Canada or other countries. The concentrations are also lower than found naturally in other geological terrains. The distribution of metals does not evidence any point source inputs of anthropogenic origin. Variations in heavy metal concentrations between sample sites are largely controlled by sedimentological parameters including texture, carbonate content, organic content, and amorphous oxides. Mean concentrations represent natural geological background values that can be used for comparison with data obtained in the future”.109
This last point is worth emphasizing: the data presented in their report provides baseline concentrations of some relevant elements, including V and Ni, against which contemporary values can be compared.
Plants
Early work in the ABS region by Addison and Puckett111 employing lichens as biomonitors at 69 locations showed decreasing V concentrations with distance from mining and upgrading activities. Their map shows that samples nearest the upgrading operation at that time contained hundreds of mg kg−1 V, compared to 3 mg kg−1 at a distance of 85 km. At that time, 3 mg kg−1 was considered the “background” abundance of V in the lichens but today, this is the average concentration of V found in lichens112 and Sphagnum moss113–115 from the ABS region. It should be noted that the V concentration data of Addison and Puckett111 was obtained at that time using INAA, a sensitive, accurate, and precise method for the determination of V in solid samples which is still in use today.35 In fact, in the recent paper on TE in Sphagnum moss,113 although V concentrations were obtained using ICP-MS of acid digests, the concentrations were confirmed using INAA of solid samples at an independent laboratory. So, the V concentrations in lichens determined long ago by Addison and Puckett111 turn out to be extremely valuable, as they show that atmospheric V deposition rates in the ABS region were much greater in the past than they are today.112
A study undertaken by the Alberta Research Council of TEs in forest moss (Pleurozium schreberi) and the lichen Usnea spp. also documented declining rates of atmospheric V deposition.116 Moss samples were collected from many sites in the ABS region during 1976, 1983, and 1990, and lichens sampled during 1983 and 1990. The V concentrations given in the summary of elemental concentrations in that report show dramatic declines over time. In respect of the quality of the analytical data, the lichens had been subjected to a full acid digestion (HNO3, HClO4 and HF) and total concentrations of trace metals determined using ICP – atomic emission spectroscopy (ICP-AES). However, as an independent check, the authors also analyzed the 1976 and 1983 samples using INAA of solid samples, and the V concentration data obtained by the two independent methods were in excellent agreement.116
Snow
Early work employing snowpack sampling showed that 90% of V being deposited in the ABS region was in particulate form.117 A follow up study using snowpack sampling the following year showed a factor of ten decline in V loadings because of the installation of electrostatic precipitators in 1979 to reduce emissions of particulate matter during bitumen upgrading.118 For the convenience of readers, the relevant maps showing V loadings in snow collected in January of 1978 versus January and February of 1981, have been redrawn.98 All of this early work on V, in fly ash119 and in snow,117,118 employed INAA.
Water
In the State of the Athabasca River Watershed report prepared for the Athabasca Watershed Council,120 TE data from provincial and federal water quality monitoring programs were evaluated for the period between 2007 and 2011. Detection limits for parameters of interest were extracted from available sources. Useable TE data is mainly restricted to metals of comparative abundance such as Al, Fe, and Mn, as well as Cu and V. However, these reports contain very little useable data for most of the potentially toxic chalcophile TEs. These government agencies have since been criticized for poor water quality monitoring in the watershed. However, this is not simply an issue of inadequate LODs. In fact, as noted earlier, the TEs of concern are found at extremely low concentrations in the dissolved fraction of the AR,81,82,91–93,121 and present a challenge for most laboratories, especially for the ones providing routine analyses.
Misconception 7. The presence of potentially toxic TEs in soils, sediments, plants and waters from northern Alberta is due exclusively to anthropogenic inputs, and mainly from the development of the ABS
It is important to recognize that all of the elements of the periodic table, from H to U, except for technetium (Tc) and promethium (Pm), occur naturally at the surface of the Earth. Eight major elements account for more than 98% of the mass of the Earth's crust: listed in decreasing order, these are O, Si, Al, Fe, Ca, Na, K and Mg; these elements range in abundance from 46.6 to 1.5 weight percent.40 These are followed by the minor elements which, in order of decreasing abundance, are Ti, Mn, P, Ba, S, F, Cl, Sr and Zr; these range in abundance from 0.38 (Ti) to 0.02 (Zr) weight percent.40 Trace elements are those elements whose abundance in the UCC is below 100 mg kg−1. In this category we find elements of environmental concern, ranging from V (97 mg kg−1) through Cr, Zn, Ni, Cu, Co, Pb, As, Be to Mo (1.1 mg kg−1). The final group of elements, all found in the UCC at levels less than 1 mg kg−1, can be considered ultratrace elements. The abundance of these elements range from Tl (90 μg kg−1) through Sb, Bi, Se, Cd to Ag (53 μg kg−1). Thus, the potentially toxic TEs of environmental concern are found throughout the lithosphere, pedosphere, hydrosphere, biosphere and atmosphere. For each element, therefore, there exists a natural “background” concentration which must either be known or become established, before anthropogenic contributions can be quantified.
Soils and sediments
All of the naturally occurring elements are found in soils and sediments, given that these materials are derived from the physical, chemical and biological weathering of crustal rocks. In general, soils and sediments are mainly made up of mineral matter, but TE concentrations become diluted as organic matter is added and incorporated. So, as a rough guide to the natural levels of TE in soils or sediments, we can start with the abundance of the element in the UCC, and apply an appropriate dilution factor, based on the amount of organic matter.105 For example, the abundance of Pb in the UCC is 17 mg kg−1:40 in a soil containing 5% organic matter, and assuming there are no anthropogenic contributions, a minimum dry weight Pb concentration of 16.2 mg kg−1 would be expected. This simplistic calculation is only a rough guide, as it does not take into account geology, mineralogy, texture, topography, age of the soil or weathering intensity which, in turns, depends on climate. Here, the main point being made is simply that TEs occur naturally in all soils and sediments, and therefore a background concentration, or range in concentrations, exists for each element. Some TEs are lost to solution during chemical weathering of rocks and soils (e.g. alkali and alkaline earth metals) whereas others occur in very stable mineral phases and become residually enriched (e.g. the conservative lithophile elements). The summary by Chesworth (1992)122 provides a helpful introduction to these transformations in soils and sediments.
When soils are eroded by wind and water, physical fractionation takes place whereby fine-grained and coarse-grained materials become separated. The pioneering study of TEs in sediments of the Lower AR watershed by Allan and Jackson109 describes this winnowing effect, and its consequences for TEs as the river reaches the Peace-Athabasca Delta. “Higher heavy metal concentrations are associated with the finer textured samples, those found downstream at sites where sediment deposition predominates. The relationship between finer textured, more organic and clay-rich sediments and slightly higher heavy metal concentrations can be seen for V and Ni and Hg and As. This relationship is general in nature because surficial sediments including river bottom sediments are normally composed of quartz, feldspars, carbonates, layer silicates, small amounts of heavy minerals, amorphous inorganic oxide and hydroxide coatings, and organic material. In a system affected only by natural loadings of heavy metals, the sediment geochemistry at any one site should result from the availability of heavy metals in geological source materials and sedimentological and fluvial parameters. At high energy sites, sediment will be coarse grained, dominated by silica, feldspar, and heavy minerals and have a low organic content. At low energy sites, sediment will be fine grained, made up largely of clay sized layer silicates and have a higher organic content. Because of their affinity for clay sized materials via sorption and exchange processes heavy metal concentrations should be naturally higher at these low energy sites”.109 This study is an excellent reminder that variations of TEs in soils and sediments must duly consider all of the natural processes which give rise to particle separation and fractionation, mineral redistribution, creation and addition of amorphous phases and organic matter, and chemical diagenesis; these factors, and their significance, is described in the seminal texts on the subject e.g. Förstner and Müller,123 Salomons and Förstner,124 Förstner and Wittman,125 and Stumm and Morgan.126
Plants
Trace elements in plants are derived from two main sources: atmospheric deposition, and root uptake. For elements that are neither actively nor passively taken up by roots from soil, or taken up only to a limited extent, atmospheric deposition can easily be the dominant source. One way to estimate the natural, background concentrations of TEs in plant materials is to analyze peat cores from ombrotrophic (i.e. rain-fed) bogs. For example, in Europe, ancient peat, 6000 to 9000 years old, pre-dating mining and metallurgy, and forest clearing for agriculture, contains the following average concentrations (μg kg−1) of TEs: 750 Cr, 550 V, and 460 Ni,127 280 Pb,128 133 As,129 8 Sb130 and 4 Tl.131 Peat bogs in Europe and North America all have remarkably similar natural background Cu concentrations: 1 mg kg−1.132 Of this list of elements, both Ni and Cu are essential to plants. Thus, the concentrations of Cu and Ni reflect both plant uptake as well as dust deposition. But the other elements listed here are not essential to plants, and would have been supplied exclusively or predominantly by atmospheric deposition. Ignoring increases in concentration due to decomposition and humification, these fossil plant materials from the Neolithic Period can be used to provide an estimate of the natural, background concentrations of TEs in contemporary plants.
Another way to estimate the natural abundance of elements in plants is through the analysis of bryophytes (such as mosses) from remote locations.133,134Sphagnum moss has been used as a biomonitor of atmospheric deposition of TEs for decades (see Shotyk et al.135 for a brief introduction). Sphagnum moss was collected from bogs in two very remote locations in northern Alberta: in the Birch Mountain Wildlands (BMW) and Caribou Mountain Wildlands (CMW), Wildlands. These sites are approximately 135 and 365 km, respectively, NW of the Town of Fort McMurray. Further, they are 59 and 89 km, respectively, from the nearest roads. Lead concentrations in Sphagnum moss from these sites are at or below the natural, background concentrations noted above. Specifically, total Pb concentrations are 177 ± 15 μg kg−1 and 186 ± 54 μg kg−1, respectively (Table 2). Given that the geochemical cycle of Pb has been affected by anthropogenic activities more than any other TE,136 we can assume that Sphagnum moss from BMW and CMW provides a guide to contemporary background values for other TEs. However, lithophile elements such as Al, Co, Cr, Fe, Li, Mn, Sc, Th, U and Y are all elevated at BMW, compared to CMW. Assuming similar plant growth rates at the two sites, these differences suggest that BMW receives greater inputs of dust, possibly from open pit bitumen mining operations. However that may be, Ag, Cd, Cu, Pb, Sb, Tl and Zn are similar at the two sites and can be taken to reflect contemporary background values of these elements. Arsenic, Pb and Tl in the moss from these sites, along with V and Ni, are at or below the levels found in the ancient peat, while Cu and Sb are within a factor of two of those values. In summary, whether they are contemporary samples growing at remote locations far from industrial activities, or fossil plant materials pre-dating anthropogenic activities, there exists a background concentration, or range in values, for all TEs in plants, even for the most toxic elements. These background values must be known or otherwise established, in order to be able to quantify TE contributions from human activities.
Table 2 Concentrations of trace elements in Sphagnum moss collected in triplicate from Birch Mountain Wildlands (BMW) and Caribou Mountain Wildlands (CMW). The samples were digested and analyzed as described in detail elsewhere.113 Unpublished dataa
Element |
Units of concentration |
BMW |
CMW |
Average |
std. dev. |
Average |
std. dev. |
For convenience, elements at or below 0.1 mg kg−1 are expressed in μg kg−1 except for Re (ng kg−1).
|
Ag |
|
μg kg−1 |
|
12.5 |
2.9 |
13.1 |
3.3 |
Al |
mg kg−1 |
|
|
259 |
12 |
138 |
31.6 |
As |
|
μg kg−1 |
|
122.8 |
40 |
79.3 |
37.1 |
Ba |
mg kg−1 |
|
|
22.0 |
9.1 |
20.5 |
6.1 |
Be |
|
μg kg−1 |
|
5.82 |
0.3 |
3.28 |
1.5 |
Cd |
|
μg kg−1 |
|
23.9 |
2.3 |
31.4 |
9.6 |
Co |
|
μg kg−1 |
|
109.2 |
7.4 |
52.2 |
5.7 |
Cr |
|
μg kg−1 |
|
399 |
20 |
231 |
51.3 |
Cu |
mg kg−1 |
|
|
1.68 |
0.1 |
1.73 |
0.1 |
Fe |
mg kg−1 |
|
|
201 |
15 |
109 |
21.3 |
Ga |
|
μg kg−1 |
|
99.8 |
7.0 |
63.3 |
3.5 |
Li |
|
μg kg−1 |
|
464 |
329 |
98 |
6.6 |
Mn |
mg kg−1 |
|
|
797 |
338 |
528 |
262 |
Mo |
|
μg kg−1 |
|
68.3 |
3.7 |
51.0 |
13.6 |
Ni |
|
μg kg−1 |
|
417 |
93 |
195 |
55.9 |
Pb |
|
μg kg−1 |
|
177 |
15 |
186 |
53.6 |
Rb |
mg kg−1 |
|
|
8.93 |
0.3 |
6.13 |
0.1 |
Re |
|
|
ng kg−1 |
219 |
118 |
44.3 |
27.7 |
Sb |
|
μg kg−1 |
|
12.6 |
1.1 |
12.2 |
5.6 |
Sc |
|
μg kg−1 |
|
46.5 |
4.0 |
19.5 |
2.2 |
Se |
|
μg kg−1 |
|
161 |
91 |
128 |
87.6 |
Sr |
mg kg−1 |
|
|
4.11 |
0.4 |
3.06 |
0.4 |
Th |
|
μg kg−1 |
|
45.8 |
4.7 |
25.6 |
7.0 |
Tl |
|
μg kg−1 |
|
5.75 |
1.5 |
4.22 |
1.3 |
U |
|
μg kg−1 |
|
15.6 |
0.6 |
9.1 |
1.6 |
V |
mg kg−1 |
|
|
0.76 |
0.1 |
0.37 |
0.0 |
Y |
|
μg kg−1 |
|
112.8 |
7.8 |
55.5 |
11.4 |
Zn |
mg kg−1 |
|
|
17.5 |
2.8 |
17.9 |
4.6 |
Water
Similarly, all of the elements found in the UCC occur in all natural waters, including rainwater, surface waters, soil and sediment porewaters, and groundwater, because of the complete or partial dissolution of minerals which constitute the rocks of the Earth's crust. The TE concentrations in the dissolved fraction of the AR shown in Fig. 6 are average values taken along the transect investigated from Fort McMurray travelling downstream, approximately 125 km to the Firebag River.91 Given that upstream and downstream concentration differences are modest, if they exist at all, these values are typical of upstream concentrations, and can be seen as a guide to the natural abundance of these elements in the river. The concentrations of TEs range over many orders of magnitude, from elements such as Al, Fe, Mn and Sr in the μg L−1 concentration range, to Ag, Cd, Pb, Sb and Tl in the ng L−1 range (Fig. 6). Again, therefore, even the most toxic of the TEs have a natural abundance, and these values must be established, to quantify anthropogenic contributions. In the case of large river systems such as the Athabasca, there is usually tremendous spatial and temporal variation: these also must be taken into account, in any studies designed to identify and quantify anthropogenic contributions.137,138
Misconception 8. Enrichments of TEs in biota are exclusively due to environmental contamination
Plants
Recent studies provide examples of pronounced natural enrichments of TE in biota. For example, Cu, Fe, Mn, Mo, Ni and Zn are enriched in wild cranberries (Vaccinium oxycoccus) collected from remote locations, relative to the substrate on which they grow, due to plant uptake via roots.139 These elements are micronutrients and essential for the growth of the berry plants.140 Thus, active uptake of these elements from the substrate is to be expected. However, it was clear that the berries were also actively taking up Cd from the substrate.139 This was true, even though the berries were collected from the CMW peat bog, an extremely remote site in northern Alberta where Pb concentrations in the underlying moss suggest that atmospheric deposition of TEs is at natural background values. Active uptake of this same suite of micronutrients, as well as Cd, has also been reported for Labrador Tea (Rhododendron groenlandicum), another common peat bog plant, even at remote locations.141 Both papers also provide evidence of passive uptake by cranberries and Labrador Tea of Ba, Rb and Sr, none of which is essential to plants.
The uptake of Ni by plants can also be seen in Rat Root (Acorus calamus). Twenty wild plants were harvested at two sites, approximately 1 km apart, along the south shore of Chip Lake, Alberta.142 This lake is approximately 125 km W of Edmonton, and far removed from the ABS region. The Ni to V ratio can be used to document metal uptake, as Ni is essential to plants140 whereas V is not. In the wild plants, the Ni/V ratio is almost a factor of 10 greater than the corresponding value for the Athabasca River, and more than 90 times the ratio in the UCC (Fig. 9). Uptake of Ni by plants is also seen in pine trees (Pinus banksiana) growing in soils developed from natural bitumen deposits versus soils free of bitumen. Trace elements were determined in tree cores, but also soil and soil solutions.143 Nickel concentrations in wood from trees growing on shallow bitumen deposits were approximately 3× greater than those growing in bitumen-free soils.
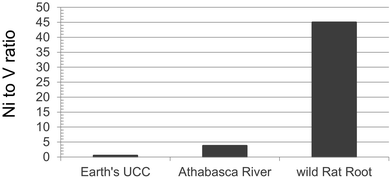 |
| Fig. 9 Ratio of Ni to V in wild Rat Root (Acorus calamus) collected in September of 2016 at Chip Lake, Alberta (53°36′35′′N 115°18′10′′W). Data from Gariepy.142 Values for the Athabasca River refer to average ratios within the dissolved fraction, autumn of 2014.81 The ratio for the UCC is from Rudnick and Gao.40 | |
Animals
Thallium and many other TEs (including Ba, Cr, Co, Cu, Fe, Mn, Mo, Ni, Pb, Sr, V and Zn) were determined in otoliths (calcium carbonate ear bones) of Trout-perch (Percopsis omiscomaycus), collected along the AR.144 The ear bones of these small fish are tiny, but quantitative measurements were made possible simply by dissolving individual otoliths (3–15 mg) in a small volume (200 μL) of double-distilled HNO3 for 30 minutes, before diluting them with ASTM Type I water and analyzing them using ICP-MS. Compared to their average abundance in the dissolved (<0.45 μm) fraction of AR, Tl showed the greatest enrichment in otoliths of any TE.144 The extent of Tl enrichment was not significantly different downstream of industry compared to upstream. This natural bioaccumulation of Tl in fish most likely reflects the ability of Tl+ to pass through the potassium ion channel in cell membranes: it is the only metal to pass as easily K+.41
In an effort to determine the natural abundance of TE in tissue of beaver (Castor canadensis), animals were harvested from a small watershed with no point source of environmental contamination by TEs, at a rural location in southern Ontario.145 Chalcophile (Ag, Cd, Co, Cu, Mo, Ni, Pb, Se, Tl, Zn) and lithophile (Al, Ba, Ce, Cr, Cs, Fe, La, Li, Mn, Nd, Rb, Sr, V, Y) trace elements (TEs) were determined in kidney, liver and muscle. To estimate the relative bioavailability of TEs in the landscape, they were also determined in the dissolved (<0.45 μm) fraction of water from the river where the animals were harvested. By far the greatest concentration ratios (tissue/water) were found for Cd (kidney, 3.6 × 106; liver, 1.1 × 106; muscle, 3.4 × 105). Despite its potential toxicity, Tl also showed considerable enrichment, with the following concentration ratios: kidney, 1.3 × 104; liver 5.5 × 103; muscle 8.2 × 104. When the Cd/Tl ratio of beaver kidney is compared with the corresponding ratio in the UCC and relevant samples from the watershed where the beaver were taken (sediments, stream water and bark from local vegetation), it becomes clear that the main source of Cd is from the vegetation that makes up the diet of these animals (Fig. 10).
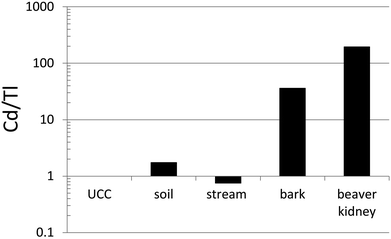 |
| Fig. 10 Ratios of Cd to Tl in beaver kidney,145 compared to bark (average values for 40 species of trees and shrubs from the farm property in southern Ontario where the beaver were collected), stream (Wye River from the same farm), soil (from the same farm; average of 10 samples taken from 13 m of sediment representing approximately 12 000 years of accumulation; the soil of the farm is derived from these sediments), and the Upper Continental Crust.40 The Wye River data is from Shotyk et al.,145 whereas the bark and sediment data are unpublished. | |
In summary, even in remote locations with background rates of atmospheric metal deposition, uptake of Cd by plants has been documented. In fish from control locations, we see that Tl is more enriched in otoliths than any other TE. In beaver from a rural watershed, Cd is enriched in kidney, liver and muscle more than any other TE. Sphagnum moss, Labrador Tea, Rat Root, Trout-perch and beaver are all promising biomonitors of environmental contamination by TEs. But these studies show that it is vital to first establish the natural background concentrations and extent of enrichment of TEs, before anthropogenic contributions can be determined.
Misconception 9. Emissions of TEs to the environment in northern Alberta have been increasing over time, due to increasing industrial development
There has been a profound increase in the production of synthetic crude oil in northern Alberta since commercial extraction and upgrading of bitumen began in 1967 (Fig. 11). If open pit bitumen mining and upgrading was contributing significant quantities of TEs to the environment, then one would intuitively expect to see a similar increase in the rates of accumulation of TEs in various archives. However, using age-dated peat cores from ombrotrophic bogs collected near mines and upgraders,97 it is clear that atmospheric Pb contamination has been in decline for decades (Fig. 12). Using the same peat cores, it was shown that Ag, Cd, Sb and Tl also had been declining for decades;98 the same is true of As and Se.148 Even the extent of atmospheric contamination by V, Ni and Mo, the three most abundant trace metals in bitumen, show similar declines in the peat profiles.98 Independent studies of age-dated sediment cores from the Peace Athabasca Delta also show declines in many of the same TEs, including As, Pb, and Sb.149 Further, the authors found no increase in concentrations of Be, Cd, Cr, Cu, Ni, V and Zn, relative to pre-industrial levels in the sediments.150
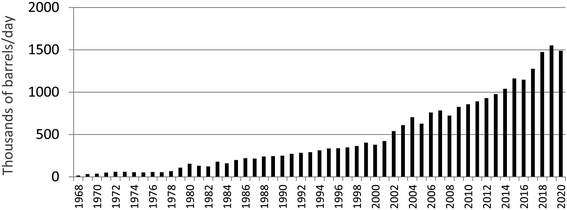 |
| Fig. 11 Bitumen production from mining in Alberta, since 1968. Downloaded from http://www.oilsandsmagazine.com/ (November 16, 2021) and used with permission.146 | |
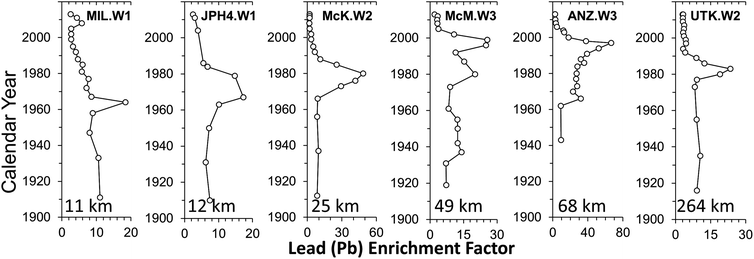 |
| Fig. 12 Crustal enrichment factor, calculated using Th, for Pb in peat cores from 5 bogs in the ABS region (MIL, JPH4, McK, McM, ANZ) and UTK, the control site located 264 km from the mid-point between the two central upgraders.97 The peat cores were dated using a combination of 210Pb and 14C, including the atmospheric bomb pulse curve.147 | |
Open pit bitumen mines generate tremendous volumes of dust, from the mines themselves, from haul roads, gravel roads, aggregate quarries, etc.151,152 Analyses of the ash contents of Sphagnum moss show increasing rates of dust deposition with increasing distance toward industry.153 Similarly, wild berries collected near the mines have more dust on their surfaces than berries collected from rural and remote locations.154,155 In the vicinity of the bitumen mines, using the same age-dated peat cores from bogs as noted above, increasing rates of dust deposition over time were documented.156 The declines in atmospheric deposition of TEs over time in the peat cores from bogs appear to contradict the increases in dust deposition over time at the same locations. However, part of the explanation may lie in the chemical analyses of dust particles extracted from the snow collected at these same bogs: the concentrations of Cd, Pb, Sb and Tl, and even V and Ni, in the dusts, are well below or far below, the corresponding crustal values.96
Misconception 10. TEs released to the environment in mineral or particulate forms have the same ecological significance as ionic forms of the same elements
For many TEs, the Guidelines for the Protection of Aquatic Life proscribed by the Canadian Council of Ministers of the Environment (CCME) are based on total concentrations. There are a number of scientific and technical limitations with this approach. In the case of the AR, total concentrations of TEs are dominated by particulate forms (Fig. 5), and particle concentrations can show extreme seasonal variation. Total concentrations of suspended solids (TSS) can be very high in the AR in the spring during snowmelt. For example, at the Town of Athabasca, far upstream from the oil industry, TSS concentrations can reach up to 1200 mg L−1.120 Assuming these solids contain TEs in the same proportions as the UCC, a simple calculation shows that 1200 mg L−1 of TSS will yield total concentrations of As, Cu and Pb of 5.8, 20.4 and 33.6 μg L−1 which all exceed the corresponding CCME guideline values. Thus, under circumstances governed exclusively by natural processes, in a section of the river where there has been no indication of anthropogenic inputs of TEs, TE concentrations may exceed guideline values for the protection of aquatic life.
What about the chemical reactivity of the particles themselves ? Studies of particles suspended in the AR using sequential extraction157 have shown that As and Cu are found almost exclusively, and Pb (and Tl) predominantly, in the residual, mineral fraction (Fig. 13). Regarding elements such as Ag, Cd and Sb, efforts to fractionate them from suspended solids using sequential extraction have failed thus far, as their concentrations are so low.157 Within the residual, mineral fraction, TEs are incorporated within the crystalline structures of various silicates, most likely dominated by those of the feldspar group, micas, and clays. In such cases, TEs will not be released to the aquatic environment at a significant rate, given that (a) the pH of the AR120 is approximately 8 and (b) the dissolution rate of silicate minerals is at a minimum at this pH:158 much lower or much higher pH values are needed to hydrolyzes the critical Al–O bonds at an appreciable rate.159 In other words, elements such as Pb contained within silicate minerals will have no direct relevance to biota. Even ingestion by aquatic organisms, despite the extremely low pH of digestive fluids, seems unlikely to have any measurable effect on such stable mineral phases, given how quickly food particles pass through the gut of the animals.
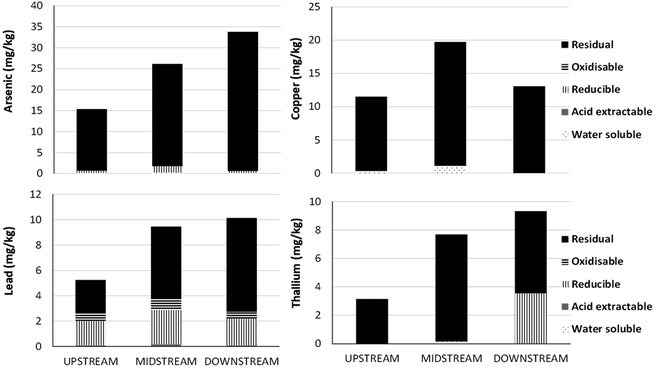 |
| Fig. 13 Sequential extraction of As, Cu, Pb and Tl using suspended solids collected from the Athabasca River. Average values for samples collected within the upstream (n = 3), midstream (n = 2), and downstream (n = 2) sections of the river. From Javed and Shotyk.157 | |
It is well known that “dissolved” concentrations provide a much better indication of potentially toxicity to aquatic organisms, compared to “total concentrations”.160 From an environmental or ecological perspective, the dissolved concentrations are clearly far more important than the totals. For this reason, water quality guidelines in the EU and the USA are based on dissolved concentrations. Referring again to the AR, it is remarkable how different total concentrations are between spring and fall (high flow versus low flow conditions) while at the same time, just as remarkable how similar the dissolved concentrations are (Fig. 14). Dissolved concentrations of V and Pb, for example, are effectively identical during spring and autumn, while total concentrations are five times greater in spring compared to autumn (Fig. 14). Ironically, even though particulate forms of V and Pb dominate the inventory of these elements in the river, toxicity testing of these metals to biota employ ionic forms of the elements.22,161
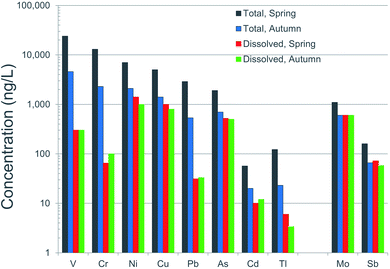 |
| Fig. 14 Comparison of total and dissolved (<0.45 μm) concentrations of trace elements in the Athabasca River. These are average values of samples collected upstream and downstream of industry, during the autumn of 2017 and spring of 2018. Data is from Ghotbizadeh.91 | |
In regard to bioavailability of TEs, as noted by the US EPA more than 50 years ago, “toxicity is dependent upon the ionic, salt, or complex forms of the element”.162 Similarly, in an early study on TEs in precipitation of Ontario, it was noted that “it is becoming increasingly clear that simple determination of total component concentration provides insufficient information to allow evaluation of its environmental impact”.163 Further, that “determination of ‘free’ component concentrations should be the ultimate aim of the researcher”.163 Within the dissolved fraction, the elements that are directly bioavailable are those in ionic form, or simple complexes with molecular mass below 500 Da, as these can directly pass through cell membranes.164 Efforts to determine free metal ion concentrations include calculations of the distribution of dissolved species using equilibrium chemical thermodynamics165,166 or direct measurements using electrochemical methods.167 The importance of chemical speciation for understanding the bioavailability of TEs is well established.168
Today, we have technologies such as flow-field flow-fractionation (AF4) coupled to ICP-MS to measure the concentrations of many TEs in the dissolved (i.e. <0.45 μm) fraction as a function of molecular size,169 and this technique has been applied to surface waters of the AR watershed.121 Using a 300 Da membrane, these efforts have shown that some TEs in the AR are found primarily or exclusively in the form of ions or simple complexes (e.g. Li, Sr) while others are to a large extent associated with organic or inorganic colloids (e.g. Cu, Pb). Given that the larger organic and inorganic colloids range in size from approximately 1 to 20 kDa,121 the TEs they contain are expected to have limited bioavailability. However, TEs associated with these materials may be potentially bioavailable i.e. they may be bioaccessible, depending on the lability and stability of the colloids.170–172 In summary, we have the technology today to reliably determine total, dissolved, and “truly dissolved” concentrations of TEs in the AR, for most of the potentially toxic TEs of interest. Regarding TEs that cannot be separated into these classes using AF4-ICP-MS i.e. Ag, Cd, Sb, and Tl, even with using state-of-the-art metal-free, ultraclean laboratory facilities, their concentrations in the AR are so low that they are very unlikely to have any ecological relevance.
Summary and conclusions
Mining and upgrading of bitumen have given rise to five decades of studies of TEs in the environment of the Lower AR watershed. The underlying assumption in most of these studies is that the ABS contain elevated concentrations of TEs, and that industrial development mobilizes them in quantities and forms that threaten terrestrial and aquatic life. In fact, the ABS ores are depleted in every element we have measured, relative to the UCC, with the exception of Mo and Re. The distribution of TEs in the ABS is dichotomous: V, Ni, Mo, Se and Re are found almost exclusively in the bitumen fraction, with enrichments of approximately 2 (V, Ni), 4 (Se), 9 (Mo) and 250 times (Re), relative to the UCC. All of the other TEs occur in the mineral fraction which is made up mainly of quartz sand.
Pioneering environmental studies focused on V, as this is the most abundant metal in bitumen, but more recent studies have shifted focus to emphasize the potentially toxic chalcophile elements (Ag, As, Cd, Pb, Sb, Tl) which along with Be and Cr, are found almost exclusively in the mineral fraction. With the number of studies and diversity of sample media being collected in the region, a considerable volume of data is being created, but varying in quality. Modern, multielement methods of instrumental analysis such as ICP-MS are excellent, but certainly not perfect, and cannot be used uncritically. Elements such as As and Se, for example, pose serious challenges because of molecular interferences in the mass spectrum. While these issues can be addressed using collision cell technologies and overcome using medium or high mass resolution using sector-field ICP-MS, there are very few of these larger, more sophisticated instruments being applied in this region. Further, acid digestion of solid samples continue to present challenges, and complete dissolution of certain phases in soil and sediment samples remains difficult. Older, established methods such as INAA do not require samples to be digested, and provide high quality data with excellent sensitivity for some lithophile elements such as Al, Cr, Sc, Th and V, but also some chalcophile elements such as As and Sb. In fact, the early measurements of V in bitumen, fly ash, snow, lichens and moss are excellent, despite their antiquity, and provide useful reference levels, for comparison with modern samples.
In regard to aquatic inputs of TEs to the AR, most of the elements of concern are found predominantly in the particulate fraction. In consequence, total concentrations of TEs in the AR show extreme seasonal variation, with springtime values commonly two to five times greater than those in autumn. Using ultraclean lab procedures and protocols, TE concentrations within the dissolved fraction are found to be extremely low (e.g. ng L−1 level for Ag, Be, Cd, Pb, Sb, Se and Tl), very similar in spring and autumn, and are remarkably reproducible, year after year. Measuring TEs as a function of molecular size within the dissolved fraction shows that some of the TEs of greatest concern, are associated to a great degree with, or dominated by, colloidal forms. Therefore, the “truly dissolved” fraction of TEs such as Pb i.e. ionic forms and simple inorganic and organic complexes, occur at extremely low concentrations.
Regarding atmospheric deposition, most TEs in Sphagnum moss and in snow, are associated with dust particles; concentrations in the filterable fractions are very low. Further, retrospective analyses using peat cores from bogs and sediment cores from the Peace-Athabasca Delta, show that emissions of TE to the environment in this region have been in decline for decades. With respect of TEs in biota, more effort is needed to determine the natural abundance of elements in plants and animals from remote or at least rural regions, for comparison with samples from industrialized areas. Also, the natural enrichment of elements such as Cu, Ni, Zn, Cd and Tl in plants and animals, living far removed from industrial activities, warrants further investigation. Similarly, the natural processes that enrich TEs in soils and sediments, relative to UCC, including physical and chemical weathering, as well as winnowing during wind erosion and sorting during water erosion, must be duly considered.
Environmental monitoring requires clearly defined scientific questions, well planned sampling campaigns which include appropriate control locations, and high quality analytical data. As noted more than 50 years ago by investigators on both sides of the Atlantic, we must move beyond total concentrations of TEs, and focus on the physical and chemical forms that have ecological significance. And we need to remember that each TE has a natural source, in addition to possible anthropogenic contributions. As our analytical methods continue to improve, we need to also remind ourselves that, just because we can measure a given element in a sample, this does not imply any relevance of that element for biota.90
Epilogue
This article was first written in draft form approximately eight years ago, before the SWAMP laboratory was constructed, and before we had any of our own data regarding TEs in the environment of the Lower Athabasca River watershed. The predictions made in that early manuscript turned out to be true: this simply means that the results being obtained today were, by and large, easily predicted, based on the pioneering geochemical studies of the past, starting with giants such as Vernadsky and Goldschmidt. This is perhaps a reminder that an understanding of the environmental impacts of resource development must begin with a quantitative understanding of the resource itself. Further, that the dispersion of elements in the atmosphere, biosphere, hydrosphere and pedosphere, and their transformations therein, follow physical and chemical principles that were discovered by pioneers of science, too numerous to list here, and summarized in their early publications. Despite the tremendous advances in analytical instrumentation and our growing abilities to determine concentrations and forms of TEs at extremely low concentrations, the Periodic Table of the Elements is still the most powerful tool in chemistry, allowing us to develop an understanding of the sources and potential transformations of the elements in the environment, based upon their electronic structures.
The misconceptions laid out in this work will be corrected by the progress of science. However, the fact that they have persisted for so many years brings with them significant costs, namely, the misdirected time, effort and research funds that have chased faulty hypotheses built upon them.§.
Conflicts of interest
There are no conflicts of interest to declare.
Acknowledgements
Our research in the Lower Athabasca River watershed has been generously supported by Alberta Innovates (AI), Canada's Oil Sands Innovation Alliance (COSIA), and the Natural Sciences and Engineering Research Council of Canada (NSERC). Special thanks to John Zhou, Brett Purdy and Dallas Johnson of AI for their continual insight and helpful perspectives, and to John Brogly of COSIA. Thanks also to the University of Alberta, the Faculty of Agricultural, Life and Environmental Sciences, and Alberta Environment and Parks for munificent start-up funds for the SWAMP lab, the Canada Foundation for Innovation for a generous equipment grant and matching funds from Alberta Enterprise and Advanced Education. Thanks to Muhammad Babar Javed and Marjan Ghotbizadeh for sharing their data and providing figures, to Rick Pelletier for the map, as well as Na Chen and Andrii Oleksandrenko for compiling the bibliography. I am grateful to Fiorella Barraza, Mandy Krebs, and Muhammad Babar Javed for reviewing a draft of the manuscript, to the reviewers for their comments on the submitted version, as well as helpful suggestions from the Editor. Finally, special thanks to the SWAMP laboratory members, past and present, for the high quality data presented here and elsewhere. This paper is dedicated to the legacies of V. I. Vernadsky and V. M. Goldschmidt who would have known it must be so.
References
-
P. Gosselin, S. E. Hrudey, M. A. Naeth, A. Plourde, R. Therrien, G. Van Der Kraak and Z. Xu, Environmental and Health Impacts of Canada’s Oil Sands Industry, Report to the Royal Society of Canada, 2010, accessed April 9, 2012, available at http://www.cwn-rce.ca/news-and-events/news/rsc-env-and-health-impacts-of-canada-osi/ Search PubMed.
- M. M. Lynam, J. T. Dvonch, J. A. Barres, M. Morishita, A. Legge and K. Percy, Oil sands development and its impact on atmospheric wet deposition of air pollutants to the Athabasca Oil Sands Region, Alberta, Canada, Environ. Pollut., 2015, 206, 469–478 CrossRef CAS PubMed.
- C. Phillips-Smith, C. H. Jeong, R. M. Healy, E. Dabek-Zlotorzynska, V. Celo, J. R. Brook and G. Evans, Sources of particulate matter components in the Athabasca oil sands region: investigation through a comparison of trace element measurement methodologies, Atmos. Chem. Phys., 2017, 17(15), 9435–9449 CrossRef CAS.
- E. N. Kelly, D. W. Schindler, P. V. Hodson, J. W. Short, R. Radmanovich and C. C. Nielsen, Oil sands development contributes elements toxic at low concentrations to the Athabasca River and its tributaries, Proc. Natl. Acad. Sci. U. S. A., 2010, 107, 16178–16183, DOI:10.1073/pnas.1008754107.
- M. A. Bari, W. B. Kindzierski and S. Cho, A wintertime investigation of atmospheric deposition of metals and polycyclic aromatic hydrocarbons in the Athabasca oil sands region, Canada, Sci. Total Environ., 2014, 485–486, 180–192, DOI:10.1016/j.scitotenv.2014.03.088.
- C. Guéguen, C. W. Cuss and S. Cho, Snowpack deposition of trace elements in the Athabasca oil sands region, Canada, Chemosphere, 2016, 153, 447–454, DOI:10.1016/j.chemosphere.2016.03.020.
- A. C. Alexander and P. A. Chambers, Assessment of seven Canadian rivers in relation to stages in oil sands industrial development, 1972–2010, Environ. Rev., 2016, 24, 484–494 CrossRef CAS.
- C. A. Cooke, C. Schwindt, M. Davies, W. F. Donahue and E. Azim, Initial environmental impacts of the Obed Mountain coal mine process water spill into the Athabasca River (Alberta, Canada), Sci. Total Environ., 2016, 557–558, 502–509 CrossRef CAS PubMed.
- A. C. Alexander, P. A. Chambers and D. S. Jeffries, Episodic acidification of 5 rivers in Canada's oil sands during snowmelt: a 25-year record, Sci. Total Environ., 2017, 599, 739–749 CrossRef PubMed.
- A. N. Schwalb, A. C. Alexander, A. J. Paul, K. Cottenie and J. B. Rasmussen, Changes in migratory fish communities and their health, hydrology, and water chemistry in rivers of the Athabasca oil sands region: a review of historical and current data, Environ. Rev., 2015, 23(2), 133–150, DOI:10.1139/er-2014-0065.
- C. J. Arens, J. C. Arens, N. S. Hogan, R. J. Kavanagh, F. Berrue, G. J. van der Kraak and M. R. van den Heuvel, Population impacts in White Sucker (Catostomus commersonii) exposed to oil sands-derived contaminants in the Athabasca River, Environ. Toxicol. Chem., 2017, 36, 2058–2067, DOI:10.1002/etc.3735.
- M. Pilote, C. André, P. Turcotte, F. Gagné and C. Gagnon, Metal bioaccumulation and biomarkers of effects in caged mussels exposed in the Athabasca oil sands area, Sci. Total Environ., 2018, 610–611, 377–390, DOI:10.1016/j.scitotenv.2017.08.023.
- C. Boutin and D. J. Carpenter, Assessment of wetland/upland vegetation communities and evaluation of soil-plant contamination by polycyclic aromatic hydrocarbons and trace metals in regions near oil sands mining in Alberta, Sci. Total Environ., 2017, 576, 829–839 CrossRef CAS PubMed.
- C. J. Curtis, R. Flower, N. Rose, J. Shilland, G. L. Simpson, S. Turner, H. D. Yang and S. Pla, Palaeolimnological assessment of lake acidification and environmental change in the Athabasca Oil Sands Region, Alberta, J. Limnol., 2010, 69, 92–104, DOI:10.3274/JL10-69-S1-10.
- K. R. Laird, B. Das, M. Kingsbury, M. T. Moos, S. Pla-Rabes, J. M. E. Ahad, B. Wiltse and B. F. Cumming, Paleolimnological assessment of limnological change in 10 lakes from northwest Saskatchewan downwind of the Athabasca oils sands based on analysis of siliceous algae and trace metals in sediment cores, Hydrobiologia, 2013, 720, 55–73 CrossRef CAS.
- C. A. Cooke, J. L. Kirk, D. C. G. Muir, J. A. Wiklund, X. Wang, A. Gleason and M. S. Evans, Spatial and temporal patterns in trace element deposition to lakes in the Athabasca oil sands region (Alberta, Canada), Environ. Res. Lett., 2017, 12, 26 Search PubMed.
-
E. S. Edgerton, J. M. Fort, K. Baumann, J. R. Graney, M. S. Landis, S. Berryman and S. Krupa, Method for extraction and multielement analysis of Hypogymnia physodes samples from the Athabasca oil sands region, in Developments in Environmental Science, ed. K. E. Percy, Elsevier, Amsterdam, New York, 2012 Search PubMed.
- J. R. Graney, M. S. Landis, K. J. Puckett, W. B. Studabaker, E. S. Edgerton, A. H. Legge and K. E. Percy, Differential accumulation of PAHs, elements, and Pb isotopes by five lichen species from the Athabasca Oil Sands Region in Alberta, Canada, Chemosphere, 2017, 184, 700–710 CrossRef CAS PubMed.
-
D. Hopkins, K. Wall and C. Wilson, Measured Concentrations of Metals and Polycyclic Aromatic Hydrocarbons in Plants, Berries and Soil Located in the Oil Sands Region North of Fort McMurray, Alberta, OSRIN Report No. TR-68, Oil Sands Research and Information Network, University of Alberta, School of Energy and the Environment, Edmonton, Alberta, 2014 Search PubMed.
- C. McAuley, A. Dersch, L. N. Kates, D. R. Sowan and C. A. Ollson, Improving risk assessment calculations for traditional foods through collaborative research with First Nations communities, Risk Anal., 2016, 36, 2195–2207 CrossRef PubMed.
-
S. M. McLachlan and C. H. Riddell, Environmental and Human Health Implications of the Athabasca Oil Sands for the Mikisew Cree First Nation and Athabasca Chipewyan First Nation in Northern Alberta, Environmental Conservation Laboratory, University of Manitoba, 2014 Search PubMed.
- J. Rodríguez-Estival, M. A. North and J. E. G. Smits, Sublethal health effects in laboratory rodents from environmentally relevant exposures to oil sands contaminants, Environ. Toxicol. Chem., 2015, 34(12), 2884–2897 CrossRef PubMed.
- J. Rodríguez-Estival and J. E. G. Smits, Small mammals as sentinels of oil sands related contaminants and health effects in northeastern Alberta, Canada, Ecotoxicol. Environ. Saf., 2016, 124, 285–295 CrossRef PubMed.
- V. M. Goldschmidt, The principles of distribution of chemical elements in minerals and rocks, J. Chem. Soc., 1937, 655–673 RSC.
-
The Encyclopedia of Geochemistry and Environmental Sciences, ed. R. W. Fairbridge, Encyclopedia of Earth Sciences Series, van Nostrand Reinhold Co., New York, 1972, vol. 4a Search PubMed.
- D. Selby and R. A. Creaser, Direct radiometric dating of hydrocarbon deposits using rhenium-osmium isotopes, Science, 2005, 308, 1293–1295 CrossRef CAS PubMed.
- M. W. Donner, B. Bicalho, C. Sinn and W. Shotyk, Distribution of selenium and sulphur in mineral and bitumen fractions of Athabasca Bituminous Sands, Fuel, 2018, 224, 718–725 CrossRef CAS.
- J. R. Moody, NBS Clean Laboratories for Trace Element Analysis, Anal. Chem., 1982, 54(13), 1358 Search PubMed.
- C. F. Boutron, A Clean Laboratory for Ultralow Concentration Heavy-Metal Analysis, Anal. Chem., 1990, 337(5), 482–491 CrossRef CAS.
- J. O. Nriagu, G. Lawson, H. K. T. Wong and J. M. Azcue, A Protocol for Minimizing Contamination in the Analysis of Trace Metals in Great Lakes Waters, Great Lakes Res., 1993, 19(1), 175–182 CrossRef CAS.
- W. Shotyk, B. Bicalho, C. W. Cuss, M. W. Donner, I. Grant-Weaver, M. B. Javed and T. Noernberg, Trace elements in the Athabasca Bituminous Sands: a geochemical explanation for the paucity of environmental contamination by chalcophile elements, Chem. Geol., 2021, 581, 120392, DOI:10.1016/j.chemgeo.2021.120392 ..
- J. Scott, G. A. Collins and G. W. Hodgson, Trace metals in the McMurray oil sands and other Cretaceous reservoirs of Alberta, Trans. Can. Inst. Min. Metall., 1954, 57, 34–40 Search PubMed.
-
B. Hitchon and R. H. Filby, Trace Elements in Alberta Crude Oils, Open File Report, 1983-02, Alberta Research Council, Edmonton, Alberta, Canada, 1983 Search PubMed.
-
H. J. M. Bowen and D. Gibbons, Radioactivation Analysis, Clarendon Press, 1963 Search PubMed.
-
Z. B. Alfassi, Instrumental neutron activation analysis (INAA), ed. Z. B. Alfassi, Determination of Trace Elements, VCH Verlagsgesellschaft GmbH, Weinheim, Germany, 1994, pp. 253–307 Search PubMed.
- H. A. Kaminsky, T. H. Etsell, D. G. Ivey and O. Omotoso, Characterization of heavy minerals in the Athabasca oil sands, Miner. Eng., 2008, 21(4), 264–271 CrossRef CAS.
-
E. P. Bertin, Principles and Practice of X-ray Spectrometric Analyses, Plenum Press, New York, 1970 Search PubMed.
-
I. Z. Pelly, Determination of trace elements by atomic absorption spectrometry, ed. Z. B. Alfassi, Determination of Trace Elements, VCH Verlagsgesellschaft GmbH, Weinheim, Germany, 1994, pp. 145–190 Search PubMed.
- B. Bicalho, I. Grant-Weaver, C. Sinn, S. Woodland, G. Pearson, S. Larter, M. J. M. Duke and W. Shotyk, Determination of trace elements in the mineral and organic fractions of Athabasca Bituminous Sands using inductively coupled plasma sector field mass spectrometry (ICP-SFMS), Fuel, 2017, 206, 248B257 CrossRef.
-
R. L. Rudnick and S. Gao, Composition of the Continental Crust, in Treatise on Geochemistry, ed. H. D. Holland and K. K. Turekian, Elsevier Science, 2nd edn, 2014, pp. 1–51 Search PubMed.
-
J. J. R. Fraústo da Silva and R. J. P. Williams, The Biological Chemistry of the Elements: the Inorganic Chemistry of Life, Oxford University Press, 2nd edn, 2001 Search PubMed.
- S. J. Hawkes, What is a “heavy metal”?, J. Chem. Educ., 1997, 74(11), 1374 CrossRef CAS.
- J. H. Duffus, “Heavy metals” - a meaningless term?, Pure Appl. Chem., 2002, 74(5), 793–807 CrossRef CAS.
- M. E. Hodson, Heavy metals—geochemical bogey men?, Environ. Pollut., 2004, 129, 341–343 CrossRef CAS PubMed.
- P. M. Chapman, Heavy metal—music not science, Environ. Sci. Technol., 2007, 41, 6C CrossRef PubMed.
- P. M. Chapman, “Heavy Metal”—Cacophony, Not Symphony, Integr. Environ. Assess. Manage., 2012, 8, 216 CrossRef PubMed.
- R. Hübner, K. B. Astin and R. J. Herbert, ‘Heavy metal’—time to move on from semantics to pragmatics?, Environ. Monit., 2010, 12(8), 1511–1514 RSC.
- H. Ali and H. Khan, What are heavy metals? Long-standing controversy over the scientific use of the term ‘heavy metals’ – proposal of a comprehensive definition, Toxicol. Environ. Chem., 2018, 100, 6–19 CrossRef CAS.
- O. Pourret, J. C. Bollinger and A. Hursthouse, Heavy metal: a misused term?, Acta Geochim., 2021, 40(3), 466–471 CrossRef CAS.
- E. Nieboer and D. H. S. Richardson, The replacement of the nondescript term ‘heavy metals’ by a biologically and chemically significant classification of metal ions, Environ. Pollut., 1980, 1(1), 3–26 CAS.
-
J. Emsley, The Elements, Clarendon Press, Oxford, UK, 3rd edn, 1998 Search PubMed.
-
A. T. Greaney, Geochemistry of Molybdenum and Other Variably Chalcophile Elements, Ph.D. dissertation, University of California, Santa Barbara, 2018.
-
V. Bouska, The Geochemistry of Coal, Elsevier, Amsterdam, 1981 Search PubMed.
-
V. Valkovic, Trace Elements in Coal, CRC Press, Boca Raton, 1983, 2 volumes Search PubMed.
-
J. M. E. Quirke, Rationalization for the Predominance of Nickel and Vanadium Porphyrins in the Geosphere, in Metal Complexes in Fossil Fuels, ed. R. H. Filby and J. F. Branthaver, American Chemical Society, Washington, DC, USA, 1987, DOI:10.1021/bk-1987-0344.ch004.
- J. J. Kyle, Apuntes sobre la existencia del vanadio en el carbon de piedra de San Rafael, An. Soc. Cient. Argent., 1891, 31, 174–175 Search PubMed.
- V. I. Vernadsky, La matière vivante et la chimie de la mer, Rev. Gen. Sci., Paris, 1924, 35, 46–54 Search PubMed.
- A. Treibs, Chlorophyll- und Hamin-Derivate in Bituminosen Gesteinen, Erdolen, Kohlen, Phosphoriten, Liebigs Ann., 1935, 517, 172–196 CrossRef CAS.
- J. B. F. Champlin and H. N. Dunning, A geochemical investigation of the Athabasca bituminous sands, Econ. Geol., 1960, 55, 797–804 CrossRef.
- R. H. Filby, Origin and nature of trace element species in crude oils, bitumens and kerogens: implications for correlation and other geochemical studies, Geol. Soc., 1994, 78(1), 203–219, DOI:10.1144/GSL.SP.1994.078.01.15.
- K. A. Clark, Athabasca bituminous sands, Fuel, 1951, 30, 49–53 CAS.
-
J. Bichard, Oil Sands Composition and the Research Papers of John A. Bichard of 1957-1965, Alberta Oil Sands Technology & Research Authority (AOSTRA) Technical Publication Series, No. 3, Edmonton, Alberta, 1987, pp. 13–46 Search PubMed.
-
T. R. Paton, The Formation of Soil Material, G. Allen & Unwin, London, Boston, 1978 Search PubMed.
- H. Heinrichs, B. Schulz-Dobrick and K. H. Wedepohl, Terrestrial geochemistry of Cd, Bi, Tl, Pb, Zn and Rb, Geochim. Cosmochim. Acta, 1980, 44, 1519–1533 CrossRef CAS.
- J. O. Nriagu, Global Metal Pollution: Poisoning the Biosphere?, Environment, 1990, 32(7), 7–11 Search PubMed , 28–33..
- J. M. Pacyna and E. G. Pacyna, An assessment of global and regional emissions of trace metals to the atmosphere from anthropogenic sources worldwide, Environ. Rev., 2001, 9(4), 269–298 CrossRef CAS.
- K. T. Whitby, R. E. Charlson, W. E. Wilson, R. K. Stevens and R. E. Lee, The size of suspended particle matter in air, Science, 1974, 183, 1098–1100 CrossRef CAS PubMed.
- K. Willeke and K. T. Whitby, Atmospheric aerosols: size distribution interpretation, J. Air Pollut. Control Assoc., 1975, 25, 529–534 CrossRef.
- D. H. Peirson, P. A. Cawse, L. Salmon and R. S. Cambray, Trace elements in the atmospheric environment, Nature, 1973, 241, 252 CrossRef CAS.
- D. H. Peirson, P. A. Cawse and R. S. Cambray, Chemical uniformity of airborne particulate material, and a maritime effect, Nature, 1974, 251, 675–679 CrossRef CAS.
- R. L. Davison, D. F. S. Natusch, J. R. Wallace and C. A. J. Evans, Trace elements in fly ash: dependence of concentration on particle size, Environ. Sci. Technol., 1974, 8, 1107 CrossRef CAS.
- D. F. S. Natusch, J. R. Wallace and C. A. Evans, Toxic trace elements: preferential concentration in respirable particles, Science, 1974, 183, 203–204 CrossRef PubMed.
-
K. A. Rahn, The Chemical Composition of the Atmospheric Aerosol, Graduate School of Oceanography, University of Rhode Island, Kingston, Rhode Island, USA, 1976 Search PubMed.
-
International Agency for Research on Cancer, Vanadium Pentoxide, 2006, available at http://monographs.iarc.fr/search.php?cx=009987501641899931167%3A2_7lsevqpdm%26cof=FORID%3A9%26ie=UTF-8%26ie=ISO-8859-1%<?pdb_no 26oe?><?pdb_no 26oe?>26oe<?pdb END?><?pdb END?>=ISO-8859-1%<?pdb_no 26sa?><?pdb_no 26sa?>26sa<?pdb END?><?pdb<?db_id PDB?> END?>=%26q=vanadium Search PubMed.
-
Environment Canada and Health Canada, Screening Assessment for the Challenge: Vanadium Oxide, 2010, available at http://www.ec.gc.ca/ese-ees/default.asp?lang=En%26n=62A2DBA9-1 Search PubMed.
- C. R. Miller, P. Biswas and G. D. Leikauf, Combustion Generated Nickel Species Aerosols: Role of Chemical and Physical Properties on Lung Injury, Aerosol Sci. Technol., 2001, 35(4), 829–839 CrossRef CAS.
- S. Sleep, I. J. Laurenzi, J. A. Bergerson and H. L. MacLean, Evaluation of Variability in Greenhouse Gas Intensity of Canadian Oil Sands Surface Mining and Upgrading Operations, Environ. Sci. Technol., 2018, 52, 11941–11951 CAS.
- J. Li, X. Chen, Y. Liu, Q. Xiong, J. Zhao and Y. Fang, Effect of ash composition (Ca, Fe, and Ni) on petroleum coke ash fusibility, Energy Fuels, 2017, 31(7), 6917–6927 CrossRef CAS.
- J. A. Nesbitt and M. B. Lindsay, Vanadium geochemistry of oil sands fluid petroleum coke, Environ. Sci. Technol., 2017, 51(5), 3102–3109 CrossRef CAS PubMed.
- J. A. Nesbitt, M. B. Lindsay and N. Chen, Geochemical characteristics of oil sands fluid petroleum coke, Appl. Geochem., 2017, 76, 148–158 CrossRef CAS.
- W. Shotyk, B. Bicalho, C. W. Cuss, M. Donner, I. Grant-Weaver, S. Haas-Neill, M. Javed, M. Krachler, T. Noernberg, R. Pelletier and C. Zaccone, Trace metals in the dissolved fraction (<0.45 μm) of the lower Athabasca River: analytical challenges and environmental implications, Sci. Total Environ., 2017, 580, 660–669 CrossRef CAS PubMed.
- M. B. Javed, C. W. Cuss and W. Shotyk, Dissolved versus particulate forms of trace elements in the Athabasca River, upstream and downstream of bitumen mines and upgraders, Appl. Geochem., 2020, 122, 104706 CrossRef CAS.
- K. W. Bruland, R. P. Franks, G. A. Knauer and J. H. Martin, Sampling and Analytical Methods for the Determination of Copper, Cadmium, Zinc, and Nickel at the Nanogram Per Liter Level in Sea-Water, Anal. Chim. Acta, 1979, 105(1), 233–245 CrossRef CAS.
- R. Sturgeon, S. S. Berman and K. Kremling, Sampling and Storage of Natural Water for Trace Metals, CRC Crit. Rev. Anal. Chem., 1987, 18(3), 209–244 CrossRef CAS.
- J. M. Tramontano, J. R. Scudlark and T. M. Church, A Method for the Collection, Handling, and Analysis of Trace Metals in Precipitation, Environ. Sci. Technol., 1987, 21(8), 749–753 CrossRef CAS PubMed.
- W. W. Ahlers, M. R. Reid, J. P. Kim and K. A. Hunter, Contamination-Free Sample Collection and Handling Protocols for Trace-Elements in Natural Fresh Waters, Aust. J. Mar. Freshwater Res., 1990, 41(6), 713–720 CrossRef CAS.
- G. Benoit, Clean Technique Measurement of Pb, Ag, and Cd in Freshwater: A Redefinition of Metal Pollution, Environ. Sci. Technol., 1994, 28(11), 1987–1991 CrossRef CAS PubMed.
- R. Rossmann and J. Barres, Trace-Element Concentrations in Near-Surface Waters of the Great-Lakes and Methods of Collection, Storage, and Analysis, Great Lakes Res., 1988, 14(2), 188–204 CrossRef CAS.
- C. L. Creasey and A. R. Flegal, Elemental analyses of goundwater: demonstrated advantage of low-flow sampling and trace-metal clean techniques over standard techniques, Hydrogeol. J., 1999, 7(2), 161–167 CrossRef.
- G. Tölg and R. P. H. Garten, Great fear of small amounts of elements - the significance of analytical chemistry in our modern industrialized community as exemplified by trace element analysis, Angew. Chem., Int. Ed. Engl., 1985, 24, 485–494 CrossRef.
-
M. Ghotbizadeh, Spatial variations of total and dissolved trace elements and their distributions amongst major colloidal forms along and across the Lower Athabasca River, M.Sc. (Eng.), University of Alberta, 2020 Search PubMed.
- M. W. Donner, M. B. Javed, W. Shotyk, K. A. Francesconi and T. Siddique, Arsenic speciation in the lower Athabasca River watershed: a geochemical investigation of the dissolved and particulate phases, Environ. Pollut., 2017, 224, 265–274 CrossRef CAS PubMed.
- M. W. Donner, C. W. Cuss, M. Poesch, R. N. Sinnatamby, T. Siddique and W. Shotyk, Selenium in surface waters of the lower Athabasca River: chemical speciation and implications for aquatic life, Environ. Pollut., 2018, 243, 1343–1351 CrossRef CAS PubMed.
- J. Vesely, S. A. Norton, P. Skrivan, V. Majer, P. Kram, T. Navratil and J. M. Kaste, Environmental chemistry of beryllium, Rev. Mineral. Geochem., 2002, 50(1), 291–317 CrossRef CAS.
- M. B. Javed, C. W. Cuss, I. Grant-Weaver and W. Shotyk, Snowmelt from the Athabasca Bituminous Sands region is an insignificant source of dissolved Pb to the river, Sci. Rep., 2017, 7, 43622 CrossRef PubMed.
- M. B. Javed, C. W. Cuss, J. Zheng, I. Grant-Weaver, T. Noenberg and W. Shotyk, Size-fractionation of trace elements in dusty snow from open pit bitumen mines and upgraders: collection, handling, preparation and analysis of samples from the Athabasca Bituminous Sands region of Alberta, Canada, Environ. Sci.: Atmos., 2022, 2, 428–440 CAS.
- W. Shotyk, P. G. Appleby, B. Bicalho, L. Davies, D. Froese, I. Grant-Weaver, M. Krachler, G. Magnan, G. Mullan-Boudreau, T. Noernberg, R. Pelletier, B. Shannon, S. van Bellen and C. Zaccone, Peat bogs in northern Alberta, Canada reveal decades of declining atmospheric Pb
contamination, Geophys. Res. Lett., 2016, 43, 9964B9974, DOI:10.1002/2016GL070952.
- W. Shotyk, P. G. Appleby, B. Bicalho, L. Davies, D. Froese, I. Grant-Weaver, M. Krachler, G. Magnan, G. Mullan-Boudreau, T. Noernberg, R. Pelletier, B. Shannon, S. van Bellen and C. Zaccone, Peat bogs document decades of declining atmospheric contamination by trace metals in the Athabasca Bituminous Sands region of northern Alberta, Canada, Environ. Sci. Technol., 2017, 51, 6237–6249 CrossRef CAS PubMed.
-
M. M. Savard, J. M. E. Ahad, P. Gammon, A. I. Calderhead, A. Rivera, R. Martel, M. Klebek, J. V. Headley, R. Lefebvre, B. Welsh, A. Smirnoff, H. Pakdel, N. Benoit, S. Liao, J. Jautzy, C. Gagnon, J. Vaive, I. Girard and K. Peru, A Local Test Study Distinguishes Natural from Anthropogenic Groundwater Contaminants near an Athabasca Oil Sands Mining Operation, Geological Survey of Canada, Open File 7195, 2012, DOI:10.4095/292074.
- W. Shotyk and M. Krachler, The isotopic evolution of atmospheric Pb in central Ontario since AD 1800, and its impacts on the soils, waters, and sediments of a forested watershed, Kawagama Lake, Geochim. Cosmochim. Acta, 2010, 74, 1963–1981 CrossRef CAS.
- W. Shotyk, M. Krachler, W. Aeschbach-Hertig, S. Hillier and J. Zheng, Trace elements in recent groundwater of an artesian flow system and comparison with snow: enrichments, depletions, and chemical evolution of the water, J. Environ. Monit., 2010, 12, 208–217 RSC.
- M. Krachler, J. Zheng, D. A. Fisher and W. Shotyk, Direct determination lead isotopes (206Pb, 207Pb, 208Pb) in Arctic ice samples at pg/g levels using ICP-SMS coupled to a high efficiency sample introduction system, Anal. Chem., 2004, 76, 5510–5517 CrossRef CAS PubMed.
- W. Shotyk, J. Zheng, M. Krachler, C. Zdanowicz, R. Koerner and D. Fisher, Predominance of industrial Pb in recent snow (1994–2004) and ice (1842–1996) from Devon Island, Arctic Canada, Geophys. Res. Lett., 2005, 32, L21814, DOI:10.1029/2005GL023860.
- J. Zheng, W. Shotyk, M. Krachler and D. Fisher, 15,800 years of atmospheric lead deposition on Devon Ice Cap, Nunavut, Canada: natural and anthropogenic enrichments, isotopic composition, and predominant sources, Global Biogeochem. Cycles, 2007, 21, GB2027, DOI:10.1029/2006GB002897.
- W. Shotyk, Trace metals in soils of the bituminous sands mining region of Alberta: a critical, geochemical perspective on the study by Boutin and Carpenter (2017) entitled Assessment of wetland/upland vegetation communities and evaluation of soil-plant contamination by polycyclic aromatic hydrocarbons and trace metals in regions near oil sands mining in Alberta, Sci. Total Environ., 2018, 618, 866–869 CrossRef CAS PubMed.
-
H. J. M. Bowen, Environmental Chemistry of the Elements, Academic Press, New York, 1979 Search PubMed.
- M. B. Javed, I. Grant-Weaver and W. Shotyk, Analytical procedure for the determination of trace (mg/kg) and ultratrace (μg/kg) elements in soil and sediments using Inductively Coupled Plasma Quadrupole Mass Spectrometry (ICP-QMS), Can. J. Soil Sci., 2020, 100, 393–407 CrossRef CAS.
- R. N. Sinnatamby, T. N. Loewen, Y. Luo, G. D. Pearson, B. Bicalho, I. Grant-Weaver, C. W. Cuss, M. Poesch and W. Shotyk, A spatial assessment of elemental concentrations from trout-perch (Percopsis omiscomaycus) otoliths from the Lower Athabasca Region, Sci. Total Environ., 2019, 655, 363–373 CrossRef CAS PubMed.
-
R. Allan and T. Jackson, Heavy Metals in Bottom Sediments of the Mainstem Athabasca River system in the AOSERP Study Area, Inland Waters Directorate, Fisheries and Environment Canada, Freshwater Institute, Winnipeg, Manitoba, for Alberta Oil Sands Environmental Research Program, 1978 Search PubMed.
-
J. A. C. Broekaert, Plasma optical emission and mass spectrometry, in Determination of Trace Elements, ed. Z. B. Alfassi, VCH Verlagsgesellschaft GmbH, Weinheim, Germany, 1994. pp. 191–251 Search PubMed.
- P. A. Addison and K. J. Puckett, Deposition of atmospheric pollutants as measured by lichen element content in the Athabasca oil sands area, Can. J. Bot., 1980, 58, 2323–2334 CrossRef CAS.
-
E. S. Edgerton, J. M. Fort, K. Baumann, J. R. Graney, M. S. Landis, S. Berryman and S. Krupa, Method for extraction and multielement analysis of Hypogymnia physodes samples from the Athabasca oil sands region, in Developments in Environmental Science, ed. K. E. Percy, Elsevier, Amsterdam, New York, 2012 Search PubMed.
- W. Shotyk, R. Belland, M. J. Duke, H. Kempter, M. Krachler, T. Noernberg, R. Pelletier, M. A. Vile, K. Wieder, C. Zaccone and S. Zhang,
Sphagnum mosses from twenty-one ombrotrophic bogs in the Athabasca Bituminous Sands region show no significant atmospheric contamination of “heavy metals”, Environ. Sci. Technol., 2014, 48, 12603–12611 CrossRef CAS PubMed.
- W. Shotyk, R. Belland, M. J. Duke, H. Kempter, M. Krachler, T. Noernberg, R. Pelletier, M. A. Vile, K. Wieder, C. Zaccone and S. Zhang, Response to comment by J. Blais and W. Donahue on Sphagnum mosses from twenty-one ombrotrophic bogs in the Athabasca Bituminous Sands region show no significant atmospheric contamination of “heavy metals”, Environ. Sci. Technol., 2015, 49, 6354–6357 CrossRef CAS PubMed.
- W. Shotyk, B. Bicalho, C. Cuss, M. J. Duke, T. Noernberg, R. Pelletier, E. Steinnes and C. Zaccone, Dust is the dominant source of “heavy metals” to peat moss (Sphagnum fuscum) from the industrial development of the Athabasca Bituminous Sands in northern Alberta, Canada, Environ. Int., 2016, 92–93, 494–506 CrossRef CAS PubMed.
-
R. W. Pauls, S. A. Abboud and L. W. Turchenek, Pollutant Deposition Impacts on Lichens, Mosses, Wood and Soil in the Athabasca Oil Sands Area, Internal report prepared for the Environment Division, Syncrude Canada, The Alberta Research Council, 1996 Search PubMed.
-
L. A. Barrie and J. Kovalick, A Wintertime Investigation of the Deposition of Pollutants Around an Isolated Power Plant in northern Alberta, Prepared for the Alberta Oil Sands Environmental Research Program by Atmospheric Environment Service, AOSERP Report No. 90, Edmonton, Alberta, Canada, 1980.
-
W. Murray, The 1981 Snowpack Survey in the AOSERP Study Area, Prepared for the Alberta Oil Sands Environmental Research Program by Promet Environmental Group, Edmonton, Alberta, Canada, 1981 Search PubMed.
-
W. Shelfentook, An Inventory System for Atmospheric Emissions in the the AOSERP Study Area, Alberta Oil Sands Environmental Research Program by SNC TottrupLtd, Edmonton, Alberta, Canada, 1978 Search PubMed.
-
Fiera, State of the Watershed Report - Phase 3: Water Quantity and Basic Water Quality in the Athabasca Watershed, Report (1234) prepared for the Athabasca Watershed Council, Fiera Biological Consulting Ltd, Hinton, Alberta, 2013 Search PubMed.
- C. W. Cuss, I. Grant-Weaver, M. W. Donner, T. Noernberg, R. N. Sinnatamby, R. Pelletier and W. Shotyk, Distribution of trace elements among colloidal species to fingerprint sources in aquatic systems using AF4-ICPMS: tributary inputs to the Athabasca River, Alberta, Canada, Sci. Total Environ., 2018, 642, 1242–1251 CrossRef CAS PubMed.
-
W. Chesworth, Weathering Systems in Weathering, Soils and Palaeosols, ed. I. P. Martini and W. Chesworth, Elsevier, Amsterdam, 1992, pp. 19–40 Search PubMed.
-
U. Förstner and G. Müller, Schwermetalle in Flüssen und Seen als Ausdruck der Umweltverschmutzung, Springer-Verlag, Berlin and Heidelberg, 1974 Search PubMed.
-
W. Salomons and U. Förstner, Metals in the Hydrocycle, Springer, Berlin and Heidelberg, 1984 Search PubMed.
-
U. Förstner and G. T. W. Wittmann, Metal Pollution in the Aquatic Environment, Springer-Verlag, Berlin, New York, 1989 Search PubMed.
-
W. Stumm and J. J. Morgan, Aquatic Chemistry: Chemical Equilibria and Rates in Natural Waters, Wiley, New York, Chichester, 1996 Search PubMed.
- M. Krachler, C. Mohl, H. Emons and W. Shotyk, Atmospheric deposition of V, Cr, and Ni since 12,370 14C yr BP recorded by a Swiss peat bog profile, Environ. Sci. Technol., 2003, 37, 2658–2667 CrossRef CAS PubMed.
- W. Shotyk, D. Weiss, P. G. Appleby, A. K. Cheburkin, R. Frei, M. Gloor, J. D. Kramers, S. Reese and W. O. van der Knaap, History of atmospheric lead deposition since 12,370 14C yr BP recorded in a peat bog profile, Jura Mountains, Switzerland, Science, 1998, 281, 1635–1640 CrossRef CAS PubMed.
- W. Shotyk, M. Krachler, A. Martinez-Cortizas, A. K. Cheburkin and H. Emons, A peat bog record of natural, pre-anthropogenic enrichments of trace elements in atmospheric aerosols since 12,370 14C yr BP, and their variation with Holocene climate change, Earth Planet. Sci. Lett., 2002, 199, 21–37 CrossRef CAS.
- W. Shotyk, M. Krachler and B. Chen, Antimony in recent peat from Switzerland and Scotland: comparison with natural background values (5,320 to 8,020 14C yr BP), correlation with Pb, and implications for the global atmospheric Sb cycle, Global Biogeochem. Cycles, 2004, 18, GB1016, DOI:10.1029/2003GB002113.
- W. Shotyk and M. Krachler, Atmospheric deposition of Ag and Tl since 12,370 14C yr BP recorded by a Swiss peat bog profile, and comparison with Pb and Cd, J. Environ. Monit., 2004, 6, 427–433 RSC.
- W. Shotyk, Natural and anthropogenic sources of copper to organic soils: a global, geochemical perspective, Can. J. Soil Sci., 2020, 100, 516–536 CrossRef.
- G. B. Wiersma, J. B. Wiersma and J. A. Elvir, Tourist use and moss contamination at Torres del Paine National Park, Chile, Adv. Air Pollut., 1990, 13, 465–472 Search PubMed.
- R. Bargagli, J. C. Sanchez-Hernandez, L. Martella and F. Monaci, Mercury, cadmium and lead accumulation in Antarctic mosses growing along nutrient and moisture gradients, Polar Biol., 1998, 19, 316–322 CrossRef.
- W. Shotyk, H. Kempter, M. Krachler and C. Zaccone, Stable (206Pb, 207Pb, 208Pb) and radioactive (210Pb) lead isotopes in one year of growth of Sphagnum moss from four ombrotrophic bogs in southern Germany: geochemical significance and environmental implications, Geochim. Cosmochim. Acta, 2015, 163, 101–125 CrossRef CAS.
-
T. C. Hutchinson and K. M. Meema, Lead, Mercury, Cadmium, and Arsenic in the Environment, John Wiley and Sons, New York, 1987 Search PubMed.
- C. W. Cuss, M. Ghotbizadeh, I. Grant-Weaver, M. B. Javed, T. Noernberg and W. Shotyk, Delayed mixing of iron-laden tributaries in large boreal rivers: implications for iron transport, water quality and monitoring, J. Hydrol., 2021, 597, 125747 CrossRef CAS.
- C. W. Cuss, C. N. Glover, M. B. Javed, A. Nagel and W. Shotyk, Geochemical and biological controls on the ecological relevance of total, dissolved, and colloidal forms of trace elements in large boreal rivers: review and case studies, Environ. Rev., 2020, 28(2), 138–163 CrossRef CAS.
- W. Shotyk, B. Bicalho, I. Grant-Weaver and S. Stachiw, A geochemical perspective on the natural abundance of trace elements in cranberries (Vaccinium oxycoccus) from remote bogs in the Boreal Zone of northern Alberta, Sci. Total Environ., 2019, 650, 1652–1663 CrossRef CAS PubMed.
- P. J. White and P. H. Brown, Plant nutrition for sustainable development and global health, Ann. Bot., 2010, 105(7), 1073–1108 CrossRef CAS PubMed.
- W. Shotyk, T. Noernberg and M. B. Javed, Trace elements in Labrador Tea (Rhododendron groenlandicum): how the predominant sources of trace elements to the plants affect the chemical composition of hot water extracts, Environ. Res., 2020, 183, 109272 CrossRef CAS PubMed.
-
E. R. Gariepy, Phytoremediation of vanadium and nickel from wastewater using Acorus calamus, M.Sc. thesis, University of Alberta, 2020.
- M. La Flèche, C. W. Cuss, T. Noernberg, W. Shotyk and J. Karst, Trace metals as indicators of tree rooting behaviour in bituminous soils, Land Degrad. Dev., 2021, 35, 1970–1980 CrossRef.
- W. Shotyk, B. Bicalho, C. W. Cuss, I. Grant-Weaver, T. Noernberg, M. Poesch and N. R. Sinnatamby, Bioaccumulation of Tl in otoliths of Trout-perch (Percopsis omiscomaycus) from the Athabasca River, upstream and downstream of bitumen mining and upgrading, Sci. Total Environ., 2019, 650, 2559–2566 CrossRef CAS PubMed.
- W. Shotyk, B. Bicalho, M. Dergousoff, I. Grant-Weaver, G. Hood, K. Lund and T. Noernberg, A geochemical perspective on the natural abundance trace elements in liver and kidney of beaver (Castor canadensis) from an agricultural region of southern Ontario, Canada, Sci. Total Environ., 2019, 672, 40–50 CrossRef CAS PubMed.
- Oil Sands Magazine official webpage,
Bitumen production from mining in Alberta, since 1968, Data obtained on November 16, 2021 and used with permission, available at https://www.oilsandsmagazine.com/.
- L. J. Davies, D. G. Froese, P. G. Appleby, S. van Bellen, B. Jensen, G. Magnan, G. Mullan-Boudreau, T. Noernberg, W. Shotyk and C. Zaccone, High-resolution age modelling of ombrotrophic peat bog profiles from northern Alberta, Canada, using pre- and post-bomb 14C, 210Pb and historical tephra, Quat. Geochronol., 2018, 47, 138–162 CrossRef.
- M. W. Donner, B. Bicalho, T. Noernberg and W. Shotyk, Contemporary and historical atmospheric deposition of arsenic and selenium in the Athabasca Bituminous Sands Region, Environ. Sci. Technol., 2019, 53, 14020–14028 CrossRef CAS PubMed.
- J. A. Wiklund, R. I. Hall, B. B. Wolfe, T. W. D. Edwards, A. J. Farwell and D. G. Dixon, Has Alberta oil sands development increased far-field delivery of airborne contaminants to the Peace-Athabasca Delta?, Sci. Total Environ., 2012, 433, 379–382 CrossRef CAS PubMed.
- J. A. Wiklund, R. I. Hall, B. B. Wolfe, T. W. D. Edwards, A. J. Farwell and D. George Dixon, Use of pre-industrial floodplain lake sediments to establish baseline river metal concentrations downstream of Alberta oil sands: a new approach for detecting pollution of rivers, Environ. Res. Lett., 2014, 9(12), 124019 CrossRef.
-
J. G. Watson, J. C. Chow, X. Wang, S. D. Kohl and L. N. R. Yatavelli, Windblown Fugitive Dust Characterization in the Athabasca Oil Sands Region, WBEA-DRI Agreement Number: T108-13, Fort McMurray, 2014 Search PubMed.
- X. Wang, J. C. Chow, S. D. Kohl, L. N. R. Yatavelli, K. E. Percy, A. H. Legge and J. G. Watson, Wind erosion potential for fugitive dust sources in the Athabasca oil sands region, Aeolian Res., 2015, 18, 121–134 CrossRef.
- G. Mullan-Boudreau, K. Devito, T. Noernberg, R. Pelletier and W. Shotyk,
Sphagnum moss as an indicator of contemporary rates of atmospheric dust deposition in the Athabasca Bituminous Sands region, Environ. Sci. Technol., 2017, 51, 7422–7431 CrossRef CAS PubMed.
- S. Stachiw, B. Bicalho, I. Grant-Weaver, T. Noernberg and W. Shotyk, Trace elements in berries collected near upgraders and open pit mines in the Athabasca Bituminous Sands Region (ABSR): distinguishing atmospheric dust deposition from plant uptake, Sci. Total Environ., 2019, 670, 849–864 CrossRef CAS PubMed.
- W. Shotyk, Trace elements in wild berries from reclaimed lands: biomonitors of contamination by atmospheric dust, Ecol. Indic., 2020, 110, 105960 CrossRef CAS.
- G. Mullan-Boudreau, L. Davies, D. Froese, K. Devito, T. Noernberg, R. Pelletier and W. Shotyk, Peat cores from ombrotrophic (rain-fed) bogs for reconstructing past rates of atmospheric dust deposition in the Athabasca Bituminous Sands region of northern Alberta, Canada, Land Degrad. Dev., 2017, 28, 2468–2481 CrossRef.
- M. B. Javed and W. Shotyk, Estimating bioaccessibility of trace metals in particles suspended in the Athabasca River using sequential extraction, Environ. Pollut., 2018, 240, 466–474 CrossRef CAS PubMed.
-
A. F. White, Chemical Weathering of Silicate Minerals in Soils in Chemical Weathering of the Silicate Minerals, Reviews in Mineralogy, ed. A. F. White and S. L. Brantley, Mineralogical Society of America, Chantilly, VA, USA, 1995, vol. 31, pp. 407–462 Search PubMed.
- W. Shotyk and J. B. Metson, The application of Secondary Ion Mass Spectrometry (SIMS) to studies of mineral dissolution in soils and sediments, Rev. Geophys., 1995, 32, 197–220 CrossRef.
- W. A. Brungs, J. R. Geckler and M. Gast, Acute and chronic toxicity of copper to the fathead minnow in a surface water of variable quality, Water Res., 1974, 10, 37–43 CrossRef.
- M. Jensen-Fontaine, W. P. Norwood, M. Brown, D. G. Dixon and X. C. Le, Uptake and speciation of vanadium in the benthic invertebrate Hyalella azteca, Environ. Sci. Technol., 2014, 48(1), 731–738, DOI:10.1021/es403252k.
-
U.S. EPA, Water Quality Criteria Data Book, Volume 2. Inorganic Chemical Pollution of Freshwater, Water Pollution Control Research Series, 18010, DPV 07/71, U.S. Environmental Protection Agency, Washington, DC, 1971 Search PubMed.
-
D. S. Jeffries, Ontario precipitation chemistry and heavy metal speciation, Ph.D. dissertation, McMaster University, Hamilton, Ontario, 1975.
- N. J. Yang and M. J. Hinner, Getting across the cell membrane: an overview for small molecules, peptides, and proteins, Methods Mol. Biol., 2015, 1233, 29–53 CrossRef PubMed.
- W. G. Sunda, D. W. Engel and R. M. Thuotte, Effect of chemical speciation on toxicity of cadmium to grass shrimp, Palaemonetes pugio: importance of free cadmium ion, Environ. Sci. Technol., 1978, 12(4), 409–413, DOI:10.1021/es60140a003.
- F. T. Bingham, G. Sposito and J. E. Strong, The effect of sulphate on the availability of cadmium, Soil Sci., 1986, 141, 172–177 CrossRef CAS.
- T. M. Florence and G. E. Batley, Chemical speciation in natural waters, CRC Crit. Rev. Anal. Chem., 1980, 9, 219–296 CAS.
- F. H. Frimmel and T. Gremm, The importance of element speciation in water analysis - a plea for further investigations, Fresenius. J. Anal. Chem., 1994, 350, 7–13, DOI:10.1007/BF00326244.
- C. W. Cuss, I. Grant-Weaver and W. Shotyk, AF4-ICPMS with the 300 Dalton membrane to resolve metal-bearing ‘colloids’ < 1 kDa: optimization, fractogram deconvolution, and advanced quality control, Anal. Chem., 2017, 89, 8027–8035 CrossRef CAS PubMed.
- L. J. Ehlers and R. G. Luthy, Contaminant bioavailability in improving risk assessment and remediation rests on better understanding bioavailability, Environ. Sci. Technol., 2003, 37, 295–302 CrossRef PubMed.
- K. T. Semple, K. J. Doick, K. C. Jones, P. Burauel, A. Craven and H. Harms, Defining bioavailability and bioaccessibility of contaminated soil and sediment is complicated, Environ. Sci. Technol., 2004, 38(12), 228A–231A CrossRef CAS PubMed.
- R. J. Reeder, M. A. A. Schoonen and A. Lanzirotti, Metal speciation and its role in bioaccessibility and bioavailability, Rev. Mineral. Geochem., 2006, 64, 59–113 CrossRef CAS.
Footnotes |
† Electronic supplementary information (ESI) available. See https://doi.org/10.1039/d2em00049k |
‡ The term “heavy metal” has criticized for many reasons and generated considerable confusion.42–49 Although there have been efforts to replace it with more precise descriptions,50 the term is still popular. Given that Be is a “light metal”, As and Sb are metalloids (or semi-metals) and Se is a non-metal,51 we prefer to simply use the term “trace element”. |
§ This brings to mind the quotation by Jonathan Swift (1667–1775): “falsehood flies, and truth comes limping after it, so that when men come to be undeceived, it is too late; the jest is over, and the tale hath had its effect: like a man, who hath thought of a good repartee when the discourse is changed, or the company parted; or like a physician, who hath found out an infallible medicine, after the patient is dead.” |
|
This journal is © The Royal Society of Chemistry 2022 |
Click here to see how this site uses Cookies. View our privacy policy here.