DOI:
10.1039/C6QO00244G
(Review Article)
Org. Chem. Front., 2016,
3, 1205-1212
Synthesis and reactivity of alkoxy-activated cyclobutane-1,1-dicarboxylates
Received
3rd June 2016
, Accepted 27th July 2016
First published on 27th July 2016
Abstract
Intermolecular cycloaddition chemistry is unarguably one of the most appreciated strategies to bring complexity to products from simple starting materials. Generation of dipolar intermediates by exploitation of ring strain in carbocycles has become an efficient option. In this regard, donor–acceptor cyclopropanes have been extensively studied, but reports on extending similar synthetic transformations to the homologous cyclobutanes are comparatively limited. Recently, our group has become interested in application of alkoxy-activated cyclobutane-1,1-dicarboxylates (AACDs) in cycloaddition chemistry. This personal account discusses our contributions in this area with contextual examples from others.
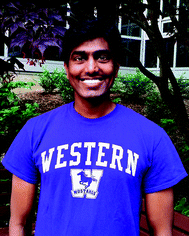 Naresh Vemula | Naresh Vemula was born in Telangana, India. He received his Bachelor's degree from Osmania University (2004), and Master's degree from Kakatiya University (2007). He then spent three years at Dr. Reddy's Laboratories Ltd as a Process Chemist (July 2007–April 2010), and another year at DuPont Crop Protection as a Discovery Chemist (May 2010–July 2011). He then moved to Canada to pursue his Ph.D. under the supervision of Prof. Brian L. Pagenkopf at The University of Western Ontario, focusing on exploring the reactivity of donor–acceptor cyclobutanes. |
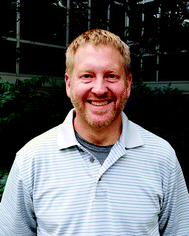 Brian L. Pagenkopf | Brian Pagenkopf was born in Wausau, Wisconsin. He earned his BS degree from the University of Minnesota, Twin Cities, in 1991, and his Ph.D. from Montana State University, Bozeman, in 1996 under the supervision of Prof. Tom Livinghouse. He was a NIH postdoctoral fellow at Caltech and the ETH in Zürich under the supervision of Prof. Erick M. Carreira. He started his independent career at the University of Texas at Austin in 1999, and then in 2005 he moved to Canada, where he is a full professor at The University of Western Ontario. His research interests include methods development, organic materials for electronic devices and natural product synthesis. |
1. Introduction
Exploitation of ring strain to generate dipolar intermediates for cycloaddition reactions is a valuable strategy in modern organic synthesis. One of the most studied motifs is that of cyclopropanes, typically bearing vicinally substituted electron donating and electron accepting groups, generally termed as donor–acceptor (DA) cyclopropanes.1 This substitution pattern polarizes the C–C bond, forming (formal) 1,3-zwitterionic intermediates which undergo facile cycloadditions with suitable dipolarophiles, typically under the influence of a Lewis acid2 (eqn (1), Scheme 1).3 While this mode of reactivity has been extensively studied, reports extending similar synthetic transformations to the homologous cyclobutanes are comparatively limited (eqn (2), Scheme 1)4 despite having similar ring strain.5
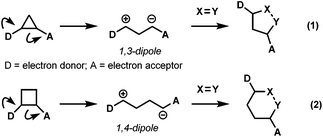 |
| Scheme 1 Reactivity of DA cyclopropanes and cyclobutanes with generic dipolarophile X = Y. | |
It was not until 1991, when the first report of formal cycloaddition of DA cyclobutanes was disclosed by Saigo and co-workers.6 In this work, amino-activated DA cyclobutanes 1 underwent a [4 + 2] cycloaddition with carbonyl compounds 2 to generate tetrahydropyrans 3 albeit with low diastereoselectivity and modest yield (Scheme 2).
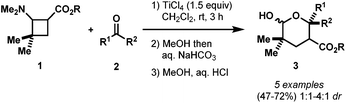 |
| Scheme 2 Cycloaddition of amino-activated DA cyclobutanes with carbonyl compounds. | |
A few years later, Suzuki and co-workers observed a [4 + 2] cycloaddition of highly activated DA cyclobutane 4 with 2-oxazoline 5 without the need for a catalyst (Scheme 3).7
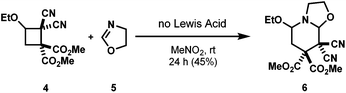 |
| Scheme 3 Cycloaddition of DA cyclobutane 4 with 2-oxazoline 5. | |
The field remained relatively dormant for a decade, and in 2008, Matsuo and co-workers observed a conceptually similar8 [4 + 2] cycloaddition with 3-alkoxycyclobutanones 7 and carbonyl compounds 2 (Scheme 4).9
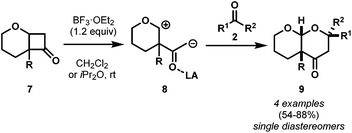 |
| Scheme 4 The [4 + 2] cycloaddition 3-alkoxycyclobutanones 7 and carbonyl compounds 2. | |
The first catalytic cycloaddition of DA cyclobutanes was reported by the research groups of Johnson and of Christie and Pritchard almost at the same time (Scheme 5). The [4 + 2] cycloaddition with aldehydes 11 were performed under mild conditions with good to excellent yields. Contrary to the work conducted by the Saigo group, these reports used carbon based electron-donor groups and 1,1-diester functionality as an electron-acceptor. Johnson found Sc(OTf)3 was able to catalyse the cycloaddition with loadings as low as 2 mol% (eqn (1), Scheme 5).10 The cycloaddition was highly diastereoselective for 2,6-cis-diastereomer with most of the aryl aldehydes investigated, but when cinnamaldehyde was used the diastereoselectivity dropped to 77
:
23, possibly due to the slow reactivity.11 The group was able to encompass aliphatic aldehydes with the more reactive and bulky Lewis acid, MADNTf2.12
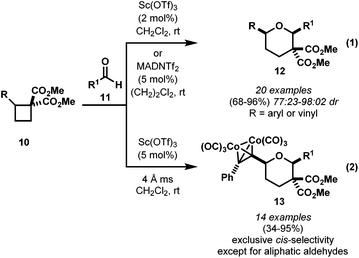 |
| Scheme 5 The [4 + 2] cycloaddition of carbon-activated cyclobutane-1,1-dicarboxylates 10 with aldehydes 11. | |
The work by Christie and Pritchard reported a similar reactivity of cyclobutanes with a cobalt–alkyne complex as an electron-donor and 1,1-diesters as electron-acceptors (eqn (2), Scheme 5).13 The group also found Sc(OTf)3 as the best catalyst for this transformation. Most of the aryl aldehydes and other electron-rich aldehydes reacted in good to excellent yields as single diastereomers. When aliphatic aldehydes were used, the diastereoselectivity significantly dropped to 20–23% de.
It became apparent from the above results that having 1,1-diesters as electron-acceptors as compared to a monoester enhances the reactivity of the DA cyclobutane as well as diastereoselectivity in cycloadditions. Inspired by these seminal reports and our ongoing interest in alkoxy-activated cyclopropane chemistry,14 we were motivated to investigate the reactivity alkoxy-activated cyclobutane-1,1-dicarboxylates (AACDs).
2. Synthesis of alkoxy-activated cyclobutane dicarboxylates
At the outset of our work, there were two literature methods available for the synthesis of AACDs.15 The use of a Michael induced ring closure of acyclic substrates (eqn (1), Scheme 6) was not selected as a preparative route as it offers limited control over the stereochemistry, and requires multiple steps.16 On the other hand, the ZnBr2-promoted [2 + 2] annulation reported by Roberts in 1986 appeared much more promising since the required alkyl enol ethers are commercially available, and the methylidene malonates can easily be made by a Knoevenagel condensation (eqn (2), Scheme 6).17 Disappointingly, duplication of the conditions reported by Roberts in our hands gave poor yields (insufficient for further study) with a complex mixture of polymerization and/or ring-opened by-products. More disappointingly, however, we couldn't extend this methodology to more reactive diethyl or dimethyl methylidene malonates.17,18 Thus, a new catalyst screening was warranted, and we found Yb(OTf)3 as the best catalyst for this [2 + 2] cycloaddition.19 With the optimized conditions in hand, the scope of the cyclobutane synthesis was explored (Table 1).20
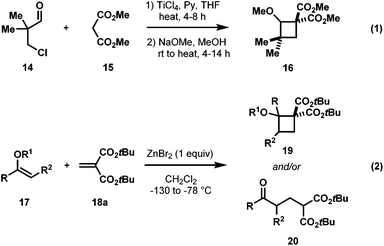 |
| Scheme 6 Literature methods for the synthesis of AACDs. | |
Table 1 The synthesis of AACDs
A range of cyclic and acyclic enol ethers were found to undergo cycloaddition with a variety of dialkyl methylidene malonates in good to excellent yields to afford AACDs as single diastereomers (22a–22g, Table 1). In addition to enol ethers, electron-rich styrenes were also found to undergo efficient cycloaddition to yield AACDs in good yields (22h–22k, Table 1).
3. Reactivity of alkoxy-activated cyclobutane dicarboxylates
With the AACDs at hand, the reactivity was explored with aldehydes 11, which were previously reported to undergo [4 + 2] cycloadditions with DA cyclobutanes 1 and 10 (see Schemes 2 and 5).
3.1. The [4 + 2] cycloaddition of AACDs with aldehydes
Interestingly, initial investigations established that Yb(OTf)3, which was used for the synthesis of AACDs (Table 1), can also be used for the [4 + 2] cycloaddition with aldehydes. A wide range of aldehydes were found to undergo cycloadditions with AACDs in good to excellent yields as single diastereomers (Table 2).21 Aryl, heteroaryl, vinyl, and alkynyl aldehydes underwent smooth cycloadditions to afford tetrahydropyrans 23 in good to excellent yields (23a–23j, Table 2). Finally, aliphatic aldehydes were also found to engage in cycloadditions without the need for another catalyst, but only in modest yields (23k–23m).
Table 2 The [4 + 2] cycloaddition of AACDs with aldehydes
3.2. The [4 + 2] cycloaddition of AACDs with imines
With the successful cycloaddition of AACDs with aldehydes 11, we were then interested to explore other possible dipolarophiles. Although, imines 24 were excellent dipolarophiles in cycloadditions with DA cyclopropanes,22 their reactivity with DA cyclobutanes was unexplored. Thus, we set out to investigate the reactivity of imines 24 with AACDs.20 Pleasingly, upon exposure of cyclobutane 22 and imine 24 (prepared in situ) to catalytic Yb(OTf)3 at −50 °C, a mixture of bicyclic piperidine 25 and piperideine 26 were formed (Table 3). In order to converge on the piperideine product 26, the reaction was simply warmed to room temperature after the cyclobutane was consumed.23 Aryl ether activated cyclobutanes were also found to undergo cycloaddition to afford exclusive 2,6-trans-piperidines, but longer reaction times were necessary (25a–25d, Table 3).
Table 3 The [4 + 2] cycloaddition of AACDs with imines
3.3. The [4 + 3] cycloaddition of AACDs with nitrones
Having successfully expanding the reactivity of AACDs to imines 24, our interest turned into exploring 3-atom dipolarophiles. Nitrones 27 proved to be excellent dipolarophiles in cycloadditions with DA cyclopropanes.24 Given this precedent, we investigated the reactivity of nitrones with AACDs under previously successful Yb(OTf)3 catalysis.
A quick optimization study established 5 mol% Yb(OTf)3 in dichloromethane as the best conditions for this cycloaddition.25 Interestingly, cis-diastereomers were formed as thermodynamic products when the reaction was performed at room temperature, but efforts to isolate the kinetic trans-diastereomer at lower temperatures gave diastereomeric mixtures (Table 4).26 Additionally, when electron-deficient nitrone 27d was used, an inseparable third diastereomer was formed (entry 4).27 The heterocycle 1,2-oxazepane, though not naturally occurring, displays interesting antiviral28 and antiproliferative29 activity.
Table 4 The [4 + 3] cycloaddition of AACDs with nitrones
3.4. BF3·OEt2-promoted reaction of AACDs with terminal alkynes
Intrigued with the success of above discussed cycloadditions, we were then interested to study an all carbon dipolarophile, such as a terminal alkyne. Terminal alkynes 29 were reported to undergo efficient [3 + 2] cycloadditions with DA cyclopropanes.30 After numerous unsuccessful attempts under a variety of conditions, we found stoichiometric BF3·OEt2 was able to promote this reaction. Interestingly, instead of the expected cycloadduct, the reaction resulted in 2,3-dihydrooxepine 30 through an addition/rearrangement sequence via a highly strained bicyclic intermediate 33 (Scheme 7).31 Only phenylacetylenes with electron-neutral and moderately electron-rich substituents proceeded through a productive reaction manifold, albeit in low yields (30a–30e, Scheme 7). Substrates with strong electron-donating substituents rapidly polymerized upon exposure to BF3·OEt2, whereas, electron-deficient alkynes failed to react.32
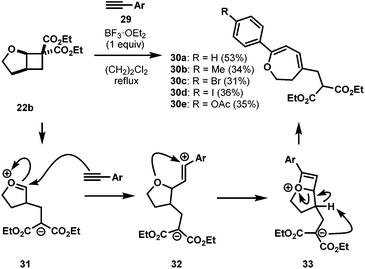 |
| Scheme 7 BF3·OEt2-promoted reaction of AACDs with terminal alkynes. | |
Interestingly, when silyloxy substituted phenylacetylene 29f was used, the reaction resulted in [4 + 2] cycloadduct 34 (Scheme 8). This was the only case we observed cycloaddition instead of rearrangement, which could be due to the increased bulk on the aryl ring inhibiting the polymerization and/or rearrangement.
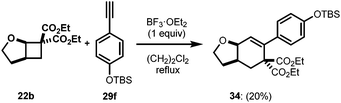 |
| Scheme 8 BF3·OEt2-promoted [4 + 2] cycloaddition of alkyne 29f with AACD 22b. | |
3.5. The [4 + 2] cycloaddition of AACDs with nitrosoarenes
Motivated with the successful cycloadditions of AACDs with aldehydes, imines, and nitrones, we were then interested in exploring all heteroatom dipolarophiles, such as nitroso compounds. Nitroso compounds have been utilized in various transformations: as dienophiles in nitroso Diels–Alder reactions,33 as enophiles in nitroso–ene reactions,34 and as either nitrogen or oxygen transfer reagents in nitroso–aldol reactions.35 But surprisingly, at the outset of this work, nitrosoarenes 35 have not seen application in cycloaddition chemistry with either DA cyclopropanes36 or cyclobutanes.37
Gratifyingly, Yb(OTf)3 was able to catalyse the reaction, with catalysts loadings as low as 0.5 mol%, but 2 mol% was chosen for experimental convenience.38 Nitrosoarenes with electron-neutral substituents were found to be excellent reaction partners (entries 1 and 2, Table 5). Substrates with a moderately deactivating ester substituent resulted in good yield, but the regioselectivity dropped to 13
:
1 (entry 3). The nitrosoarenes with strong electron-withdrawing groups afforded moderate yields; however, the regioselectivity decreased significantly to 3
:
1 (entry 4). Nitrosoarene with weakly electron donating methyl substituent resulted in a poor 29% yield (entry 5). Upon incorporation of a strong electron donating group no reaction was observed, likely due to the sequestration of the ytterbium catalyst by the electron-rich nitrosoarene 35f (entry 6).
Table 5 The [4 + 2] cycloaddition of AACDs with nitrosoarenes
In an attempt to extend the reaction scope to encompass electron-rich substrates, we screened several Lewis acids under a variety of conditions, and it was found that MgI2 was the best, albeit in low yield (Table 6).39 Interestingly the regioisomer isolated under these conditions was acetal 37 and not the aminal 36 as expected, which could be due to the enhanced nucleophilicity of the nitroso oxygen from methoxy substituent (Scheme 9). More interestingly, when the reaction was left to stir for two days at room temperature or when 37 was treated with 50 mol% MgI2 at room temperature overnight, pyrrolidine 38 was formed (entry 2, Table 6) plausibly via MgI2-promoted deoxygenation process of acetal product as shown in Scheme 9.40 The nitroso-heteroarenes 35h and 35i participated in the cycloaddition, also in low yields (entries 5 and 6).
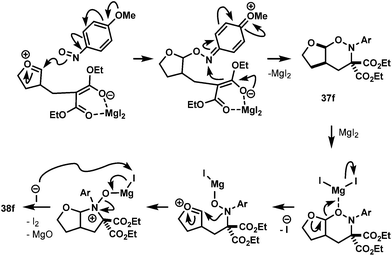 |
| Scheme 9 Plausible mechanism for the formation of 37f and 38f. | |
Table 6 The MgI2 promoted cycloaddition of AACDs with nitrosoarenes
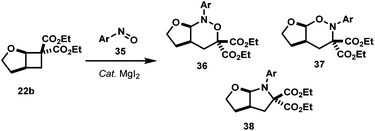
|
Entry |
Nitrosoarene |
Product |
MgI2 (mol%) |
Yield (%) |
1 |
35f: Ar = 4-C6H4OMe |
37f
|
10 |
20 |
2 |
35f: Ar = 4-C6H4OMe |
38f
|
50 |
26 |
2 |
35g: Ar = 4-C6H4N(CH3)2 |
38g
|
50 |
22 |
5 |
35h: Ar = 2-pyridine |
37h
|
50 |
28 |
6 |
35i: Ar = N-BOC-5-nitrosoindole |
37i
|
10 |
19 |
4. Additional cyclobutane-1,1-dicarboxylates
In recent years, several others reported interesting cycloadditions of DA cyclobutanes. Most recently, Tang and co-workers reported an enantioselective [4 + 3] cycloaddition of DA cyclobutanes and nitrones (Scheme 10).41 While contributing the first enantioselective variant, the group also added several new DA cyclobutanes to the library.
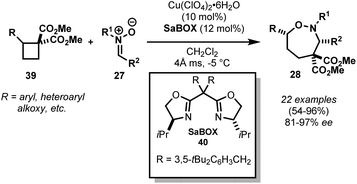 |
| Scheme 10 Enantioselective [4 + 3] cycloaddition of DA cyclobutanes 39 with nitrones 27. | |
Waser and co-workers enhanced the family of DA cyclobutanes by developing an Fe(III)-catalyzed [2 + 2] cycloaddition of enimides 41 and alkylidene malonates 21 to access amino-activated cyclobutane-1,1-dicarboxylates 42 (Scheme 11).42
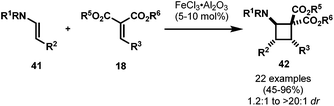 |
| Scheme 11 Synthesis of amino-activated cyclobutane-1,1-dicarboxylates via a [2 + 2] cycloaddition. | |
The group also disclosed the reactivity of these DA cyclobutanes with aldehydes and silyl enolethers (Scheme 12).43 Interestingly, in the reaction with aldehydes 11 (eqn (1), Scheme 12), the less substituted DA cyclobutanes 42 (R2 = R3 = H) were able to activated with Sc(OTf)3, but the more substituted 42 (R2 = R3 ≠ H) required FeCl3·Al2O3. More interestingly, thymine- or fluorouracil-substituted cyclobutanes were also found to undergo cycloaddition with aldehydes under Hf(OTf)4 catalysis to access six-membered ring carbonucleoside analogues. In reaction with silyl enolethers, only less substituted DA cyclobutanes 42 (R2 = R3 = H) were found to undergo cycloadditions (eqn (2), Scheme 12).
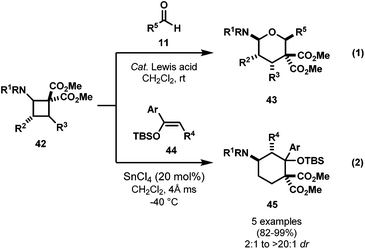 |
| Scheme 12 [4 + 2] cycloadditions of amino-activated cyclobutane-1,1-dicarboxylates. | |
The first intramolecular cycloaddition of DA cyclobutanes was recently reported by France and co-workers.44,45 The authors described a Sc(OTf)3-catalyzed [5 + 2] cycloaddition approach for the synthesis of azepino[1,2-a]indoles 50via DA cyclobutane intermediates (Scheme 13).
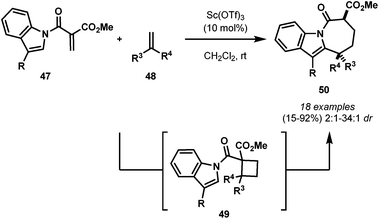 |
| Scheme 13 The [5 + 2] cycloaddition approach for the synthesis of azepino[1,2-a]indoles via DA cyclobutane intermediates. | |
5. Conclusions
In summary, application of the long ignored DA cyclobutanes in cycloaddition chemistry has recently garnered significant attention and a number of reaction partners have been found to undergo efficient annulations to facilitate rapid access to structurally intriguing carbo- and heterocyclic frameworks. Except in few instances, the cycloadditions have a broad substrate scope and displays high level of stereo control. Recently, asymmetric and intramolecular cycloaddition variants were reported, yet the chemistry of DA cyclobutanes is only in its infancy and further studies will surely prove fruitful. Mechanistic insights into unexpected formation of 2,3-dihydrooxepines and pyrrolidines in cycloadditions with terminal alkynes and nitrosoarenes, respectively, has not yet been studied. Revelation of the mechanism will surely bring about new and exciting opportunities for this field of chemistry. In addition, elaboration of these cycloaddition adducts remains to be exploited for the synthesis of complex natural products. We hope this account serves as a guide to the reactivity of DA cyclobutanes to the interested readers.
Acknowledgements
We thank the University of Western Ontario and the Natural Sciences and Engineering Research Council of Canada for financial support. We also thank past and present co-workers cited herein, especially Mahmoud M. Abd Rabo Moustafa (Ph.D. 2011, UWO) for their hard work and dedication.
Notes and references
- The term donor–acceptor substituted cyclopropane was introduced in 1980: H.-U. Reissig and E. Hirsch, Angew. Chem., Int. Ed. Engl., 1980, 19, 813 CrossRef.
- Although, Lewis acids were widely used for activation, there are variety ways to activate the DA cyclopropane. For example, see: B. M. Trost, P. J. Morris and S. J. Sprague, J. Am. Chem. Soc., 2012, 134, 17823 CrossRef CAS PubMed.
- For a review on chemistry of DA cyclopropanes, see:
(a) H.-U. Reissig, Top. Curr. Chem., 1988, 144, 73 CrossRef CAS;
(b) H.-U. Reissig and R. Zimmer, Chem. Rev., 2003, 103, 1151 CrossRef CAS PubMed;
(c) M. Yu and B. L. Pagenkopf, Tetrahedron, 2005, 61, 321 CrossRef CAS;
(d) D. Agrawal and V. K. Yadav, Chem. Commun., 2008, 6471 RSC;
(e) C. A. Carson and M. A. Kerr, Chem. Soc. Rev., 2009, 38, 3051 RSC;
(f) F. De Simone and J. Waser, Synthesis, 2009, 3353 CAS;
(g) M. J. Campbell, J. S. Johnson, A. T. Parsons, P. D. Pohlhaus and S. D. Sanders, J. Org. Chem., 2010, 75, 6317 CrossRef CAS PubMed;
(h) T. P. Lebold and M. A. Kerr, Pure Appl. Chem., 2010, 82, 1797 CrossRef CAS;
(i) J. Kaschel and D. B. Werz, Nachr. Chem., 2011, 59, 729 CrossRef CAS;
(j) M. Y. Mel'nikov, E. M. Budynina, O. A. Ivanova and I. V. Trushkov, Mendeleev Commun., 2011, 21, 293 CrossRef;
(k) Z. Wang, Synlett, 2012, 2311 CrossRef CAS;
(l) M. A. Cavitt, L. H. Phun and S. France, Chem. Soc. Rev., 2014, 43, 804 RSC;
(m) T. F. Schneider, J. Kaschel and D. B. Werz, Angew. Chem., Int. Ed., 2014, 53, 5504 CrossRef CAS PubMed;
(n) F. de Nanteuil, F. De Simone, R. Frei, F. Benfatti, E. Serrano and J. Waser, Chem. Commun., 2014, 50, 10912 RSC;
(o)
S. D. R. Christie and H. T. A. Watson, Cycloaddition Reactions of Small Rings, in Methods and Applications of Cycloaddition Reactions in Organic Syntheses, ed. N. Nishiwaki, John Wiley & Sons, Inc., Hoboken, New Jersey, 2014, p. 241 Search PubMed;
(p) R. A. Novikov and Yu. V. Tomilov, Mendeleev Commun., 2015, 25, 1 CrossRef CAS;
(q) H. K. Grover, M. R. Emmett and M. A. Kerr, Org. Biomol. Chem., 2015, 13, 655 RSC.
- For a brief review on chemistry of DA cyclobutanes, see:
(a) T. Seiser, T. Saget, D. N. Tran and N. Cramer, Angew. Chem., Int. Ed., 2011, 50, 7740 CrossRef CAS PubMed;
(b) J. I. Matsuo, Tetrahedron Lett., 2014, 55, 2589 CrossRef CAS;
(c) F. de Nanteuil, F. De Simone, R. Frei, F. Benfatt, E. Serrano and J. Waser, Chem. Commun., 2014, 50, 10912 RSC;
(d) H.-U. Reissig and R. Zimmer, Angew. Chem., Int. Ed., 2015, 54, 5009 CrossRef CAS PubMed;
(e) L. Wang and Y. Tang, Isr. J. Chem., 2016, 56, 463 CrossRef CAS.
-
K. B. Wiberg, in The Chemistry of Cyclobutanes, ed. Z. Rappoport and J. F. Liebman, John Wiley & Sons Ltd., Chichester, England, 2005, Part I, pp. 4–5 Search PubMed.
- S. Shimada, K. Saigo, H. Nakamura and M. Hasegawa, Chem. Lett., 1991, 20, 1149 CrossRef.
- T. Yokozawa, M. Tagami, T. Takehana and T. Suzuki, Tetrahedron, 1997, 53, 15603 CrossRef CAS.
- Although 3-alkoxycyclobutanones are not formally defined as DA cyclobutanes (see footnote 25 in ref. 44), they do undergo annulation reactions
through a 1,4-zwiterionic intermediate similar to DA cyclobutanes. See ref. 4b for details.
- J.-i. Matsuo, S. Sasaki, H. Tanaka and H. Ishibashi, J. Am. Chem. Soc., 2008, 130, 11600 CrossRef CAS PubMed.
- A. T. Parsons and J. S. Johnson, J. Am. Chem. Soc., 2009, 131, 14202 CrossRef CAS PubMed.
- More strong Lewis acid, Hf(OTf)4 was used for this example.
- Methylaluminum bis(2,6-di-tert-butyl-4-methylphenoxide) trifluoromethanesulfonimide.
- E. A. Allart, S. D. R. Christie, G. J. Pritchard and M. R. J. Elsegood, Chem. Commun., 2009, 7339 RSC.
-
(a) M. Yu and B. L. Pagenkopf, J. Am. Chem. Soc., 2003, 125, 8122 CrossRef CAS PubMed;
(b) M. Yu and B. L. Pagenkopf, Org. Lett., 2003, 5, 5099 CrossRef CAS PubMed;
(c) M. Yu, G. D. Pantos, J. L. Sessler and B. L. Pagenkopf, Org. Lett., 2004, 6, 105 Search PubMed;
(d) B. Bajtos, M. Yu, H. Zhao and B. Pagenkopf, J. Am. Chem. Soc., 2007, 129, 9631 CrossRef CAS PubMed;
(e) C. L. Morales and B. L. Pagenkopf, Org. Lett., 2008, 10, 157 CrossRef CAS PubMed;
(f) M. M. A. R. Moustafa and B. L. Pagenkopf, Org. Lett., 2010, 12, 3168 CrossRef PubMed.
- The synthesis of silyloxy-substituted DA cyclobutanes is well-developed: K. Takasu, Isr. J. Chem., 2016, 56, 488 CrossRef CAS and references therein.
- S. Mangelinckx, B. Vermaut, V. Roland and N. De Kimpe, Synlett, 2008, 2697 CAS.
- M. R. Baar, P. Ballesteros and B. W. Roberts, Tetrahedron Lett., 1986, 27, 2083 CrossRef CAS.
- For a more detailed discussion, see:
M. M. A. R. Moustafa, New Synthetic Methodologies Directed toward Pharmacologically Active Compounds as well as Silole Based Chromophores for Analytical and Optoelectronic Applications, Ph.D. Thesis, The University of Western Ontario, March 2011 Search PubMed.
- Yb(OTf)3 has recently emerged as an efficient catalyst for many synthetic transformations. For general references, see:
(a)
S. Kobayashi, in Lanthanides: Chemistry and Use in Organic Synthesis, ed. S. Kobayashi, Springer, New York, 1999, pp. 63–118 Search PubMed;
(b) S. Kobayashi, M. Sugiura, H. Kitagawa and W. W. L. Lam, Chem. Rev., 2002, 102, 2227 CrossRef CAS PubMed;
(c) R. W. Marshman, Aldrichimica Acta, 1995, 28, 77 CAS;
(d) L.-J. Zhang, H.-L. Lu, Z.-W. Wu and Y.-S. Huang, Curr. Org. Chem., 2013, 17, 2906 CrossRef CAS.
- M. M. A. R. Moustafa and B. L. Pagenkopf, Org. Lett., 2010, 12, 4732 CrossRef CAS PubMed.
- M. M. A. R. Moustafa, A. C. Stevens, B. P. Machin and B. L. Pagenkopf, Org. Lett., 2010, 12, 4736 CrossRef CAS PubMed.
- For examples, see:
(a) P. B. Alper, C. Meyers, A. Lerchner, D. R. Siegel and E. M. Carreira, Angew. Chem., Int. Ed., 1999, 38, 3186 CrossRef CAS;
(b) M. Lautens and W. Han, J. Am. Chem. Soc., 2002, 124, 6312 CrossRef CAS PubMed;
(c) F. Bertozzi, M. Gustafsson and R. Olsson, Org. Lett., 2002, 4, 3147 CrossRef CAS PubMed;
(d) C. Marti and E. M. Carreira, J. Am. Chem. Soc., 2005, 127, 11505 CrossRef CAS PubMed;
(e) M. Yu and B. L. Pagenkopf, Tetrahedron, 2005, 61, 321 CrossRef CAS;
(f) C. A. Carson and M. A. Kerr, J. Org. Chem., 2005, 70, 8242 CrossRef CAS PubMed;
(g) Y.-B. Kang, Y. Tang and X.-L. Sun, Org. Biomol. Chem., 2006, 4, 299 RSC.
- The transformation can also be done in one-pot: the cyclobutane synthesis and subsequent imine cycloaddition in one pot gave comparable yields. See ref. 20.
- For a review on reaction of DA cyclopropanes with nitrones, see: M. A. Kerr, Isr. J. Chem., 2016, 56, 476 CrossRef CAS and references therein.
- A. C. Stevens, C. Palmer and B. L. Pagenkopf, Org. Lett., 2011, 13, 1528 CrossRef CAS PubMed.
- Conditions that allow for exclusive formation of the trans-diastereomer were not found to date, despite exploring various temperatures, catalysts, and solvents.
- For a more detailed discussion, see:
A. C. Stevens, Cycloaddition Chemistry for the Synthesis of Heterocyclic Compounds and Progress Towards the Total Synthesis of Grandilodine A, Ph.D. Thesis, The University of Western Ontario, July 2013 Search PubMed.
- T. Kurihara, Y. Sakamoto, T. Kimura, H. Ohishi, S. Harusawa, R. Yoneda, T. Suzutani and M. Azuma, Chem. Pharm. Bull., 1996, 44, 900 CrossRef CAS PubMed.
- J. H. van Maarseveen, H. W. Scheeren, E. De Clercq, J. Balzarini and C. G. Kruse, Bioorg. Med. Chem., 1997, 5, 955 CrossRef CAS PubMed.
- V. K. Yadav and V. Sriramurthy, Angew. Chem., Int. Ed., 2004, 43, 2669 CrossRef CAS.
- B. P. Machin and B. L. Pagenkopf, Synlett, 2011, 2799 CAS.
- For a more detailed discussion, see:
B. P. Machin, Donor–Acceptor Cyclobutanes and Their Application for Heterocycle Synthesis and Progress Towards Biselide A, Ph.D. Thesis, The University of Western Ontario, August 2013 Search PubMed.
- For a review, see:
(a) Y. Yamamoto and H. Yamamoto, Eur. J. Org. Chem., 2006, 2031 CrossRef CAS;
(b) B. S. Bodnar and M. J. Miller, Angew. Chem., Int. Ed., 2011, 50, 5630 CrossRef CAS PubMed.
- For a review, see:
(a) W. Adam and O. Krebs, Chem. Rev., 2003, 103, 4131 CrossRef CAS PubMed;
(b) M. Baidya and H. Yamamoto, Synthesis, 2013, 1931 CrossRef CAS.
- For a few examples, see:
(a) Y. Yamamoto, N. Momiyama and H. Yamamoto, J. Am. Chem. Soc., 2004, 126, 5962 CrossRef CAS PubMed;
(b) M. Kawasaki, P. Li and H. Yamamoto, Angew. Chem., Int. Ed., 2008, 47, 3795 CrossRef CAS PubMed;
(c) A. Yanagisawa, S. Takeshita, Y. Izumi and K. Yoshida, J. Am. Chem. Soc., 2010, 132, 5328 CrossRef CAS PubMed;
(d) A. Yanagisawa, T. Fujinami, Y. Oyokawa, T. Sugita and K. Yoshida, Org. Lett., 2012, 14, 2434 CrossRef CAS PubMed.
- Following our report (ref. 38), cyclopropanes were reported to undergo cycloadditions with nitrosoarenes:
(a) S. Chakrabarty, I. Chatterjee, B. Wibbeling, C. G. Daniliuc and A. Studer, Angew. Chem., Int. Ed., 2014, 53, 5964 CrossRef CAS PubMed;
(b) S. Das, S. Chakrabarty, C. G. Daniliuc and A. Studer, Org. Lett., 2016, 18, 2784 CrossRef CAS PubMed;
(c) T. Chidley, N. Vemula, C. A. Carson, M. A. Kerr and B. L. Pagenkopf, Org. Lett., 2016, 18, 2922 CrossRef CAS PubMed.
- For examples of NO insertion into the cyclopropane ring, see:
(a) N. Ichinose, K. Mizuno, T. Tamai and Y. Otsuji, Chem. Lett., 1988, 233 CrossRef CAS;
(b) K. Mizuno, N. Ichinose, T. Tamai and Y. Otsuji, J. Org. Chem., 1992, 57, 4669 CrossRef CAS;
(c) F. Cermola, G. Lucrezia Di, G. Maria Liliana and I. Maria Rosaria, J. Chem. Res., 2005, 10, 677 CrossRef.
- N. Vemula, A. C. Stevens, T. B. Schon and B. L. Pagenkopf, Chem. Commun., 2014, 50, 1668 RSC.
- N. Vemula and B. L. Pagenkopf, Eur. J. Org. Chem., 2015, 4900 CrossRef CAS.
- Disappointingly, use of stoichiometric MgI2 further lowered the yields with considerable decomposition of cyclobutane.
- J.-L. Hu, L. Wang, H. Xu, Z. Xie and Y. Tang, Org. Lett., 2015, 17, 2680 CrossRef CAS PubMed.
- F. de Nanteuil and J. Waser, Angew. Chem., Int. Ed., 2013, 52, 9009 CrossRef CAS PubMed.
-
(a) D. Perrotta, S. Racine, J. Vuilleumier, F. de Nanteuil and J. Waser, Org. Lett., 2015, 17, 1030 CrossRef CAS PubMed;
(b) S. Racine, J. Vuilleumier and J. Waser, Isr. J. Chem., 2016, 56, 566 CrossRef CAS.
- R. Shenje, M. C. Martin and S. France, Angew. Chem., Int. Ed., 2014, 53, 13907 CrossRef CAS PubMed.
- Intra- and intermolecular additions to the DA cyclobutanes was recently reported: R. Brimioulle and T. Bach, Angew. Chem., Int. Ed., 2014, 53, 12921 CrossRef CAS PubMed.
|
This journal is © the Partner Organisations 2016 |
Click here to see how this site uses Cookies. View our privacy policy here.