DOI:
10.1039/C5RA03622D
(Paper)
RSC Adv., 2015,
5, 42376-42387
Efficient adsorption of Europhtal onto activated carbon modified with ligands (1E,2E)-1,2-bis(pyridin-4-ylmethylene)hydrazine (M) and (1E,2E)-1,2-bis(pyridin-3-ylmethylene)hydrazine (SCH-4); response surface methodology
Received
28th February 2015
, Accepted 23rd April 2015
First published on
24th April 2015
Abstract
Porous activated carbon was modified with (1E,2E)-1,2-bis(pyridin-4-ylmethylene)hydrazine (M) and (1E,2E)-1,2-bis(pyridin-3-ylmethylene)hydrazine (SCH-4). Characterization was performed using different techniques such as SEM, FTIR and 1H NMR analysis. Then, these new adsorbents were used for the efficient removal of Europhtal (sulfonated cobalt phthalocyanine). Response surface methodology (RSM) was used to achieve operational conditions for the efficient removal of Europhtal using an activated carbon modified with novel ligands. To investigate the individual effect of variables involved and the effect of their possible interactions on the adsorption process, several systematic experiments were designed by varying the variables such as pH, sonication time, adsorbent dosage and dye concentration in the range of 2.0–11, 2–8 min, 0.005–0.035 g and 4–30 mg L−1, respectively. The optimum values of pH, sonication time, adsorbent dosage and Europhtal concentration were found to be 7, 2 min, 30 mg L−1 and 0.03 g for the sample modified with M and 7, 5 min, 30 mg L−1 and 0.03 g for the one modified with SCH-4, at which the removal percentages were more than 98%. Adsorption equilibrium data were well fitted to the Freundlich model with maximum adsorption capacities of 56.69 and 17.84 mg g−1 for M and SCH-4, respectively. The study of adsorption kinetics indicated that the dye uptake process follows the pseudo second-order and saturation type rate expressions for each ligand.
1 Introduction
The effluents composed of synthetic dyes are liberated from different sources to the environment. Dyes are widely utilized in various industrial activities such as coloring, paper, textiles, cosmetics and food.1–3 Their complex (aromatic) molecular structures cause an increase in their resistance to heat, oxidizing agents and biodegradation. Most dyes are toxic and harmful to some microorganisms.4 The toxicity of dyes on plant, animal and human health is not considerably known.5,6 Several versatile approaches, including ion exchange, photo-oxidation processes, flocculation, precipitation, microbiological decomposition and ozonation, have been applied for wastewater treatment and water quality enhancement. Low cost and easy-to-prepare adsorbents are the best candidates for wastewater treatment and removal of pollutants via the adsorption process.7–14 The adsorption process provides an attractive alternative for water treatment because it is easy to handle, requires less maintenance and produces smaller amounts of sludge.5,15–17 Most adsorbents have a high abundance and are cheap; however, they have drawbacks such as poor mechanical strength and heat resistance and relatively limited adsorption capacity for dyes.18–20 Thus, the modification of adsorbents and improvement of their properties, viz reactive centers and surface area, is in great demand. A catalyst solution comprised of 30% of sulphonated cobalt phthalocyanine (Europhtal under the name 802) is generally used to remove mercaptan from hydrocarbon based on either its solution or liquid catalyst loaded on an activated carbon. This catalyst is extensively applied in numerous refineries worldwide and it is necessary to develop novel materials and methods for its removal from various media. In this regard, development and search for novel adsorbent, especially nanoscale adsorbents, is a good option to improve the characteristic performance of the removal procedure. Abundant reactive centers and sites on the surface of nanomaterials make them suitable for trapping other species. Activated carbon-based adsorbents with unique porous reactive sites in the presence of versatile reaction centers, such as hydroxide, carboxylic and amide groups, are good materials for subsequent modification through hydrogen bonding, π–π bonding and electrostatic reactions. Various organic reagents and chelating agents can be used for the modification of the surface of such adsorbents, leading to more improvement in the performance of the removal process.
The present study focuses on the synthesis of (1E,2E)-1,2-bis(pyridin-4-ylmethylene)hydrazine (M) and (1E,2E)-1,2-bis(pyridin-3-ylmethylene)hydrazine (SCH-4) and their subsequent loading on AC followed by characterization by SEM, FTIR and 1H NMR. Then, the potential feasibility of M- and SCH-4-loaded AC for Europhtal removal (as a response) was investigated and the influences of various variables on the response were investigated and optimized. Statistical analysis was applied on the experimental data and results to make a predictive model and test the validity. The kinetic and equilibrium data were analyzed to understand the adsorption mechanism by applying different models to fit the experimental data.
2 Materials and methods
2.1 Materials and instruments
The Europhtal (Table 1) was purchased from Sigma Aldrich and its stock solution (100 mg L−1) was prepared in double distilled water. The concentration of Europhtal was determined by obtaining and analyzing the absorbance spectra before and after each experiment by a 2800-DR (UV-Vis) spectrophotometer at 664 nm. An ultrasonic bath with a heating system (Bandelin sonorex) at a frequency of 40 kHz with a power of 130 W was used for the ultrasound-assisted adsorption procedure. For pH measurements, a Metrohm pH meter model-780 was used. The morphology and size distribution of the M- and SCH-4-loaded AC were observed by scanning electron microscopy (SEM; Hitachi S-4160) at an acceleration voltage of 15 kV. The FTIR spectra of all the compounds were recorded using a JASCO-FTIR-480 instrument in the range of 4000–400 cm−1 using samples prepared in a KBr disc.
Table 1 Properties of the Europhtal dye
Product Identification |
|
Trade name |
Europhtal Additive 802 |
Appearance |
Blue liquid solution |
Chemical name or generic name |
Sulfonated cobalt phthalocyanine (sodium salt) |
Molecular structure |
|
Chemical formula |
C32H16−iN8Co(SO3Na)i |
CAS number |
90294-83-0 |
EINECS number |
290-971-9 |
Shelf life |
Not limited when stored in normal conditions: (temperature: 5 to 40 °C; relative humidity: less than 90%) |
Maximum wavelength (λmax), nm |
664 nm |
USES |
Desulphuration catalyst for oils |
Physical state |
Liquid |
Colour |
Blue |
pH |
7.0 to 9.0 |
Decomposition temperature |
>300 °C |
Relative density (water) |
1.15 |
Solubility |
Soluble in water |
Acute toxicity |
LD 50 > 2000 mg kg−1 (oral rat) |
Hazardous reaction with |
None under normal conditions of use |
2.2 Ultrasound-assisted adsorption method
A batch process was used to evaluate the performance of Europhtal adsorption onto M- and SCH-4-loaded AC in the presence of ultrasound. Adsorption experiments were performed in a cylindrical glass vessel by adding 0.02 g of either of the adsorbents into 50 mL of Europhtal solution at known concentrations (4 and 30 mg L−1) at pH 7, which was optimized by CCD. The vessel was immersed in an ultrasonic bath for 2.8 min at room temperature. Subsequently, the solutions were analyzed for determining the final Europhtal concentration using a UV-Vis spectrophotometer set at a wavelength of 664 nm. The experiments were also performed in the initial dye concentration range of 5–40 mg L−1 to obtain adsorption isotherms. The dye removal percentage (R%) was calculated using the following equation: |  | (1) |
where C0 and Ce (mg L−1) are the initial and equilibrium dye concentrations in an aqueous solution, respectively. The amount of adsorbed dye (qe (mg g−1)) was calculated by the following mass balance relationship: |  | (2) |
where V (L) is the volume of the solution and W (g) is the mass of the adsorbent.
2.3 Preparation of AC from acorn
The seed shells correspond to approximately 25% of the whole acorn. To separate shell from seeds, about 2 kg of acorn was cooked in a 1.0 L glass beaker for 2 h. Then, the acorn seeds were immersed in 2 L of deionized water and boiled for two more hours to remove the water soluble phenolic compounds and to avoid their release during the adsorption experiments. Subsequently, the acorn wastes were washed with distilled water and dried at 70 °C in an air-supplied oven for 8 h. These were then ground in a disk-mill and sieved (70–100 mesh). The sieved mass was then subjected to a carbonization process in an argon atmosphere at a temperature increase rate of 5 °C min−1 to the final temperature of 500 °C and maintained at that temperature for 1 h. The mass was then cooled and washed thoroughly several times by distilled water and dried.21
2.4 Synthesis of (1E,2E)-1,2-bis(pyridin-4-ylmethylene)-hydrazine (M)
1 mL (11 mmol) of hydrazine was added dropwise to a solution of isonicotinaldehyde (2.35 mL, 22 mmol) dissolved in ethanol (15 mL). Two drops of acetic acid were added and the mixture was stirred at room temperature for 24 h. The yellow solid that formed (yield = 73%) was filtered and washed several times with ethanol/ether (1
:
1) (see Fig. 1(a) for the synthesis procedure).
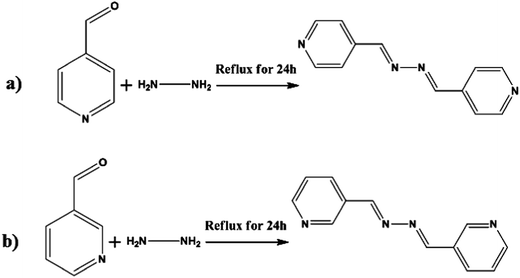 |
| Fig. 1 Procedure for the synthesis of (a) (1E,2E)-1,2-bis(pyridin-4-ylmethylene)hydrazine and (b) (1E,2E)-1,2-bis(pyridin-3-ylmethylene)hydrazine. | |
2.5 Synthesis of (1E,2E)-1,2-bis(pyridin-3-ylmethylene)hydrazine (SCH-4)
1 mL (11 mmol) of hydrazine was added dropwise to a solution of nicotinaldehyde (2.4 mL, 20 mmol) dissolved in ethanol (15 mL). Two drops of formic acid were added and the mixture was stirred at room temperature for 24 h. The product (yield = 73%) was filtered and washed in the same way as that for ligand M (see Fig. 1(b) for the synthesis procedure).
2.6 Central composite design (CCD)
To reduce the number of experiments required, a standard CCD (with 31 experiments) under RSM was applied. It helped us to determine regression model equations and operating conditions at which the maximum R% (as response) is achieved.22–24 Moreover, the advantage of this procedure is the possibility of finding the effects of interaction between each pair of the variables involved, such as pH (x1), sonication time (x2), adsorbent dosage (x3) and Europhtal concentration (x4), on the response (R%) in addition to their individual effects. As mentioned above, two materials were used as an adsorbent, namely, M- and SCH-4-loaded activated carbon. The ranges of variation were set to 2–11 for pH, 2–8 min for sonication time, 0.005–0.035 g for adsorbent amount, and 4–30 mg L−1 for Europhtal concentration (see Tables 2 and 3). CCD has been discussed in detail in the literature.13,19,25 In general, the following equation may be used to plot the experimentally obtained response versus the variables involved. |  | (3) |
Table 2 Factors in CCD and their levels
Factors |
Levels |
Low (−1) |
Central (0) |
High (+1) |
−α |
+α |
x
1: pH |
4.25 |
6.5 |
8.75 |
2 |
11 |
x
2: sonication time (min) |
3.5 |
5 |
6.5 |
2 |
8 |
x
3: adsorbent (g) |
0.0125 |
0.02 |
0.0275 |
0.005 |
0.035 |
x
4: dye concentration (mg L−1) |
10.5 |
17 |
23.5 |
4 |
30 |
Table 3 Experimental runs and responses
Run |
x
1
|
x
2
|
x
3
|
x
4
|
M |
SCH-4 |
Europhtal R% |
Europhtal R% |
1 |
8.75 |
3.5 |
0.0125 |
10.5 |
70.059 |
73.548 |
2 |
8.75 |
3.5 |
0.0125 |
23.5 |
56.111 |
62.209 |
3 |
4.25 |
6.5 |
0.0275 |
23.5 |
87.209 |
95.312 |
4 |
4.25 |
3.5 |
0.0275 |
10.5 |
94.230 |
94.820 |
5 |
8.75 |
6.5 |
0.0125 |
10.5 |
76.219 |
64.400 |
6 |
6.50 |
8.0 |
0.0200 |
17.0 |
92.277 |
90.079 |
7 |
11.0 |
5.0 |
0.0200 |
17.0 |
64.070 |
70.370 |
8 |
4.25 |
3.5 |
0.0275 |
23.5 |
90.285 |
86.140 |
9(C) |
6.50 |
5.0 |
0.0200 |
17.0 |
90.079 |
91.320 |
10 |
2.00 |
5.0 |
0.0200 |
17.0 |
78.930 |
84.830 |
11 |
6.50 |
2.0 |
0.0200 |
17.0 |
88.537 |
76.404 |
12 |
8.75 |
3.5 |
0.0275 |
23.5 |
93.181 |
88.251 |
13 |
8.75 |
3.5 |
0.0275 |
10.5 |
95.977 |
92.215 |
14 |
6.50 |
5.0 |
0.0050 |
17.0 |
48.863 |
65.640 |
15(C) |
6.50 |
5.0 |
0.0200 |
17.0 |
89.030 |
90.706 |
16 |
6.50 |
5.0 |
0.0350 |
17.0 |
95.454 |
93.632 |
17 |
4.25 |
6.5 |
0.0125 |
23.5 |
65.934 |
72.012 |
18 |
8.75 |
6.5 |
0.0125 |
23.5 |
52.189 |
82.954 |
19 |
6.50 |
5.0 |
0.0200 |
4.00 |
97.159 |
95.238 |
20(C) |
6.50 |
5.0 |
0.0200 |
17.0 |
90.545 |
84.980 |
21 |
6.50 |
5.0 |
0.0200 |
30.0 |
84.120 |
94.495 |
22 |
4.25 |
3.5 |
0.0125 |
10.5 |
81.976 |
90.384 |
23(C) |
6.50 |
5.0 |
0.0200 |
17.0 |
90.545 |
90.944 |
24 |
8.75 |
6.5 |
0.0275 |
23.5 |
94.525 |
97.413 |
25 |
4.25 |
6.5 |
0.0275 |
10.5 |
95.348 |
90.909 |
26 |
4.25 |
3.5 |
0.0125 |
23.5 |
64.102 |
60.240 |
27 |
4.25 |
6.5 |
0.0125 |
10.5 |
79.6511 |
79.084 |
28(C) |
6.50 |
5.0 |
0.0200 |
17.0 |
85.144 |
85.433 |
29(C) |
6.50 |
5.0 |
0.0200 |
17.0 |
85.144 |
88.432 |
30(C) |
6.50 |
5.0 |
0.0200 |
17.0 |
85.144 |
88.076 |
31 |
8.75 |
6.5 |
0.0275 |
10.5 |
94.827 |
90.797 |
In this equation, β0, βi, and βii are constant coefficients corresponding to zero-, first- and second-order terms, respectively. βij is the coefficient of interaction term. Depending on the extent to which each term plays a role this model may be varied.
2.7 Model fitting and statistical analysis
The CCD-based experimental data were analyzed using Minitab software version 17.0 to be fitted to a second-order polynomial equation, and then the regression coefficients were obtained. The analysis of variance (ANOVA) was performed to justify the significance and adequacy of the developed regression model. The adequacy of the model was also evaluated by calculating the determination coefficient (R2) in addition to testing it for the lack of fit.
3 Results and discussion
3.1 Characterization of adsorbent
The FTIR and 1H NMR spectra of the ligand M were acquired and are shown in Fig. 2. The FTIR absorption band at 1628 cm−1 for the imine factor, νC
N, confirms the formation of the Schiff-base ligand. Some important absorption bands of the ligands are given in Table 4 and are in good agreement with the reported ones.26,27
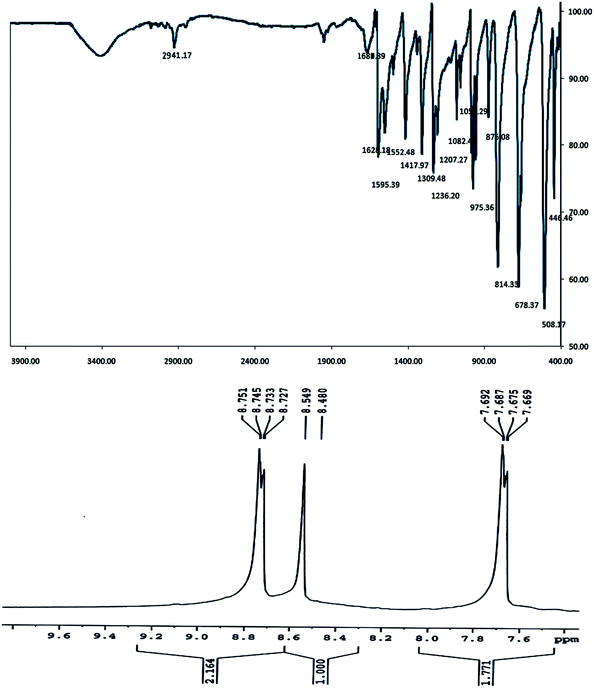 |
| Fig. 2 FT-IR and 1H NMR spectra of ligand (1E,2E)-1,2-bis(pyridin-4ylmethylene)hydrazine (M). | |
Table 4 Wavenumbers (cm−1) of the vibrational modes of ligand
Ligand |
ν
C N imine |
ν
C N imine |
ν
C–N, νC–N aromatic |
M |
2941.17 |
1628.18 |
1595.39, 1552.48, 1417.97 |
SCH-4 |
2925.95 |
1625.28 |
1586.23, 1482.09, 1417.00 |
Fig. 3 shows the FTIR and 1H NMR spectra of the ligand SCH-4. The absorption band appears around the frequency of 1625 cm−1 in the FTIR spectrum of the ligand SCH-4, which corresponds to νC
N, confirming the formation of the Schiff-base ligand. Detailed absorption bands of this ligand are given in Table 4, which are consistent to those reported elsewhere.28
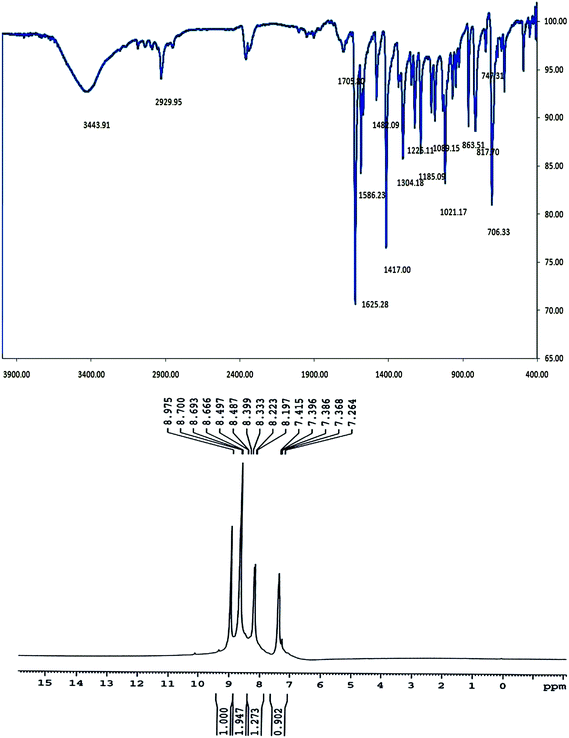 |
| Fig. 3 FT-IR and 1H NMR spectra of ligand (1E,2E)-1,2-bis(pyridin-3-ylmethylene)hydrazine (SCH-4). | |
The morphology of the non-modified AC (Fig. 4(a)) and that of the modified one with either ligand M (Fig. 4(b)) or SCH-4 (Fig. 4(c)) were characterized by SEM, which showed that the approximate particle size was in the range of 40–80 nm. The surface modification is clearly seen after the deposition of ligands onto AC (Fig. 4(b) and (c)).
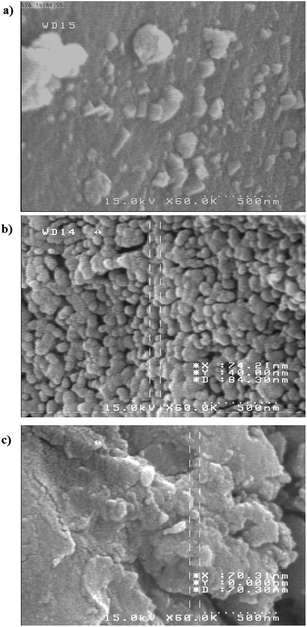 |
| Fig. 4 SEM of AC (a) non-modified and (b) modified by (1E,2E)-1,2-bis(pyridin-4-ylmethylene)hydrazine (M) and (c) modified by (1E,2E)-1,2-bis(pyridin-3-ylmethylene)hydrazine (SCH-4). | |
3.2 Central composite design (CCD)
A total of 31 experiments (runs) were designed by Minitab software version 17.0. The tests for the significance of the regression model for both responses were evaluated and the results of ANOVA tests are presented in Table 5. From ANOVA, the factors with p-values less than 0.05 were distinguished to be significant. The p-values (0.343 and 0.0554 for M and SCH-4 data, respectively) that correspond to the lack of fit for the models applied indicated a non-significant lack of fit. However, the model for predicting R% of Europhtal, corresponding to either M- or SCH-4-based adsorbent, was reconstructed to filter out non-significant factors. Thus, the predictive models were applied as follows: | R%Europhtal = 64.9 + 5.49x1 + 2228x3 − 2.416x4 − 0.827x12 − 71468x32 + 179.7x1x3 + 69.7x3x4, for M-loaded AC | (4) |
| R%Europhtal = 114.3 + 0.25x1 − 1.77x2 + 1761x3 − 5.92x4 − 0.584x12 − 0.686x22 − 43475x32 + 0.0322x42 + 0.2195x1x4 + 0.491x2x4 + 36.4x3x4, for SCH-4-loaded AC | (5) |
Table 5 Analysis of variance (ANOVA)
Source of variation |
M |
SCH-4 |
Sum of square |
Dfa |
Mean square |
F-value |
P-value |
Sum of square |
Dfa |
Mean square |
F-value |
P-value |
Degree of freedom.
|
x
1
|
127.729 |
1 |
127.729 |
17.9149 |
0.005484 |
88.295 |
1 |
88.295 |
12.9980 |
0.011295 |
x
1
2
|
500.791 |
1 |
500.791 |
70.2394 |
0.000157 |
249.621 |
1 |
249.621 |
36.7469 |
0.000914 |
x
2
|
2.319 |
1 |
2.319 |
0.3253 |
0.589131 |
114.514 |
1 |
114.514 |
16.8577 |
0.006316 |
x
2
2
|
8.404 |
1 |
8.404 |
1.1787 |
0.319299 |
68.180 |
1 |
68.180 |
10.0368 |
0.019366 |
x
3
|
3565.419 |
1 |
3565.419 |
500.0748 |
0.000001 |
1785.555 |
1 |
1785.555 |
262.8525 |
0.000004 |
x
3
2
|
462.131 |
1 |
462.131 |
64.8171 |
0.000196 |
171.012 |
1 |
171.012 |
25.1747 |
0.002410 |
x
4
|
511.799 |
1 |
511.799 |
71.7833 |
0.000148 |
45.679 |
1 |
45.679 |
6.7245 |
0.041037 |
x
4
2
|
10.297 |
1 |
10.297 |
1.4443 |
0.274728 |
53.054 |
1 |
53.054 |
7.8101 |
0.031384 |
x
1
x
2
|
1.491 |
1 |
1.491 |
0.2092 |
0.663497 |
11.573 |
1 |
11.573 |
1.7037 |
0.239624 |
x
1
x
3
|
147.147 |
1 |
147.147 |
20.6383 |
0.003921 |
25.270 |
1 |
25.270 |
3.7200 |
0.102025 |
x
1
x
4
|
0.423 |
1 |
0.423 |
0.0593 |
0.815772 |
164.866 |
1 |
164.866 |
24.2701 |
0.002640 |
x
2
x
3
|
0.769 |
1 |
0.769 |
0.1079 |
0.753718 |
0.055 |
1 |
0.055 |
0.0081 |
0.931297 |
x
2
x
4
|
3.634 |
1 |
3.634 |
0.5097 |
0.502087 |
366.998 |
1 |
366.998 |
54.0260 |
0.000325 |
x
3
x
4
|
184.878 |
1 |
184.878 |
25.9304 |
0.002239 |
50.325 |
1 |
50.325 |
7.4084 |
0.034559 |
Lack-of-fit |
101.970 |
10 |
10.197 |
1.4302 |
0.342826 |
263.462 |
10 |
26.346 |
3.8784 |
0.055384 |
Pure error |
42.779 |
6 |
7.130 |
|
|
40.758 |
6 |
6.793 |
|
|
Total |
5660.113 |
30 |
|
|
|
3483.314 |
30 |
|
|
|
Positive and negative signs in each equation imply synergistic and antagonistic effect of the variables, respectively. The values of determination coefficient (R2) were found to be 0.985 and 0.968 for the Europhtal removal percentage using M- and SCH-4-loaded AC, respectively, confirming the good agreement between the experimental and predicted values.
3.3 Response surface methodology
The curvature natures of Fig. 5 and 6 show the response surface plots of removal (%) and confirm a strong interaction among the variables.
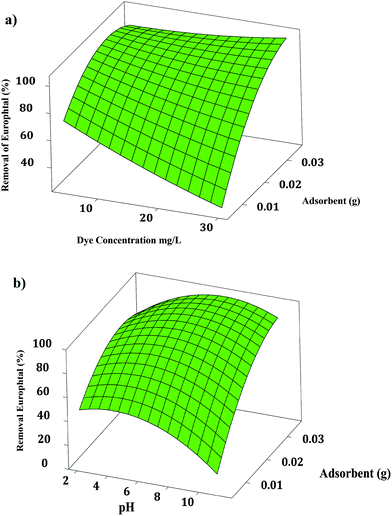 |
| Fig. 5 Response surfaces for the Europhtal removal by M-loaded AC: (a) x1–x3 and (b) x3 and x4. | |
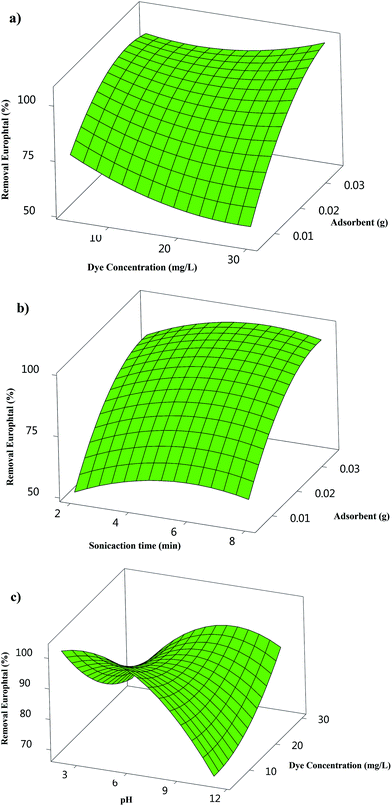 |
| Fig. 6 Response surfaces for the Europhtal removal by SCH-4-loaded AC: (a) x1–x4; (b) x2–x4 and (c) x3 and x4. | |
Fig. 5(a) and 6(a) strongly support the fact that elevating the adsorbent amount and dye concentration leads to an enhancement in the Europhtal removal percentage. An increase in the dye concentration leads to a decrease in the removal percentage. The low and high initial dye concentrations exhibit an opposite trend. The small ratio of solute concentrations to non-occupied reactive adsorbent sites causes an acceleration of dye adsorption and a subsequent increase in removal percentage, while the saturation of adsorption sites is associated with a reduction in the removal percentage at a higher amount of target compounds. The opposite correlation is exhibited for the initial Europhtal concentration; the removal percentage and actual amount of adsorbed Europhtal clearly indicate that the adsorption of Europhtal depends on its initial concentration.
Fig. 5(b) shows the combined effect of adsorbent and pH on the adsorption of Europhtal onto adsorbent at a constant Europhtal initial concentration (30 mg L−1). Increasing pH leads to a significant influence on the removal percentage, and at a constant pH, removal percentage increases with the amount of M. The Europhtal removal decreases with lower amounts of adsorbent, and an approximately constant trend was seen with higher amounts of adsorbent.
The increase in the amount of adsorbent leads to a significant decrease in sonication time (Fig. 6(b)), while the removal percentage at a fixed sonication time has a positive correlation with the amount of adsorbent.
Fig. 6(d) shows the interaction of pH with initial Europhtal concentration and their relation with removal percentage. The pH has a positive correlation with removal percentage, while a higher pH and lower adsorbent concentration lead to the achievement of a lower removal percentage. At a low initial pH, the protonation of the functional groups of adsorbent leads to the generation of a positive charge and appearance of strong attractive forces between the Europhtal molecule and adsorbent surface, thus increasing the removal percentage.
3.4 Optimization
The profile for a desirable option with predicted values in the Minitab software was used for the optimization of the process (Fig. 7). The profile for desirable responses was chosen after specifying the DF for each dependent variable (removal percentage) by assigning predicted values. The scale in the range of 0.0 (undesirable) to 1.0 (very desirable) was used to obtain a global function (D) that its maximum (100%) value related to Europhtal adsorption was achieved in this study.
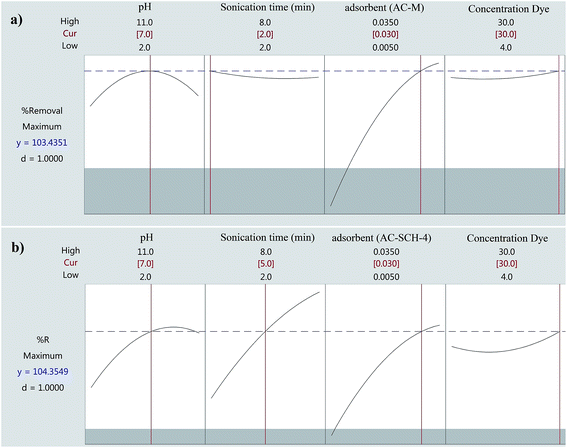 |
| Fig. 7 Profiles for predicated values and the desirability function for the removal percentage of Europhtal by ligands M and SCH-4. Dashed line indicates the current values after optimization. | |
The maximum removal (>98.8%) was obtained at the optimum conditions set as follows: 2 min, 0.03 g of adsorbent, initial Europhtal concentration of 30 mg L−1 at pH 7 using AC-M and 5 min, 0.03 g of adsorbent, initial Europhtal concentration of 30 mg L−1 at pH 7 using AC-SCH-4. The validity of duplicate assenting experiments at the optimized value of all parameters was investigated. The results were closely correlated with the data obtained from the optimization analysis using CCD.
3.5 Adsorption equilibrium study
Common isotherm models, such as Langmuir, Freundlich, Temkin and Dubinin–Radushkevich (D–R), are generally applied to discuss the equilibrium characteristics of adsorption processes.29–32 The constant parameters of the isotherms equations for this adsorption process and their correlation coefficient (R2) are summarized in Table 6. Based on the linear form of the Langmuir isotherm model29 (according to Table 6), the values of KL (the Langmuir adsorption constant (L mg−1)) and Qmax (theoretical maximum adsorption capacity (mg g−1)) were obtained, respectively, from the intercept and slope of the plot of Ce/qe vs. Ce.
Table 6 Various isotherm constants and correlation coefficients calculated for the adsorption of Europhtal dye onto ligands
Isotherm |
Equation |
Plot |
Parameters |
M |
SCH-4 |
0.03 g |
0.02 g |
Langmuir |
C
e/qe = 1/KaQm + Ce/Qm |
A Plot Ce/qe versus Ce should indicate a straight line of slope 1/Qm and an intercept of 1/(KaQm) |
Q
max (mg g−1) |
56.69 |
17.84 |
K
a (L mg−1) |
6.563 |
19.33 |
R
2
|
0.983 |
0.978 |
Freundlich |
ln qe = ln KF + (1/n)ln Ce |
The values of Kf and 1/n were determined from the intercept and slope of linear plot of ln qe versus ln Ce, respectively |
1/n |
0.283 |
0.339 |
K
F (L mg−1) |
4.539 |
3.217 |
R
2
|
0.992 |
0.994 |
Temkin |
q
e = B1 ln KT + B1 ln Ce |
Values of B1 and KT were calculated from the plot of qe against ln Ce |
B
1
|
7.019 |
3.244 |
K
T (L mg−1) |
184.32 |
87.28 |
R
2
|
0.918 |
0.971 |
Dubinin–Radushkevich |
ln qe = ln Qs − Kε2 |
Values of Qm and B were calculated from the plot of ε2 against ln qe |
Q
s (mg g−1) |
38.63 |
14.48 |
B × 10−8 |
1.10 |
1.70 |
E
|
6742 |
5423 |
R
2
|
0.797 |
0.933 |
The parameters of the Freundlich isotherm model,30 such as KF and 1/n (the capacity and intensity of the adsorption), were calculated from the intercept and slope of the linear plot of ln
qe versus ln
Ce, respectively (see Table 6). The values of 1/n were found to be 0.283 and 0.3385 corresponding to M-AC and SCH-4-AC, which show the high tendency of the adsorbents for the adsorption of Europhtal. Furthermore, the high R2 values (0.992 and 0.994 for M-AC and SCH-4-AC, respectively) show the suitability of Freundlich isotherm model for fitting the experimental data over the whole concentration range.
The heat of adsorption and the adsorbent–adsorbate interaction were evaluated using the Temkin isotherm model.33 B represents the Temkin constant related to the heat of the adsorption (J mol−1), T is the absolute temperature (K), R is the universal gas constant (8.314 J mol−1 K−1) and KT is the equilibrium binding constant (L mg−1). R2 values obtained from applying this model are lower than that corresponding to the Freundlich and Langmuir models. Therefore, it is concluded that the Freundlich isotherm represents a better fit to the experimental data than the Langmuir isotherm.
Another commonly used model to explain equilibrium data for the adsorption onto a surface in liquid phase is Dubinin–Radushkevich (D–R),32 which is used to estimate the porosity apparent free energy and the characteristics of adsorption. In the D–R isotherm, K (mol2 (kJ2)−1) is a constant related to the adsorption energy, Qmax (mg g−1) is the theoretical saturation capacity, and ε is the Polanyi potential. The slope of the plot of ln
qe versus ε2 gives K and the intercept yields the Qm value. The mean free energy of the adsorption (E) is known as the free energy change when the energy required to transfer one mole of ion from the solution to the surface of the adsorbent is calculated. The value of the correlation coefficient obtained from the D–R model was considerably lower than that from the other isotherms mentioned above. This means that the D–R equation represents the poorer fit to the experimental data compared to the other isotherm equations.
3.6 Kinetics study
To examine the mechanism of the adsorption process such as mass transfer and chemical reaction, a suitable kinetic model is needed to analyze the rate data. Many models, such as homogeneous surface diffusion model, pore diffusion model and heterogeneous diffusion model (also known as pore and diffusion model), have been extensively applied in batch reactors to describe the transport of adsorbates inside the adsorbent particles.34,35 The kinetics of Europhtal adsorption onto M-AC and SCH-4-AC was assessed using different models such as pseudo-first-order,36 pseudo-second-order,37 intraparticle diffusion38 and Elovich39 models (see Table 7). Among these models, the criterion for their applicability was based on the respective correlation coefficient (R2) and agreement between the experimental and calculated value of qe. The high values of R2 (∼0.999) and good agreement between two qe values obtained from applying pseudo-second-order kinetic model indicate that the adsorption of Europhtal on either of M-AC and SCH-4-AC follows this model (Table 7).
Table 7 Kinetic parameters for the adsorption of Europhtal dye onto the ligands
Model |
Equation |
Plot |
Parameters |
Value of parameters |
M |
SCH-4 |
5 mg L−1 |
40 mg L−1 |
First-order kinetic |
|
Plot the values of log (qe − qt) versus t to give a linear relationship, from which k1 and qe can be determined from the slope and intercept, respectively |
k
1 (min−1) |
0.087 |
0.126 |
q
e (calc) (mg g−1) |
1.060 |
1.373 |
R
2
|
0.914 |
0.874 |
Pseudo-second-order kinetic |
|
Plot the values of (t/qt) versus t to give a linear relationship, from which k2 and qe can be determined from the slope and intercept, respectively |
k
2 (min−1) |
0.005 |
0.149 |
q
e (calc) (mg g−1) |
6.20 |
33.45 |
R
2
|
0.999 |
0.999 |
Intraparticle diffusion |
q
t
= Kdif t1/2 + C |
The values of Kdiff and C were calculated from the slopes of qt versus t1/2 |
K
diff (mg g−1 min−1/2) |
0.226 |
0.715 |
C (mg g−1) |
4.790 |
30.163 |
R
2
|
0.972 |
0.920 |
Elovich |
|
Plot the values of (qt) versus ln (t) to give a linear relationship from which α and β can be determined from the slope and intercept, respectively |
β (g mg−1) |
2.477 |
1.1756 |
α (mg g−1 min−1) |
99.89 |
35.842 |
R
2
|
0.940 |
0.960 |
|
q
e (exp) (mg g−1) |
5.989 |
33.139 |
In this study, we have chosen two long, rigid bipyridyl-based ligands, (1E,2E)-1,2-bis(pyridin-4-ylmethylene)hydrazine and (1E,2E)-1,2-bis(pyridin-3-ylmethylene)hydrazine to react with cobalt phthalocyanine. They are natural ligands with two suitable sites for chelating with metals. The N atoms in the aromatic rings (pyridyl groups) connect to metal centers. These ligands can link two metals (or metals groups).26,27 Schematic diagrams for the adsorption of this catalyst on both the new adsorbents are presented in Fig. 8 and 9.
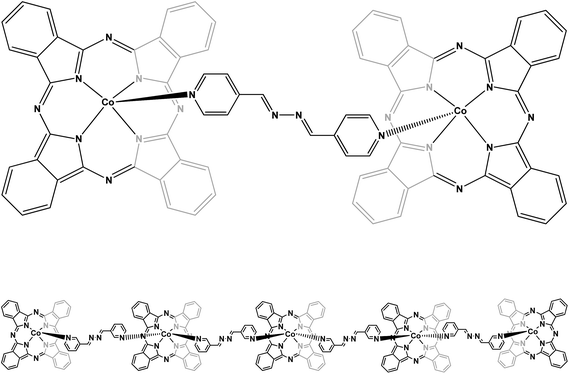 |
| Fig. 8 Reaction between (1E,2E)-1,2-bis(pyridin-4-ylmethylene)hydrazine and cobalt phthalocyanine. | |
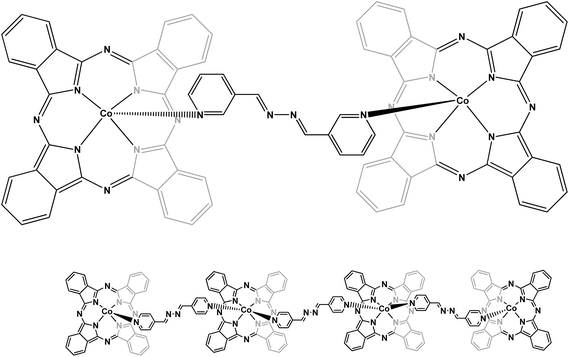 |
| Fig. 9 Reaction between (1E,2E)-1,2-bis(pyridin-3-ylmethylene)hydrazine and cobalt phthalocyanine. | |
3.7 Application to real samples
To observe the efficiency of M-AC and SCH-4-AC for the removal of Europhtal dye from real effluents, three effluent streams were collected separately and the adsorption experiments were performed. Adsorption experiments were conducted using the optimum conditions. More than 96% of dye removal was achieved for all the effluents (Table 8).
Table 8 Percentage removal of the Europhtal dye from four different effluents (N = 3)
Samples |
Initial dye concentration (mg L−1) |
Removal (%) |
M-AC |
SCH-4-AC |
River water |
30 |
99.50 ± 1.4 |
99.21 ± 0.7 |
Sanitary wastewater |
30 |
98.75 ± 2.5 |
99.12 ± 1.21 |
Industrial waste |
30 |
97.54 ± 1.9 |
96.63 ± 2.5 |
Water output from the refinery |
30 |
97.62 ± 2.1 |
96.45 ± 1.7 |
4 Conclusion
Activated carbon was modified with ligands (1E,2E)-1,2-bis(pyridin-4-ylmethylene)hydrazine (M) and (1E,2E)-1,2-bis(pyridin-3-ylmethylene)hydrazine (SCH-4) and characterized using SEM, FTIR and 1H NMR analysis. A very small amount of each adsorbent (0.03 g) was used for the efficient adsorption of Europhtal assisted by ultrasonication. The statistical modeling of the experiments resulted in the prediction of optimal conditions at which a very high percentage of Europhtal was rapidly removed within a couple of minutes. Different models were applied to study the isotherm and kinetics of the adsorption process, which showed the applicability of the Freundlich isotherm and pseudo-second-order kinetics for both the adsorbents. The adsorption capacities of M-AC and SCH-4-AC were found to be 56.69 mg g−1 and 17.84 mg g−1, respectively.
Acknowledgements
The authors express their appreciation to the Graduate School and Research Council of the Islamic Azad University and Fars Science and Research Branch for the financial support of this work.
References
- M. Ghaedi, S. Heidarpour, S. Nasiri Kokhdan, R. Sahraie, A. Daneshfar and B. Brazesh, Powder Technol., 2012, 228, 18–25 CrossRef CAS PubMed
.
- F. A. Pavan, E. C. Lima, S. L. Dias and A. C. Mazzocato, J. Hazard. Mater., 2008, 150, 703–712 CrossRef CAS PubMed
.
- A. Asfaram, M. Ghaedi, S. Agarwal, I. Tyagi and V. Kumar Gupta, RSC Adv., 2015, 5, 18438–18450 RSC
.
- S. Arivoli, M. Hema, S. Parthasarathy and N. Manju, J. Chem. Pharm. Res., 2010, 2, 626–641 CAS
.
- M. Ghaedi, B. Sadeghian, A. A. Pebdani, R. Sahraei, A. Daneshfar and C. Duran, Chem. Eng. J., 2012, 187, 133–141 CrossRef CAS PubMed
.
- H. Lata, V. Garg and R. Gupta, Dyes Pigm., 2007, 74, 653–658 CrossRef CAS PubMed
.
- S. Hajati, M. Ghaedi, F. Karimi, B. Barazesh, R. Sahraei and A. Daneshfar, J. Ind. Eng. Chem., 2014, 20, 564–571 CrossRef CAS PubMed
.
- M. Ghaedi, M. Pakniat, Z. Mahmoudi, S. Hajati, R. Sahraei and A. Daneshfar, Spectrochim. Acta, Part A, 2014, 123, 402–409 CrossRef CAS PubMed
.
- M. Fathi, A. Asfaram and A. Farhangi, Spectrochim. Acta, Part A, 2015, 135, 364–372 CrossRef CAS PubMed
.
- S. Hajati, M. Ghaedi and S. Yaghoubi, J. Ind. Eng. Chem., 2015, 21, 760–767 CrossRef CAS PubMed
.
- V. Garg, R. Gupta and T. Juneja, Chem. Biochem. Eng. Q., 2004, 18, 417–422 Search PubMed
.
- P. K. Malik, Dyes Pigm., 2003, 56, 239–249 CrossRef CAS
.
- M. Ghaedi, H. Mazaheri, S. Khodadoust, S. Hajati and M. K. Purkait, Spectrochim. Acta, Part A, 2015, 135, 479–490 CrossRef CAS PubMed
.
- J. Fu, Z. Chen, M. Wang, S. Liu, J. Zhang, J. Zhang, R. Han and Q. Xu, Chem. Eng. J., 2015, 259, 53–61 CrossRef CAS PubMed
.
- J. P. Silva, S. Sousa, I. Gonçalves, J. J. Porter and S. Ferreira-Dias, Sep. Purif. Technol., 2004, 40, 163–170 CrossRef CAS PubMed
.
- N. K. Amin, J. Hazard. Mater., 2009, 165, 52–62 CrossRef CAS PubMed
.
- M. Ghaedi, S. Hajjati, Z. Mahmudi, I. Tyagi, S. Agarwal, A. Maity and V. Gupta, Chem. Eng. J., 2015, 268, 28–37 CrossRef CAS PubMed
.
- A. Baçaoui, A. Yaacoubi, A. Dahbi, C. Bennouna, R. P. T. Luu, F. Maldonado-Hodar, J. Rivera-Utrilla and C. Moreno-Castilla, Carbon, 2001, 39, 425–432 CrossRef
.
- S. Hajati, M. Ghaedi and H. Mazaheri, Desalin. Water Treat., 2014, 1–15 CrossRef PubMed
.
- R. Azargohar and A. Dalai, Microporous Mesoporous Mater., 2005, 85, 219–225 CrossRef CAS PubMed
.
- M. Ghaedi, H. Hossainian, M. Montazerozohori, A. Shokrollahi, F. Shojaipour, M. Soylak and M. K. Purkait, Desalination, 2011, 281, 226–233 CrossRef CAS PubMed
.
- A. Asfaram, M. Ghaedi, S. Hajati, A. Goudarzi and A. A. Bazrafshan, Spectrochim. Acta, Part A, 2015, 145, 203–212 CrossRef CAS PubMed
.
- M. Roosta, M. Ghaedi, A. Daneshfar, R. Sahraei and A. Asghari, J. Ind. Eng. Chem., 2015, 21, 459–469 CrossRef CAS PubMed
.
- M. Ghaedi, S. Khodadoust, H. Sadeghi, M. A. Khodadoust, R. Armand and A. Fatehi, Spectrochim. Acta, Part A, 2015, 136, 1069–1075 CrossRef CAS PubMed
.
- M. Roosta, M. Ghaedi, A. Daneshfar, S. Darafarin, R. Sahraei and M. Purkait, Ultrason. Sonochem., 2014, 21, 1441–1450 CrossRef CAS PubMed
.
- Y.-B. Dong, M. D. Smith, R. C. Layland and H.-C. zur Loye, Chem. mater., 2000, 12, 1156–1161 CrossRef CAS
.
- D. M. Ciurtin, Y.-B. Dong, M. D. Smith, T. Barclay and H.-C. zur Loye, Inorg. chem., 2001, 40, 2825–2834 CrossRef CAS PubMed
.
- D.-Q. Li, X. Liu and J. Zhou, Inorg. Chem. Commun., 2008, 11, 367–371 CrossRef CAS PubMed
.
- I. Langmuir, J. Am. Chem. Soc., 1916, 38, 2221–2295 CrossRef CAS
.
- H. Freundlich, Z. Phys Chem, 1906, 57, 385–471 CAS
.
- M. Temkin and V. Pyzhev, Chem. Abstr., 1940, 34, 6512 Search PubMed
.
- M. Dubinin and L. Radushkevich, Chem. Zentralbl., 1947, 1, 875–889 Search PubMed
.
- M. Temkin and V. Pyzhev, Acta physiochim. URSS, 1940, 12, 217–222 Search PubMed
.
- M. Ghaedi, A. Ansari, M. Habibi and A. Asghari, J. Ind. Eng. Chem., 2014, 20, 17–28 CrossRef CAS PubMed
.
- I. D. Mall, V. C. Srivastava, N. K. Agarwal and I. M. Mishra, Colloids Surf., A, 2005, 264, 17–28 CrossRef CAS PubMed
.
- S. Lagergren, K. Sven. Vetenskapsakad. Handl., 1898, 24, 1–39 Search PubMed
.
- Y.-S. Ho and G. McKay, Process Biochem., 1999, 34, 451–465 CrossRef CAS
.
- H. A. AL-Aoh, R. Yahya, M. Jamil Maah and M. Radzi Bin Abas, Desalin. Water Treat., 2014, 52, 6720–6732 CrossRef CAS PubMed
.
- S. Kaur, S. Rani, R. K. Mahajan, M. Asif and V. K. Gupta, J. Ind. Eng. Chem., 2015, 22, 19–27 CrossRef CAS PubMed
.
|
This journal is © The Royal Society of Chemistry 2015 |