Tetrahydrofuran (THF)-assisted alkaline extraction to determine hexavalent chromium (Cr(VI)) in retardant polymers containing Sb(III)†
Received
10th July 2014
, Accepted 4th September 2014
First published on 4th September 2014
Abstract
Low recovery of hexavalent chromium (Cr(VI)) in polymers due to the reduction to Cr(III) by Sb(III) has been a big issue for the implementation of the Regulation of Hazardous Substances (RoHS) directive. In this work, we have developed a THF (tetrahydrofuran)-assisted alkaline extraction method to improve the extraction efficiency of Cr(VI) in the presence of Sb(III) by suppressing the reduction through the formation of an Sb(III)–THF adduct. The adduct formed was identified as Sb2O5Cl5(THF)3 using various analytical techniques, such as XRD, NMR, and MALDI-TOF-MS, and by molecular simulation through the calculation of ground state energy. The efficiency of the developed method was demonstrated by the analysis of in-house manufactured reference polymers containing Sb(III). The recovery was obtained in the range of 48.9–77.5%, which was about 19.6–31.0 times more efficient than the current RoHS method. Furthermore, when the THF solvent was mixed with 2-propanol (isopropyl alcohol, IPA) or 1-methyl-2-pyrrolidone (NMP), the recovery was significantly improved to over 95.2%, whereas a mixture with EDTA and NMP had a recovery of <20.0%.
Introduction
Determination of hexavalent chromium Cr(VI), which is used as a pigment in plastics, has become one of the biggest issues for the implementation of the RoHS Directive. However, the current analytical method (IEC 62321:2008), which is based on an alkaline digestion followed by UV-Vis spectrometry has seriously suffered from poor accuracy and precision.1 Recently, an organic-assisted alkaline method was introduced to extract Cr(VI) from plastics to overcome the drawbacks of the alkaline digestion method, in which a polar aprotic solvent, such as NMP, was used to dissolve the plastic samples, followed by alkaline extraction of Cr(VI) ions from the organic solvent.2 Nowadays, most common brominated flame retardants used in electronic polymers contain extensive amounts of antimony trioxide (Sb2O3) as a synergist to enhance the effectiveness of flame retardants. However, the added Sb2O3 reacts with Cr(VI) during the treatment, converting it to Cr(III),3 resulting in serious analytical error, i.e., a low recovery of typically <2.5% for the ABS powder ground to the size of 1000 μm using the current RoHS method. Therefore, the determination of Cr(VI) in plastics containing Sb(III) has been a great challenge for the effective implementation of the RoHS Directive.
So far, there have been several efforts to improve the recovery by preventing the reduction of Cr(VI) through the suppression of Sb(III) oxidation. For example, formation of a Sb(III) complex using a chelate, such as ethylenediaminetetraacetic acid (EDTA), one of the most common chelates for di- and trivalent metals, can be an excellent way to mask Sb(III).4–11 Lintschinger et al. demonstrated the chelation of Sb(III) and Sb(V) species with EDTA, forming a negatively charged complex.12
An alternative way to suppress the reduction of Cr(VI) is formation of an Sb(III) adduct with an organic solvent, such as tetrahydrofuran (THF), a colorless, water-miscible organic liquid with low viscosity at standard temperature and pressure. THF has an oxygen atom that can coordinate to metal ions as a Lewis base. Sian C. James et al. characterized the structure of the [SbI3(THF)] compound, the first THF adduct of antimony halide. The structure was comprised of a polymeric arrangement of SbI3(THF) units, with antimony centers being bridged alternately by pairs of I atoms and pairs of THF ligands, so that each Sb atom has an octahedral coordination geometry.13 In addition, THF has the advantage of good solubility for various electronic polymers, such as ABS, HIPS, and PC. Hence, it can be used as a solvent for polymers as well as a chelating agent for Sb(III) without the reduction of Cr(VI).
Now, the aim of this study was to develop a new extraction method using THF to determine the amount of Cr(VI) with high recovery through the masking of Sb(III). For comparison, the chelating agent EDTA in NMP solvent was also tested on various polymers. Since no reference polymer material exists in the current market, in-house polymer reference materials (RMs) containing Cr(VI) and Sb(III) were specially manufactured for this work using an extrusion method. Under optimized conditions, analytical figures-of-merits of the developed method, such as calibration linearity, limits of detection, and recovery, followed by UV-Vis spectra were determined in this work.
Experimental
Reagents and materials
All chemicals were of analytical reagent grade. All aqueous solutions were prepared using distilled water (DI water). The analytical working solutions of Cr(VI) were prepared from 1000 mg L−1 Cr(VI) standard stock solution (AccuStandard, USA) using DI water. Phosphate buffer (0.5 M K2HPO4/0.5 M KH2PO4) of pH 7 was prepared by dissolving 87.09 g K2HPO4 (Aldrich) and 68.04 g KH2PO4 (Sigma-Aldrich) in a 1 L beaker with DI water. The color developing agent was prepared by dissolving 0.25 g of 1,5-diphenylcarbazide (Aldrich) in a 50 mL volumetric flask and diluting with acetone, and was then stored in a freezer. The alkaline extraction solution was prepared by adding 20.0 (±0.05) g sodium hydroxide (Sigma-Aldrich) and 30.0 (±0.05) g sodium carbonate (Sigma-Aldrich) to a 1 L volumetric flask and diluting with DI water. The pH of this solution should be adjusted to 11.5 or greater prior to use. Magnesium chloride (Sigma-Aldrich) was used without any dilution. 35% (m/m) nitric acid was prepared by diluting 70% (m/m) nitric acid in a 100 mL volumetric flask and was stored in an amber Teflon bottle. 30% (m/m) sulfuric acid was prepared by diluting 30 mL of concentrated sulfuric acid to 100 mL with DI water in a volumetric flask. All glassware, beakers, plastic bottles, etc., were soaked in a 5% (v/v) nitric acid solution for 24 h and rinsed with DI water prior to use.
Preparation of in-house polymer reference materials (RMs)
ABS (SD-0150, Cheil Industries Inc., Korea), HIPS (HR-1360, Cheil Industries Inc., Korea) and PC (SC-1220R, Cheil Industries, Korea) were used as base polymer materials for the preparation of in-house polymer RMs. Each base material contained a small amount of organic and inorganic additives at <2%. As additives, lead chromate (PbCrO4, Sigma-Aldrich) and antimony trioxide (Sb2O3, Sigma-Aldrich) were added to each base material with a suitable dispersing agent. Then, the mixtures were extruded at a melt temperature of about 200 °C using an extruder (TEK30, SM PLATEK Inc., Korea). The obtained granulates were mixed and extruded repeatedly to achieve optimum homogeneity. It was intended to produce two polymer samples (5 kg each) containing 322 and 1165 mg kg−1 of Cr(VI). The resulting materials were cut into pieces of ca. 10 mg and were stored in a 50 g bottle.
Instrumentation
An inductively coupled plasma-atomic emission spectrometer (ICP-AES, 7300DV, PerkinElmer-Sciex) was used to determine the total contents of chromium and antimony in the in-house RMs. A UV-Vis spectrophotometer (V-550, JASCO, Japan) equipped with a quartz cell was used to analyze the extracted Cr(VI) complex.
X-ray diffraction patterns were obtained using a PRO MPD diffractometer (Panalytical X'Pert). Unless otherwise stated, all runs were over a 2θ range of 10 to 70° at 0.02° increments using a Cu Kα radiation source at 40 kV to 30 mA for vitreous samples.
All MALDI-TOF mass spectra were acquired using a MALDI SYNAPT G2 mass spectrometer (Waters, UK). The system utilizes a pulsed nitrogen laser (2.5 kHz), emitting at 337 nm. The acquisition mode was sensitive mode and the polarity was positive. The mass range was from 100 to 1000 Da and there was no matrix material. NMR was performed using a Bruker Ascend™ 600 MHz wide bore NMR spectrometer operating at 151.06 MHz for the 13C and 600.7 MHz for the 1H resonance frequency. Cross-polarization with magic angle spinning (CPMAS) was applied at 35 kHz. A contact time of 1 ms was used, with a pulse delay of 5 s.
Extraction procedure and method
NMP-assisted alkaline extraction with EDTA chelation.
Samples were directly dissolved in 1-methyl-2-pyrrolidone (NMP) with EDTA. 0.15 g of the sample and 10 g of NMP (with excess EDTA) were placed in 20 mL vials and dissolved by using a shaker for 3 h. About 5 g of the sample solution and 20 mL of the alkali solution with EDTA were added to a 150 mL beaker. Then, 1 mL of phosphate buffer solution and magnesium chloride was added. After being adjusted to pH 7~8 with 35% nitric acid, the resulting solutions were diluted to 50 g using DI-water. Then, this solution was adjusted to the pH 2.0~2.5 with 10% sulfuric acid. Finally, 2.5 mL of 0.5 M diphenylcarbazide (DPC, Special Grade, Merck), a colour developing agent, was added for chelation with Cr(VI) and the resulting violet solution was introduced into a UV-Vis spectrophotometer. The absorption peak of the Cr(III)–DPCO complex was observed at 540 nm. The absorbance reading of the sample was corrected by subtracting the absorbance of a blank carried through the color development procedures. From the corrected absorbance, the concentration of Cr(VI) was determined by referring to the calibration curve.
THF-assisted alkaline extraction for ABS and HIPS polymers.
ABS and HIPS polymer samples were directly dissolved in THF. 0.15 g of the sample and 6 g of THF were placed into a 20 mL tube and dissolved by using a shaker for 1 h. After that, 3 g of isopropyl alcohol (IPA) was added to the solution. Then, 7 g sample solution and 20 mL of the alkali solution were mixed in a 150 mL beaker. The analytical procedure was then performed in accordance with the NMP-assisted alkaline extraction with EDTA chelation, mentioned in the section above.
THF-assisted alkaline extraction for the PC polymer.
PC polymer samples were directly dissolved in 1-methyl-2-pyrrolidone (NMP). 0.15 g of the sample and 6 g of NMP were placed into 20 mL tubes and dissolved by using a shaker for 1 h. After that, 3 g of IPA was added to the solution. Then, 7 g sample solution and 20 mL of the alkali solution were mixed together in a 150 mL beaker. The rest of the analytical procedure was performed in accordance with the NMP-assisted alkaline extraction with EDTA chelation, mentioned in the section above.
Additive reaction between Sb(III) and Cr(VI).
For the study of the additive reaction of Sb(III)–Cr(VI), 1.89 g of 10
000 mg mL−1 Sb(III) solution and 2.2 g of 1000 mg mL−1 Cr(VI) solution were charged into 20 mL sealable glass bottles. 8.4 g of distilled water was added to the tubes, which were then tightly capped and shaken in an air bath at 25 °C for 3 h. Similarly, the additive reaction was tested in THF, i.e., 2.1 g of 10
000 mg mL−1 Sb(III) solution and 2.00 g of 1000 mg mL−1 Cr(VI) solution were charged into 20 mL sealable glass bottles. 9.3 g of distilled water was added to the bottle and 1.5 g of THF was added dropwise. After the reaction, the bottle was tightly capped and placed in an air bath at 25 °C for 3 h for suspension. The suspended particles were then collected by centrifugal separation at 20
000 rpm, filtered with a membrane filter (pore size 0.45 μm), and dried at 70 °C for 2 h. The total antimony and chromium content of the filtrates was determined by ICP-AES and the Cr(VI) content was determined by using a UV-Vis spectrophotometer. The solid phase was analyzed by X-ray diffraction (XRD), NMR and MALDI-TOF-MS.
Results and discussion
Homogeneity test of the in-house manufactured polymer RMs
Prior to the development of a new method, reference materials (RM) should be prepared and verified by an appropriate analytical method. Unfortunately, since no commercialized polymer RM containing Cr(VI) with Sb(III) is currently available on the market, in-house RMs based on ABS, HIPS and PC polymers were specially produced for use in this work. The homogeneity was tested following the guidelines of ISO (guide 35, 2006) and the international harmonized protocol. Following the guidelines, the bulk RMs were divided into 10 bottles each containing 0.10 g. All the RM samples were then treated using acid decomposition and ICP-AES was performed on the resulting solutions to determine the chromium and antimony content. Analytical results of the homogeneity tests are listed in Table 1. As shown in the table, the measured concentrations of total Cr in all the RMs were very close to the expected concentrations with a relative standard deviation (RSD) of <5%. One-way analysis of variance (one-way ANOVA) using Excel 2007 software was performed on the results. The null hypothesis of the one-way ANOVA test, H0, indicated that there was no difference among the mean concentration of the bottles, or, that the analytical results came from populations with the same mean. If the calculated statistics of the test, F, was lower than the critical Fc value, there would be no evidence to reject H0. Since F was indeed smaller than Fc, as shown in the table, it was concluded that the all the in-house RMs were sufficiently homogeneous. However, the F value and p-value of RM-2 for ABS and PC were not sufficient, compared to other RMs because of the high content of Sb2O3 (>4.1%).
Table 1 Analytical results (n = 10) of the homogeneity study of in-house RMs using ICP-AES
Base material |
Standards |
Expected Cr(VI) (mg kg−1) |
Measured Cr(VI) (mg kg−1) |
RSD (%) |
F
|
p-value |
F
c
|
F = (between-group variability/within-group variability) = MSB/MSW (Sb(III) concentration: 2.5–4.3%).
F
c for α (significance level) = 0.05, ν1 (between-group degrees of freedom) = 9, and ν2 (within-group degrees of freedom) = 20.
|
ABS |
RM-1 |
322 |
292 |
2.6 |
0.77 |
0.642 |
2.39 |
RM-2 |
1165 |
1103 |
1.0 |
2.28 |
0.060 |
2.39 |
HIPS |
RM-1 |
322 |
228 |
2.9 |
0.44 |
0.896 |
2.39 |
RM-2 |
1165 |
862 |
1.1 |
0.39 |
0.926 |
2.39 |
PC |
RM-1 |
322 |
284 |
3.0 |
0.34 |
0.950 |
2.39 |
RM-2 |
1165 |
1076 |
4.1 |
2.19 |
0.069 |
2.39 |
Effect of EDTA complexation on the recovery of Cr(VI)
The stability constant of the Sb(III)–EDTA complex was 24.80, and no report on Cr(VI) and EDTA complexation has considered it as a way to suppress the oxidation of Sb(III) and to protect Cr(VI) from reduction. The masking effect of EDTA on the recovery of Cr(VI) in NMP-assisted alkaline extraction was tested by UV-Vis spectrometry using the in-house manufactured RMs and the analytical results were compared with the results from ICP-AES. As listed in Table 2, the recovery of Cr(VI), as compared to the analytical result of ICP-AES, was <20.0% for all the polymers. This suggested that the addition of EDTA to the NMP-assisted alkaline extraction improved the recovery of Cr(VI), compared to the current RoHS method, which has a known recovery of <5%, but there was still plenty of room for improvement, to obtain complete suppression of the redox reaction of Sb(III)–Cr(VI).
Table 2 Analytical results of Cr(VI) content using NMP-assisted alkaline extraction with EDTA masking
Classification |
ABS |
HIPS |
PC |
[Concentration by UV-Vis]/[concentration by ICP-AES] × 100.
|
Measured concentration of total Cr by ICP-AES (mg kg−1) |
291.6 ± 3.9 |
228.0 ± 3.2 |
283.6 ± 3.9 |
Measured concentration of Cr(VI) by UV-Vis spectrometry (mg kg−1) |
31.4 ± 8.3 |
45.7 ± 10.0 |
43.2 ± 26.0 |
Recovery rate (%) of Cr(VI)a |
10.8 |
20.0 |
15.2 |
THF-assisted alkaline extraction to suppress the redox reaction
Effect of the additive reaction of THF with Sb(III) on the determination of Cr(VI).
After screening various organic solvents, we found that THF possessed the superior extraction efficiency for Cr(VI) in the presence of Sb(III) without the polymer matrix. When THF was added, a solid adduct was formed. Conceptually, two additive reactions were possible for the adduct, i.e., the formation of Sb(III)–THF and/or Cr(VI)–THF. In order to clarify the adduct identity, the concentration of Cr(VI) in the filtrate was determined using ion chromatography and the precipitate was analyzed using ICP-AES after filtration. The results strongly indicated that the adduct identity was Sb(III)–THF, as the precipitates and the filtrate contained large amounts of Sb and Cr(VI), respectively. In addition, though the recovery rate of Cr(VI) obtained by the current RoHS method applied in this study was only about 2.5%, it increased to about 95.0% when THF was added. Therefore, we concluded that the use of THF in the alkaline extraction method uniquely suppressed the reduction of Cr(VI) by the formation of an Sb–THF complex.
Identification of Sb(III)–THF adducts by MALDI-TOF-MS.
For further structural confirmation, the structure of the Sb(III)–THF adduct was analyzed using MALDI-TOF-MS (Fig. 2). From the fragment pattern, the molecular weight of the Sb(III)–THF adduct was identified at 717 mass-to-charge ratio (m/z), because the pattern of all molecular ion clusters showed a separation of 2 mass units due to the presence of the chlorine atom. Generally, compounds containing the chlorine atom can be readily recognized in mass spectrometry, and the number of chlorine atoms can also be determined by the chlorine rule. The assignment of 717 m/z as the molecular weight (MW) was very important because it can be considered as the conclusive evidence of the presence of the Sb(III)–THF adduct, formed by the additive reaction.
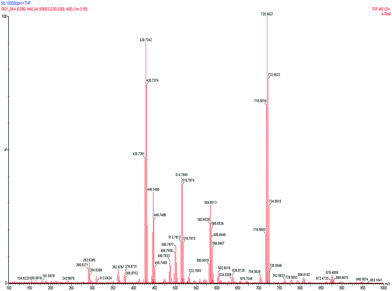 |
| Fig. 2 Positive ion MALDI-TOF-MS spectrum of the solid product formed in the reaction between Sb(III) and THF. The peak at 716.6 (m/z) was identified to be the molecular weight of the Sb(III)–THF adduct. | |
The number of split isotope patterns, molecular weight (observed), expected fragment structure, and molecular weights (calculated) in each mass range are listed in Table 4.
Table 4 Assignment of the fragment structure for the solid adduct from the data obtained using MALDI-TOF/MS
Peak pattern (mother ion) |
Number of split isotope patterns |
Chlorine atoms contained |
Molecular weight (observed) |
Expected fragment structure |
Molecular weight (calculated) |
716–726(716) |
6 |
5 |
716.56 |
Sb2O5Cl5 (THF)3 |
716.77 |
580–588(580) |
6 |
5 |
580.65 |
H1Sb2O1Cl5 (THF)2 |
581.77 |
512–518(512) |
4 |
3 |
512.78 |
H4Sb2O1Cl3 (THF)2 |
513.87 |
496–502(496) |
4 |
3 |
496.78 |
H3Sb2Cl3 (THF)2 |
496.87 |
444–450 |
4 |
3 |
444.74 |
H6Sb2O1Cl3 (THF)1 |
444.97 |
426–432 |
4 |
3 |
426.74 |
H5Sb2Cl3 (THF)1 |
426.87 |
360–364 |
3 |
2 |
360.87 |
Sb2O3Cl2 |
362.42 |
308–312 |
3 |
2 |
308.84 |
H4SbO7Cl2 |
308.66 |
290–294 |
3 |
2 |
290.83 |
H4SbO6Cl2 |
292.66 |
In the m/z range of 716 to 726, the peak was split into 6 molecular ions (MW of 716, 718, 720, 722, 724, and 726), which strongly implied that the solid product contained 5 chlorine atoms by the (n − 1) chlorine rule. The presence of 3 THF units was identified from the mass spectra of 3 regions at 586 to 514, 518 to 446, and 362 to 290 m/z, which showed a difference of molecular weight of 72 mass units.
From the interpretation, the structure was narrowed down to three possibilities, as shown in Fig. 3. Since the predicted structures had five coordination numbers, it provided no chance to reduce Cr(VI), which agreed with the analytical results of UV-Vis measurement. Among the structures, structure (a) (–Sb–O–Sb–) was found to be the most stable from the calculation of ground state energy using the molecular simulation by density functional theory (DFT)-generalized gradient approximation (GGA). As listed in the figure, the calculated thermodynamic energy of structure (a) was 4.2017832 × 107 kJ mol−1, which was the largest of the three. Based on all the analytical results and the calculations, we conclude that the product of the additive reaction, Sb2O5Cl5(THF)3, clearly has the structure shown in Fig. 3a.
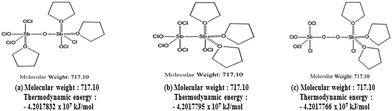 |
| Fig. 3 Predicted structures of the Sb(III)–THF adduct based on MALDI-TOF/MS and the ground state energy calculation using a molecular simulation by density functional theory (DFT)-Generalized Gradient Approximation (GGA). | |
Application of the THF-assisted alkaline extraction to polymer samples
When the developed THF-assisted alkaline extraction method was applied to ABS and HIPS polymers, recoveries of 48.9% and 77.5% were obtained, respectively, in comparison to the results obtained by ICP-AES (Table 5). Although those results were significantly larger than the recoveries obtained by the current RoHS method or by the NMP-assisted alkaline extraction with the addition of EDTA, the recovery was still significantly low, compared to that without the polymer matrix.
Table 5 Analytical results of Cr(VI) in polymer samples using the developed THF-assisted alkaline extraction
Classification |
ABS |
HIPS |
Measured concentration of total Cr by ICP-AES (mg kg−1) |
291.6 |
228.0 |
Measured concentration of Cr(VI) by UV-visible spectrometry (mg kg−1) |
142.5 |
176.6 |
Recovery rate of Cr(VI) (%) |
48.9 |
77.5 |
Optimization of the developed THF-assisted alkaline extraction
In order to maximize the recovery of Cr(VI), the amount of THF extraction solvent was optimized using the mixture of IPA or NMP, varying the solvent ratio to THF while the extraction time and pH of the final solutions were fixed at 60 min and 2.0, respectively (ESI Fig. S-1 and S-2†). The optimum ratio of 6
:
3 for both THF–IPA and NMP–THF mixtures was chosen for ABS/HIPS and PC polymers, respectively. When the optimized solvent was used, the recovery was increased to >95.2%, as shown in the figures, which was nearly 19–38 fold better than that obtained by the current RoHS method.
Analytical figures-of-merits
All the analytical figures-of-merits of the developed THF-assisted alkaline extraction (6
:
3 = THF–IPA) were determined by UV-Vis measurements, following the requirements of the ICH Guideline Q2B,14–17 for linearity, precision, accuracy, limits of detection (LOD) and limits of quantification (LOQ). The linearity was estimated in the desired concentration range of 0–1.5 mg L−1 of Cr(VI) under the optimum conditions, as described above. Six-point calibration curves with matrix matching were obtained, of which the linear regression coefficient (R2) was >0.999 (ESI Fig. S-3†).
The LOD and LOQ were calculated as follows:
and
where
σ is the standard deviation of the blank and
S is the slope of the calibration curve. The calculated LOQs for ABS, HIPS and PC polymers were 0.058, 0.044 and 0.040 mg kg
−1, respectively. The dilution factor for the developed extraction method was about 500×, thus the final LOQ multiplied by the dilution factor was less than about 30 mg kg
−1. The results indicated that the new quantitative method with the proposed extraction method was sufficiently sensitive to determine the accurate Cr(
VI) composition for the evaluation of RoHS compliance, which requires a threshold of <1000 mg kg
−1 for electronic products.
The accuracy and precision of the method were also assessed by analyzing the recovery and repeatability. The repeatability was calculated as the relative standard deviation (RSD). For repeatability, the quantitative analysis was carried out on ten identical RM samples under the same experimental conditions. The results are listed in Table 6, showing recoveries in the range of 95.2 to 100.3% with an RSD of <5%, as compared to the analytical results obtained by ICP-AES (Table 1) for method validation.
Table 6 Comparison of the analytical result from UV-Vis spectrometry for the optimized alkaline extraction method with ICP-AES results
Base material |
Standards |
Measured total Cr conc. by ICP-AESa (mg kg−1) |
Measured Cr(VI) conc. by UV-Visb (mg kg−1) |
Recoveryc (%) |
RSD (%) |
Table 1.
Number of analytical replicates: 10.
[Cr(VI) concentration by UV-Vis]/[total Cr concentration by ICP-AES] × 100.
|
ABS |
RM-1 |
292 |
278 ± 5 |
95.2 |
1.7 |
RM-2 |
1103 |
1082 ± 21 |
98.1 |
2.0 |
HIPS |
RM-1 |
228 |
228 ± 11 |
100.3 |
4.8 |
RM-2 |
862 |
829 ± 11 |
96.1 |
1.4 |
PC |
RM-1 |
284 |
270 ± 9 |
95.3 |
3.1 |
RM-2 |
1076 |
1043 ± 18 |
97.0 |
1.7 |
Conclusions
In this work, a THF-assisted alkaline extraction method was developed to improve the recovery of Cr(VI) in plastic materials in the presence of Sb(III). The developed method achieved recoveries >95.2%, which showed significant improvement, over the current RoHS method, typically obtaining <5% in our work. From experimentation, we also proved that THF efficiently suppresses the conversion of Cr(VI) to Cr(III) by the formation of a Sb(III)–THF adduct, and identified the structure to be Sb2O5Cl5(THF)3 based on the analytical results of XRD, NMR, and MALDI-TOF-MS. In addition, all the analytical figures-of-merits using UV-Vis measurement were determined satisfactorily, and the method was verified by ICP-AES. Therefore, this new extraction technique should be suitable as a successful new sample preparation method to determine Cr(VI) in IEC62321 for the International Electrotechnical Commission's Technical Committee 111 (IEC TC111). A new edition of IEC 62321 is currently in progress, with reflection of the method developed in this work.
References
-
W. Leung and R. J. Starink, Report on the Results of Proficiency Test Metals in Plastics, September 2007, http://www.iisnl.com/pdf/iis07P03X.pdf Search PubMed.
- J. S. Kim and Y. R. Choi, Anal. Chim. Acta, 2011, 690, 182–189 CrossRef CAS PubMed.
-
S. S. Lau and J. P. Kuczynski, 44th International Symposium on Microelectronics 2011 (IMAPS 2011), TP4, 2011, p. 345 Search PubMed.
- B. Sun, F. J. Zhao, E. Lombi and S. P. McGrath, Environ. Pollut., 2011, 113, 111–120 CrossRef.
- K. E. Toghill, M. Lu and R. G. Compton, Int. J. Electrochem. Sci., 2011, 6, 3057–3076 CAS.
- G. Capodaglio, C. M. G. Van den Berg and G. Scarponi, J. Electroanal. Chem., 1987, 235, 275–286 CrossRef CAS.
- N. Nakiboglu, I. Sahin and F. N. Ertas, Anal. Lett., 2008, 41, 2621–2633 CrossRef CAS.
- G. A. E. Mostafa, Talanta, 2007, 71, 1449–1454 CrossRef CAS PubMed.
- J. Zheng, M. Ohata and N. Furuta, Anal. Sci., 2000, 16, 75–80 CrossRef CAS.
- N. Yonehara, Y. Nishimoto and M. Kamada, Anal. Chim. Acta, 1985, 172, 183–190 CrossRef CAS.
- J. Zheng and M. Ohata, Anal. Sci., 2001, 17, i45–i48 CrossRef.
- J. Lintschinger, I. Koch, S. Serves, J. Feldmann and W. R. Cullen, Fresenius. J. Anal. Chem., 1997, 359, 484–491 CrossRef CAS.
- S. C. James, N. C. Norman, O. A. Guy and M. J. Quayle, Acta Crystallogr., Sect. C: Cryst. Struct. Commun., 1997, 53, 1024–1027 Search PubMed.
-
ISO Guide 34, General Requirements for the Competence of Reference Material Producers, International Organization for Standardization, Geneva, 2009 Search PubMed.
- ICH Harmonized Tripartite Guideline – Guideline on Validation of Analytical Procedures: Methodology developed to complement the Parent Guideline (Q2B), 2005.
-
ISO Guide 35, Reference Materials – General and Statistical Principles for Certification, International Organization for Standardization, Switzerland, 2006 Search PubMed.
-
EURACHEM/CITAC Guide, Quantifying Uncertainty in Analytical Measurement, EURACHEM/CITAC, United Kingdom, 2nd edn, 2000 Search PubMed.
Footnote |
† Electronic supplementary information (ESI) available: Fig. S-1–S-3. See DOI: 10.1039/c4ja00226a |
|
This journal is © The Royal Society of Chemistry 2015 |
Click here to see how this site uses Cookies. View our privacy policy here.