DOI:
10.1039/C4QO00112E
(Research Article)
Org. Chem. Front., 2014,
1, 608-613
Cationic gold(I)-catalyzed enantioselective hydroalkylation of unactivated alkenes: influence of the chloride scavenger on the stereoselectivity†
Received
17th April 2014
, Accepted 30th April 2014
First published on 5th May 2014
Abstract
Enantioselective intramolecular additions of β-ketoamides to unactivated alkenes have been accomplished by means of gold catalysis. Chiral LAuCl complexes have been activated by various Lewis acids (Cu, Ag, Ga, In, Si, Bi etc.). The influence of these activators on the stereoselectivity has been studied.
Catalytic C–C bond forming reactions enjoy a pre-eminent place in organic chemistry. Among them, addition of carbon nucleophiles (or pronucleophiles1) to alkynes, allenes, or alkenes, has become an essential synthetic tool.2 Whereas catalytic asymmetric hydroalkylation and hydroarylation of alkynes3 and allenes4,5 have been well-studied (Scheme 1, eqn (1) and (2)), the case of simple olefins has received little attention (eqn (3)).6
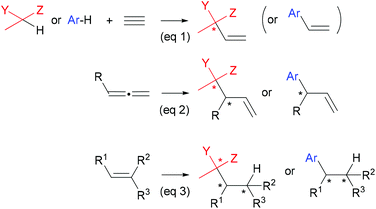 |
| Scheme 1 Prototypical hydrocarbonations of alkynes, allenes, and alkenes, leading to chiral products (Y, Z = COR, CO2R, CN, SO2Ph, Meldrum acid etc.). | |
In contrast with catalytic asymmetric additions to activated alkenes (Michael type reactions) which can be achieved in a variety of ways, including with pronucleophiles,7 the corresponding transformations of readily available unactivated substrates are still scarce8 and mostly rely on stoichiometric carbometallation.9 Only a few catalytic enantioselective hydroarylations of unactivated alkenes have been reported.10 Widenhoefer et al. notably described platinum-catalyzed intramolecular additions of indoles to alkenes (Scheme 2).10a,b
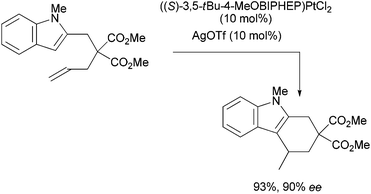 |
| Scheme 2 Example of enantioselective metal-catalyzed hydroarylation of an unactivated alkene. | |
To the best of our knowledge, no catalytic asymmetric hydroalkylation of an unactivated alkene had been reported until we described11 the cyclization of ene-β-ketoamide 1a in the presence of (R)-DTBM-SEGPHOS(AuCl)2A1 and Cu(OTf)2 (Scheme 3).12–14 This reaction led to a mixture of the separable enantioenriched diastereomers 2a and 2a′.15
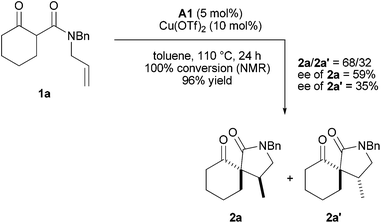 |
| Scheme 3 Enantioselective hydroalkylation of ene-β-ketoamide 1a. | |
In our previous study, our aim was to show that not only silver salts could serve as scavengers to generate active cationic gold complexes from the corresponding chlorides,16 but also Cu(I) and Cu(II) complexes such as Cu(OTf)2. The interest of using a copper salt is to gradually deliver the fragile cationic gold species from the very stable gold chloride. Among various reactions, we have carried out the one shown in Scheme 3 as a proof that the cyclization was not merely attributable to the formation of triflic acid or molecular Au(0) clusters in the reaction medium.17,18 No attempt to improve the stereoselectivity was made. The possible influence of the activator on the stereoselectivity was not studied either. Since two contiguous stereogenic sp3 carbons are formed, this case seems more challenging than the related Conia-ene reaction on alkynes.3 Nevertheless, considering its innovative ground and its inherent synthetic interest,19 we decided to study in detail the experimental factors governing the asymmetric hydroalkylation of ene-β-ketoamides.
Contrary to the previously studied substrates under Au/Cu catalysis,11a Cu(OTf)2 alone proved able to promote the cyclization of 1a, albeit at a slower pace (Table 1, entry 1, 32% conversion without gold),20,21 which possibly hampers the enantioinduction. Lowering the temperature to 50 °C dramatically decreased the conversion to 9% (entry 2), even after raising the proportion of Cu(OTf)2 to 25 mol% (entry 3). The use of 10 mol% of (MeCN)5Cu(SbF6)2 increased the conversion to 29% at 50 °C (entry 4). It reached 51% with 25 mol% of the Cu(II) salt (entry 5). Finally, the Cu(I) salt CuOTf·0.5C6H6 allowed 92% conversion at 50 °C provided 25 mol% were engaged (entries 6 and 7). In the context of the development of silver-free two-component catalytic mixtures in gold catalysis, the higher activity of CuOTf compared to Cu(OTf)2 under smooth conditions is an interesting feature. However, there is no positive effect of lowering the temperature on the stereoselectivity.
Table 1 Enantioselective hydroalkylation of ene-β-ketoamide 1a using the chiral gold complex A and copper salts
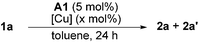
|
Entry |
[Cu] |
x
|
T (°C) |
Conva,b (%) |
dra |
eea,c (%) |
Estimated by chiral SFC; diastereomeric ratios shown are 2a/2a′.
Conversion without gold is indicated in parentheses.
Corresponding to 2a/2a′.
The dr without gold is 64/36.
|
1 |
Cu(OTf)2 |
10 |
110 |
100 (32) |
68/32d |
59/35 |
2 |
Cu(OTf)2 |
10 |
50 |
9 (6) |
84/16 |
49/22 |
3 |
Cu(OTf)2 |
25 |
50 |
9 (7) |
85/15 |
44/44 |
4 |
(MeCN)5Cu(SbF6)2 |
10 |
50 |
29 (6) |
77/23 |
52/14 |
5 |
(MeCN)5Cu(SbF6)2 |
25 |
50 |
51 (11) |
71/29 |
45/13 |
6 |
CuOTf·0.5C6H6 |
10 |
50 |
34 (16) |
78/22 |
52/28 |
7 |
CuOTf·0.5C6H6 |
25 |
50 |
92 (18) |
65/35 |
51/25 |
Since the diastereoselectivity with Cu(OTf)2 alone was similar to that obtained with the Au/Cu catalytic system (64/36 vs. 68/32 respectively, see Table 1, entry 1), the question of a possible chiral ligand exchange was raised. However, the addition of (R)-DTBM-SEGPHOS (10 mol%) to Cu(OTf)2 (10 mol%) shut down the reactivity. It is therefore obvious that the enantioselectivity of the reaction stems from the gold catalyst. Nevertheless, the unexpected activity of copper in this reaction encouraged us to briefly examine the case of chiral ligands located at copper instead of gold, however, the enantioselectivity did not exceed 38% (see Table S1 in the ESI†). We also checked whether a matched pair of chiral complexes could be formed, but this was not the case (see Table S2 of the ESI†).
Since the ee did not exceed 59% with the Au/Cu system, we next investigated the Au–AgOTf catalytic mixture (Table 2). With complex A1 in toluene at 110 °C, the two diastereomers were obtained with 63% and 67% ees (entry 1). As opposed to copper, lowering the temperature to 50 °C improved the ee of the major product which reached 71% (entry 2). A similar enantioselectivity for the major diastereomer was observed in THF or dioxane, although the dr was lower than in toluene (entries 3 and 4). The use of nitromethane as more-polar solvent led to low selectivities (entry 5), which indicates that the proximity of the counterion to the cationic gold center is an important factor in the enantioinduction.22
Table 2 Solvent and gold catalyst screening in the enantioselective hydroalkylation of ene-β-ketoamide 1a using AgOTf

|
Entry |
[Au] |
Solvent |
T (°C) |
Time (h) |
Conva (%) |
dra |
eea,b (%) |
Estimated by chiral SFC; diastereomeric ratios shown are 2a/2a′.
Corresponding to 2a/2a′.
91% isolated yield.
|
1 |
A1
|
Toluene |
110 |
24 |
100 |
66/34 |
63/67 |
2 |
A1
|
Toluene |
50 |
24 |
100 |
78/22 |
71/62 |
3 |
A1
|
THF |
50 |
24 |
100 |
66/34 |
72/25 |
4 |
A1
|
Dioxane |
50 |
24 |
99 |
72/28 |
73/49 |
5 |
A1
|
MeNO2 |
50 |
24 |
49 |
59/41 |
5/18 |
6 |
A2
|
Toluene |
50 |
2 |
98 |
79/21 |
71/59 |
7 |
B1
|
Toluene |
50 |
0.5 |
100 |
80/20 |
24/31 |
8 |
B2
|
Toluene |
50 |
2 |
100 |
82/18 |
44/38 |
9 |
C1
|
Toluene |
50 |
24 |
100c |
43/57 |
82/96 |
10 |
C2
|
Toluene |
50 |
2 |
100 |
74/26 |
65/80 |
11 |
D
|
Toluene |
50 |
2 |
100 |
74/26 |
32/33 |
12 |
E
|
Toluene |
50 |
23 |
53 |
67/33 |
4/5 |
13 |
F
|
Toluene |
50 |
5 |
100 |
71/29 |
4/18 |
15 |
G
|
Toluene |
50 |
4 |
98 |
75/25 |
31/35 |
16 |
H
|
Toluene |
50 |
4 |
97 |
79/21 |
41/3 |
17 |
I
|
Toluene |
50 |
8 |
98 |
79/21 |
16/7 |
18 |
J
|
Toluene |
50 |
2 |
100 |
74/26 |
3/12 |
19 |
K
|
Toluene |
50 |
23 |
80 |
22/78 |
8/5 |
Various chiral mono- and digold complexes were next evaluated (entries 6–19). The chiral gold complexes used in this study are shown in Fig. 1. Compounds A1, A1′,23A2,24B1,25B2,26C1,27C2,28D,29E,23,30G,31H,32I,33 and K34 have been previously described. Complexes F33,35 and J36 are new but structurally close to other reported gold catalyst. With C1, although the diastereoselectivity was low, 2a and 2a′ were obtained with high enantioselectivities of 82 and 96% respectively (entry 9).
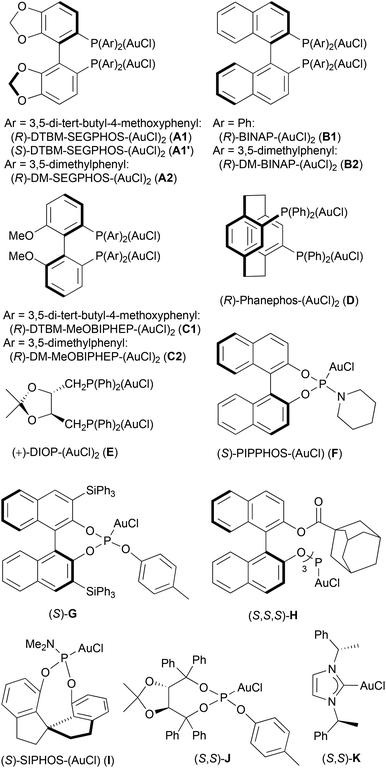 |
| Fig. 1 Chiral gold complexes used in this study. | |
Using the digold complex C1, additional silver salts and other activators were scrutinized (Table 3, the result with AgOTf is reminded in entry 1 for clarity).‡ The conversion reached in the absence of gold is indicated in parentheses. It remained very modest if any.37 A very good dr of 91/9 was achieved with AgBF4 (entry 2). The major diastereomer was obtained in 83% ee in this case. The use of AgPF6, AgSbF6, and AgNTf2 led to lower induction levels (entries 3–5). Pertaining to our research program aimed at developing silver-free two-component approaches in gold catalysis,11 various Lewis acids were tested next. With Cu(OTf)2, 2a was formed in 66% ee and 2a′ in 93% ee (entry 6). Similarly to AgOTf, 2a and 2a′ were formed with low diastereoselectivity but in high ees with In(OTf)3 and Bi(OTf)3 (entries 7 and 8). With TMSOTf, the stereoselectivity was comparable to other metal triflates but full conversion was not reached (entry 9). Other activators such as Zn(OTf)2, Ga(OTf)3, and In(NTf2)3 have also been tested towards the chiral gold complex A1 (see Table S3 of the ESI†), yet none outperformed silver salts.
Table 3 Screening of activators in the enantioselective hydroalkylation of ene-β-ketoamide 1a
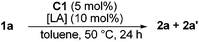
|
Entry |
[LA] |
Conva,b (%) |
dra |
eea,c (%) |
Estimated by chiral SFC; diastereomeric ratios shown are 2a/2a′.
Conversion without gold is indicated in parentheses.
Corresponding to 2a/2a′.
|
1 |
AgOTf |
100 (3) |
43/57 |
82/96 |
2 |
AgBF4 |
100 (3) |
91/9 |
83/2 |
3 |
AgPF6 |
100 (4) |
74/26 |
78/66 |
4 |
AgSbF6 |
100 (5) |
84/16 |
66/14 |
5 |
AgNTf2 |
100 (4) |
88/12 |
68/33 |
6 |
Cu(OTf)2 |
12 (6) |
53/47 |
66/93 |
7 |
In(OTf)3 |
100 (5) |
48/52 |
80/85 |
8 |
Bi(OTf)3 |
100 (9) |
43/57 |
82/92 |
9 |
TMSOTf |
59 (5) |
47/53 |
83/95 |
Table 4 Gold(I)-catalyzed enantioselective hydroalkylation of ene-β-ketoamides 1b–g
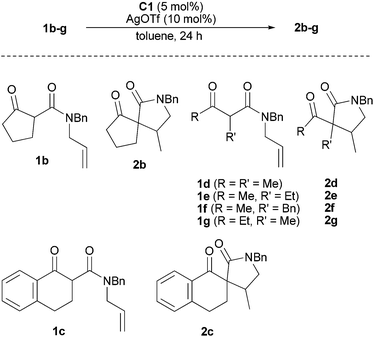
|
Entry |
Substrate |
T (°C) |
Conva (%) |
Yieldb (%) |
dra |
eea (%) |
Estimated by chiral SFC.
Isolated product.
The two diastereomers were separated.
With AgBF4: dr 26/74, ee 55/66, 92% yield.
The two diastereomers were separated, the two methyl groups have a trans relationship in the major one (see ESI).
|
1 |
1b
|
50 |
100 |
90 |
70/30 |
98/72 |
2 |
1c
|
50 |
87 |
|
45/55 |
88/96 |
3 |
1c
|
110 |
100 |
89 |
46/54c |
83/95 |
4 |
1d
|
50 |
94 |
|
46/54 |
93/82 |
5d |
1d
|
110 |
100 |
94 |
49/51e |
90/81 |
6 |
1e
|
50 |
9 |
|
52/48 |
73/62 |
7 |
1e
|
110 |
88 |
74 |
59/41 |
79/86 |
8 |
1f
|
50 |
25 |
|
68/32 |
81/87 |
9 |
1f
|
110 |
95 |
80 |
69/31 |
78/83 |
10 |
1g
|
50 |
29 |
|
55/45 |
90/95 |
11 |
1g
|
110 |
98 |
86 |
53/47 |
85/91 |
Thus, the nature of the major product greatly varies as a function of the Lewis acid. Nevertheless, as far as the enantioselectivity is concerned, it is with AgOTf that the best result was obtained. Focusing on our objective to show that catalytic asymmetric hydroalkylation of unactivated alkenes can be achieved, the C1–AgOTf mixture was used with the ene-β-ketoamides 1b–g (Table 4). We selected malonates displaying a tertiary α-carbon to avoid epimerization of the final product.11a Gratifyingly, 1b transformed into the expected spiro derivative 2b with an excellent ee of 98% for the major diastereomer (entry 1). With 1c, which is the benzo-fused analog of 1a, high ees were also obtained but full conversion was not reached at 50 °C (entry 2). Raising the temperature to 110 °C allowed full conversion without significantly affecting the stereoselectivity (entry 3). The transformation of the acyclic substrates 1d–g furnished the desired products with good to high ees, even at 110 °C which was a required temperature to achieve high conversions (entries 4–11). In each case, the products could be isolated in good to high yields. As for the transformation of 1a, the diastereoselectivity remained moderate to low. It could be improved from 49/51 to 26/74 by using AgBF4 instead of AgOTf (entry 5), but this was at the expense of the enantioselectivity which became 55/66 instead of 90/81.
Even though it is now well-established that both the ligand and the counterion influence the selectivity in Au(I)-catalysis, it is not yet possible to establish a clear structure–activity relationship.38 Nevertheless, a plausible mechanism can be summarized as follows (Scheme 4). According to our study in the Au/Cu catalytic system,11a we suppose that all of the activators used in this study are able to promote chloride abstraction from the gold precatalyst. The C–C bond formation likely involves the coordination of the alkene by the cationic gold π-acid catalyst,39 triggering an anti or syn nucleophilic attack40 of the malonate activated in the form of a metal enolate or an enol ether.41,42 The 5-exo trig cyclization gives rise to a σ-gold complex that would finally undergo protodemetallation with YH. As shown above, the aptitude of the counterion Y− to remain close to the “spectator” gold atom and serve as proton shuttle43 is a likely determining factor.
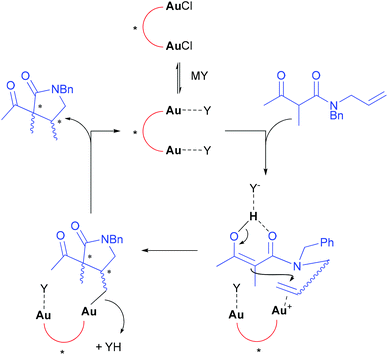 |
| Scheme 4 Mechanistic rationale (Y = counterion). | |
Conclusions
This study has shown for the first time the feasibility of asymmetric hydroalkylation of unactivated alkenes, a synthetic approach which avoids the use of activating groups and their subsequent removal. Enantioselective transformations of ene-β-ketoamides into 2-azaspirodiones and 3-acetylpyrrolidin-2-ones have been accomplished by means of gold catalysis. High enantioselectivities have been reached by using (R)-DTBM-MeOBIPHEP-(AuCl)2 and various triflates such as AgOTf, In(OTf)3, and Bi(OTf)3.
We are grateful for the financial support from ANR JCJC HAONA, UPS, CNRS, the Chinese Scholarship Council, and IUF. We are also thankful to Arnaud Voituriez and Angela Marinetti (ICSN) for a gift of a chiral gold complex. Dedicated to Prof. Max Malacria on the occasion of his 65th birthday.
Notes and references
- Pronucleophiles are compounds that are prone to form nucleophilic species upon treatment with a base or a metal.
-
(a) T. Lechel, F. Pfrengle, H.-U. Reissig and R. Zimmer, ChemCatChem, 2013, 5, 2100 CrossRef CAS;
(b) N. T. Patil, R. D. Kavthe and V. S. Shinde, Tetrahedron, 2012, 68, 8079 CrossRef CAS PubMed;
(c) C. Liu, C. F. Bender, X. Han and R. A. Widenhoefer, Chem. Commun., 2007, 3607 RSC;
(d) G. Balme, D. Bouyssi and N. Monteiro, Pure Appl. Chem., 2006, 78, 231 CrossRef CAS;
(e) Y. Yamamoto and U. Radhakrishnan, Chem. Soc. Rev., 1999, 28, 199 RSC.
- For enantioselective Conia-ene reactions, see:
(a) F. Sladojevich, Á. L. Fuentes de Arriba, I. Ortín, T. Yang, A. Ferrali, R. S. Paton and D. J. Dixon, Chem. – Eur. J., 2013, 19, 14286 CrossRef CAS PubMed;
(b) A. Matsuzawa, T. Mashiko, N. Kumagai and M. Shibasaki, Angew. Chem., Int. Ed., 2011, 50, 7616 CrossRef CAS PubMed;
(c) T. Yang, A. Ferrali, F. Sladojevich, L. Campbell and D. J. Dixon, J. Am. Chem. Soc., 2009, 131, 9140 CrossRef CAS PubMed;
(d) B. K. Corkey and F. D. Toste, J. Am. Chem. Soc., 2005, 127, 17168 CrossRef CAS PubMed;
(e) I. Kadota, A. Shibuya, Y. S. Gyoung and Y. Yamamoto, J. Am. Chem. Soc., 1998, 120, 10262 CrossRef CAS.
- For enantioselective hydroalkylation of allenes, see:
(a) M. L. Meiling, S. Datta, D. M. Barber and D. J. Dixon, Org. Lett., 2012, 14, 6350 CrossRef PubMed;
(b) B. M. Trost, J. Xie and J. D. Sieber, J. Am. Chem. Soc., 2011, 133, 20611 CrossRef CAS PubMed;
(c) B. M. Trost, A. B. C. Simas, B. Plietker, C. Jäkel and J. Xie, Chem. – Eur. J., 2005, 11, 7075 CrossRef CAS PubMed;
(d) B. M. Trost, C. Jäkel and B. Plietker, J. Am. Chem. Soc., 2003, 125, 4438 CrossRef CAS PubMed.
- For enantioselective hydroarylation of allenes, see:
(a) H. Teller, M. Corbet, L. Mantilli, G. Gopakumar, R. Goddard, W. Thiel and A. Fürstner, J. Am. Chem. Soc., 2012, 134, 15331 CrossRef CAS PubMed;
(b) M.-Z. Wang, C.-Y. Zhou, Z. Guo, E. L.-M. Wong, M.-K. Wong and C.-M. Che, Chem. – Asian J., 2011, 6, 812 CrossRef CAS PubMed;
(c) C. Liu and R. A. Widenhoefer, Org. Lett., 2007, 9, 1935 CrossRef CAS PubMed.
-
R. A. Widenhoefer, in Catalytic Asymmetric Friedel–Crafts Alkylations, ed. M. Bandini and A. Umani-Ronchi, Wiley-VHC, 2009, ch. 6 Search PubMed.
- For reviews, see:
(a) J. Christoffers and A. Baro, Angew. Chem., Int. Ed., 2003, 42, 1688 CrossRef CAS PubMed;
(b) S. Matsunaga, T. Ohshima and M. Shibasaki, Adv. Synth. Catal., 2002, 344, 3 CrossRef CAS For selected examples, see:
(c) Y.-Q. Yang, Z. Chai, H.-F. Wang, X.-K. Chen, H.-F. Cui, C.-W. Zheng, H. Xiao, P. Li and G. Zhao, Chem. – Eur. J., 2009, 15, 13295 CrossRef CAS PubMed;
(d) H. Li, J. Song, X. Liu and L. Deng, J. Am. Chem. Soc., 2005, 127, 8948 CrossRef CAS PubMed;
(e) T. Ooi, D. Ohara, K. Fukumoto and K. Maruoka, Org. Lett., 2005, 7, 3195 CrossRef CAS PubMed;
(f) M. T. Hechavarria Fonseca and B. List, Angew. Chem., Int. Ed., 2004, 43, 3958 CrossRef CAS PubMed;
(g) Y. Hamashima, D. Hotta and M. Sodeoka, J. Am. Chem. Soc., 2002, 124, 11240 CrossRef CAS PubMed.
-
(a) F. Dénès, A. Pérez-Luna and F. Chemla, Chem. Rev., 2010, 110, 2366 CrossRef PubMed;
(b) L. F. Tietze, H. Ila and H. P. Bell, Chem. Rev., 2004, 104, 3453 CrossRef CAS PubMed.
-
(a) A. Pérez-Luna, C. Botuha, F. Ferreira and F. Chemla, New J. Chem., 2008, 32, 594 RSC;
(b) I. Marek, J. Chem. Soc., Perkin Trans. 1, 1999, 535 RSC.
-
(a) X. Han and R. A. Widenhoefer, Org. Lett., 2006, 8, 3801 CrossRef CAS PubMed;
(b) C. Liu, X. Han, X. Wang and R. A. Widenhoefer, J. Am. Chem. Soc., 2004, 126, 3700 CrossRef CAS PubMed;
(c) R. K. Thalji, J. A. Ellman and R. G. Bergman, J. Am. Chem. Soc., 2004, 126, 7192 CrossRef CAS PubMed.
-
(a) A. Guérinot, W. Fang, M. Sircoglou, C. Bour, S. Bezzenine-Lafollée and V. Gandon, Angew. Chem., Int. Ed., 2013, 52, 5848 CrossRef PubMed;
(b) W. Fang, M. Presset, A. Guérinot, C. Bour, S. Bezzenine-Lafollée and V. Gandon, Chem. – Eur. J., 2014, 20, 5439 CrossRef CAS PubMed.
- For examples of gold-catalyzed hydroalkylation of unactivated alkenes with pronucleophiles leading to achiral or racemic products, see:
(a) Y.-P. Xiao, X.-Y. Liu and C.-M. Che, Angew. Chem., Int. Ed., 2011, 50, 4937 CrossRef CAS PubMed;
(b) C.-Y. Zhou and C.-M. Che, J. Am. Chem. Soc., 2007, 129, 5828 CrossRef CAS PubMed;
(c) R.-V. Nguyen, X.-Q. Yao, D. S. Bohle and C.-J. Li, Org. Lett., 2005, 7, 673 CrossRef CAS PubMed;
(d) X. Yao and C.-J. Li, J. Am. Chem. Soc., 2004, 126, 6884 CrossRef CAS PubMed.
- For some reviews on enantioselective gold catalysis, see:
(a)
N. Huguet and A. M. Echavarren, in Asymmetric Synthesis II: More Methods and Applications, ed. M. Christmann and S. Bräse, Wiley-VCH, 2013, ch. 26 Search PubMed;
(b) Y.-M. Wang, A. D. Lackner and F. D. Toste, Acc. Chem. Res., 2014, 47, 889 CrossRef CAS PubMed;
(c) A. Marinetti, H. Jullien and A. Voituriez, Chem. Soc. Rev., 2012, 41, 4884 RSC;
(d)
F. D. Toste and M. J. Campbell, in Comprehensive Chirality, ed. H. Yamamoto and E. Carreira, Elsevier, 2012, ch. 4.20, vol. 4 Search PubMed;
(e) A. Pradal, P. Y. Toullec and V. Michelet, Synthesis, 2011, 1501 CAS;
(f) S. Sengupta and X. Shi, ChemCatChem, 2010, 2, 609 CrossRef CAS;
(g) D. J. Gorin, B. D. Sherry and F. D. Toste, Chem. Rev., 2008, 108, 3351 CrossRef CAS PubMed;
(h) R. A. Widenhoefer, Chem. – Eur. J., 2008, 14, 5382 CrossRef CAS PubMed;
(i) H. C. Shen, Tetrahedron, 2008, 64, 3885 CrossRef CAS PubMed;
(j) Z. Li, C. Brouwer and C. He, Chem. Rev., 2008, 108, 3239 CrossRef CAS PubMed.
- For a formal catalytic enantioselective hydroalkylation of unactivated olefins which involves a hidden Michael addition, see: A. Quintard, T. Constancieux and J. Rodriguez, Angew. Chem., Int. Ed., 2013, 52, 12883 CrossRef CAS PubMed.
- In the minor 2a′, the 1H NMR signal of the methyl group is shielded by the ketone functionality:
(a) J. Cossy, A. Bouzide and C. Leblanc, J. Org. Chem, 2000, 65, 7257 CrossRef CAS PubMed;
(b) J. Cossy and A. Bouzide, Tetrahedron, 1997, 53, 5775 CrossRef CAS.
- On the non-innocent role of silver in gold-catalysis, see:
(a) A. Homs, I. Escofet and A. M. Echavarren, Org. Lett., 2013, 15, 5782 CrossRef CAS PubMed;
(b) Y. Zhu, C. S. Day, L. Zhang, K. J. Hauser and A. C. Jones, Chem. – Eur. J., 2013, 19, 12264 CrossRef CAS PubMed;
(c) D. Wang, R. Cai, S. Sharma, J. Jirak, S. K. Thummanapelli, N. G. Akhmedov, H. Zhang, X. Liu, J. L. Petersen and X. Shi, J. Am. Chem. Soc., 2012, 134, 9012 CrossRef CAS PubMed;
(d) H. Schmidbaur and A. Schier, Z. Naturforsch., 2011, 66b, 329 CrossRef PubMed;
(e) D. Weber and M. R. Gagné, Org. Lett., 2009, 11, 4962 CrossRef CAS PubMed.
- For a review on catalytic activation of olefins by metal triflates, see: G. Lemière and E. Duñach, Chem. – Eur. J., 2013, 19, 3270 CrossRef PubMed.
- Molecular gold clusters can be sometimes extremely active, see inter alia:
(a) J. Oliver-Meseguer, A. Leyva-Pérez and A. Corma, ChemCatChem, 2013, 5, 3509 CrossRef CAS;
(b) J. Oliver-Meseguer, J. R. Cabrero-Antonino, I. Domínguez, A. Leyva-Pérez and A. Corma, Science, 2012, 338, 1452 CrossRef CAS PubMed.
- The 2-azaspirodione core is present in a number of natural products, see inter alia:
(a) C.-J. Zheng, C.-L. Shao, L.-Y. Wu, M. Chen, K.-L. Wang, D.-L. Zhao, X.-P. Sun, G.-Y. Chen and C.-Y. Wang, Mar. Drugs, 2013, 11, 2054 CrossRef PubMed;
(b) J. Xu, E. J. E. Caro-Diaz, L. Trzoss and E. A. Theodorakis, J. Am. Chem. Soc., 2012, 134, 5072 CrossRef CAS PubMed;
(c) J. Deng, B. Zhu, Z. Lu, H. Yu and A. Li, J. Am. Chem. Soc., 2012, 134, 920 CrossRef CAS PubMed;
(d) G. Ding, H. Wang, L. Li, A. Juan Chen, L. Chen, H. Chen, H. Zhang, X. Liu and Z. Zou, Eur. J. Org. Chem., 2012, 2516 CrossRef CAS;
(e) S. M. Canham, L. E. Overman and P. S. Tanis, Tetrahedron, 2011, 67, 9837 CrossRef CAS PubMed;
(f) J.-H. Jang, Y. Asami, J.-P. Jang, S.-O. Kim, D. O. Moon, K.-S. Shin, D. Hashizume, M. Muroi, T. Saito, H. Oh, B. Y. Kim, H. Osada and J. S. Ahn, J. Am. Chem. Soc., 2011, 133, 6865 CrossRef CAS PubMed;
(g) Z. Lin, T. Zhu, H. Wei, G. Zhang, H. Wang and Q. Gu, Eur. J. Org. Chem., 2009, 3045 CrossRef CAS;
(h) Y. Shiono, K. Shimanuki, F. Hiramatsu, T. Koseki, M. Tetsuya, N. Fujisawa and K.-i. Kimura, Bioorg. Med. Chem. Lett., 2008, 18, 6050 CrossRef CAS PubMed;
(i) P. de A. Leone, A. R. Carroll, L. Towerzey, G. King, B. M. McArdle, G. Kern, S. Fisher, J. N. A. Hooper and R. J. Quinn, Org. Lett., 2008, 10, 2585 CrossRef PubMed;
(j) N. Phonkerd, S. Kanokmedhakul, K. Kanokmedhakul, K. Soytong, S. Prabpai and P. Kongsearee, Tetrahedron, 2008, 64, 9636 CrossRef CAS PubMed.
- For copper-catalyzed cycloisomerization reactions, see:
(a) C. Fehr, M. Vuagnoux, A. Buzas, J. Arpagaus and H. Sommer, Chem. – Eur. J., 2011, 17, 6214 CrossRef CAS PubMed;
(b) V. Rauniyar, Z. J. Wang, H. E. Burks and F. D. Toste, J. Am. Chem. Soc., 2011, 133, 8486 CrossRef CAS PubMed;
(c) C. Fehr, B. Winter and I. Magpantay, Chem. – Eur. J., 2009, 15, 9773 CrossRef CAS PubMed.
- For Cu(OTf)2-catalyzed intermolecular addition of activated methylene compounds to alkenes, see:
(a) Y. Li, Z. Yu and S. Wu, J. Org. Chem., 2008, 73, 5647 CrossRef CAS PubMed;
(b) H. E. Lanman, R.-V. Nguyen, X. Yao, T.-H. Chan and C.-J. Li, J. Mol. Catal. A: Chem., 2008, 279, 218 CrossRef CAS PubMed.
- G. L. Hamilton, E. J. Kang, M. Mba and F. D. Toste, Science, 2007, 317, 496 CrossRef CAS PubMed.
- M. J. Johansson, D. J. Gorin, S. T. Staben and F. D. Toste, J. Am. Chem. Soc., 2005, 127, 18002 CrossRef CAS PubMed.
- D. Vasu and R. Liu, Chem. – Eur. J., 2012, 18, 13638 CrossRef CAS PubMed.
- M. P. Muñoz, J. Adrio, J. C. Carretero and A. M. Echavarren, Organometallics, 2005, 24, 1293 CrossRef.
- G. L. Lalonde, B. D. Sherry, E. J. Kang and F. D. Toste, J. Am. Chem. Soc., 2007, 129, 2452 CrossRef PubMed.
- Z. Zhang, C. F. Bender and R. A. Widenhoefer, J. Am. Chem. Soc., 2007, 129, 14148 CrossRef CAS PubMed.
- P. García-García, A. Martínez, A. M. Sanjuán, M. A. Fernández-Rodríguez and R. Sanz, Org. Lett., 2012, 14, 4970 CrossRef PubMed.
-
(a) A. Pradal, C.-M. Chao, M. R. Vitale, P. Y. Tosnullec and V. Michelet, Tetrahedron, 2011, 67, 4371 CrossRef CAS PubMed;
(b) C. Sarcher, A. Lühl, F. C. Falk, S. Lebedkin, M. Kühn, C. Wang, J. Paradies, M. M. Kappes, W. Klopper and P. W. Roesky, Eur. J. Inorg. Chem., 2012, 5033 CrossRef CAS.
- A. Arnanz, C. González-Arellano, A. Juan, G. Villaverde, A. Corma, M. Iglesias and F. Sánchez, Chem. Commun., 2010, 46, 3001 RSC.
- N. Delpont, I. Escofet, P. Pérez-Galán, D. Spiegl, M. Raducan, C. Bour, R. Sinisi and A. M. Echavarren, Catal. Sci. Technol., 2013, 3, 3007 CAS.
- A. Z. González and F. D. Toste, Org. Lett., 2010, 12, 200 CrossRef PubMed.
- A. Z. González, D. Benitez, E. Tkatchouk, W. A. Goddard III and F. D. Toste, J. Am. Chem. Soc., 2011, 133, 5500 CrossRef PubMed.
- B. W. Gung, L. N. Bailey, D. T. Craft, C. L. Barnes and K. Kirschbaum, Organometallics, 2010, 29, 3450 CrossRef CAS.
- I. Alonso, B. Trillo, F. López, S. Montserrat, G. Ujaque, L. Castedo, A. Lledós and J. L. Mascareñas, J. Am. Chem. Soc., 2009, 131, 13020 CrossRef CAS PubMed.
- H. Teller, S. Flügge, R. Goddard and A. Fürstner, Angew. Chem., Int. Ed., 2010, 49, 1949 CrossRef CAS PubMed.
- For Lewis and Brønsted acid catalyzed intermolecular addition of activated methylene compounds to alkenes, see:
(a) Cu: ref. 21; ;
(b) Ag: Y. Yao and C.-J. Li, J. Org. Chem., 2005, 70, 5752 CrossRef PubMed;
(c) Ga: R.-V. Nguyen and C.-J. Li, J. Am. Chem. Soc., 2005, 127, 17184 CrossRef CAS PubMed;
(d) Bi: M. Rueping, B. J. Nachtsheim and A. Kuenkel, Synlett, 2007, 1391 CrossRef CAS PubMed;
(e) H+: P. N. Liu, L. Dang, Q. W. Wang, S. L. Zhao, F. Xia, Y. J. Ren, X. Q. Gong and J. Q. Chen, J. Org. Chem., 2010, 75, 5017 CrossRef CAS PubMed.
- D. Zuccaccia, L. Belpassi, A. Macchioni and F. Tarantelli, Eur. J. Inorg. Chem., 2013, 4121 CrossRef CAS.
- R. E. M. Brooner and R. A. Widenhoefer, Angew. Chem., Int. Ed., 2013, 52, 11714 CrossRef CAS PubMed.
- For a discussion on enantioselective hydroamination of alkenes by digold complexes, see: J. H. Kim, S.-W. Park, S. R. Park, S. Lee and E. J. Kang, Chem. – Asian J., 2011, 6, 1982 CrossRef CAS PubMed.
- In the case of Cu(OTf)2, the formation of a copper enolate was observed by 1H NMR spectroscopy, see ref. 11.
- For other bimetallic activation of ene-dicarbonyl compounds leading to achiral or racemic products, see:
(a) R. W. Coscia and T. H. Lambert, J. Am. Chem. Soc., 2009, 131, 2496 CrossRef CAS PubMed;
(b) C. Liu and R. A. Widenhoefer, Tetrahedron Lett., 2005, 46, 285 CrossRef CAS PubMed;
(c) X. Han, X. Wang, T. Pei and R. A. Widenhoefer, Chem. – Eur. J., 2004, 10, 6333 CrossRef CAS PubMed.
-
(a) M. Bandini, A. Bottoni, M. Chiarucci, G. Cera and G. P. Miscione, J. Am. Chem. Soc., 2012, 134, 20690 CrossRef CAS PubMed;
(b) J. H. Kim, S. W. Park, S. R. Park, S. Lee and E. J. Kang, Chem. – Asian J., 2011, 6, 1982 CrossRef CAS PubMed;
(c) J. Zhang, W. Shen, L. Li and M. Li, Organometallics, 2009, 28, 3129 CrossRef CAS;
(d) G. Kovács, G. Ujaque and A. Lledós, J. Am. Chem. Soc., 2008, 130, 853 CrossRef PubMed;
(e) Y. Xia, A. S. Dubnik, V. Gevorgyan and Y. Li, J. Am. Chem. Soc., 2008, 130, 6940 CrossRef CAS PubMed.
Footnotes |
† Electronic supplementary information (ESI) available: Experimental details and characterizations. See DOI: 10.1039/c4qo00112e |
‡ General procedure pertaining to Table 3, entry 1, and Table 4: A 10 mL oven-dried tube equipped with a Teflon-coated magnetic stir bar was charged with C1 (8.2 mg, 0.005 mmol), silver(I) triflate (2.6 mg, 0.010 mmol), and toluene (0.5 mL) and the mixture was stirred at room temperature for 1 min. The substrate (0.10 mmol) in toluene (0.5 mL) was added and the tube was sealed with a plastic stopper. The tube was covered by aluminum foil and immersed and stirred in a preheated oil bath at the indicated temperature for 24 h. Then, the reaction mixture was filtered through a short pad of silica gel which was rinsed with diethyl ether. After evaporation, the residue was purified by chromatography on silica gel to afford the desired product. |
|
This journal is © the Partner Organisations 2014 |
Click here to see how this site uses Cookies. View our privacy policy here.