DOI:
10.1039/D4EN00854E
(Perspective)
Environ. Sci.: Nano, 2025,
12, 1801-1808
Tailoring nanomaterials towards global One Health: a promising nano-strategy against antibiotic resistance
Received
13th September 2024
, Accepted 20th January 2025
First published on 25th January 2025
Abstract
The management of antibiotic resistance gene (ARG) contamination in soil–plant systems is a critical area of research with significant implications for public health and environmental sustainability. Recently, engineered nanomaterials (ENMs) have been developed to enhance plant growth and address the global food crisis. Studies on the effects of nanomaterials mostly indicate an increase in the spread of antibiotic resistance, while emerging findings reveal the potential of ENMs in mitigating ARG pollution. Unlike existing mechanisms such as adsorption, DNA damage, and microbial disinfection involved in ARG removal, ENMs are specifically modified (e.g., with particular chemical compositions or surface charge adjustment) to inhibit the transfer of ARGs and migration of antibiotic-resistant bacteria. The integration of ENMs with advanced technologies (e.g., CRISPR gene editing) holds great promise for remediating antibiotic resistance in soil–plant systems. Here, we provide an overview of ENM–ARG interactions and propose applications of tailored ENMs to inhibit ARG dissemination during the development of nano-enabled agriculture, addressing major challenges and directions for optimizing the efficacy and safety of ENM-based strategies for mitigating ARG contamination in agriculture.
Environmental significance
Nano-enabled agriculture is currently undergoing intensive investigation for the sustainable development of the environment and human health. The emergence of antibiotic resistance in agricultural systems has become a major concern due to its potential threats to global public health. While nanomaterials have been found to promote the spread of antibiotic resistance genes (ARGs), modifications of nanomaterials offer opportunities for effectively combating the increasing antibiotic resistance in a sustainable manner. A comprehensive understanding of the interactions between nanomaterials used in agriculture and ARGs associated with soil–plant systems, and their impacts on ecosystems will greatly contribute to the advancement of nano-enabled agriculture towards achieving global One Health.
|
Introduction
The extensive use of antibiotics in human healthcare and livestock production leads to the accumulation of antibiotic residues in animal manure and wastewater.1,2 The presence of these antibiotics significantly increases the prevalence of antibiotic-resistant bacteria (ARB) and antibiotic resistance genes (ARGs) in animal manure and wastewater.1 Existing wastewater treatment plants are not specifically designed to eliminate antibiotics and ARGs, and agricultural practices involving the application of ARB and ARG-contaminated manure, wastewater, and sewage sludge as soil amendments and fertilizers promote the horizontal transfer of ARGs to native bacteria and potentially to human pathogens in the soil. As a result, ARGs can spread from soil microbiota to plant microbiota, facilitating the transmission of antibiotic resistance through the food chain. This process contributes to the emergence of antibiotic resistance and poses a substantial threat to public health, thereby necessitating the development of innovative strategies to address this critical challenge from a “One Health” perspective that considers the interconnections between human, animal, and environmental microbiota.1,3
Remediation efforts targeting ARG pollution have predominantly focused on eliminating ARGs from wastewater through treatments such as bioremediation, advanced oxidation processes, adsorption, disinfection, and membrane bioreactors.4 Microbial disinfection, sorption and DNA fragmentation are the primary mechanisms for the removal of ARGs. However, practical applications face challenges and limitations including: 1) the high cost, time-consuming, and high dosage requirements (e.g., disinfectants, ozone) needed to effectively inactivate antibiotic-resistant bacteria (ARB)/ARGs; 2) low removal efficiency in complex environments (e.g., unable to target all types of ARGs, increased presence of transposon genes, small size of ARGs that cannot be intercepted by membranes); 3) formation of toxic by-products/transformation products such as bromate during ozone disinfection; 4) regeneration/recovery of ARB/ARGs and development of resistant strains; 5) side effects such as catalyst toxicity; 6) formation of new reservoirs for ARGs (e.g., ARG-containing sludge or substrate of constructed wetlands).4–9 Novel strategies are thus urgently needed to intervene in the spread and persistence of ARGs across various environmental compartments. The application of innovative materials, such as carbon-based materials (e.g., biochar) has been promoted for combating antibiotic resistance in the environment through passivation, adsorption, and interactions with ARG-containing DNA or bacterial cells. Biochar can directly adsorb free plasmids and immobilize bacteria cells, and can even trigger intramolecular plasmid condensation and DNA deactivation, thereby inhibiting the dissemination of ARGs.10 However, certain challenges remain unresolved, including the potential release of endogenous pollutants (such as PAHs, PCBs, PTEs) from carbon-based materials, and biochar may even become new carriers of ARGs and pollutants.10,11 Therefore, it is crucial to understand the influencing factors and associated potential environmental risks in order to develop effective management strategies.
The advent of nanotechnology has opened new avenues for addressing antibiotic resistance through the development of engineered nanomaterials (ENMs). These promising ENMs exhibit antibacterial properties and can potentially inhibit the horizontal gene transfer (HGT) of ARGs. However, studies have revealed that certain nanomaterials (NMs) may actually stimulate the HGT of ARGs, necessitating further investigation into the underlying mechanisms responsible for the inhibitory effect of ENMs to guide their future development. The prevalence and dissemination of antibiotic resistance in soil–plant systems have raised critical concerns regarding public health during fresh food digestion.12 Notably, ENMs have been intensively studied in recent research as promising candidates for sustainable agriculture and ensuring food safety, improving plant growth and resistance to pathogens and pests.13 However, the potential impacts of these beneficial effects on the spread of ARGs remain largely unknown. This perspective discusses situations where ENMs may promote antibiotic resistance, and more importantly addresses the potential of nanomaterial-based strategies in the context of One Health for combating antibiotic resistance, highlighting the diverse applications of ENMs in environmental remediation and agriculture.
Alteration of HGT processes by nanomaterials and their potential inhibitory effects
It is known that the dissemination of antibiotic resistance is predominantly attributed to the increased occurrence of HGT and ARB.12 HGT is recognized as the primary mechanism for the transfer of ARGs, utilizing mobile genetic elements (MGEs) such as transposons and integrons as vectors. Transposons are mobile DNA elements located on plasmids, while integrons can capture genes and stimulate their expression.1,14 ARGs are classified into intracellular ARGs (iARGs) and extracellular ARGs (eARGs). iARGs are intracellular DNA that can promote the development of ARB through conjugation involving direct cell-to-cell contact, while eARGs are extracellular DNA which can be acquired by competent non-resistant bacteria via transformation, thus contributing to the propagation of antibiotic resistance.15 Conjugation and transformation are the most commonly observed HGT mechanisms responsible for the dissemination of plasmids carrying ARGs (Fig. 1).16 Oxidative stress resulting from overaccumulation of reactive oxygen species (ROS) facilitates the development of antimicrobial resistance. ROS are key signaling molecules which enhance membrane permeability and trigger the SOS response, thereby promoting HGT of ARGs.17 Activation of the SOS response can induce HGT mechanisms (e.g., conjugation and transformation) by upregulating gene expression involved in plasmid transfer and the uptake of ARGs by competent strains. Furthermore, ATP plays a critical role in the conjugation process by providing the necessary energy for DNA transfer and facilitating the morphogenesis of conjugative machinery.17,18 Numerous studies have demonstrated that NMs (such as CuO NMs, Ag NMs, TiO2 NMs, ZnO NMs, and nanofullerene) can stimulate HGT predominantly through elevated SOS response and generation of ROS; moreover, NM exposure resulted in enhanced cell membrane permeability and up-regulated expression of HGT-related genes, contributing to plasmid mobility and formation of transfer channels.19–22 Metal-based NMs are extensively studied due to their potential role in promoting antibiotic resistance, and ion release is one of the major concerns regarding the effects of metal-based NMs. Both the “nano-effect” and “ion effect” have been identified as contributing factors to the altered ARG proliferation. For instance, Ag NMs tend to aggregate with soil particles due to their large specific surface area, reducing their bioavailability; however, Ag ions significantly increase ARG abundance in soil and pose a stronger impact on ARGs compared to Ag NMs.23 In contrast, CuO NMs exhibited a higher frequency of conjugative transfer compared to Cu2+ ions due to the antimicrobial capacity displayed by Cu2+ at relatively higher concentrations (from 10–100 μmol L−1).19 Unlike the conjugation process, both Ag NMs (1 mg L−1) and CuO NMs (10 mg L−1) showed an increased transformation frequency compared to their ion counterparts. This enhanced transformation of ARGs was attributed to membrane damage resulting from the overproduction of ROS induced by the binding of nanosized Ag and CuO to the Mg2+ on the bacterial surface.21 The nano-specific effect was also observed for ZnO NMs, while no significant alteration was observed upon exposure to Zn2+ ions.24 The increase in transformation induced by ZnO NMs was attributed to stimulated SOS response and ATP synthesis.21 Interestingly, Al2O3 NMs, rather than their bulk counterparts, can bind to plasmids encoding ARGs and serve as delivery vectors for the entrance of plasmids into bacterial cells, promoting ARG transformation while preventing DNA from endonuclease cleavage,25 contributing to the persistence and transfer of ARGs.
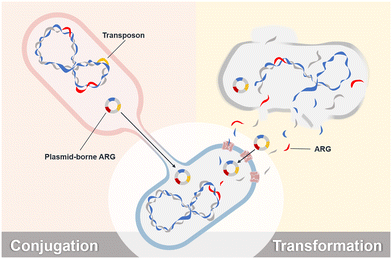 |
| Fig. 1 Conjugation and transformation processes of HGT. | |
Although the contributions of the “nano-effect” and “ion effect” may vary, the “ion effect” mainly leads to increased ROS production and antimicrobial growth, while the “nano-effect” generally plays a diverse and potentially inhibitory role in the ARG abundance. In contrast to metal-based NMs, nanosized biochar (nano-BC) induces a substantial reduction in ARG abundance, while this capability is absent in bulk biochar due to steric hindrance that hinders contact with ARGs.26 Additionally, the effects of size and concentration also contribute to the altered HGT processes. NMs with a size <100 nm demonstrated enhanced efficacy in facilitating plasmid transformation compared to their ionic counterparts, as they can be internalized by bacteria through endocytosis.21 Low concentrations of NMs (e.g., 0.2–30 mg L−1 ZnO NMs) significantly increased ARG abundance by increasing ARB abundance or promoting biofilm formation for HGT,27,28 whereas high concentrations (e.g., ZnO NMs >30 mg L−1, graphene oxide nanosheets/graphene quantum dots >10 mg L−1) hindered biofilm formation and impeded the adhesion of donor and recipient cells or even damaged the antibiotic resistant plasmids.21,27,29,30 Generally, high concentrations (e.g., >100 ppm) of metal-based NMs can reduce the HGT frequencies due to their bactericidal effects.21 However, from a practical standpoint, higher concentrations imply a substantial introduction of NMs into the environment that may lead to unintended side-effects. Considering that released ions might partially contribute to the promotion of ARGs, inert NMs without ion dissolution are preferable for mitigating environmental antibiotic resistance.
Engineering NMs for the regulation of antibiotic resistance
The ARG controlling potential of NMs has primarily been attributed to their ability to adsorb ARGs, induce DNA damage, and reduce the diversity and richness of ARB.31 Electrostatic interactions, π-stacking interactions, H-bonding, and covalent conjugation enable the adsorption of extracellular DNA (e.g., extracellular plasmid-borne ARGs) onto ENMs (such as GO – graphene oxide nanosheets, nTiO2, and nano-biochar),26,32,33 largely reducing the mobility of free ARGs. It should be noteworthy that release/regrowth of ARGs/ARB might still occur after the successful adsorption, and most ENMs are not selective for bacterial removal,34 indicating that the survival of non-target microorganisms might be threatened in the presence of ENMs. To mitigate the release of adsorbed ARGs from nano-biochar, elevating the pyrolysis temperature (up to 700 °C) demonstrated irreversible and more efficient adsorption of ARGs.35 However, high-temperature synthesis may not be the optimal choice for large-scale applications. Additionally, ENMs such as zero-valent iron (nZVI) can easily undergo passivation and aggregation, leading to a decrease in the ROS yield and bioavailability for controlling ARGs.36 Moreover, the underlying mechanism regarding the impact of nZVI on the ARB community remains to be further clarified. It appears that both adsorption and antibacterial capacity could result in undesired and uncontrolled consequences. In comparison, inhibiting HGT of ARGs may enhance control efficiency while reducing side effects (Fig. 2). Furthermore, maintaining the functionality of ENMs for continuous removal of ARGs/ARB would contribute to sustainable remediation of ARG contamination.
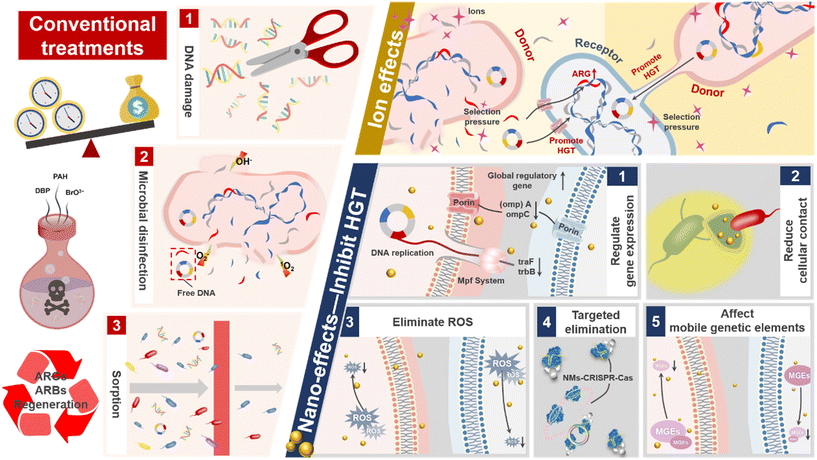 |
| Fig. 2 Mechanisms underlying conventional treatments and ENMs in controlling ARB and the transfer of ARGs. Conventional treatments (depicted on the left side), ion effects (upper right), and nano-effects (lower right) are presented with a light red, yellow, and gray background, respectively. | |
Modification of ENMs has been proposed to facilitate the development of environmentally friendly ENMs for combating the spread of ARGs.37 Modification of nZVI with sulfide helps to improve the antibacterial capacity of nZVI by constructing a more efficient electron transport stream.36 Notably, sulfidation enhances the stability of iron nanoparticles, reducing the release of Fe2+ ions and decreasing the toxicity of nZVI towards bacteria,36,38 thereby elevating the nano-effect while mitigating the ion-effect on antibiotic resistance. Compositing Fe3O4 with MoS2 (Fe3O4@MoS2 nanocomposite) not only eliminates the HGT-promoting effect of Fe3O4, but also strongly inhibits HGT by promoting the expression of the global regulatory gene and suppressing the expressions of conjugative transfer genes involved in mating pair formation (Mpf), DNA replication, and porins.37 The Mpf system functions as a secretion machinery for DNA transfer during conjugation,39 and the suppressed expression of the Mpf system indicates a restricted ARG transfer between bacteria. Importantly, Fe3O4 nanoparticles embedded within MoS2 sheets (<100 mg L−1) exhibited minimal toxicity and TEM images revealed that the Fe3O4@MoS2 composite remained outside of the cells, potentially preventing cellular contact for conjugative transfer.37 Although ENMs are not internalized into bacterial cells, it is crucial to investigate their accumulation in the environment and consider their long-term impacts after application.
Overaccumulation of ROS is known as the primary driving force that initiates the HGT of ARGs. Conversely, elimination of ROS has been proven to effectively inhibit the conjugative transfer of ARGs.40 Nano-cerium oxide (nCeO2), known for its remarkable capacity to scavenge ROS due to the presence of oxygen vacancies, possesses unique redox chemistry. By modifying the surface valence states of nCeO2, such as achieving a Ce3+/Ce4+ ratio of around 30%, nCeO2 exhibits an effective and sustainable ability to eliminate ROS while maintaining low biotoxicity levels.41 Emerging evidence suggests that nCeO2 has great potential in inhibiting both conjugation and transformation processes of ARGs through the elimination of ROS, reduction in plasmid availability, and down-regulation of genes associated with HGT.17,18 Combined application of nanomaterials and microorganisms or more effective techniques can further optimize the reduction of ARG abundances. For example, the combined application of nano-biochar and Bacillus cereus in a soil–lettuce system demonstrated superior performance compared to individual applications, as the addition of Bacillus cereus affected the bacterial community and mobile genetic elements, resulting in more efficient suppression of ARG proliferation in lettuce.42 Application of advanced strategies such as the gene editing technique clustered regularly interspaced short palindromic repeats/CRISPR-associated proteins system (CRISPR/Cas) has provided a rapid, simple, and precise targeting approach for preventing the spread of ARGs in the environment. Carbon-based ENMs (e.g., nitrogen-doped carbon dots, carbon nanotubes) have been shown to facilitate the delivery of CRISPR/Cas into ARB,43,44 achieving sustainable elimination of target ARGs in the soil environment.44 These emerging studies offer promising perspectives on controlling ARGs. However, while the autocatalytic properties of nCeO2 suggest sustained inhibition of HGT without continuous input, its bioavailability may be hindered by aggregation with soil particles and microorganisms. Therefore, further long-term investigations are necessary to determine optimal application details for ENMs to maintain their HGT-inhibiting effect. Additionally, it is crucial to investigate whether the introduction of ENMs or microorganisms may disrupt indigenous microbial communities for environmental safety purposes. Furthermore, developing representative sequences for nano-CRISPR/Cas that can effectively target all ARB would greatly enhance the practicality and applicability of these advancing techniques.
Will the emergence of nano-enabled agriculture combat the crop-related antimicrobial resistance?
Applications of conventional pesticides, fertilizers, and plastics in agricultural practices have contributed to the dissemination of ARGs and ARB in soil–plant systems.45–47 Nano-enabled agriculture has emerged as a sustainable strategy in response to the impending global food crisis.48 Nano-pesticides/fertilizers exhibit a median increase in efficacy of 20–30% compared to conventional products.48 In addition to promoting plant growth, ENMs exhibit great potential in managing biotic stresses by inhibiting pathogen and pest growth, activating host resistance mechanisms, and regulating host metabolism.49 Moreover, ENMs have been shown to shape a healthy rhizosphere environment by enriching beneficial rhizobacteria and inducing root exudates,13 which can contribute to pathogen inhibition.50 The rhizosphere is known as the pathway for ARG and ARB transmission from soil into plants.51 Root exudates comprise both low-molecular weight compounds (e.g., amino acids, organic acids, and phenolics) and high-molecular weight compounds (e.g., mucilage and proteins).13 High molecular weight root exudates have been shown to facilitate the deposition of antibiotics, enhance the expression of ARGs, and promote the growth of ARB in the rhizosphere, while low molecular weight root exudates exhibit the opposite effects.52 Root exposure to nCeO2 can stimulate the secretion of organic acids, amino acids, and phenols (e.g., as citric acid, asparagine, and alpha-linolenic acid), as well as increase the relative abundance of beneficial bacteria (e.g., Altererythrobacter, Gemmatimonas, and Sphingomonas) in the rhizosphere.53 It has been suggested that rhizosphere bacteria may limit the growth of ARB and their transmission into plants through resource competition or antagonism.54 However, the understanding of the interactions between the ENM-affected rhizosphere and ARG/ARB remains limited. Future research should explore whether ENMs can reduce the abundance of ARGs and ARB by regulating low molecular weight root exudates and recruiting ARB-antagonistic rhizobacteria, thereby intercepting the rhizosphere-mediated “transmission pathway” and mitigating the development of antibiotic resistance in plants. Building on our previous findings that nCeO2 efficiently inhibits HGT of ARGs, we recently discovered that exposing roots to nCeO2 can even decrease ARG accumulation in edible parts of hydroponically grown garlic. The reduction can be attributed to an enhanced chemical and physical plant defence system that inhibits the migration of ARB.55 However, it remains unclear whether the plant defence initiated by nCeO2 selectively targets ARB. Additional research is required to elucidate the distribution of bacteria within nCeO2-treated plants following simultaneous exposure to ARB, beneficial bacteria, and pathogenic bacteria. Foliar application of ENMs has been suggested as more efficient and environmentally friendly compared to root exposure, and ENMs can quickly penetrate plant cells through the stomata or cuticle.56 Notably, aerosols carrying ARGs may easily enter the stomatal apertures, representing an additional significant source of ARGs in the phyllosphere.57 Collectively, controlling airborne ARGs in leaves using foliar-applied ENMs and investigating their potential direct interactions with these ARGs should be a key focus for future research. Meanwhile, the uptake and accumulation of ENMs in plants, especially in the edible portions, during both foliar and root applications warrant further evaluation. Our previous study using simulated gastrointestinal digestion experiments has demonstrated the potential ENM accessibility to humans after ingestion of foliar-applied ENMs.58 Thus, ENMs composed of essential nutrients such as carbon, iron, and selenium which exhibit low biotoxicity are considered more favorable. Nevertheless, the long-term cumulative effects and their impact on antibiotic resistance in the human gut microbiome remain largely unknown and require urgent investigation. Additionally, the effects of root-applied ENMs on soil properties, particularly their influence on the soil microbiome, should also be carefully considered. Given that ENM-induced plant innate defence systems promote both resistance and growth, further research should investigate how nano-agricultural practices alleviate ARG contamination while also optimizing different application methods (Fig. 3).
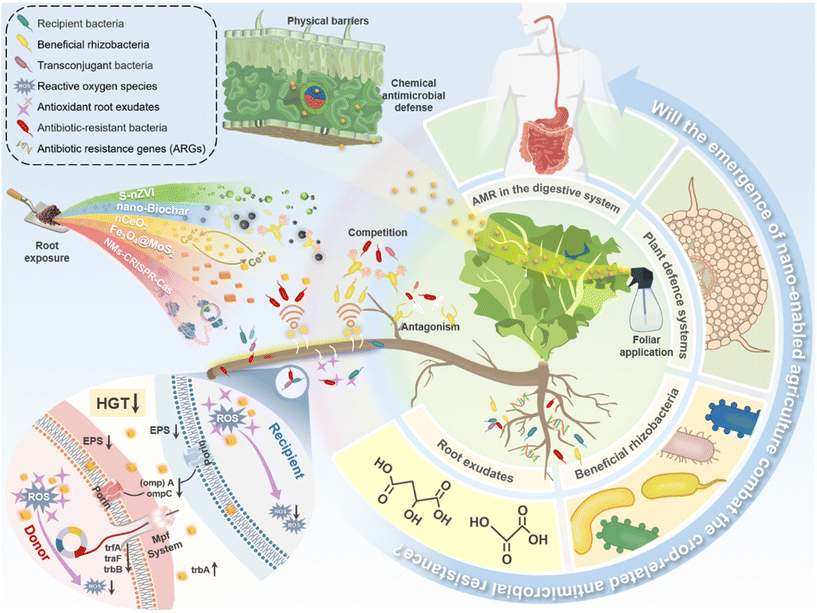 |
| Fig. 3 Engineering nanomaterials for the remediation of ARG contamination in soil–plant systems. The primary mechanisms involved are illustrated in the central ring, while specific mechanisms resulting from root and foliar exposure are detailed in the magnified views on the lower left and upper left sides, respectively. | |
Tailoring ENMs towards One Health: the challenges
While ENMs can be tailored to possess a high surface area, tunable surface chemistry, and specific binding affinity for efficient control of ARG transfer, several challenges and considerations must be addressed. These include potential ecological impacts, long-term stability, and the fate of ENMs in environmental systems. Additionally, the development of sustainable and cost-effective nanomaterial-based solutions requires careful consideration of regulatory frameworks and risk assessment strategies. Considering the emergence of antibiotic resistance in agricultural pathogens and disruption of microbial community balance in soil and on plants due to widespread application of conventional products, long term monitoring of antibiotic resistance in nano-enabled agriculture and human consumption of ENM-treated plants will enhance the bio-functionality of ENMs for agricultural practice. Ongoing research efforts and integration with emerging technologies are essential for advancing our understanding of NM–ARG interactions and developing sustainable solutions to mitigate ARG contamination towards global One Health.
Data availability
No primary research results, software or code have been included and no new data were generated or analysed as part of this perspective.
Author contributions
Feiran Chen: conceptualization, writing, review & editing, funding acquisition. Shuhan Zhang: writing, formal analysis. Xi Wang: review & editing, formal analysis. Zhenyu Wang: conceptualization, funding acquisition, review & editing.
Conflicts of interest
The authors declare no conflicts of interest.
Acknowledgements
This work was supported by the National Natural Science Foundation of China (42192572, 42377252, 42421005), the Natural Science Foundation of Jiangsu Province (BK20230107), and the Qing Lan Project of Jiangsu Province.
References
- J. Wang, L. Wang, L. Zhu, J. Wang and B. Xing, Antibiotic resistance in agricultural soils: source, fate, mechanism and attenuation strategy, Crit. Rev. Environ. Sci. Technol., 2022, 52, 847–889 CrossRef CAS.
- M. Pazda, J. Kumirska, P. Stepnowski and E. Mulkiewicz, Antibiotic resistance genes identified in wastewater treatment plant systems-A review, Sci. Total Environ., 2019, 697, 134023 CrossRef CAS PubMed.
- D. G. J. Larsson and C. F. Flach, Antibiotic resistance in the environment: prioritizing risks, Nat. Rev. Microbiol., 2022, 20, 257–269 CrossRef CAS PubMed.
- A. S. Ezeuko, M. O. Ojemaye, O. O. Okoh and A. I. Okoh, Technological advancement for eliminating antibiotic resistance genes from wastewater: A review of their mechanisms and progress, J. Environ. Chem. Eng., 2021, 9, 106183 CrossRef CAS.
- F. Li, K. Liu, Y. Bao, Y. Li, Z. Zhao, P. Wang and S. Zhan, Molecular level removal of antibiotic resistant bacteria and genes: A review of interfacial chemical in advanced oxidation processes, Water Res., 2024, 254, 121373 CrossRef CAS PubMed.
- P. Chen, X. Guo, S. Li and F. Li, A review of the bioelectrochemical system as an emerging versatile technology for reduction of antibiotic resistance genes, Environ. Int., 2021, 156, 106689 CrossRef CAS PubMed.
- M. Foroughi, M. Khiadani, S. Kakhki, V. Kholghi, K. Naderi and S. Yektay, Effect of ozonation-based disinfection methods on the removal of antibiotic resistant bacteria and resistance genes (ARB/ARGs) in water and wastewater treatment: A systematic review, Sci. Total Environ., 2022, 811, 151404 CrossRef CAS PubMed.
- J. Wang and X. Chen, Removal of antibiotic resistance genes (ARGs) in various wastewater treatment processes: An overview, Crit. Rev. Environ. Sci. Technol., 2022, 52, 571–630 CrossRef CAS.
- N. Zhang, D. Lu, H. Sheng, J. Xia, P. Kan, Z. Yao, H. Chen, G. Li, D. Z. Zhu and H. Liu, Constructed wetlands as hotspots of antibiotic resistance genes and pathogens: Evidence from metagenomic analysis in Chinese rural areas, J. Hazard. Mater., 2023, 447, 130778 CrossRef CAS PubMed.
- Y. Fu, F. Wang, L. Xiang, J. D. Harindintwali, M. Elsner, W. Amelung, S. Kueppers, X. Jiang, M. Virta, M. Sillanpää, A. Schäffer, Y. Zhu and J. M. Tiedje, Combating antibiotic resistance in the human-impacted environment with carbon-based materials: Applications and challenges, Crit. Rev. Environ. Sci. Technol., 2024, 54, 699–721 CrossRef CAS.
- B. Shao, Z. Liu, L. Tang, Y. Liu, Q. Liang, T. Wu, Y. Pan, X. Zhang, X. Tan and J. Yu, The effects of biochar on antibiotic resistance genes (ARGs) removal during different environmental governance processes: A review, J. Hazard. Mater., 2022, 435, 129067 CrossRef CAS PubMed.
- R. Xiao, D. Huang, L. Du, B. Song, L. Yin, Y. Chen, L. Gao, R. Li, H. Huang and G. Zeng, Antibiotic resistance in soil-plant systems: A review of the source, dissemination, influence factors, and potential exposure risks, Sci. Total Environ., 2023, 869, 161855 CrossRef CAS PubMed.
- Z. Wang, L. Yue, O. P. Dhankher and B. Xing, Nano-enabled improvements of growth and nutritional quality in food plants driven by rhizosphere processes, Environ. Int., 2020, 142, 105831 CrossRef CAS PubMed.
- S. Wu, P. Ren, Y. Wu, J. Liu, Q. Huang and P. Cai, Effects of hematite on the dissemination of antibiotic resistance in pathogens and underlying mechanisms, J. Hazard. Mater., 2022, 431, 128537 CrossRef CAS PubMed.
- P. Dong, H. Wang, T. Fang, Y. Wang and Q. Ye, Assessment of extracellular antibiotic resistance genes (eARGs) in typical
environmental samples and the transforming ability of eARG, Environ. Int., 2019, 125, 90–96 CrossRef CAS PubMed.
- C. M. Thomas and K. M. Nielsen, Mechanisms of, and barriers to, horizontal gene transfer between bacteria, Nat. Rev. Microbiol., 2005, 3, 711–721 CrossRef CAS PubMed.
- K. Yu, F. Chen, L. Yue, Y. Luo, Z. Wang and B. Xing, CeO2 nanoparticles regulate the propagation of antibiotic resistance genes by altering cellular contact and plasmid transfer, Environ. Sci. Technol., 2020, 54, 10012–10021 CrossRef CAS PubMed.
- Y. Xu, H. Du, C. Wang, L. Yue, F. Chen and Z. Wang, CeO2 Nanoparticles-regulated plasmid uptake and bioavailability for reducing transformation of extracellular antibiotic resistance genes, Nanomaterials, 2023, 13, 969 CrossRef CAS PubMed.
- S. Zhang, Y. Wang, H. Song, J. Lu, Z. Yuan and J. Guo, Copper nanoparticles and copper ions promote horizontal transfer of plasmid-mediated multi-antibiotic resistance genes across bacterial genera, Environ. Int., 2019, 129, 478–487 CrossRef CAS PubMed.
- X. Han, P. Lv, L. G. Wang, F. Long, X. L. Ma, C. Liu, Y. J. Feng, M. F. Yang and X. Xiao, Impact of nano-TiO2 on horizontal transfer of resistance genes mediated by filamentous phage transduction, Environ. Sci.: Nano, 2020, 7, 1214–1224 RSC.
- S. Zhang, J. Lu, Y. Wang, W. Verstraete, Z. Yuan and J. Guo, Insights of metallic nanoparticles and ions in accelerating the bacterial uptake of antibiotic resistance genes, J. Hazard. Mater., 2022, 421, 126728 CrossRef CAS PubMed.
- Q. Ji, C. Zhang and D. Li, Influences and mechanisms of nanofullerene on the horizontal transfer of plasmid-encoded antibiotic resistance genes between E. coli strains, Front. Environ. Sci. Eng., 2020, 14, 108 CrossRef CAS.
- Q. L. Chen, D. Zhu, X. L. An, J. Ding, Y. G. Zhu and L. Cui, Does nano silver promote the selection of antibiotic resistance genes in soil and plant?, Environ. Int., 2019, 128, 399–406 CrossRef CAS PubMed.
- X. Wang, F. Yang, J. Zhao, Y. Xu, D. Mao, X. Zhu, Y. Luo and P. J. J. Alvarez, Bacterial exposure to ZnO nanoparticles facilitates horizontal transfer of antibiotic resistance genes, NANO, 2018, 10, 61–67 Search PubMed.
- C. Ding, M. Jin, J. Ma, Z. Chen, Z. Shen, D. Yang, D. Shi, W. Liu, M. Kang, J. Wang, J. Li and Z. Qiu, Nano-Al2O3 can mediate transduction-like transformation of antibiotic resistance genes in water, J. Hazard. Mater., 2021, 405, 124224 CrossRef CAS PubMed.
- F. Lian, W. Yu, Q. Zhou, S. Gu, Z. Wang and B. Xing, Size Matters: Nano-biochar triggers decomposition and transformation inhibition of antibiotic resistance genes in aqueous rnvironments, Environ. Sci. Technol., 2020, 54, 8821–8829 CrossRef CAS PubMed.
- K. Ouyang, M. Mortimer, P. A. Holden, P. Cai, Y. Wu, C. Gao and Q. Huang, Towards a better understanding of Pseudomonas putida biofilm formation in the presence of ZnO nanoparticles (NPs): Role of NP concentration, Environ. Int., 2020, 137, 105485 CrossRef CAS PubMed.
- Y. Chen, X. Guo, J. Feng, D. Lu, Z. Niu, F. Tou, L. Hou, M. Liu and Y. Yang, Impact of ZnO nanoparticles on the antibiotic resistance genes (ARGs) in estuarine water: ARG variations and their association with the microbial community, Environ. Sci.: Nano, 2019, 6, 2405–2419 RSC.
- M. T. Guo and G. S. Zhang, Graphene oxide in the water environment could affect tetracycline-antibiotic resistance, Chemosphere, 2017, 183, 197–203 CrossRef CAS PubMed.
- X. Hu, Y. Xu, S. Liu, F. O. Gudda, W. Ling, C. Qin and Y. Gao, Graphene quantum dots nonmonotonically influence the horizontal transfer of extracellular antibiotic resistance genes via bacterial transformation, Small, 2023, 19, 2301177 CrossRef CAS PubMed.
- W. Z. Zhang, J. F. Gao, W. J. Duan, D. Zhang, J. X. Jia and Y. W. Wang, Sulfidated nanoscale zero-valent iron is an efficient material for the removal and regrowth inhibition of antibiotic resistance genes, Environ. Pollut., 2020, 263, 114508 CrossRef CAS PubMed.
- X. Lu, J. Hou, K. Yang, L. Zhu, B. Xing and D. Lin, Binding force and site-determined desorption and fragmentation of antibiotic resistance genes from metallic nanomaterials, Environ. Sci. Technol., 2021, 55, 9305–9316 CrossRef CAS PubMed.
- W. Yu, S. Zhan, Z. Shen, Q. Zhou and D. Yang, Efficient removal mechanism for antibiotic resistance genes from aquatic environments by graphene oxide nanosheet, Chem. Eng. J., 2017, 313, 836–846 CrossRef CAS.
- S. Zhou, H. Li, Z. Wu, S. Li, Z. Cao, B. Ma, Y. Zou, N. Zhang, Z. Liu, Y. Wang, X. Liao and Y. Wu, The addition of nano zero-valent iron during compost maturation effectively removes intracellular and extracellular antibiotic resistance genes by reducing the abundance of potential host bacteria, Bioresour. Technol., 2023, 384, 129350 CrossRef CAS PubMed.
- J. Fang, W. Li, Y. Tian, Z. Chen, Y. Yu, S. Shan, V. D. Rajput, S. Srivastava and D. Lin, Pyrolysis temperature affects the inhibitory mechanism of biochars on the mobility of extracellular antibiotic resistance genes in saturated porous media, J. Hazard. Mater., 2022, 439, 129668 CrossRef CAS PubMed.
- Y. Wang, J. Gao, W. Duan, W. Zhang, Y. Zhao and J. Liu, Inactivation of sulfonamide antibiotic resistant bacteria and control of intracellular antibiotic resistance transmission risk by sulfide-modified nanoscale zero-valent iron, J. Hazard. Mater., 2020, 400, 123226 CrossRef CAS PubMed.
- H. Wang, H. Qi, S. Gong, Z. Huang, C. Meng, Y. Zhang, X. Chen and X. Jiao, Fe3O4 composited with MoS2 blocks horizontal gene transfer, Colloids Surf., B, 2020, 185, 110569 CrossRef CAS PubMed.
- Y. Cheng, H. Dong, Y. Lu, K. Hou, Y. Wang, Q. Ning, L. Li, B. Wang, L. Zhang and G. Zeng, Toxicity of sulfide-modified nanoscale zero-valent iron to Escherichia coli in aqueous solutions, Chemosphere, 2019, 220, 523–530 CrossRef CAS PubMed.
- G. Schröder and E. Lanka, The mating pair formation system of conjugative plasmids-A versatile secretion machinery for transfer of proteins and DNA, Plasmid, 2005, 54, 1–25 CrossRef PubMed.
- H. Li, R. Song, Y. Wang, R. Zhong, Y. Zhang, J. Zhou, T. Wang, H. Jia and L. Zhu, Inhibited conjugative transfer of antibiotic resistance genes in antibiotic resistant bacteria by surface plasma, Water Res., 2021, 204, 117630 CrossRef CAS PubMed.
- G. Pulido-Reyes, I. Rodea-Palomares, S. Das, T. S. Sakthivel, F. Leganes, R. Rosal, S. Seal and F. Fernandez-Pinas, Untangling the biological effects of cerium oxide nanoparticles: the role of surface valence states, Sci. Rep., 2015, 5, 15613 CrossRef CAS PubMed.
- M. Duan, Z. Li, R. Yan, B. Zhou, L. Su, M. Li, H. Xu and Z. Zhang, Mechanism for combined application of biochar and Bacillus cereus to reduce antibiotic resistance genes in copper contaminated soil and lettuce, Sci. Total Environ., 2023, 884, 163422 CrossRef CAS PubMed.
- K. Yu, Z. Cheng, Y. Sun, H. Ren, H. Chen and Y. Xia, Eliminated colistin-resistance dissemination by a carbon nanotube-mediated CRISPR/Cas9 system – a combined effect of curing plasmid and conjugation blocking, Environ. Sci.: Nano, 2023, 10, 2387–2398 RSC.
- F. Chen, H. Du, M. Tao, L. Xu, C. Wang, J. C. White, Z. Wang and B. Xing, Nitrogen-doped carbon dots facilitate CRISPR/Cas for reducing antibiotic resistance genes in the environment, J. Agric. Food Chem., 2024, 72, 3397–3405 CrossRef CAS PubMed.
-
FAO and WHO, Joint FAO/WHO expert meeting in collaboration with OIE on foodborne antimicrobial resistance: role of the environment, crops and biocides-Meeting report, Microbiological Risk Assessment Series no. 34. Rome, 2019 Search PubMed.
- L. Liu, Y. Sun, S. Du, Y. Li and J. Wang, Nanoplastics promote the dissemination of antibiotic resistance genes and diversify their bacterial hosts in soil, Eco-Environ. Health, 2024, 3, 1–10 CrossRef PubMed.
- H. Liao, X. Li, Q. Yang, Y. Bai, P. Cui, C. Wen, C. Liu, Z. Chen, J. Tang, J. Che, Z. Yu, S. Geisen, S. Zhou, V. P. Friman and Y. G. Zhu, Herbicide selection promotes antibiotic resistance in soil microbiomes, Mol. Biol. Evol., 2021, 38, 2337–2350 CrossRef CAS PubMed.
- M. Kah, R. S. Kookana, A. Gogos and T. D. Bucheli, A critical evaluation of nanopesticides and nanofertilizers against their conventional analogues, Nat. Nanotechnol., 2018, 13, 677–684 CrossRef CAS PubMed.
- X. Cao and Z. Wang, Application of nano-agricultural technology for biotic stress management: mechanisms, optimization, and future perspectives, Environ. Sci.: Nano, 2022, 9, 4336–4353 RSC.
- J. Yuan, N. Zhang, Q. Huang, W. Raza, R. Li, J. M. Vivanco and Q. Shen, Organic acids from root exudates of banana help root colonization of PGPR strain Bacillus amyloliquefaciens NJN-6, Sci. Rep., 2015, 5, 13438 CrossRef CAS PubMed.
- N. Scaccia, I. Vaz-Moreira and C. M. Manaia, The risk of transmitting antibiotic resistance through endophytic bacteria, Trends Plant Sci., 2021, 26, 1213–1226 CrossRef CAS PubMed.
- M. Zhang, Z. Cai, G. Zhang, D. Zhang and X. Pan, Abiotic mechanism changing tetracycline resistance in root mucus layer of floating plant: The role of antibiotic-exudate complexation, J. Hazard. Mater., 2021, 416, 125728 CrossRef CAS PubMed.
- M. Zhao, F. Chen, X. Li, C. Wang, X. Cao, L. Jiao, L. Yue and Z. Wang, Rhizosphere regulation with cerium oxide nanomaterials promoted carrot taproot thickening, Environ. Sci.: Nano, 2024, 11, 3359–3373 RSC.
- H. Xu, Z. Chen, R. Huang, Y. Cui, Q. Li, Y. Zhao, X. Wang, D. Mao, Y. Luo and H. Ren, Antibiotic resistance gene-carrying plasmid spreads into the plant endophytic bacteria using soil bacteria as carriers, Environ. Sci. Technol., 2021, 55, 10462–10470 CrossRef CAS PubMed.
- Y. Xu, M. Tao, W. Xu, L. Xu, L. Yue, X. Cao, F. Chen and Z. Wang, Nano-CeO2 activates physical and chemical defenses of garlic (Allium sativum L.) for reducing antibiotic resistance genes in plant endosphere, Ecotoxicol. Environ. Saf., 2024, 276, 116289 CrossRef CAS PubMed.
- M. Wang, C. Mu, X. Lin, W. Ma, H. Wu, D. Si, C. Ge, C. Cheng, L. Zhao, H. Li and D. Zhou, Foliar application of nanoparticles reduced cadmium content in wheat (Triticum aestivum L.) grains via long-distance “leaf–root–microorganism” regulation, Environ. Sci. Technol., 2024, 58, 6900–6912 CrossRef CAS PubMed.
- P. Chen, K. Yu and Y. He, The dynamics and transmission of antibiotic resistance associated with plant microbiomes, Environ. Int., 2023, 176, 107986 CrossRef PubMed.
- J. Wang, L. Yue, J. Zhao, X. Cao, C. Wang, F. Chen, Z. Xiao, Y. Feng and Z. Wang, Uptake and bioaccumulation of nanoparticles by five higher plants using single-particle-inductively coupled plasma-mass spectrometry, Environ. Sci.: Nano, 2022, 9, 3066–3080 RSC.
|
This journal is © The Royal Society of Chemistry 2025 |
Click here to see how this site uses Cookies. View our privacy policy here.